Abstract
1. The properties of whole-cell and single-channel Na+ and K+ currents in immunocytochemically identified bovine lactotrophs were studied using the patch-clamp technique. 2. In the whole-cell, current-clamp mode, cells had membrane potentials of -94.7 +/- 6.7 mV and input resistances of 2-17 G omega. Current-induced action potentials were recorded with a threshold around -35 mV and amplitude of 40-65 mV. Repetitive firing was not sustained at frequencies greater than 1-2 Hz without total inactivation. 3. Under voltage clamp, action potentials were shown to be composed of an inward TTX-sensitive Na+ current and an outward K+ current that was abolished by internal Cs+. 4. The isolated Na+ current had a threshold for activation around -35 mV and rapidly inactivated to a steady state during a test voltage pulse. Inactivation was strongly voltage-dependent, with the Na+ current being half-inactivated at -20 mV. 5. Recovery from inactivation was voltage dependent and at a holding potential of -60 mV, 50% reactivation was achieved after 420 ms. The implications of this long reactivation time on sustained action potential frequency are discussed. 6. Single Na+ channel activity was examined with the outside-out patch configuration and yielded single-channel conductances of 22.5 pS. Reconstruction of the voltage and time dependence of single-channel currents provided an accurate picture of the whole-cell Na+ current. 7. Whole-cell outward current carried by K+ in the absence of Na+ and Ca2+ had a