Abstract
1. Calcium current (ICa) was studied in single isolated smooth muscle cells of a guinea-pig taenia caeci dialysed with Cs+-containing solution to suppress K+ outward current. 2. With increasing step depolarizations up to +10 mV, acceleration of ICa inactivation was observed. With further increase of step depolarization, ICa inactivation was slowed down. The largest ICa (observed at +10 mV) was characterized by the maximal speed of inactivation. 3. Comparison of ICa in different external concentrations of Ca2+ ions ([Ca2+]o) revealed that at the same membrane potential the time course of ICa inactivation was slower, the smaller the amplitude of ICa. Slowing down of ICa inactivation was observed also during its partial block by Co2+ ions. 4. Elevation of temperature increased ICa peak amplitude and accelerated its decay. The amplitude of ICa was increased by a factor of 1.7 +/- 0.14 (n = 6) when the temperature was raised by 10 degrees C. 5. Calculations of Ca2+ entry during ICa as a time integral of Co2+-sensitive current, and comparison with the degree of ICa inactivation, showed that inactivation was tightly related to Ca2+ entry in the membrane potential range -20 to +40 mV. 6. Ba2+ current through Ca2+ channels was larger than ICa and its inactivation was considerably slower. 7. Recovery of ICa from inactivation was found to be potential dependent. When the cell membrane was hyperpolarized, ICa recovery was accelerated. 8. It was concluded that inactivation and recovery of ICa in smooth muscle cells were influenced by both Ca2+ entry and membrane potential. It