large conductance, was slowly activated and demonstrated no inactivation. A second, more rapidly activating Ca2+-dependent K+ current could also be demonstrated. 8. Ensemble analysis of whole-cell K+ currents in the absence of Ca2+ showed underlying single-channel amplitudes of 1.2 pA at +10 mV, with the lactotroph having about 350 active channels at this potential. 9. Recordings of single K+ channels also demonstrated two classes of channel: a small (50 pS) voltage-activated channel and a higher-conductance (100 pS) Ca2+- sensitive channel. 10. Prolactin secretion was shown to be TTX-insensitive but sensitive to membrane potential, demonstrated as increased release following increased external K+ but not Na+ concentration.(ABSTRACT TRUNCATED AT 400 WORDS)
Full text
PDF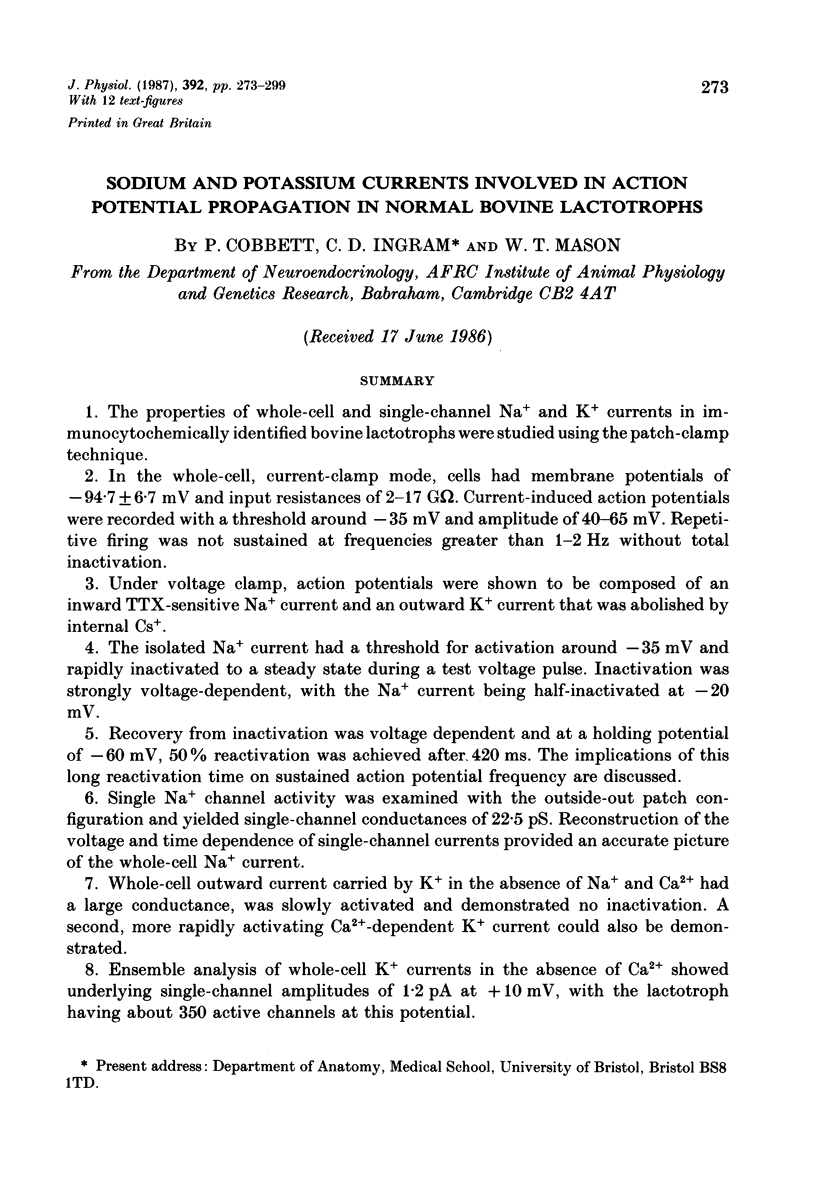
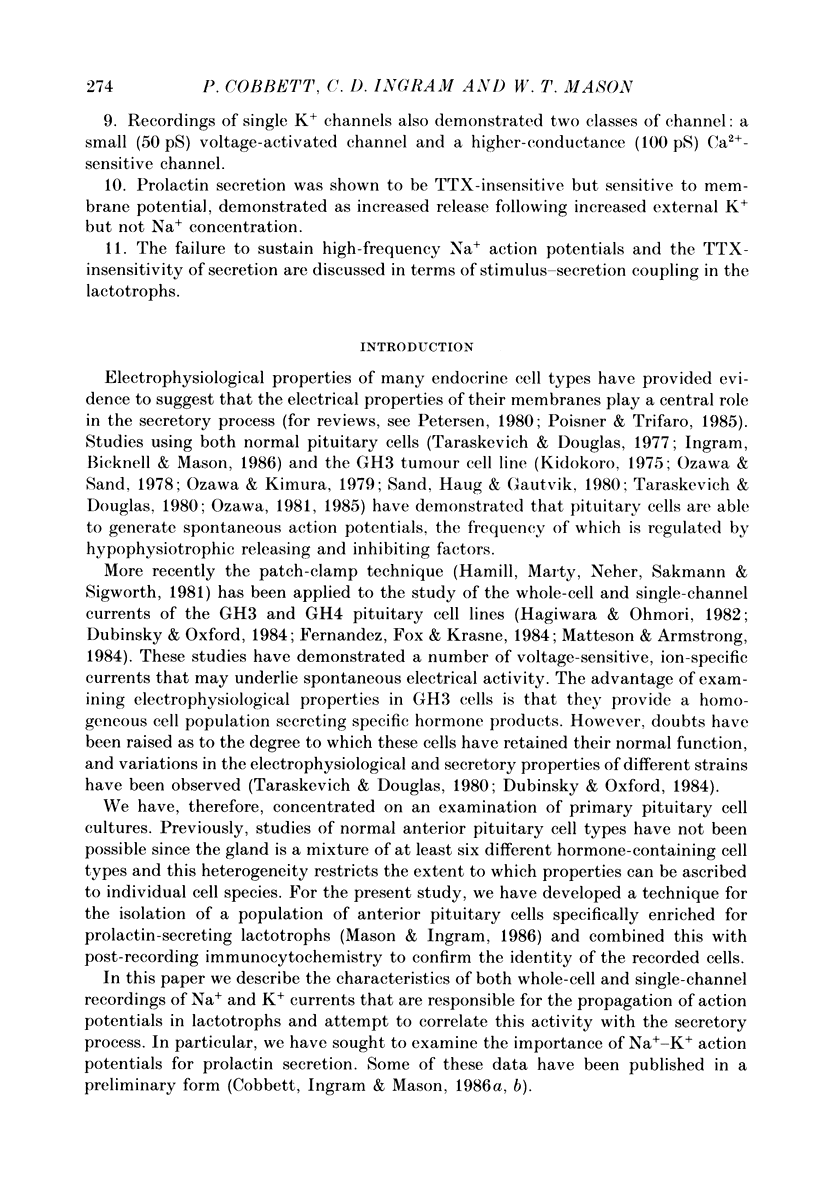
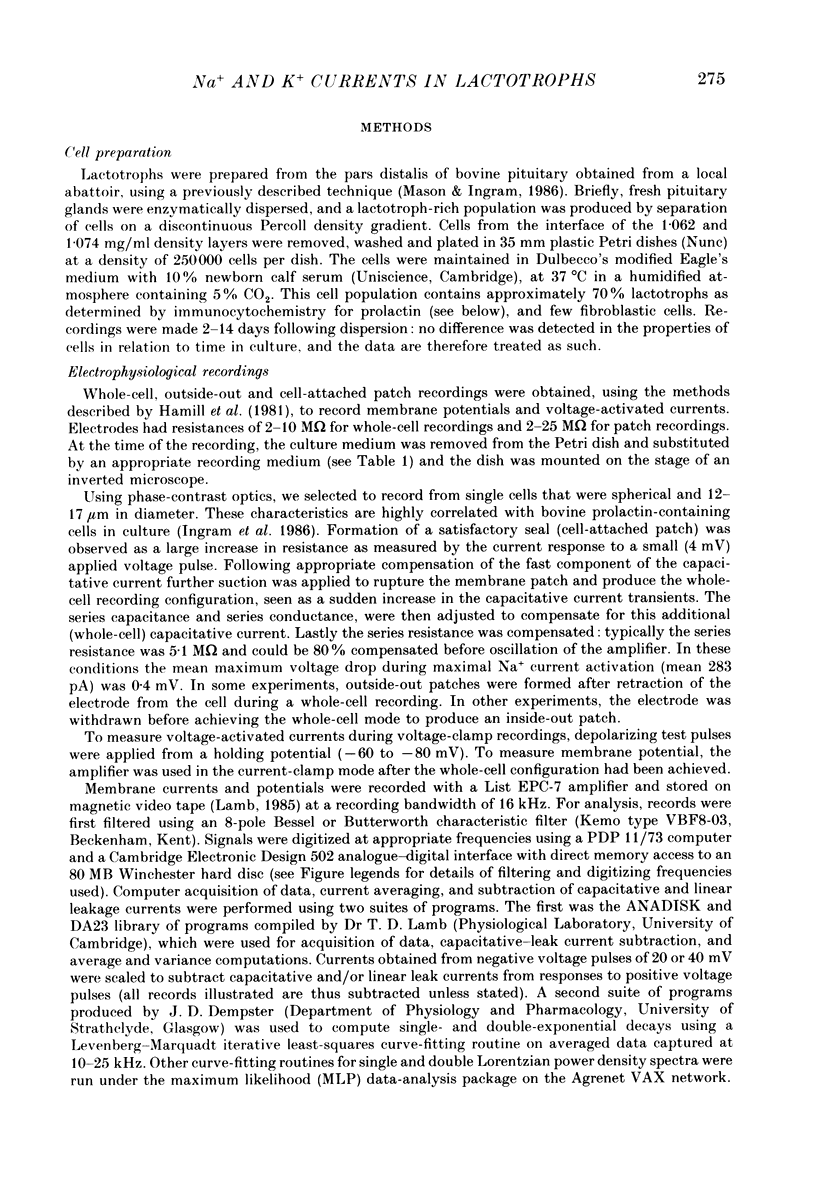
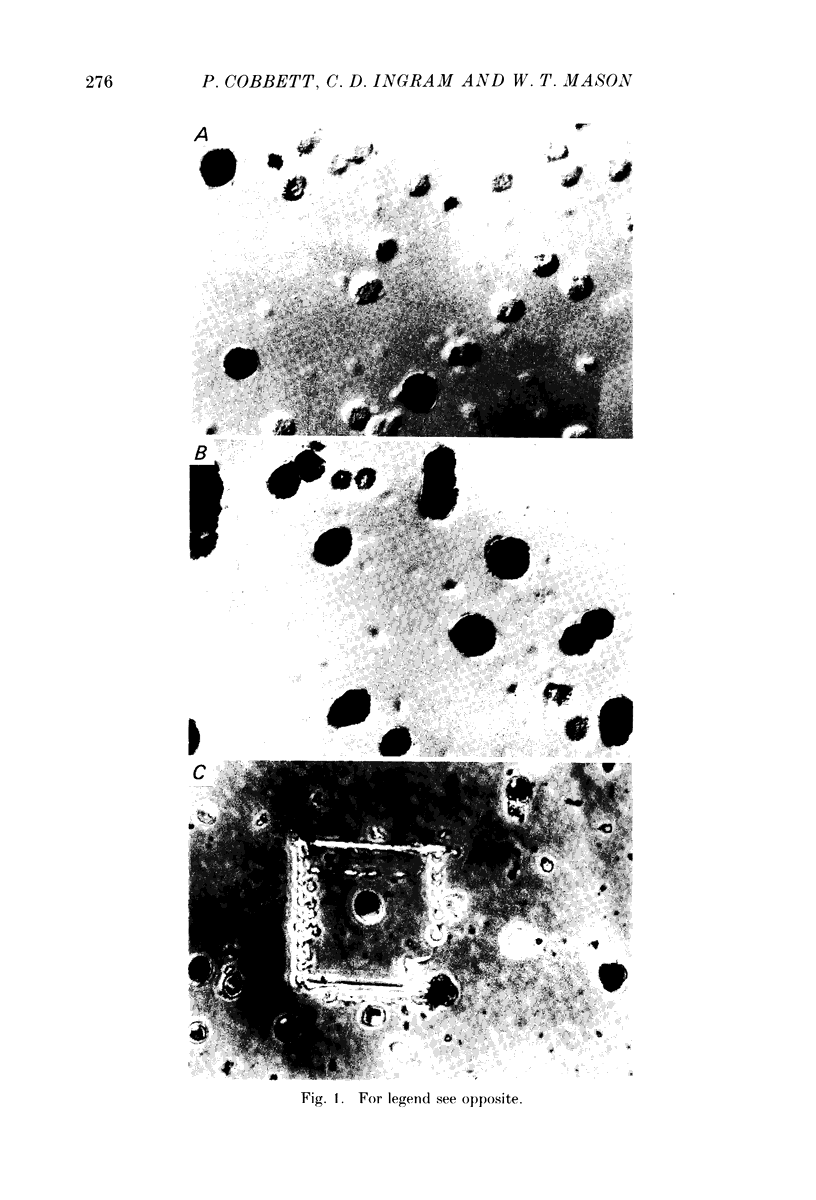
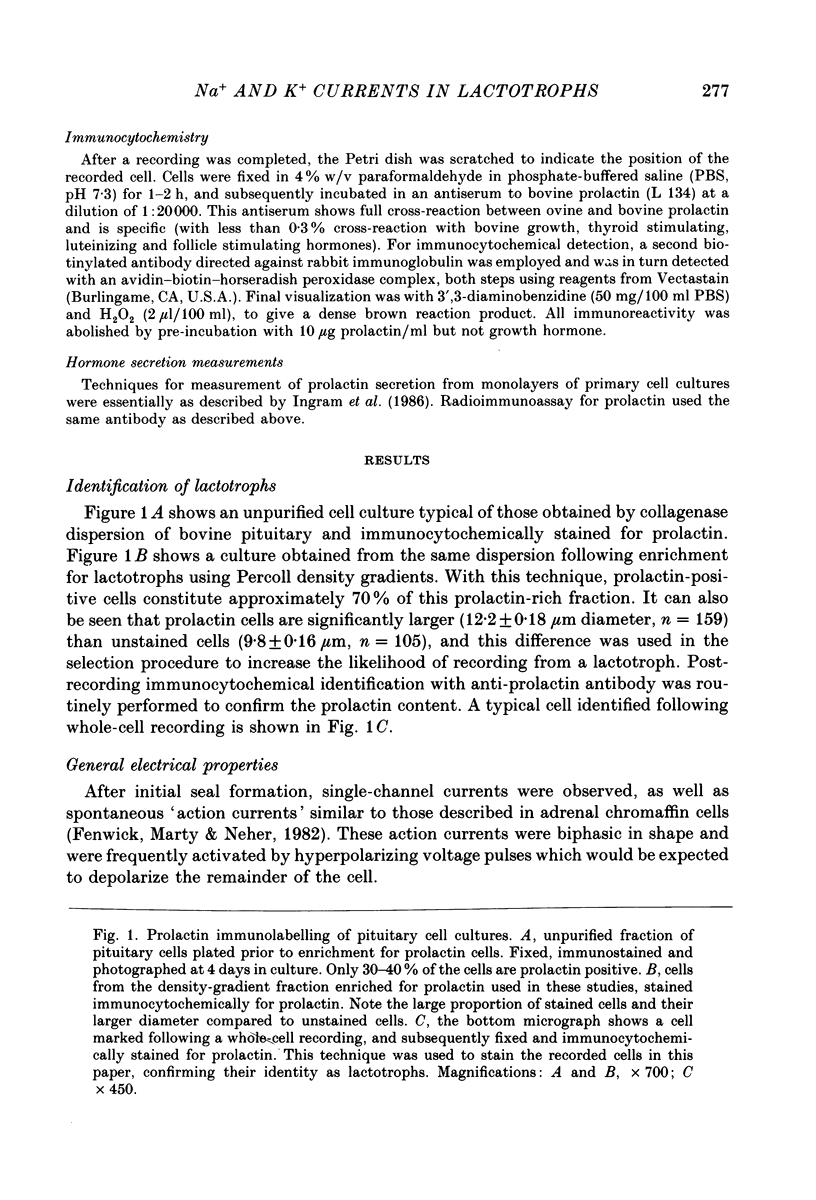
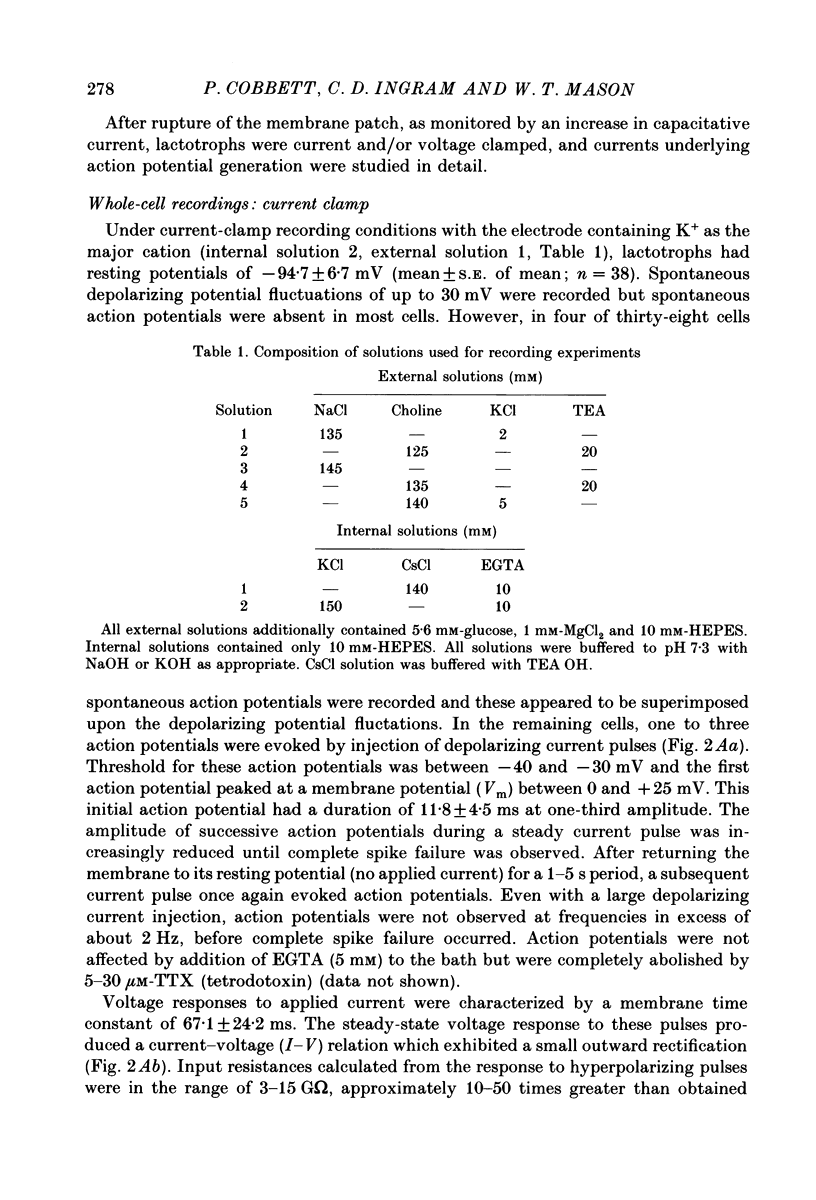
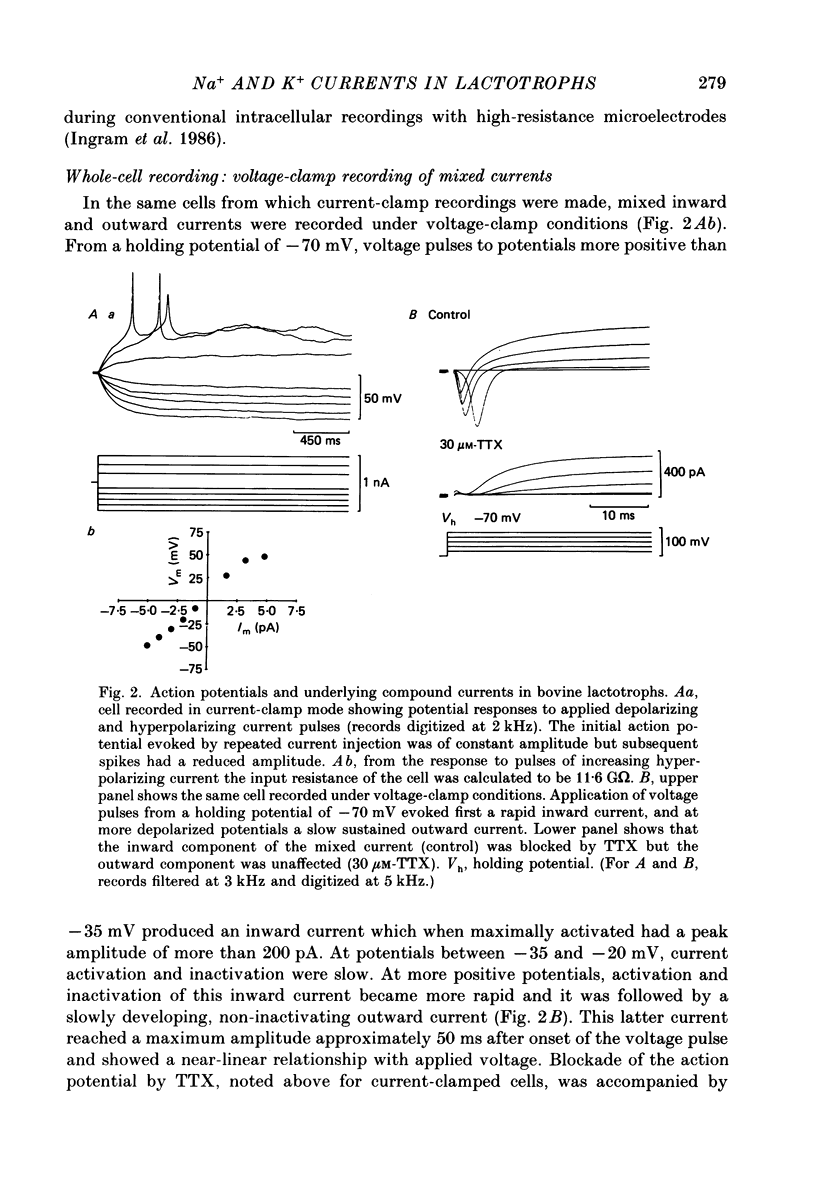
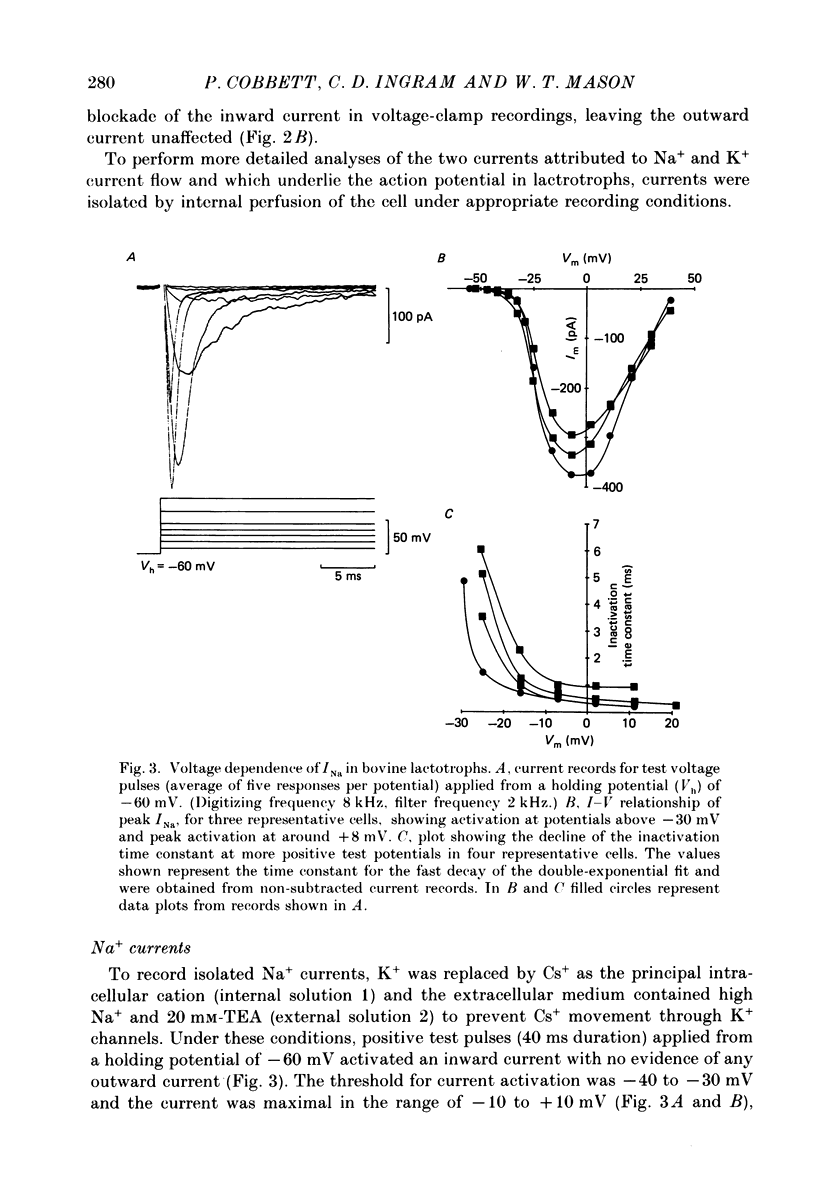
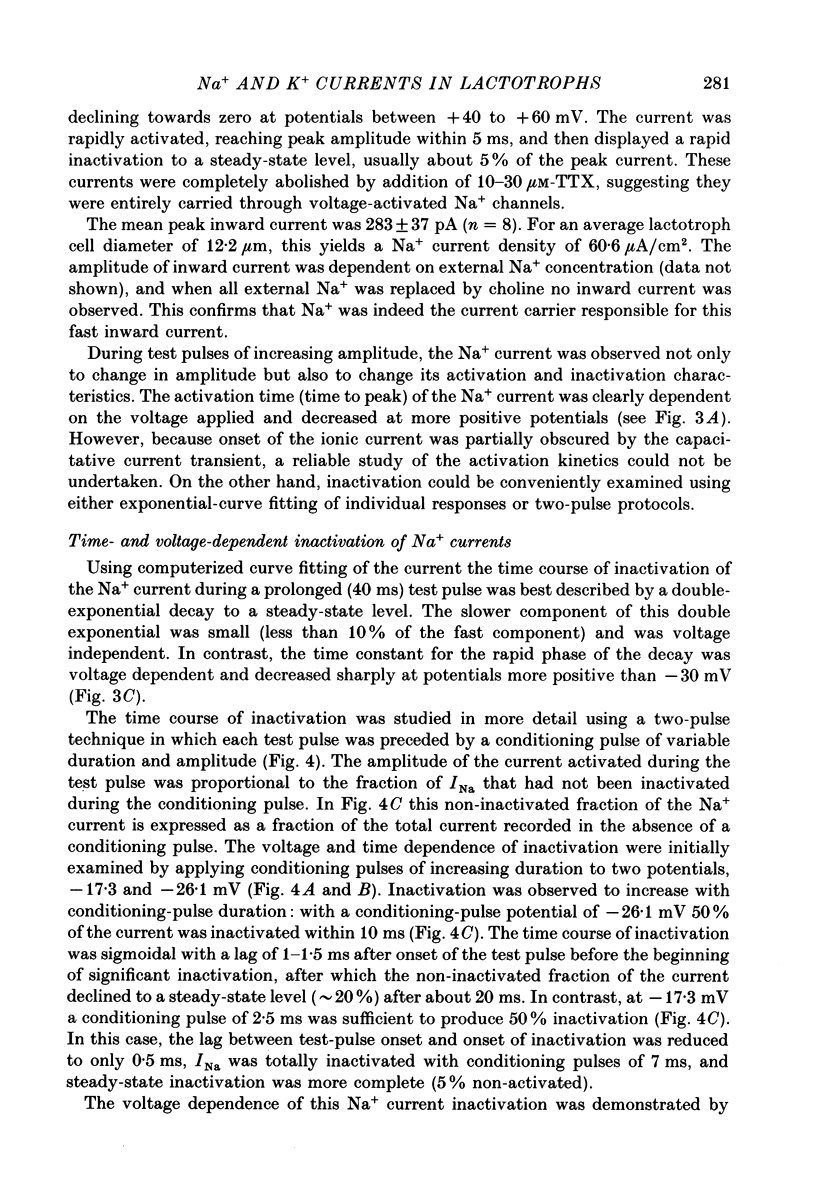
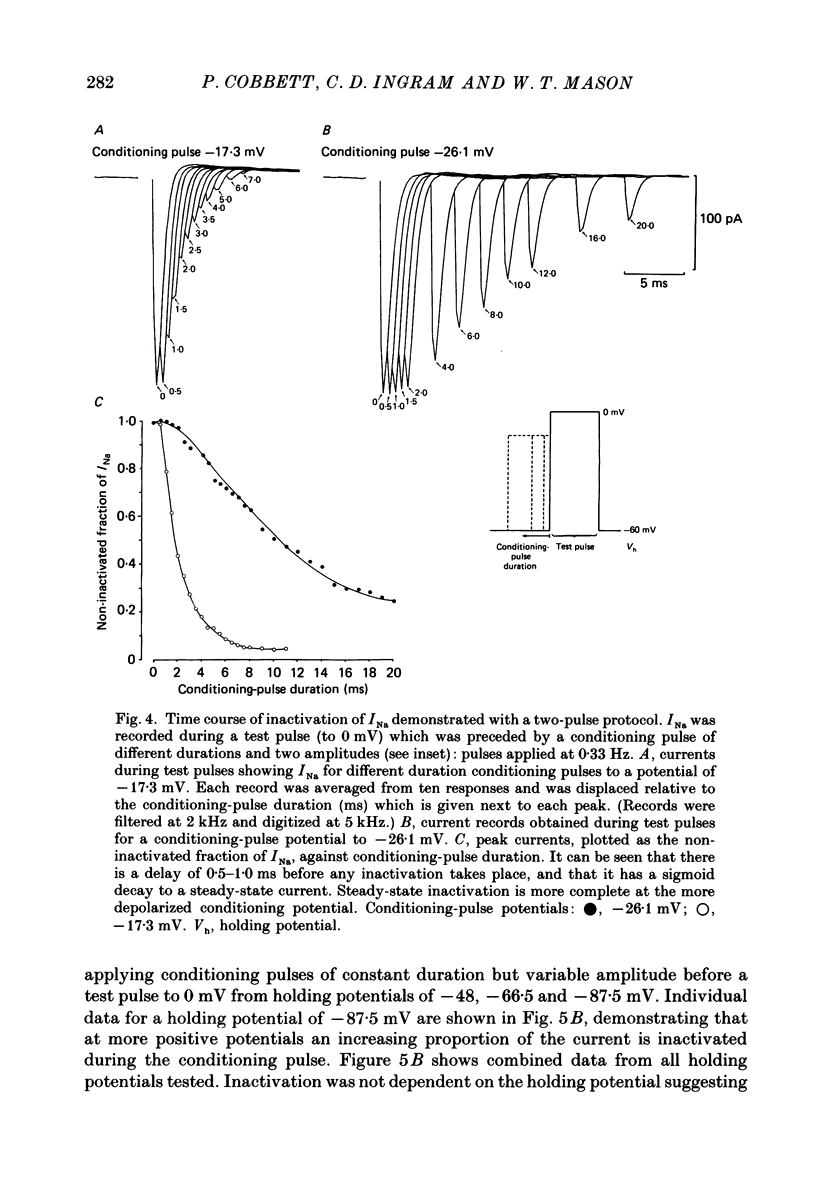
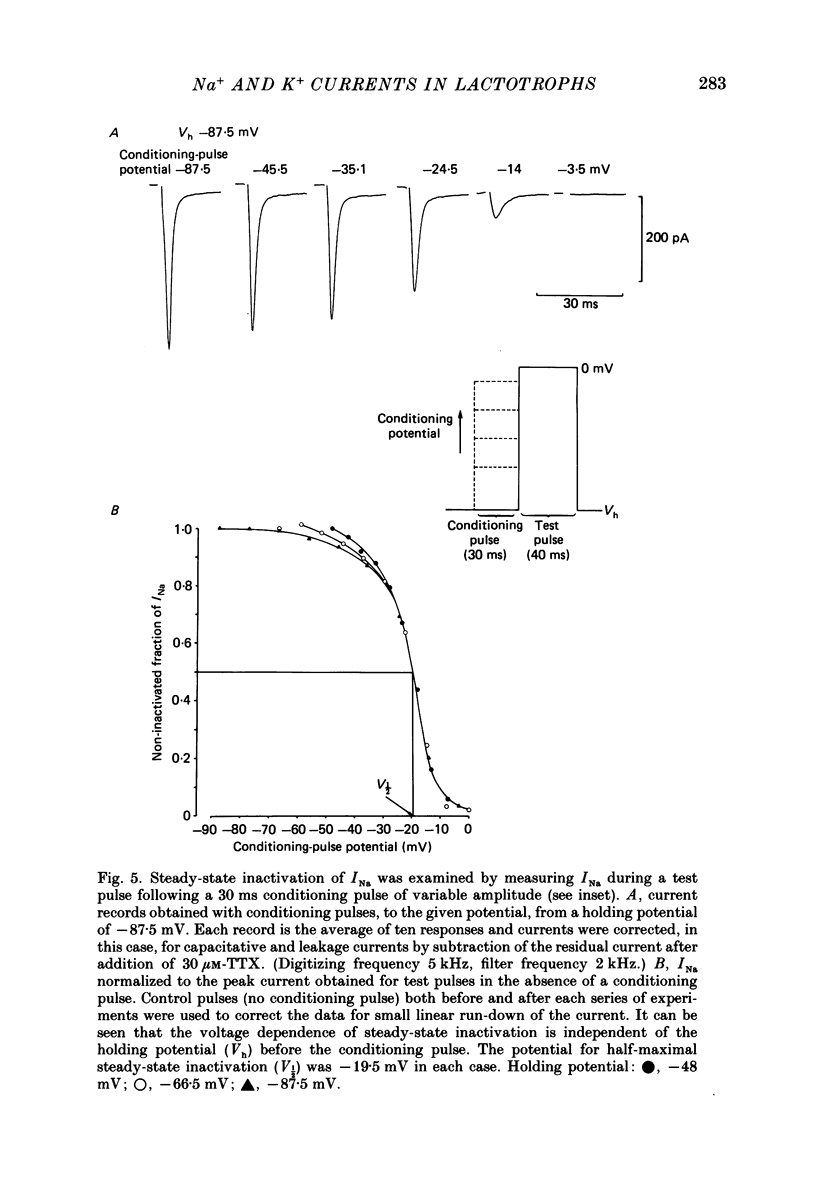
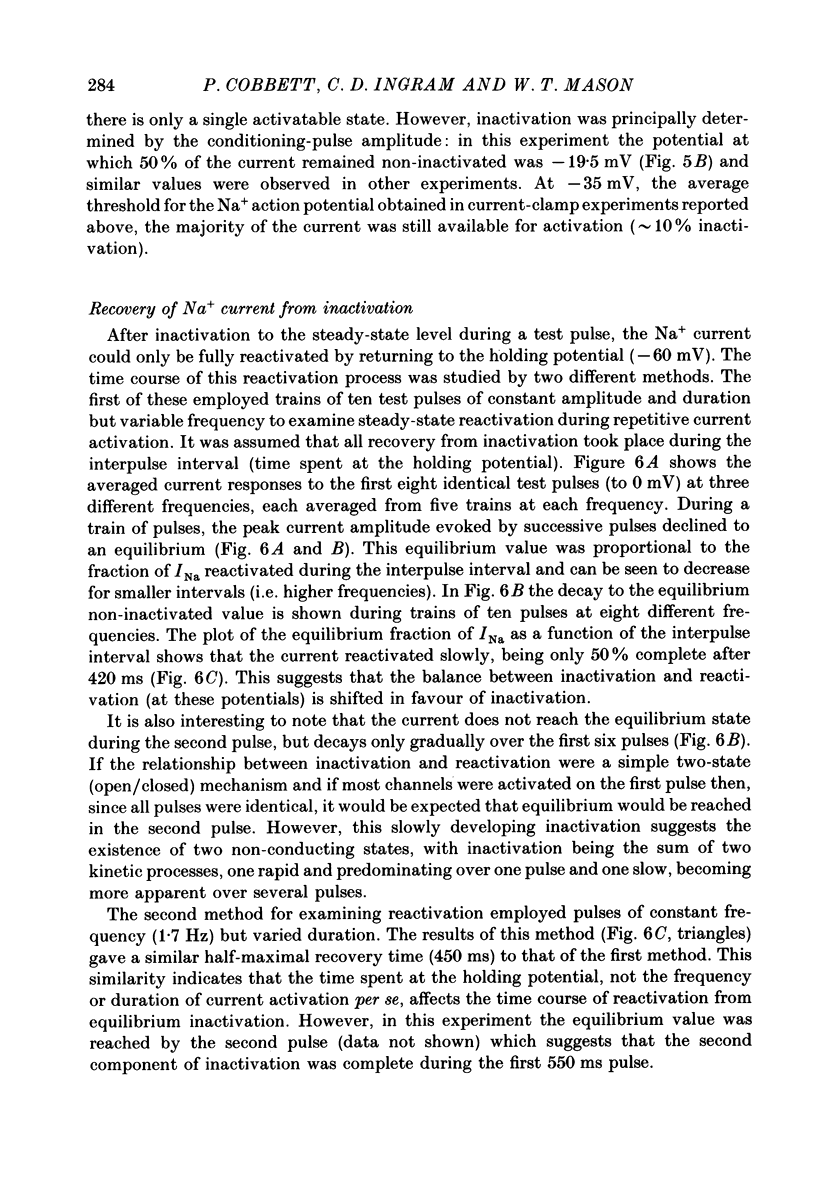
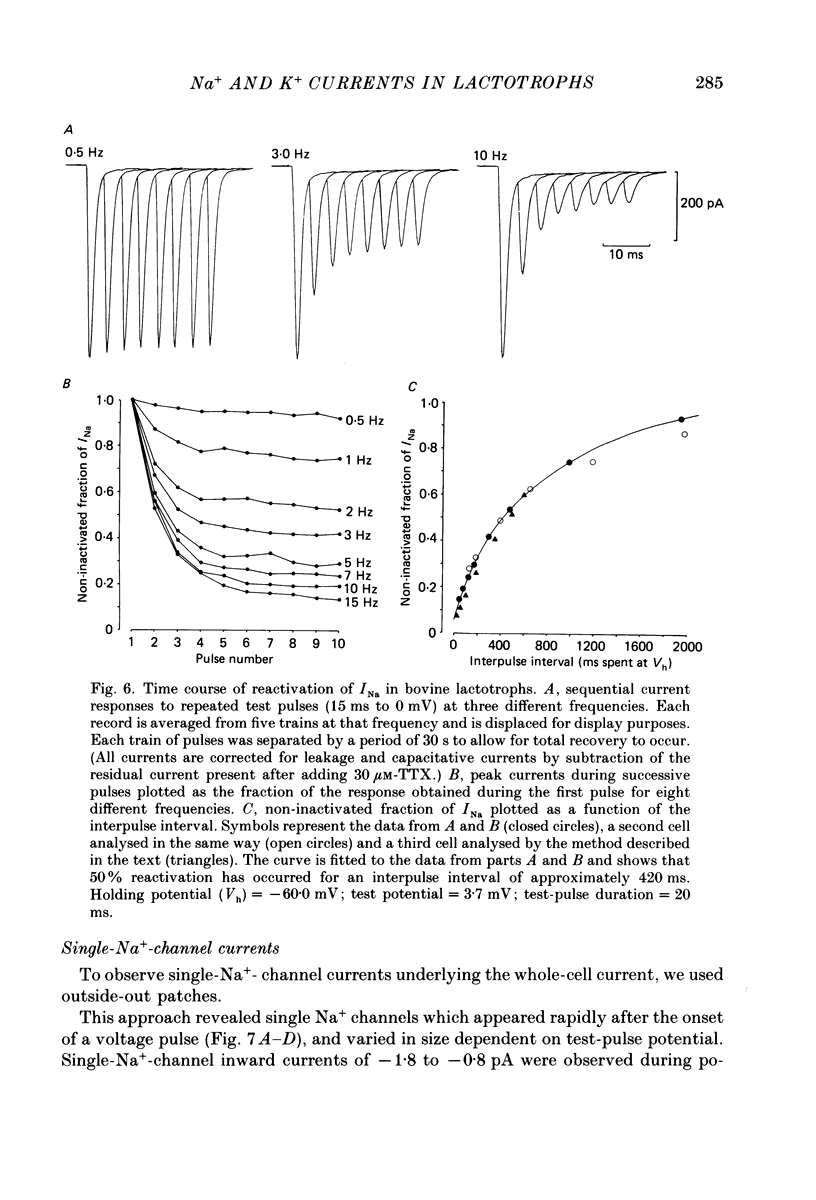
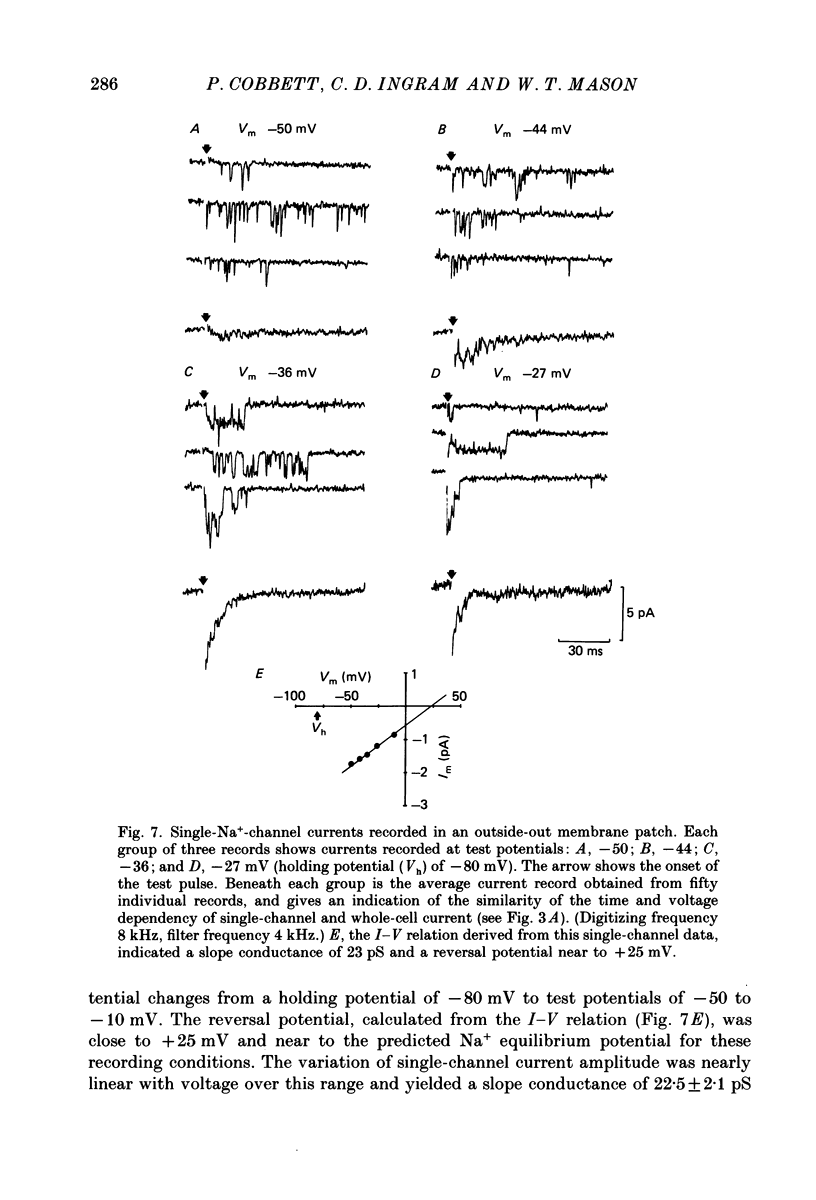
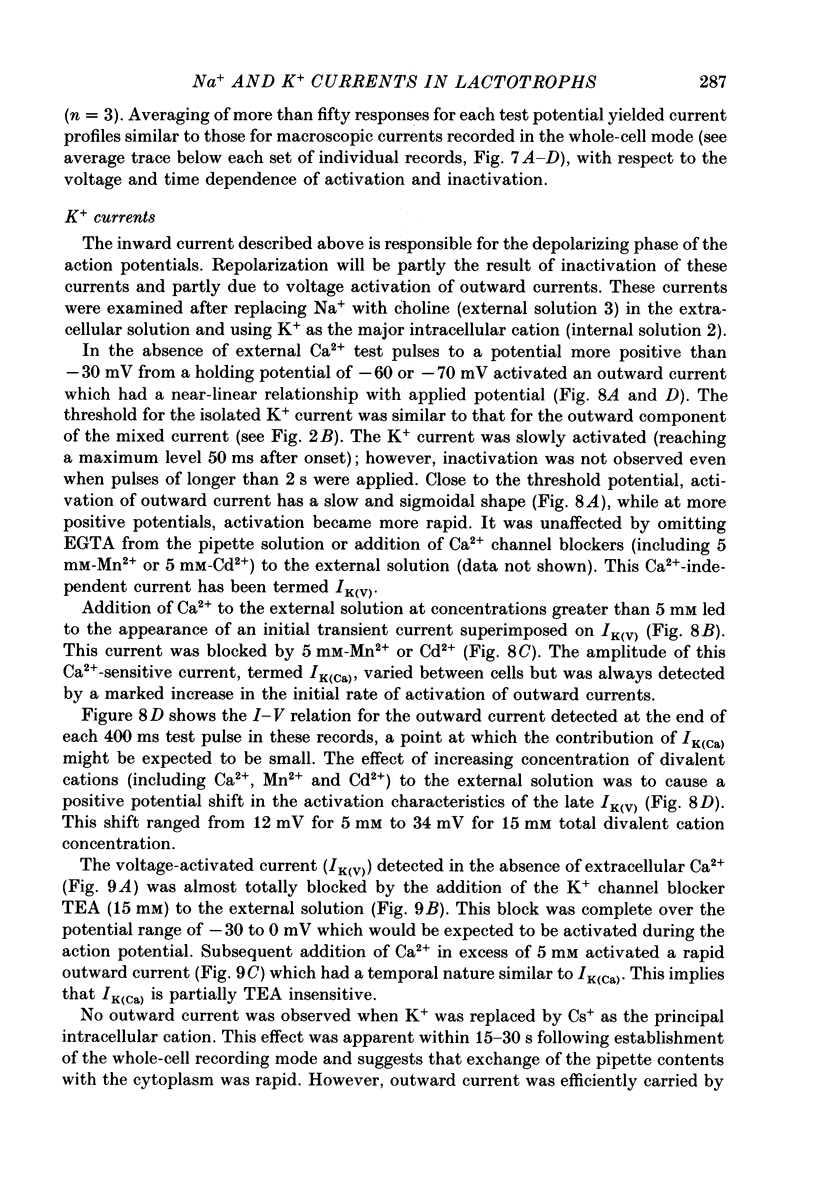
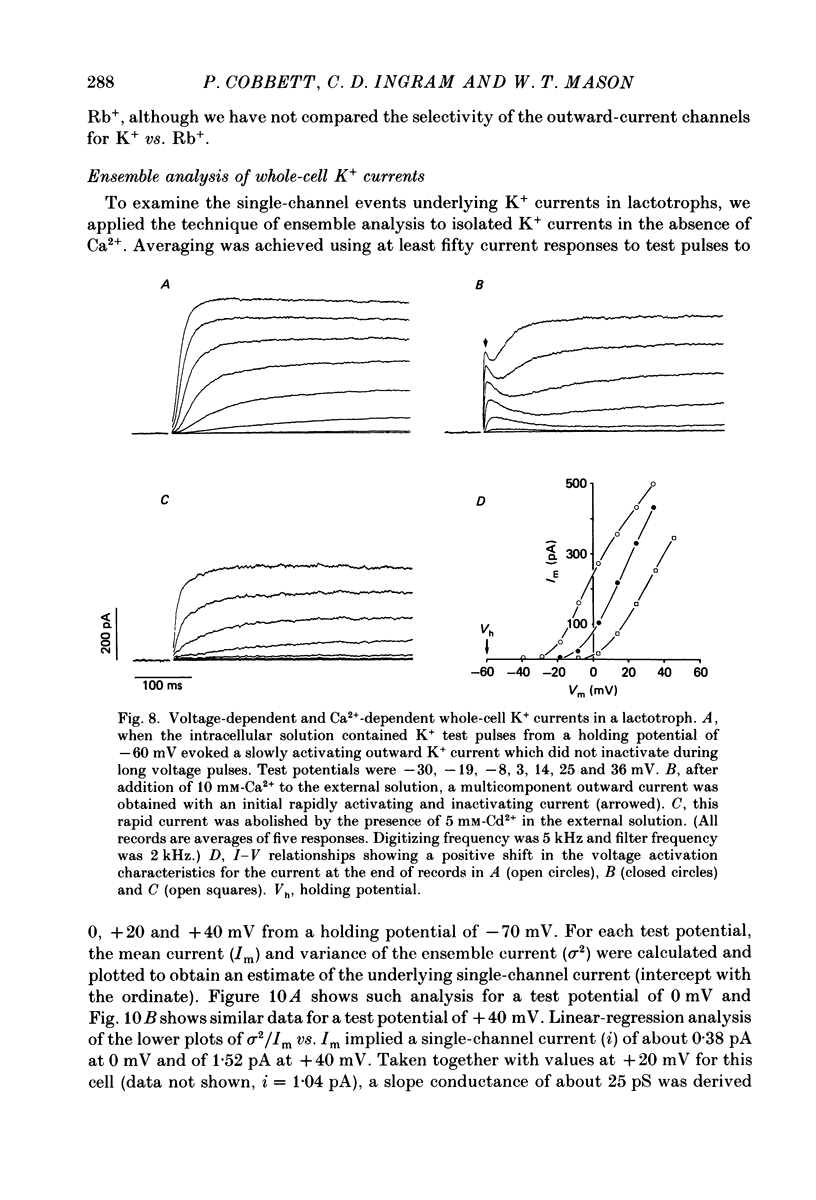
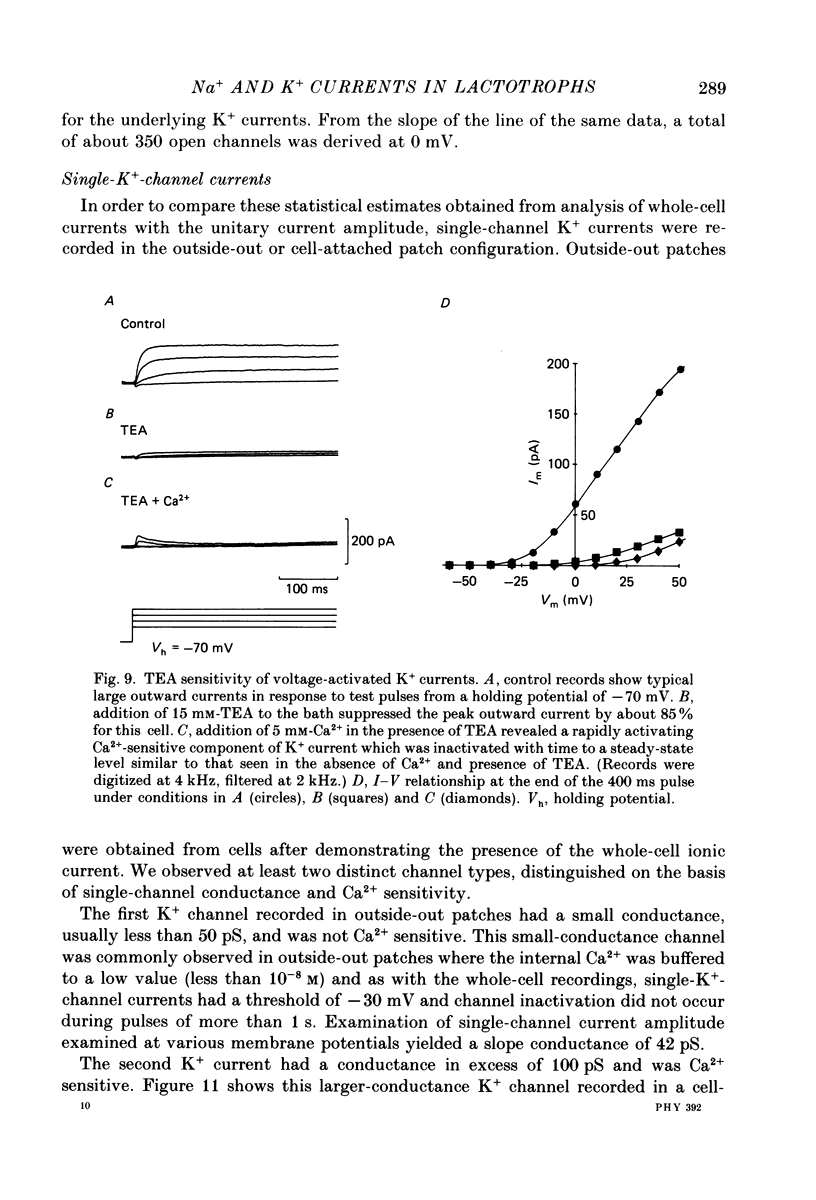
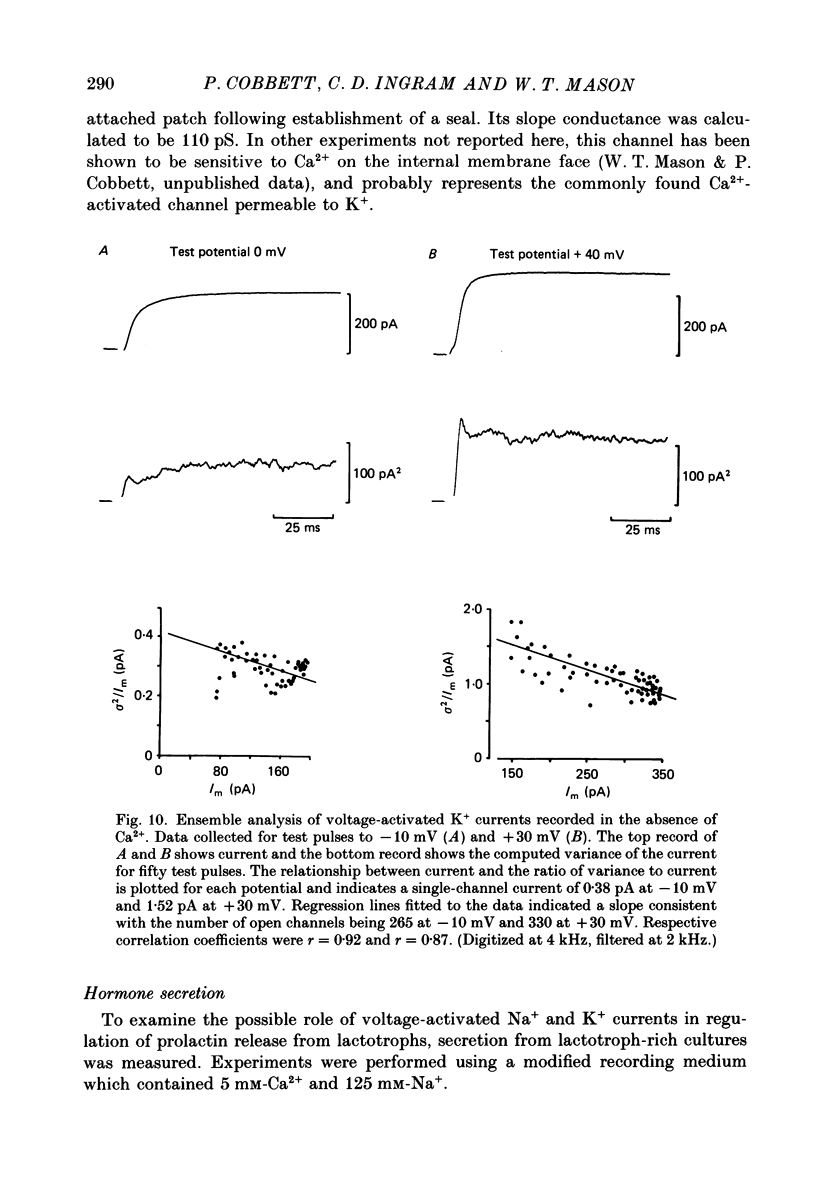