was also pointed out that the observed events are difficult to explain by the hypothesis that inactivation was produced simply by accumulation of Ca2+ ions near the inner side of the membrane, and that recovery was due to lowering of internal free Ca2+ ion concentration ([Ca2+]i).
Full text
PDF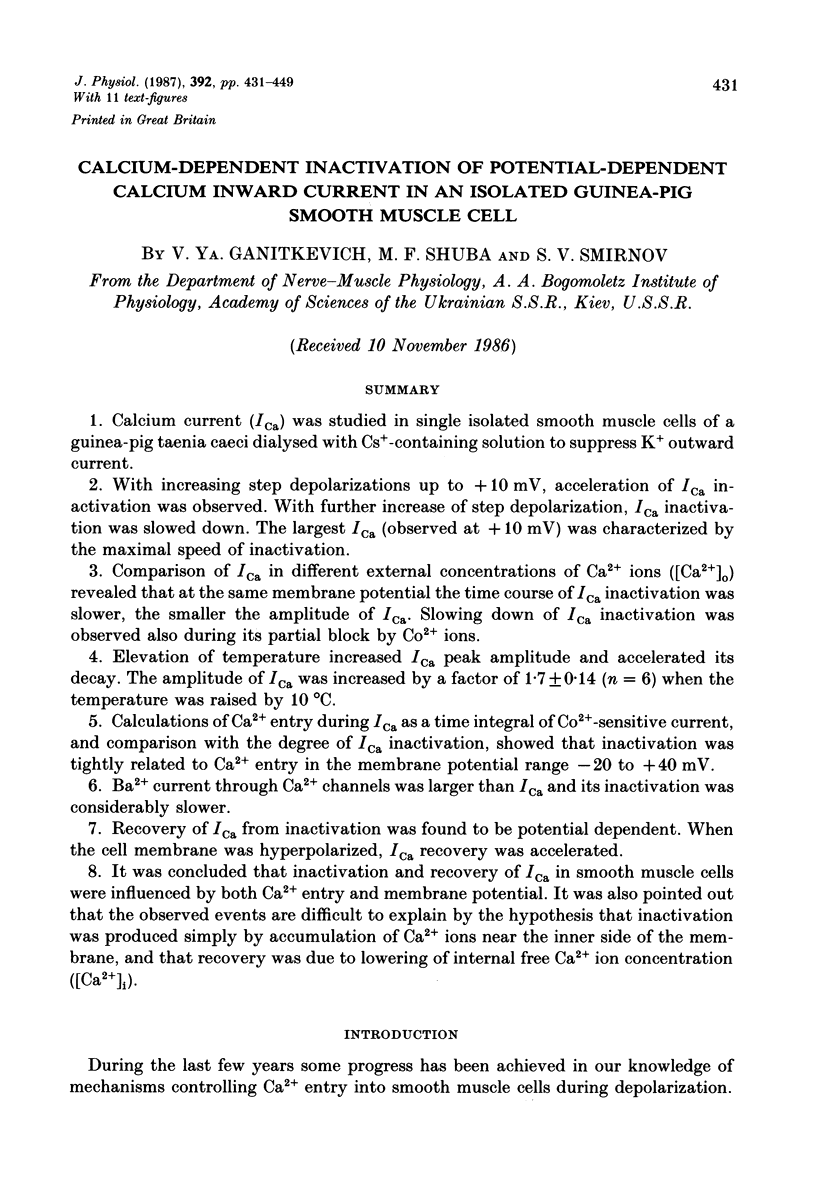
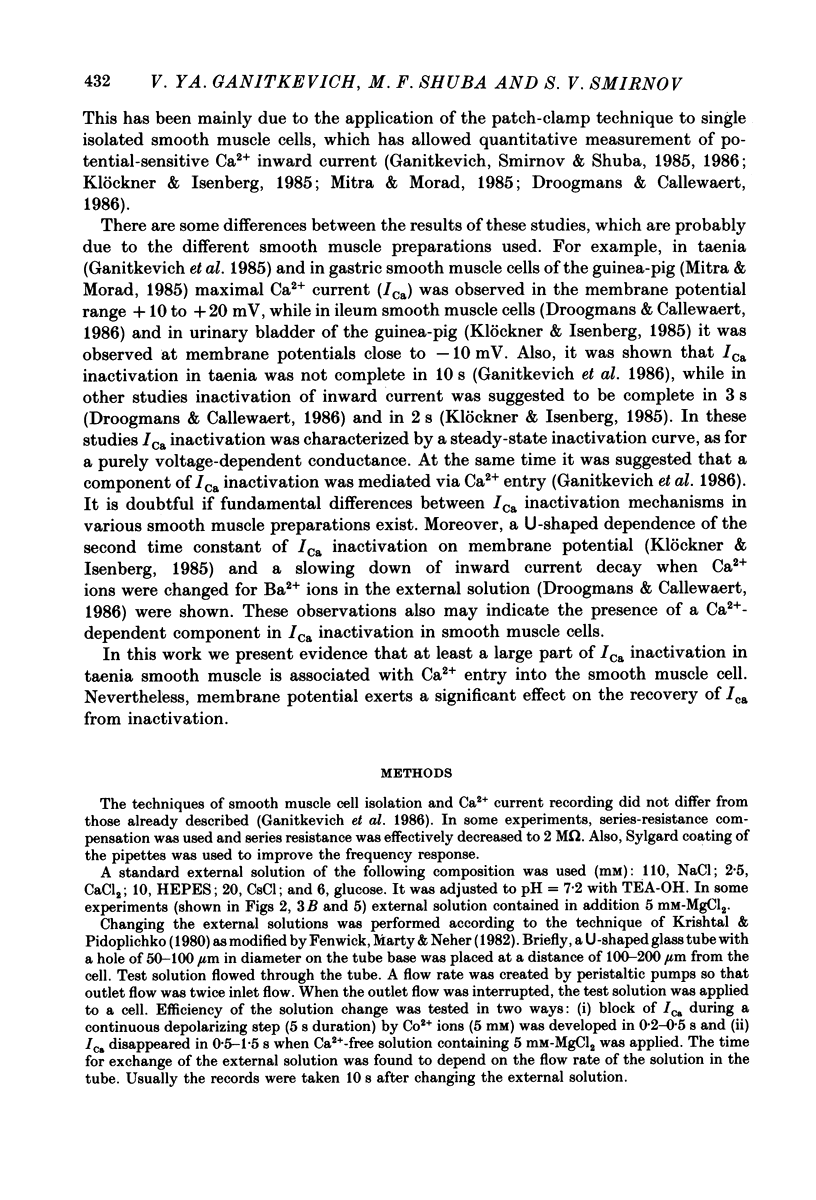
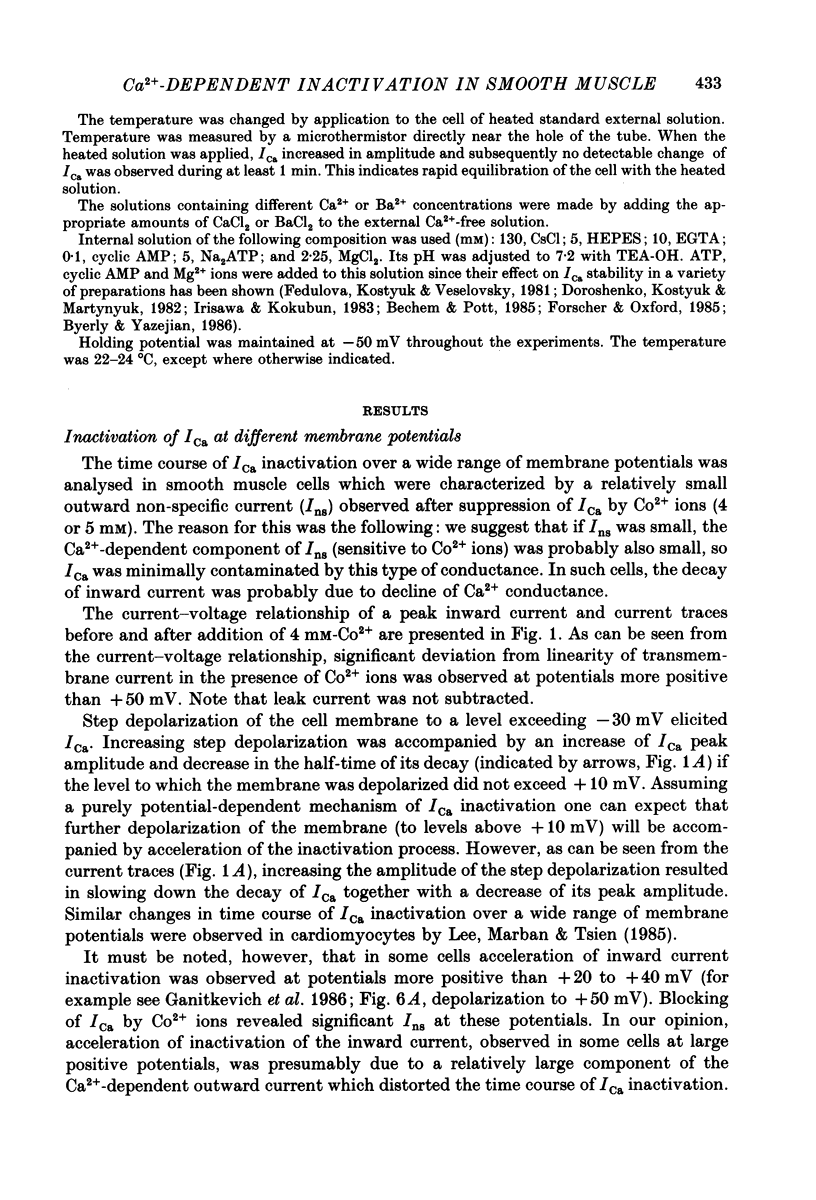
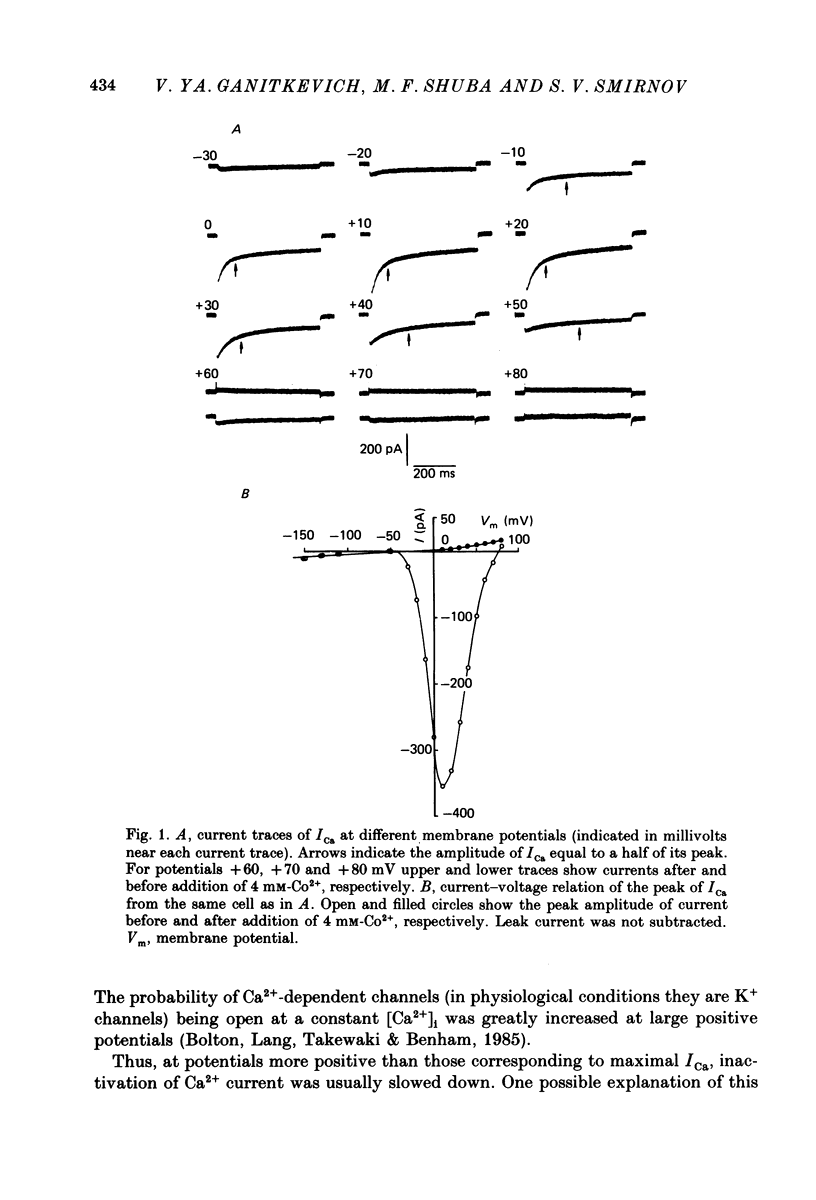
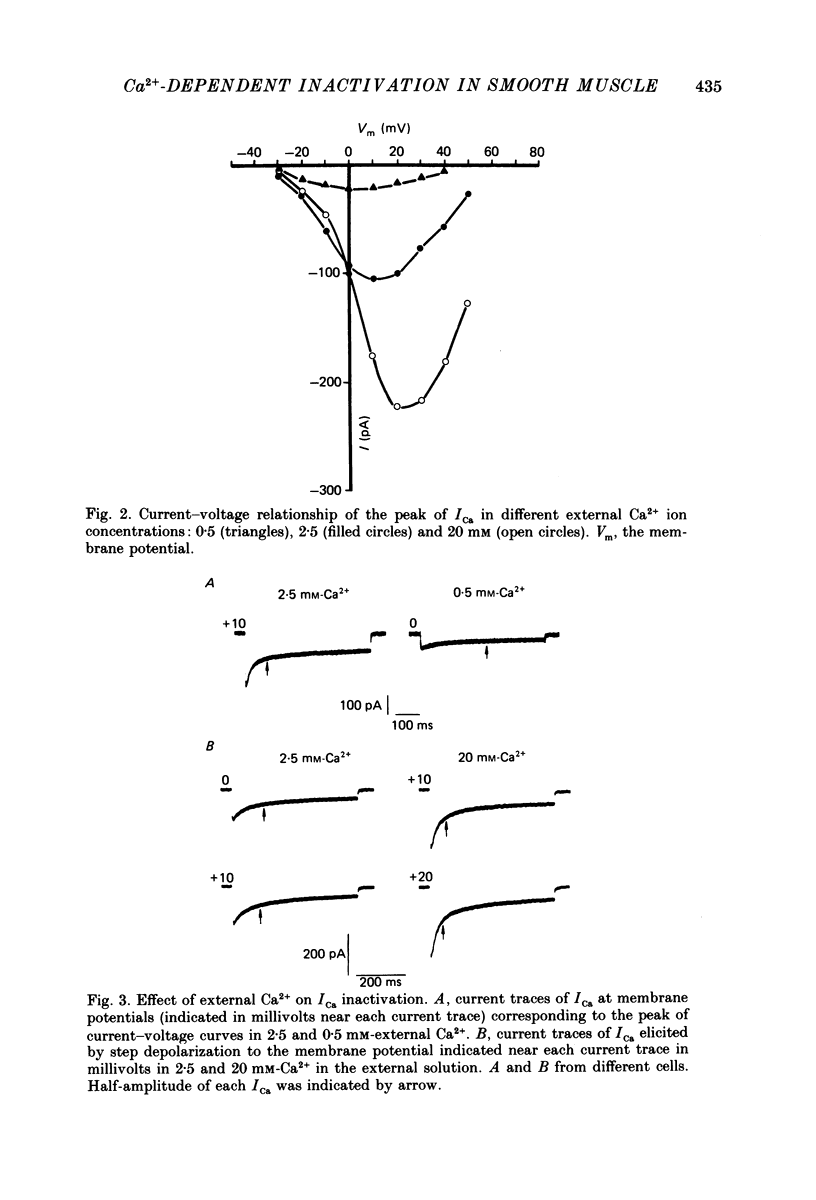
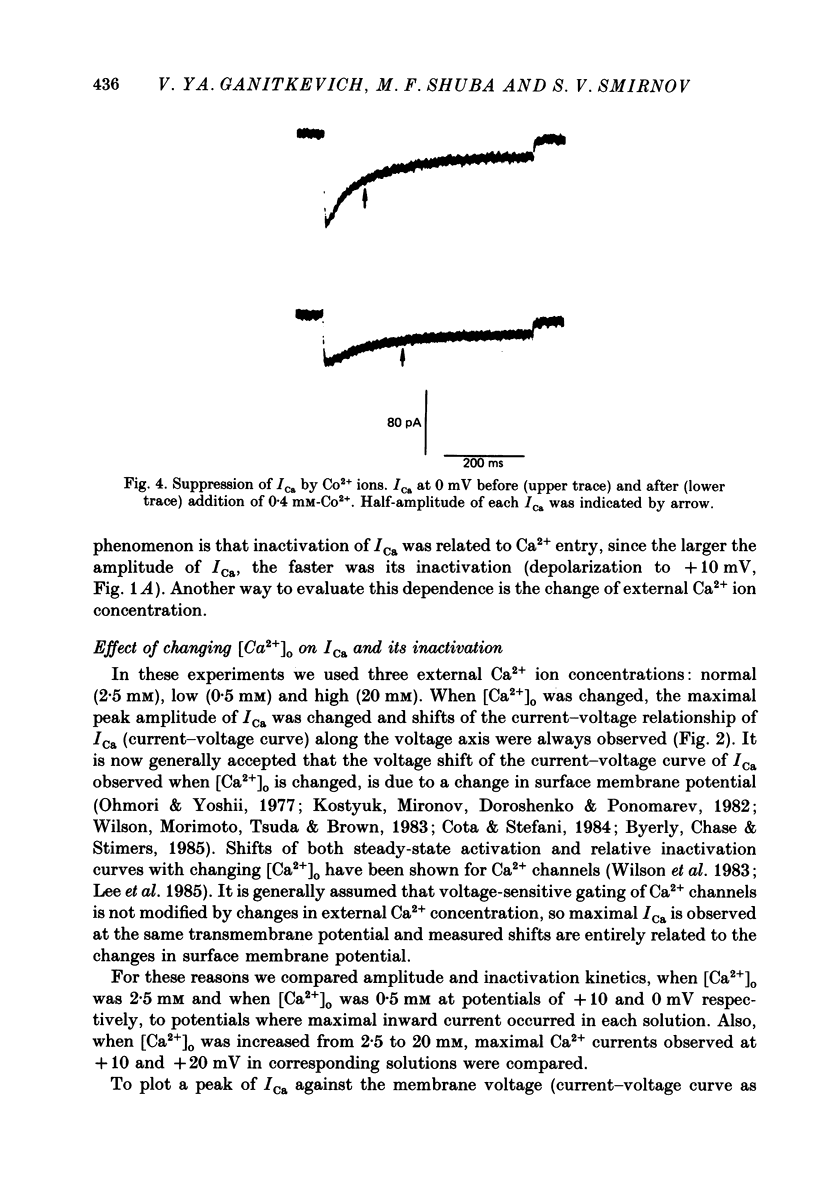
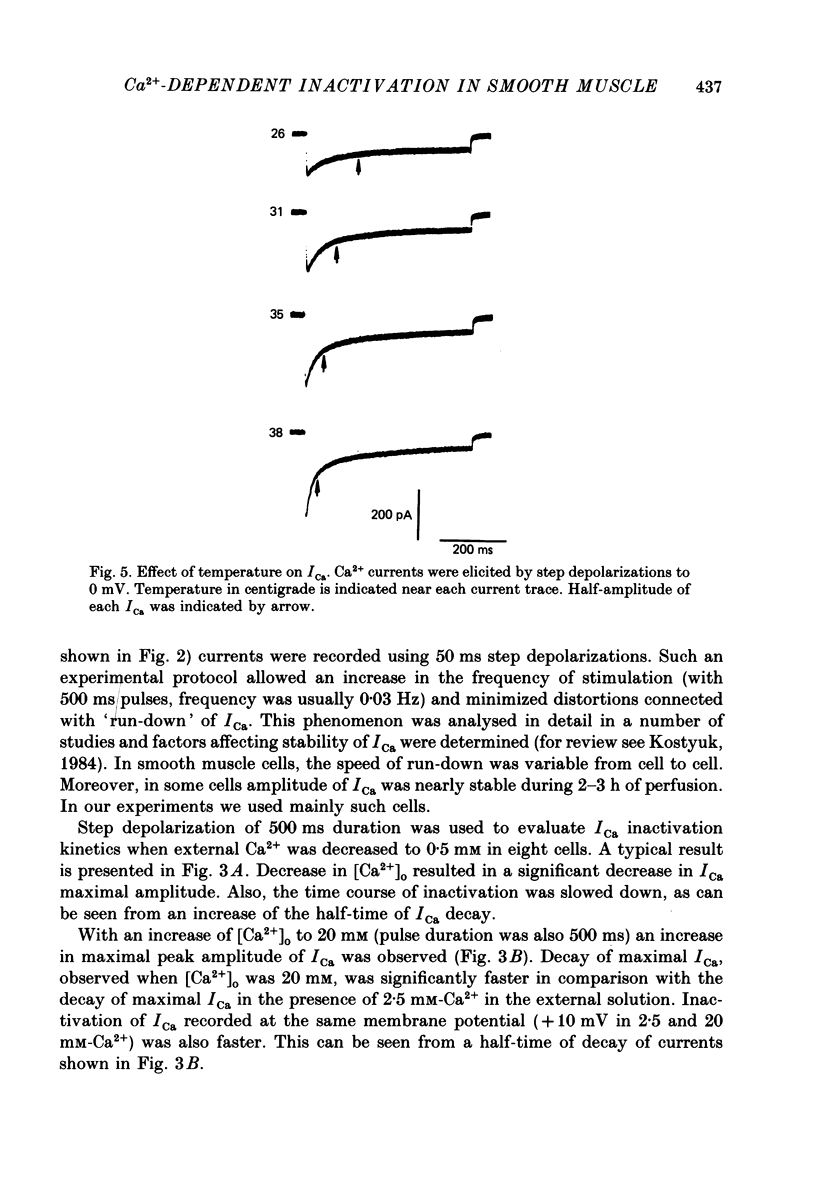
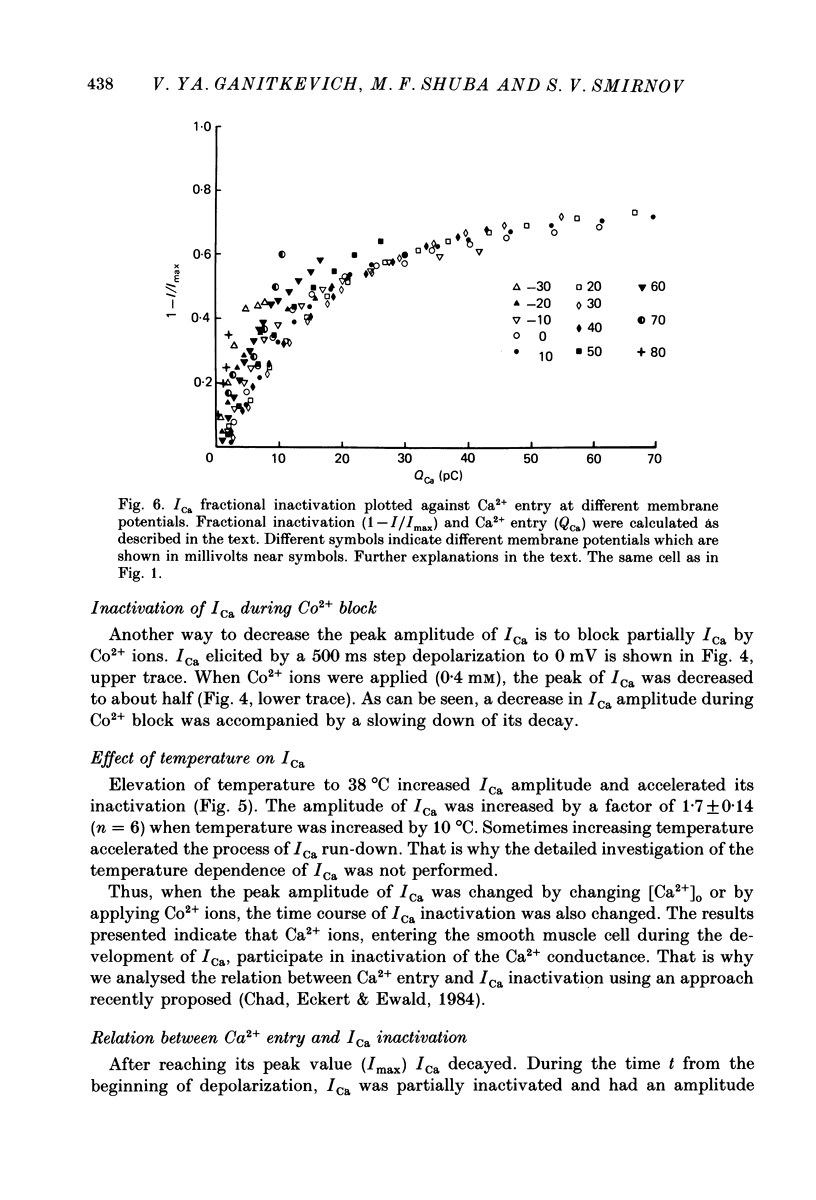