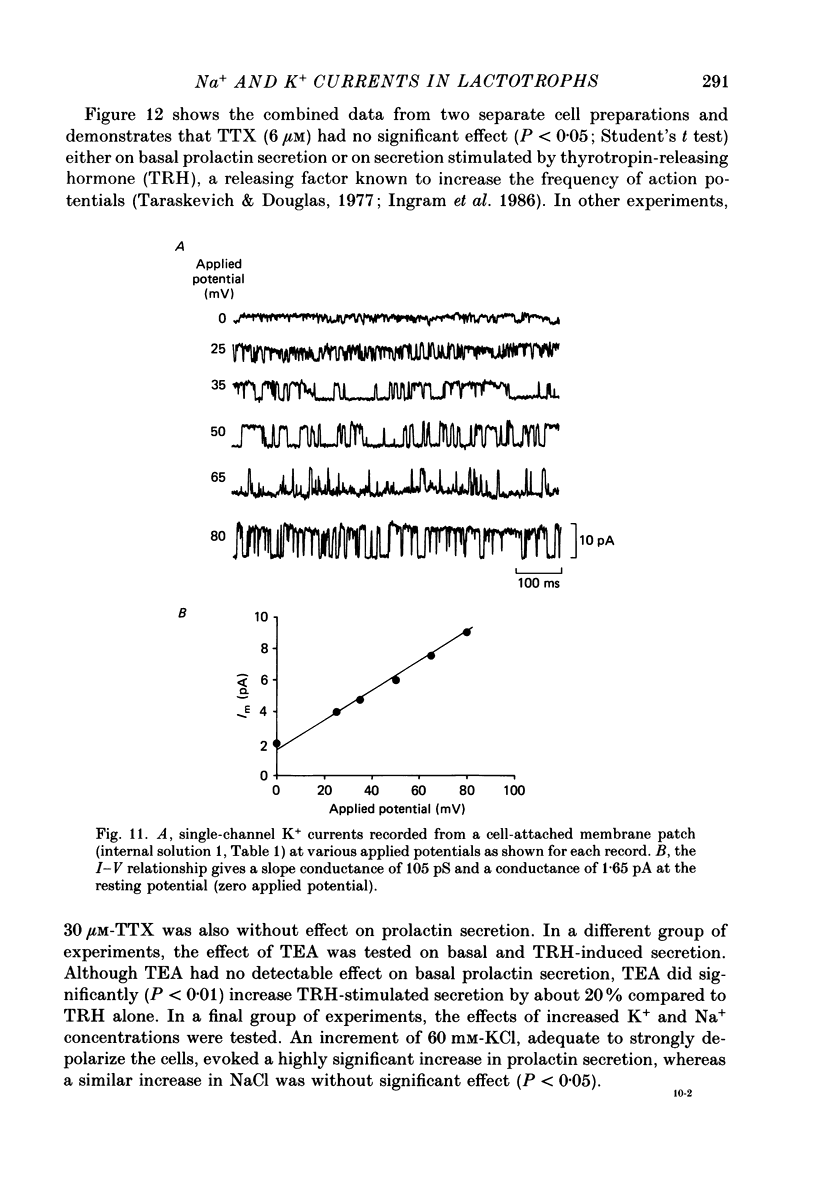
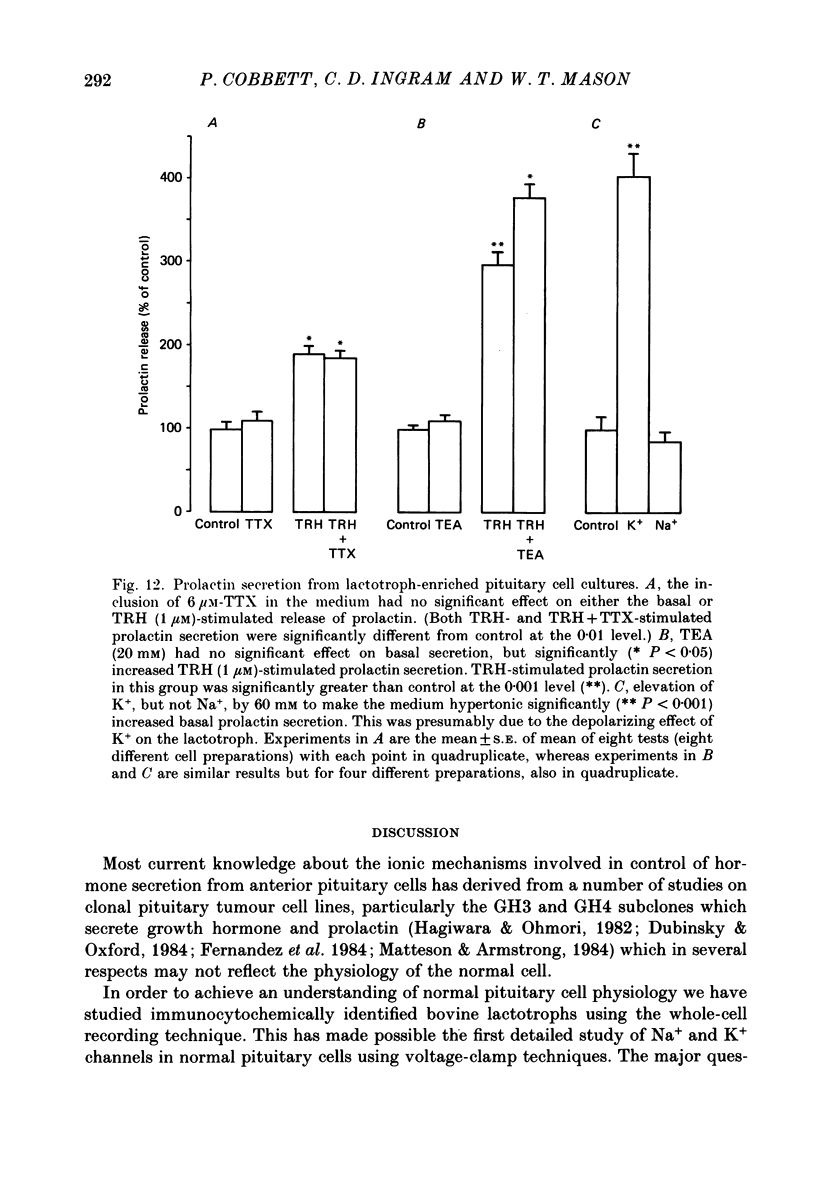
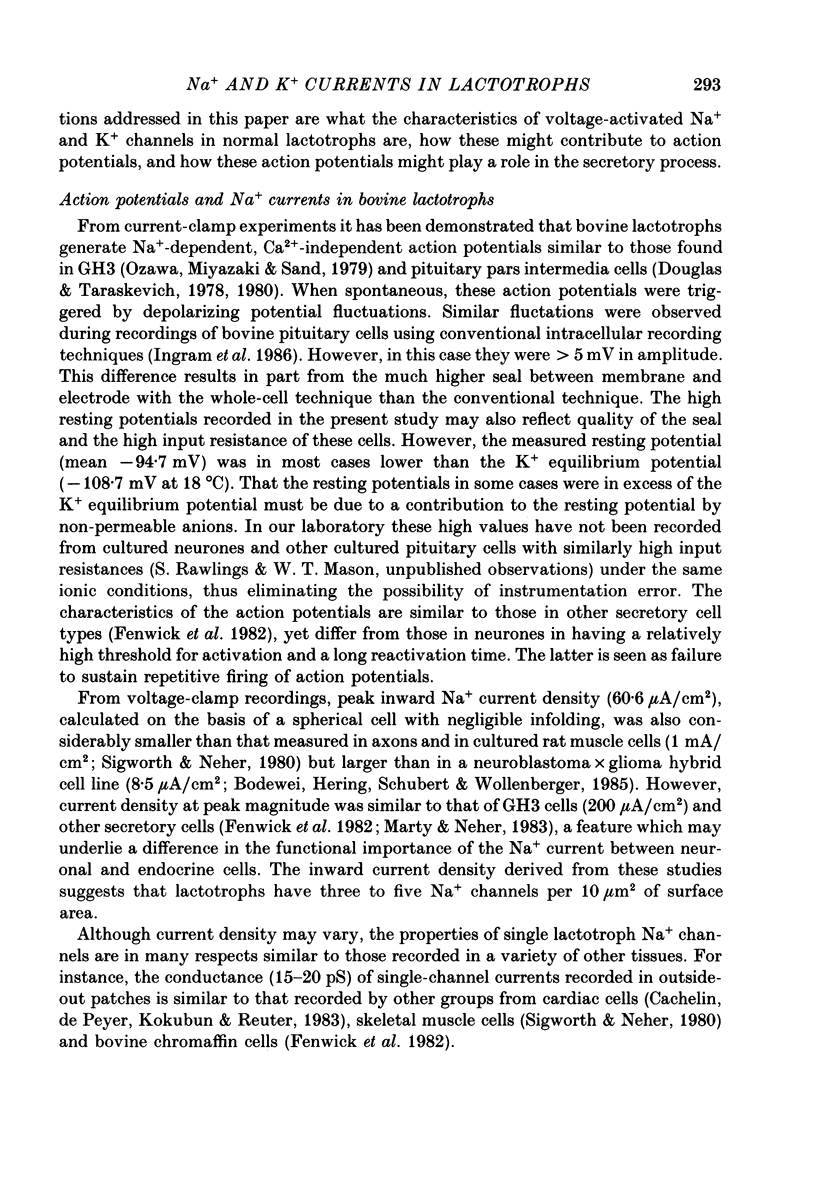
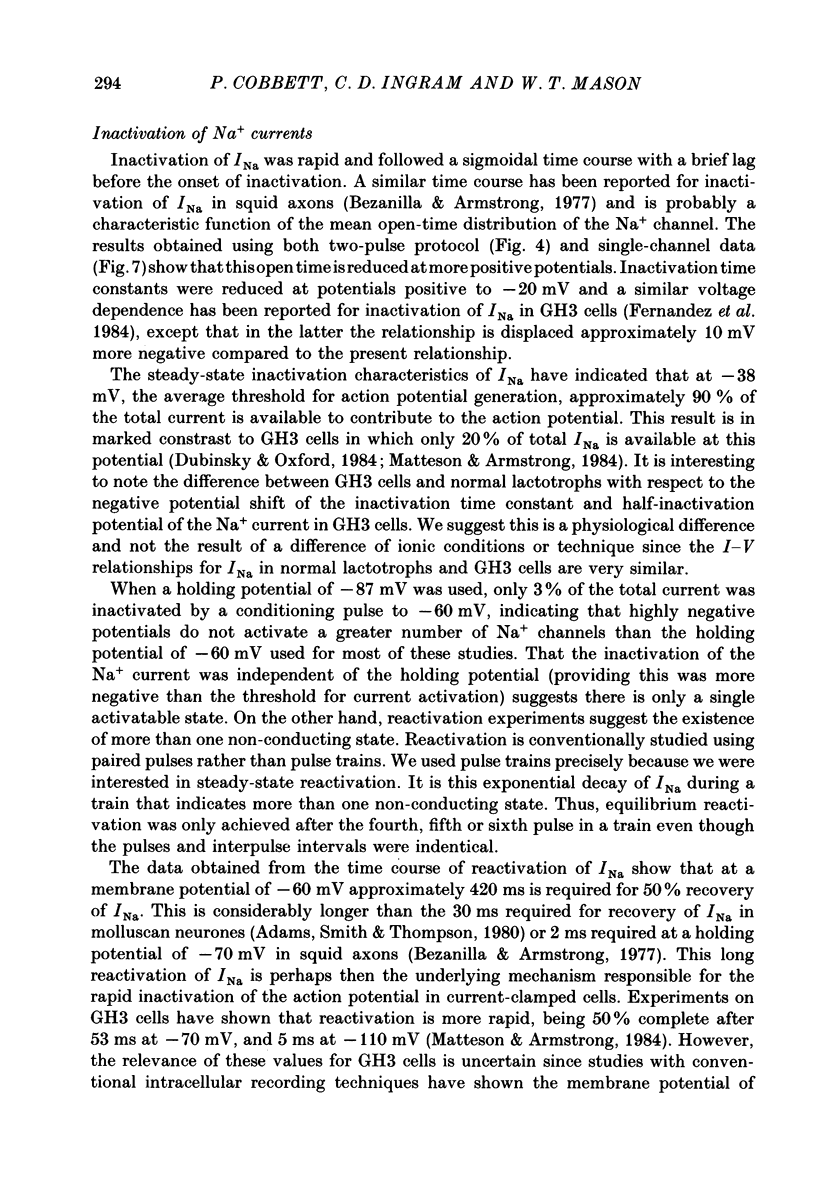
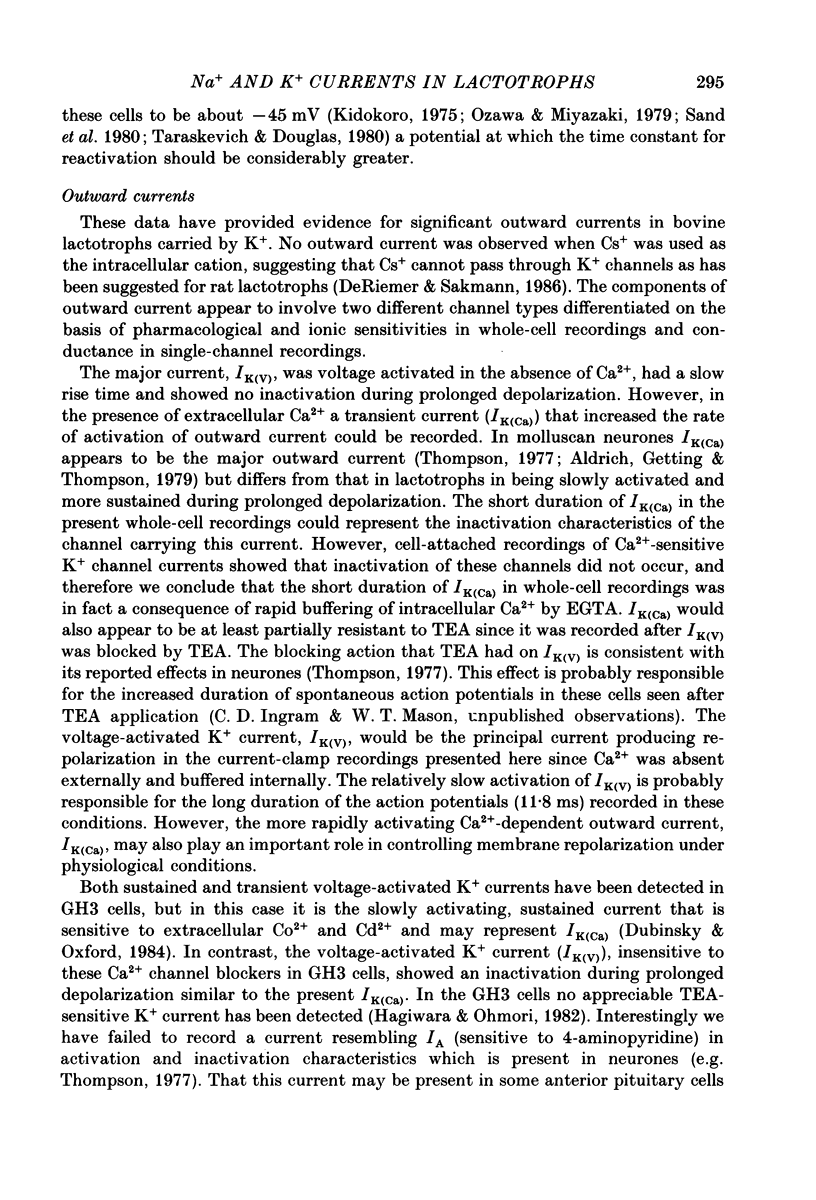
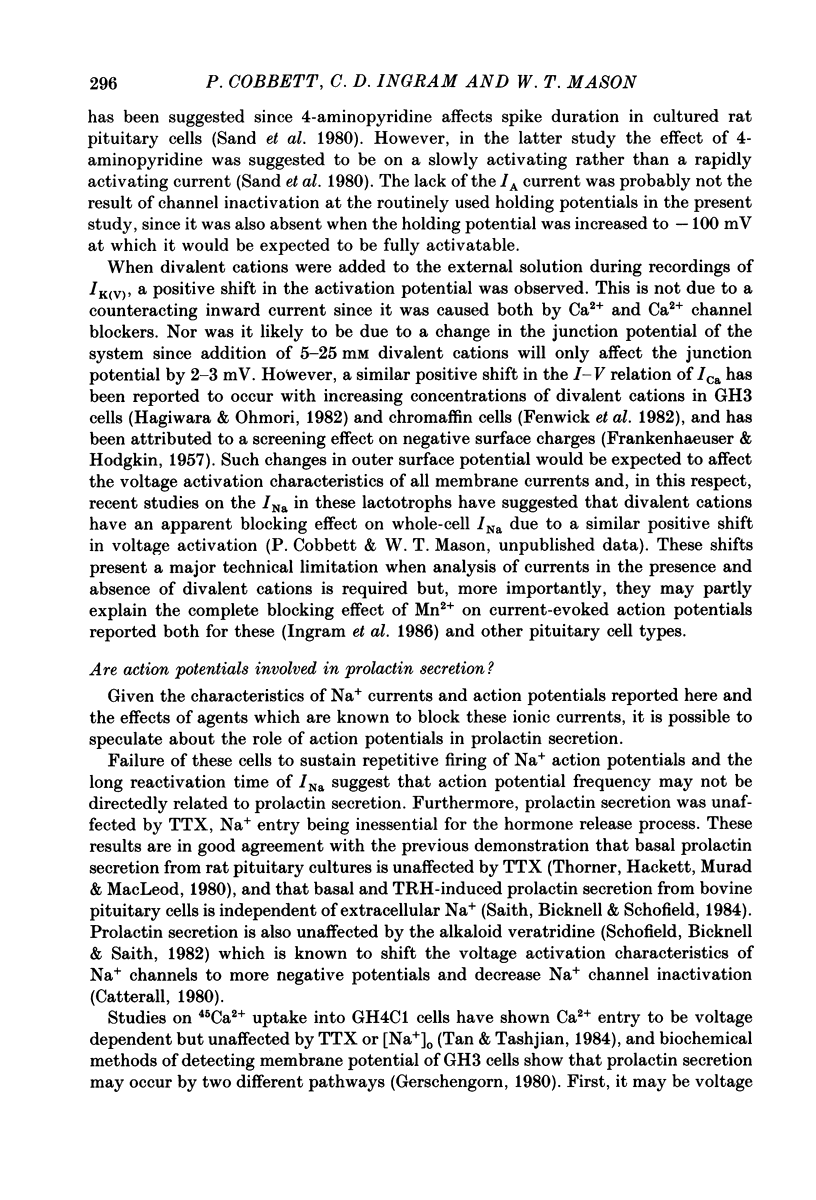
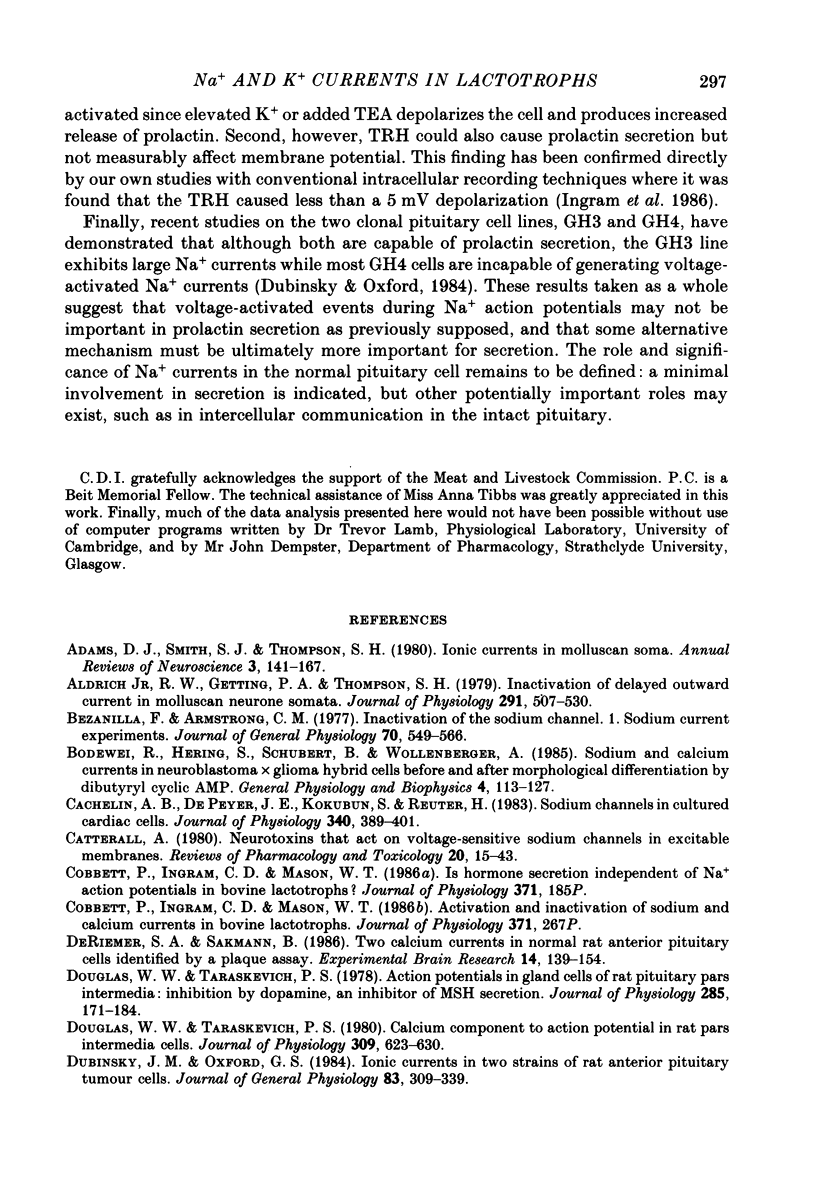
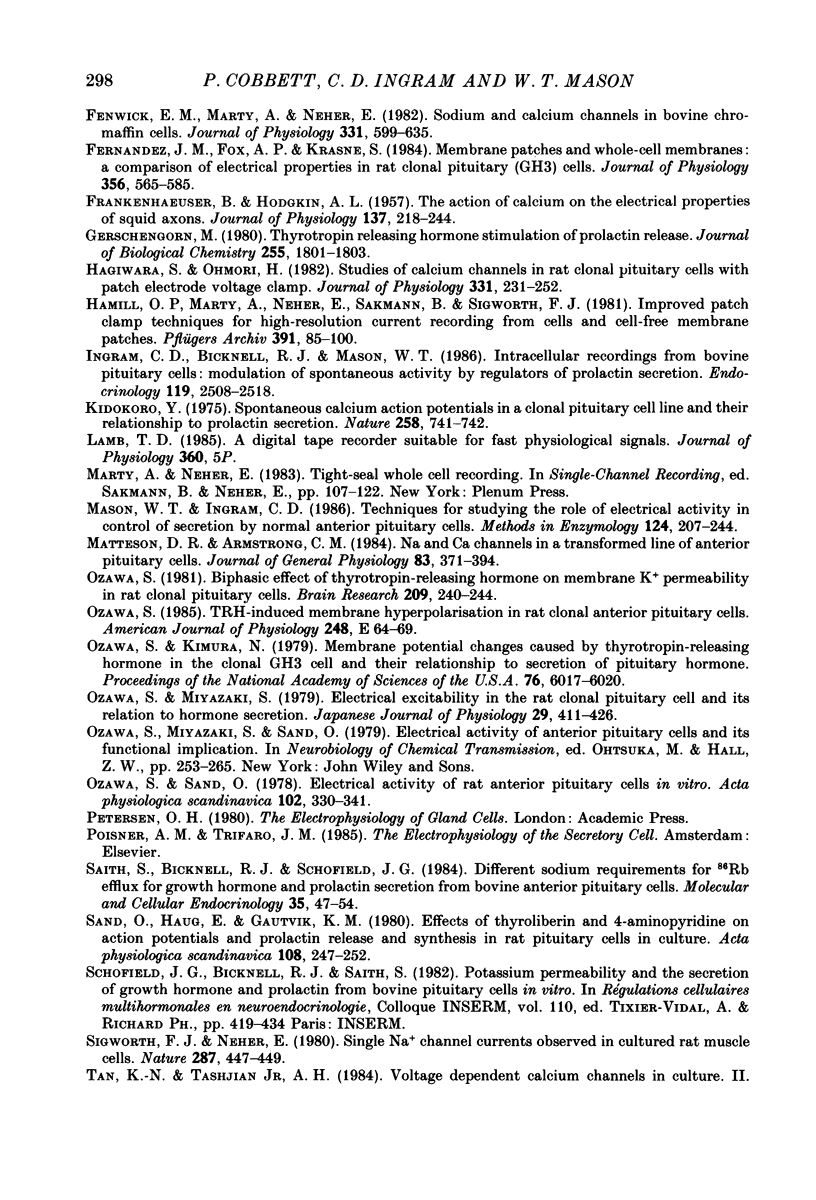
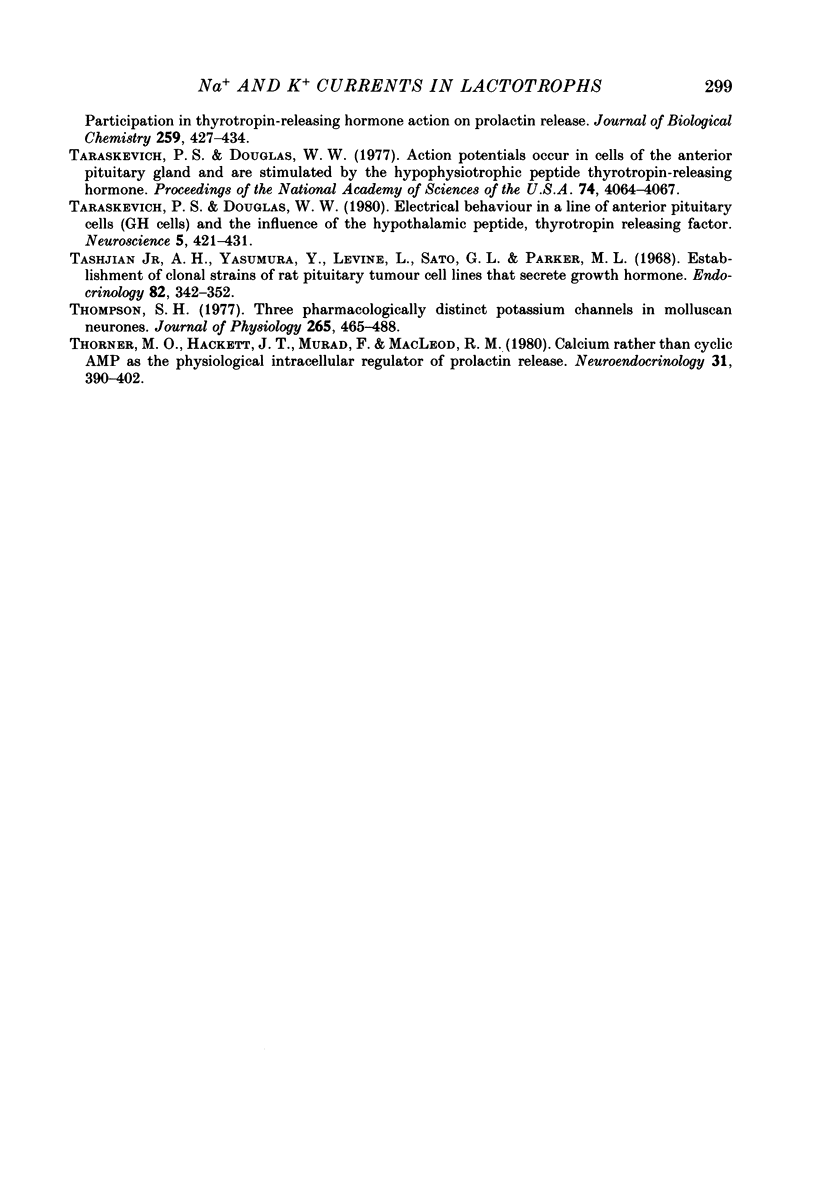
Images in this article
Selected References
These references are in PubMed. This may not be the complete list of references from this article.
- Adams D. J., Smith S. J., Thompson S. H. Ionic currents in molluscan soma. Annu Rev Neurosci. 1980;3:141–167. doi: 10.1146/annurev.ne.03.030180.001041. [DOI] [PubMed] [Google Scholar]
- Aldrich R. W., Jr, Getting P. A., Thompson S. H. Inactivation of delayed outward current in molluscan neurone somata. J Physiol. 1979 Jun;291:507–530. doi: 10.1113/jphysiol.1979.sp012828. [DOI] [PMC free article] [PubMed] [Google Scholar]
- Arthur S. K., Green R. Fluid reabsorption by the proximal convoluted tubule of the kidney in lactating rats. J Physiol. 1986 Feb;371:267–275. doi: 10.1113/jphysiol.1986.sp015973. [DOI] [PMC free article] [PubMed] [Google Scholar]
- Bezanilla F., Armstrong C. M. Inactivation of the sodium channel. I. Sodium current experiments. J Gen Physiol. 1977 Nov;70(5):549–566. doi: 10.1085/jgp.70.5.549. [DOI] [PMC free article] [PubMed] [Google Scholar]
- Bodewei R., Hering S., Schubert B., Wollenberger A. Sodium and calcium currents in neuroblastoma x glioma hybrid cells before and after morphological differentiation by dibutyryl cyclic AMP. Gen Physiol Biophys. 1985 Apr;4(2):113–127. [PubMed] [Google Scholar]
- Cachelin A. B., De Peyer J. E., Kokubun S., Reuter H. Sodium channels in cultured cardiac cells. J Physiol. 1983 Jul;340:389–401. doi: 10.1113/jphysiol.1983.sp014768. [DOI] [PMC free article] [PubMed] [Google Scholar]
- Catterall W. A. Neurotoxins that act on voltage-sensitive sodium channels in excitable membranes. Annu Rev Pharmacol Toxicol. 1980;20:15–43. doi: 10.1146/annurev.pa.20.040180.000311. [DOI] [PubMed] [Google Scholar]
- Douglas W. W., Taraskevich P. S. Action potentials in gland cells of rat pituitary pars intermedia: inhibition by dopamine, an inhibitor of MSH secretion. J Physiol. 1978 Dec;285:171–184. doi: 10.1113/jphysiol.1978.sp012565. [DOI] [PMC free article] [PubMed] [Google Scholar]
- Douglas W. W., Taraskevich P. S. Calcium component to action potentials in rat pars intermedia cells. J Physiol. 1980 Dec;309:623–630. doi: 10.1113/jphysiol.1980.sp013530. [DOI] [PMC free article] [PubMed] [Google Scholar]
- Dubinsky J. M., Oxford G. S. Ionic currents in two strains of rat anterior pituitary tumor cells. J Gen Physiol. 1984 Mar;83(3):309–339. doi: 10.1085/jgp.83.3.309. [DOI] [PMC free article] [PubMed] [Google Scholar]
- FRANKENHAEUSER B., HODGKIN A. L. The action of calcium on the electrical properties of squid axons. J Physiol. 1957 Jul 11;137(2):218–244. doi: 10.1113/jphysiol.1957.sp005808. [DOI] [PMC free article] [PubMed] [Google Scholar]
- Fenwick E. M., Marty A., Neher E. Sodium and calcium channels in bovine chromaffin cells. J Physiol. 1982 Oct;331:599–635. doi: 10.1113/jphysiol.1982.sp014394. [DOI] [PMC free article] [PubMed] [Google Scholar]
- Fernandez J. M., Fox A. P., Krasne S. Membrane patches and whole-cell membranes: a comparison of electrical properties in rat clonal pituitary (GH3) cells. J Physiol. 1984 Nov;356:565–585. doi: 10.1113/jphysiol.1984.sp015483. [DOI] [PMC free article] [PubMed] [Google Scholar]
- Gershengorn M. C. Thyrotropin releasing hormone stimulation of prolactin release. Evidence for a membrane potential-independent, Ca2+-dependent mechanism of action. J Biol Chem. 1980 Mar 10;255(5):1801–1803. [PubMed] [Google Scholar]
- Hagiwara S., Ohmori H. Studies of calcium channels in rat clonal pituitary cells with patch electrode voltage clamp. J Physiol. 1982 Oct;331:231–252. doi: 10.1113/jphysiol.1982.sp014371. [DOI] [PMC free article] [PubMed] [Google Scholar]
- Hamill O. P., Marty A., Neher E., Sakmann B., Sigworth F. J. Improved patch-clamp techniques for high-resolution current recording from cells and cell-free membrane patches. Pflugers Arch. 1981 Aug;391(2):85–100. doi: 10.1007/BF00656997. [DOI] [PubMed] [Google Scholar]
- Ingram C. D., Bicknell R. J., Mason W. T. Intracellular recordings from bovine anterior pituitary cells: modulation of spontaneous activity by regulators of prolactin secretion. Endocrinology. 1986 Dec;119(6):2508–2518. doi: 10.1210/endo-119-6-2508. [DOI] [PubMed] [Google Scholar]
- Kidokoro Y. Spontaneous calcium action potentials in a clonal pituitary cell line and their relationship to prolactin secretion. Nature. 1975 Dec 25;258(5537):741–742. doi: 10.1038/258741a0. [DOI] [PubMed] [Google Scholar]
- Mason W. T., Ingram C. D. Techniques for studying the role of electrical activity in control of secretion by normal anterior pituitary cells. Methods Enzymol. 1986;124:207–242. doi: 10.1016/0076-6879(86)24017-3. [DOI] [PubMed] [Google Scholar]
- Matteson D. R., Armstrong C. M. Na and Ca channels in a transformed line of anterior pituitary cells. J Gen Physiol. 1984 Mar;83(3):371–394. doi: 10.1085/jgp.83.3.371. [DOI] [PMC free article] [PubMed] [Google Scholar]
- Ozawa S. Biphasic effect of thyrotropin-releasing hormone on membrane K+ permeability in rat clonal pituitary cells. Brain Res. 1981 Mar 23;209(1):240–244. doi: 10.1016/0006-8993(81)91188-4. [DOI] [PubMed] [Google Scholar]
- Ozawa S., Kimura N. Membrane potential changes caused by thyrotropin-releasing hormone in the clonal GH3 cell and their relationship to secretion of pituitary hormone. Proc Natl Acad Sci U S A. 1979 Nov;76(11):6017–6020. doi: 10.1073/pnas.76.11.6017. [DOI] [PMC free article] [PubMed] [Google Scholar]
- Ozawa S., Miyazaki S. I. Electrical excitability in the rat clonal pituitary cell and its relation to hormone secretion. Jpn J Physiol. 1979;29(4):411–426. doi: 10.2170/jjphysiol.29.411. [DOI] [PubMed] [Google Scholar]
- Ozawa S., Sand O. Electric activity of rat anterior pituitary cells in vitro. Acta Physiol Scand. 1978 Mar;102(3):330–341. doi: 10.1111/j.1748-1716.1978.tb06080.x. [DOI] [PubMed] [Google Scholar]
- Saith S., Bicknell R. J., Schofield J. G. Different sodium requirements for 86Rb efflux and for growth hormone and prolactin secretion from bovine anterior pituitary cells. Mol Cell Endocrinol. 1984 Apr;35(1):47–54. doi: 10.1016/0303-7207(84)90029-7. [DOI] [PubMed] [Google Scholar]
- Sand O., Haug E., Gautvik K. M. Effects of thyroliberin and 4-aminopyridine on action potentials and prolactin release and synthesis in rat pituitary cells in culture. Acta Physiol Scand. 1980 Mar;108(3):247–252. doi: 10.1111/j.1748-1716.1980.tb06530.x. [DOI] [PubMed] [Google Scholar]
- Sigworth F. J., Neher E. Single Na+ channel currents observed in cultured rat muscle cells. Nature. 1980 Oct 2;287(5781):447–449. doi: 10.1038/287447a0. [DOI] [PubMed] [Google Scholar]
- Taraskevich P. S., Douglas W. W. Action potentials occur in cells of the normal anterior pituitary gland and are stimulated by the hypophysiotropic peptide thyrotropin-releasing hormone. Proc Natl Acad Sci U S A. 1977 Sep;74(9):4064–4067. doi: 10.1073/pnas.74.9.4064. [DOI] [PMC free article] [PubMed] [Google Scholar]
- Taraskevich P. S., Douglas W. W. Electrical behaviour in a line of anterior pituitary cells (GH cells) and the influence of the hypothalamic peptide, thyrotrophin releasing factor. Neuroscience. 1980;5(2):421–431. doi: 10.1016/0306-4522(80)90117-7. [DOI] [PubMed] [Google Scholar]
- Tashjian A. H., Jr, Yasumura Y., Levine L., Sato G. H., Parker M. L. Establishment of clonal strains of rat pituitary tumor cells that secrete growth hormone. Endocrinology. 1968 Feb;82(2):342–352. doi: 10.1210/endo-82-2-342. [DOI] [PubMed] [Google Scholar]
- Thompson S. H. Three pharmacologically distinct potassium channels in molluscan neurones. J Physiol. 1977 Feb;265(2):465–488. doi: 10.1113/jphysiol.1977.sp011725. [DOI] [PMC free article] [PubMed] [Google Scholar]
- Thorner M. O., Hackett J. T., Murad F., MacLeod R. M. Calcium rather than cyclic AMP as the physiological intracellular regulator of prolactin release. Neuroendocrinology. 1980 Dec;31(6):390–402. doi: 10.1159/000123109. [DOI] [PubMed] [Google Scholar]