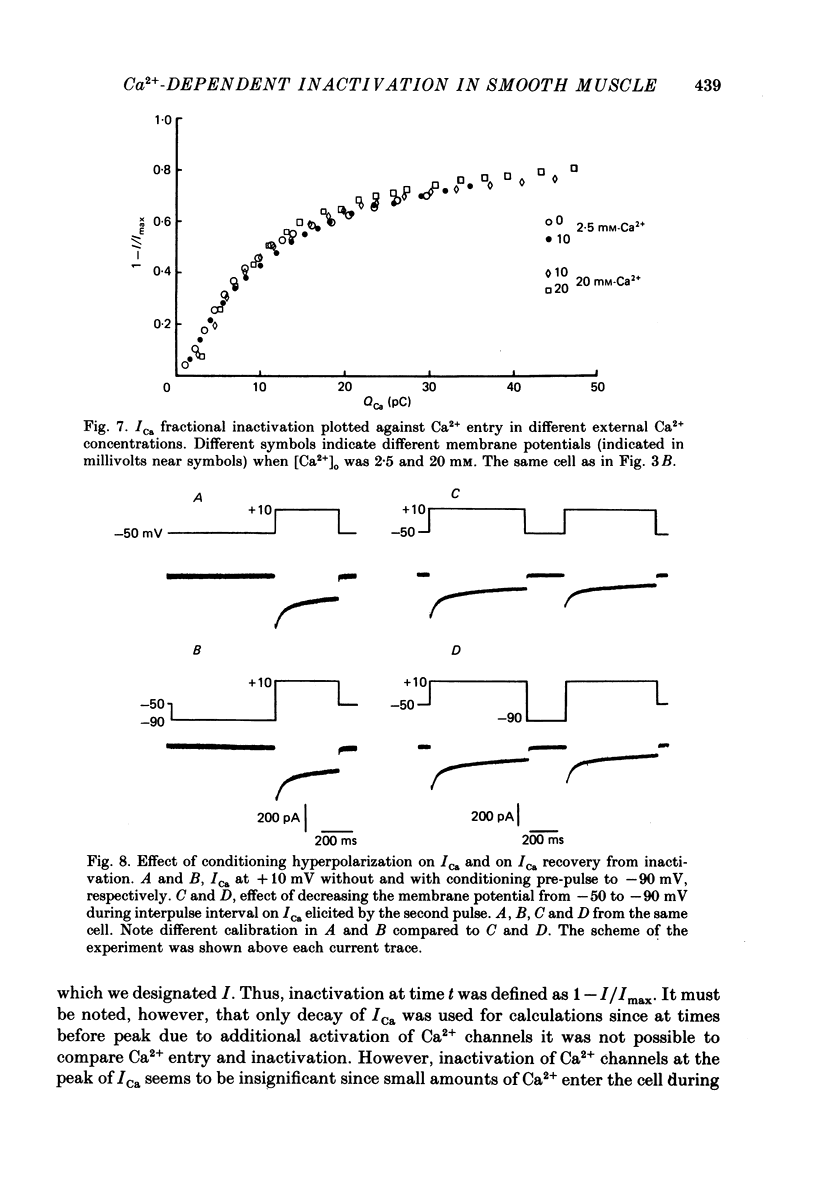
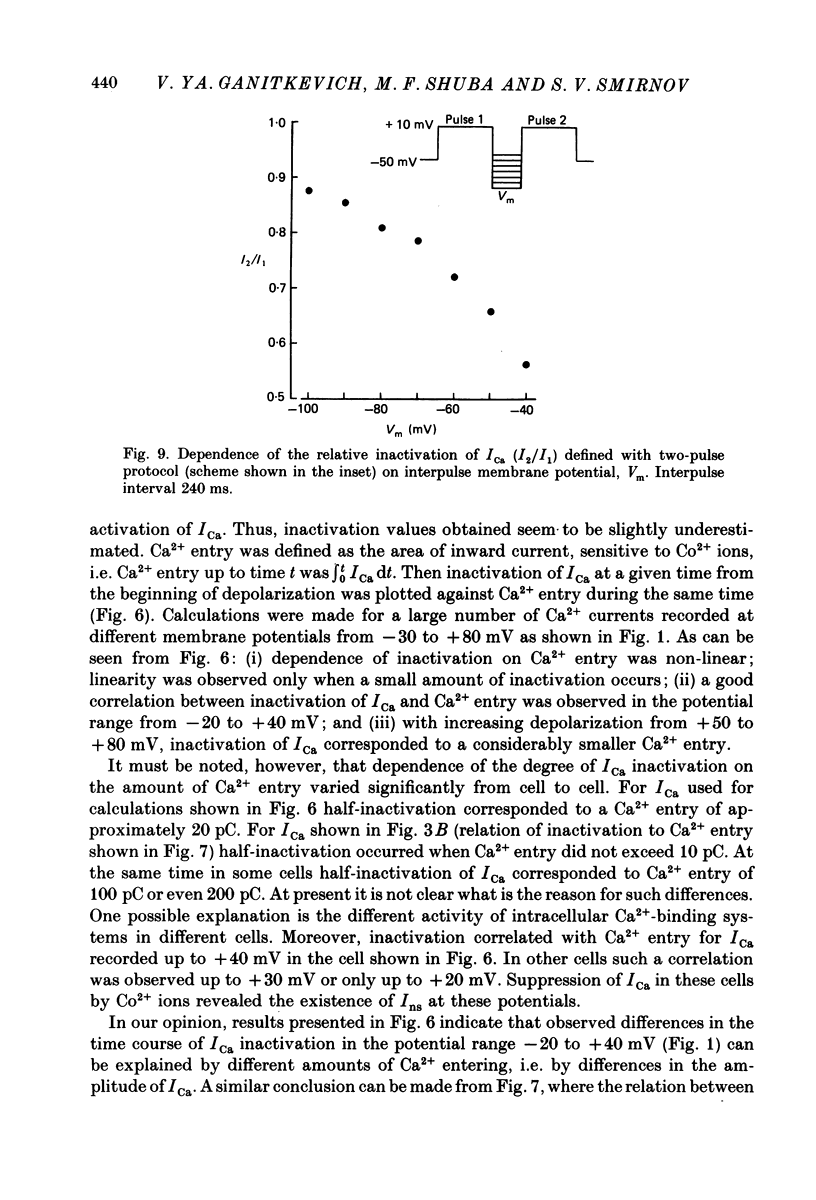
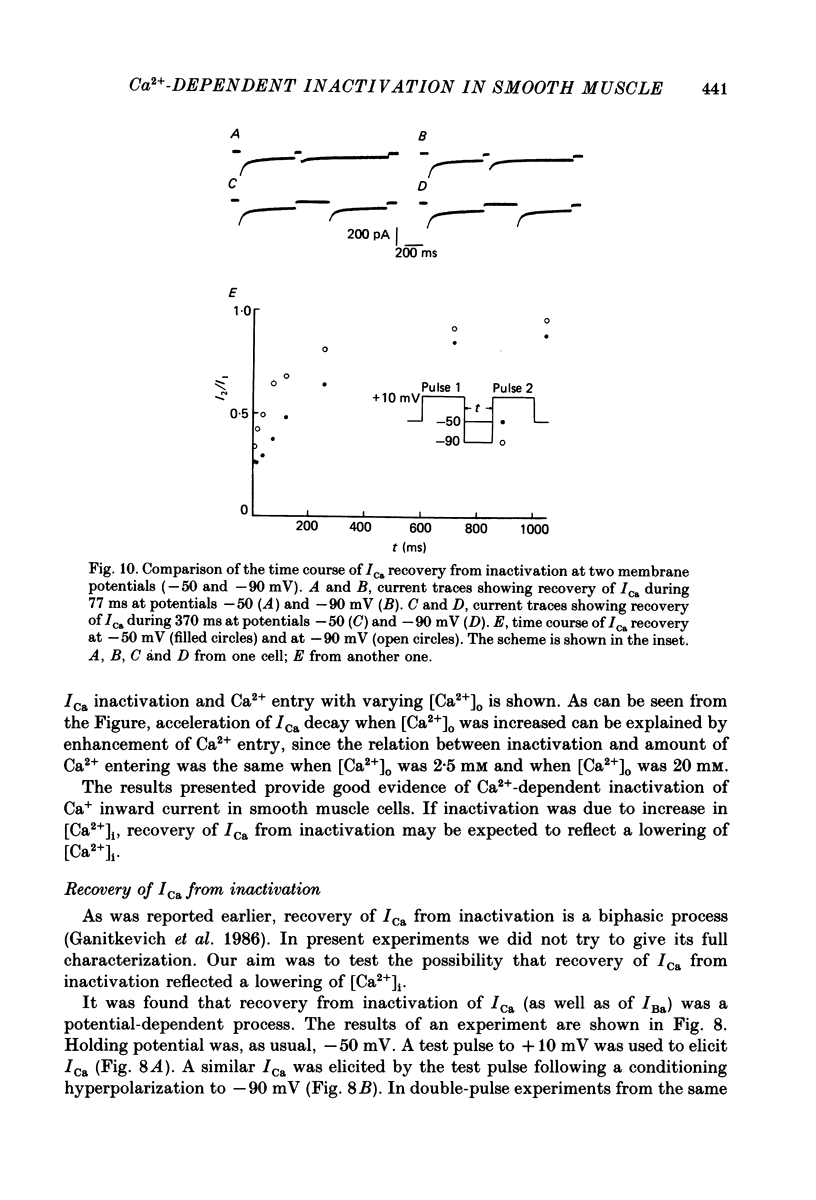
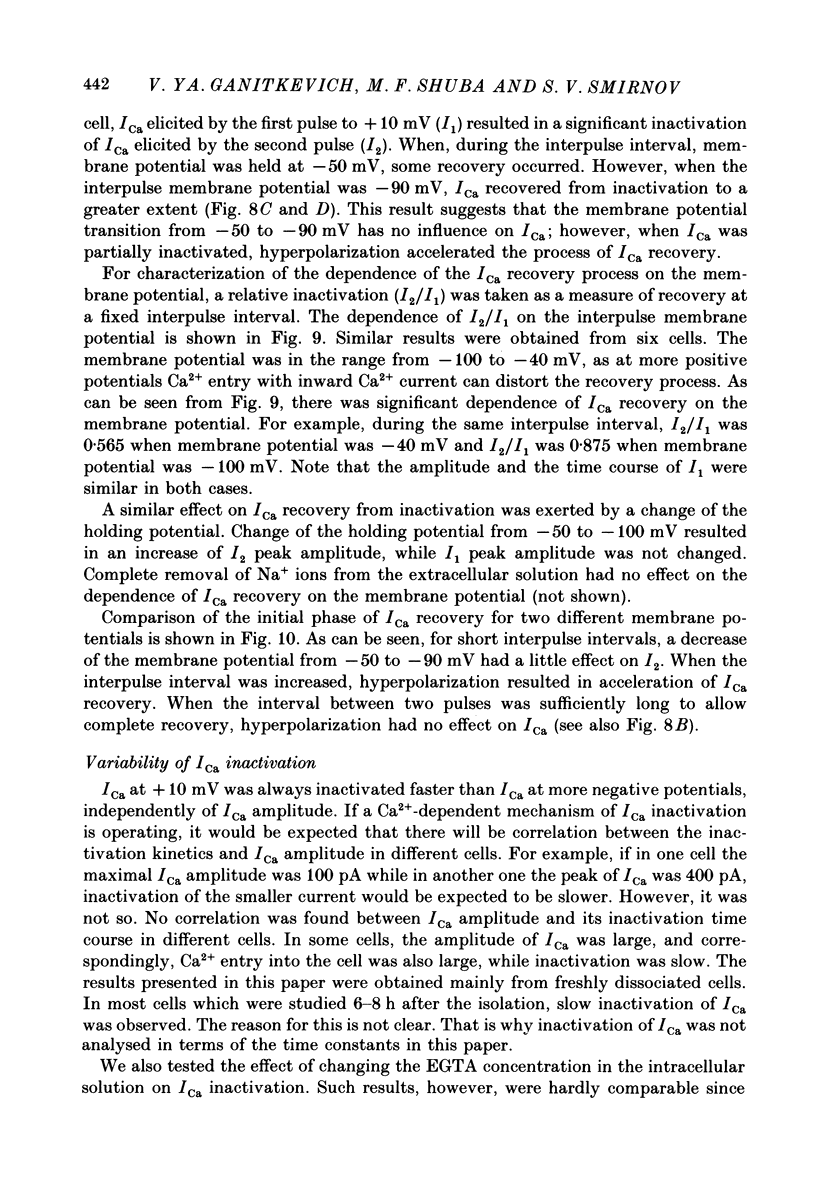
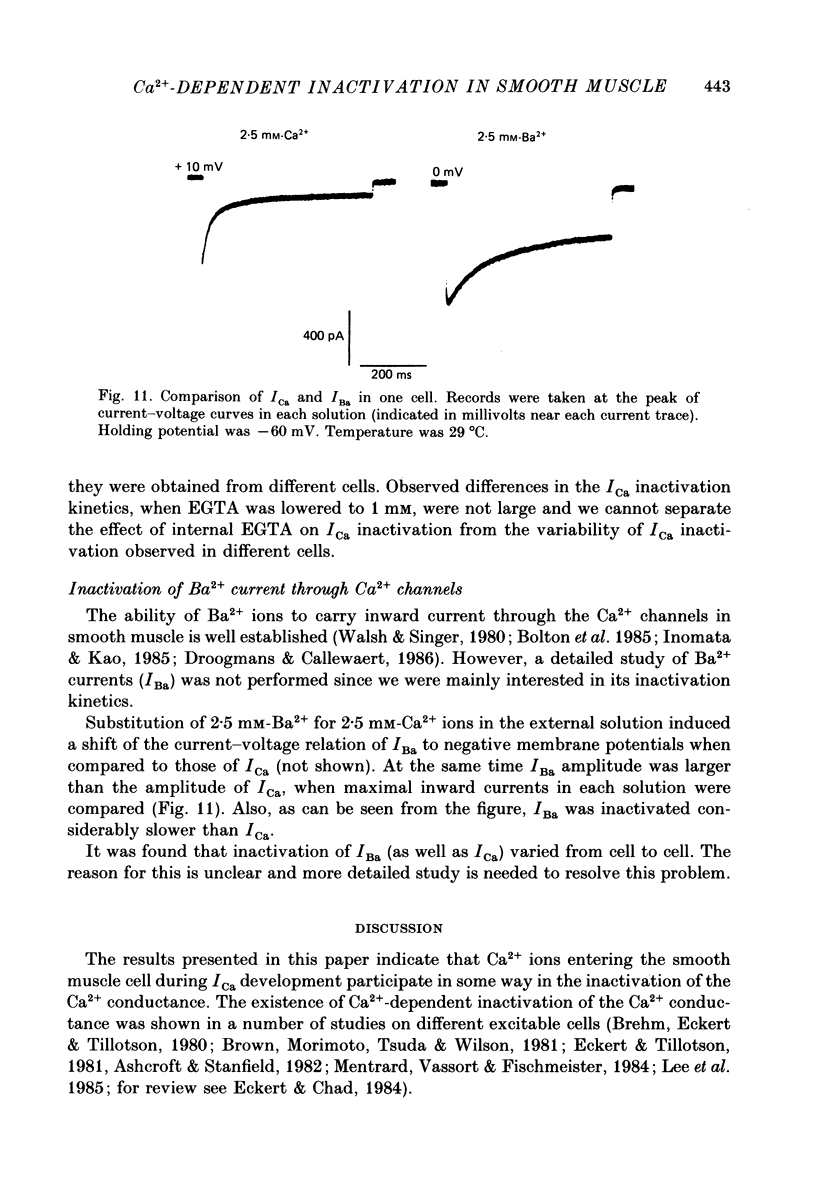
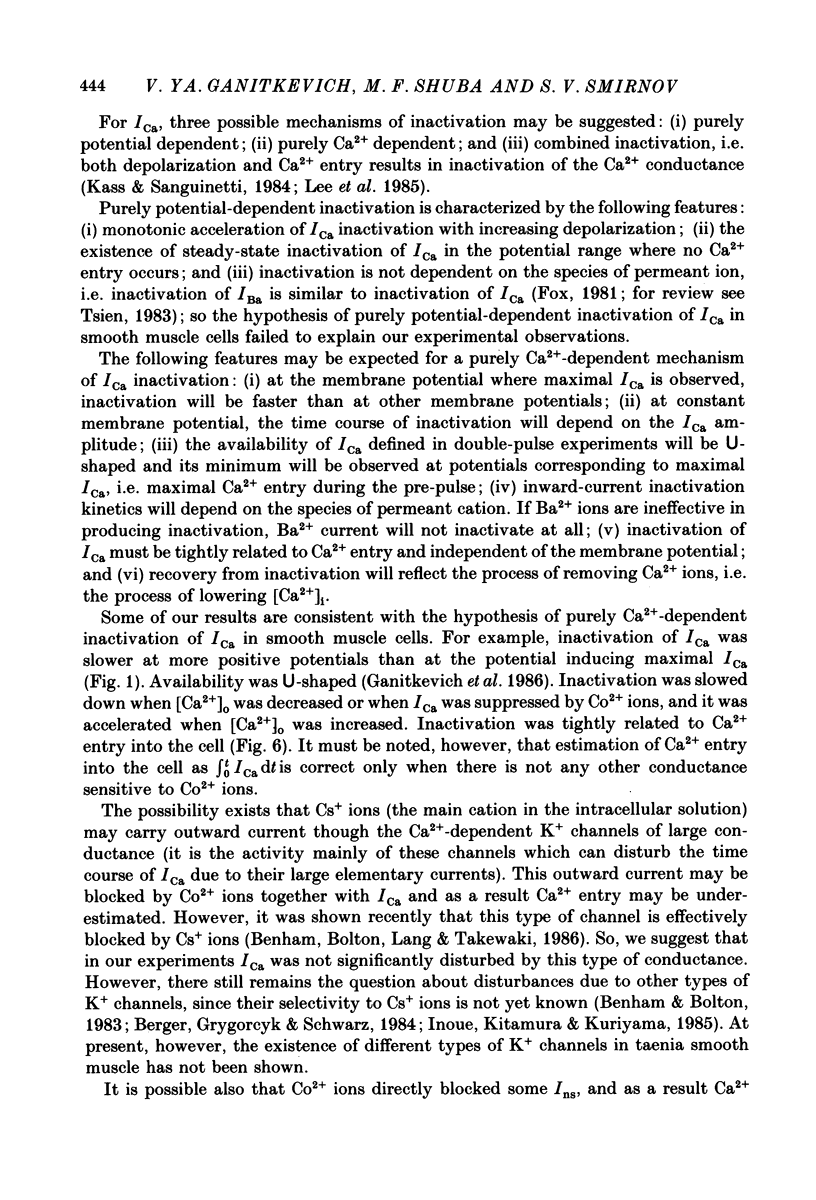
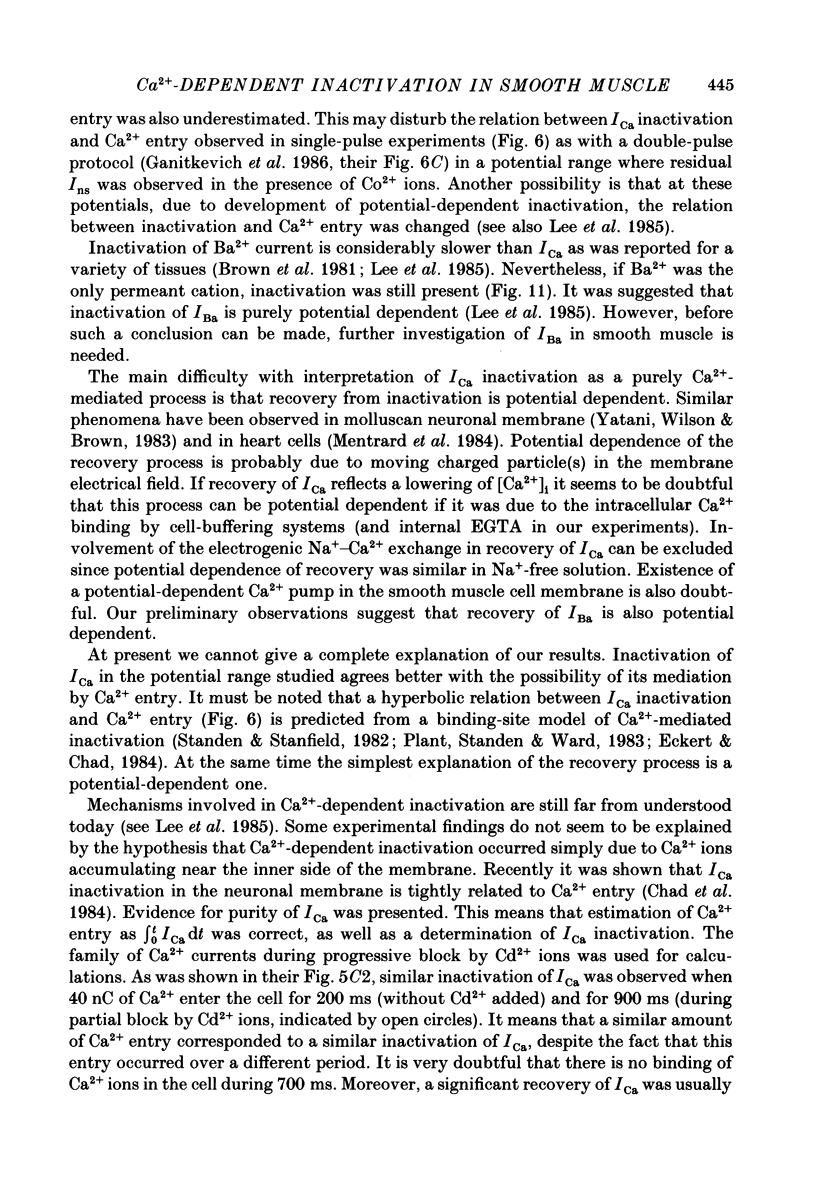
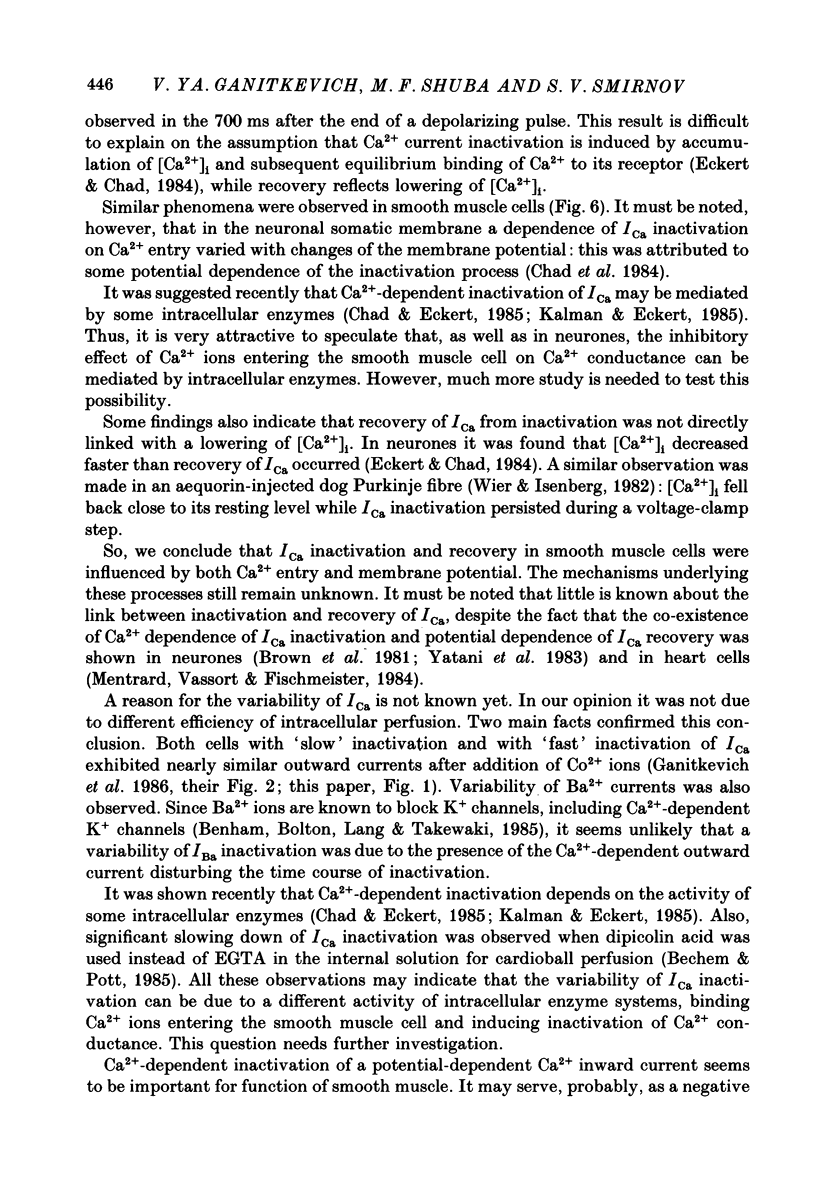
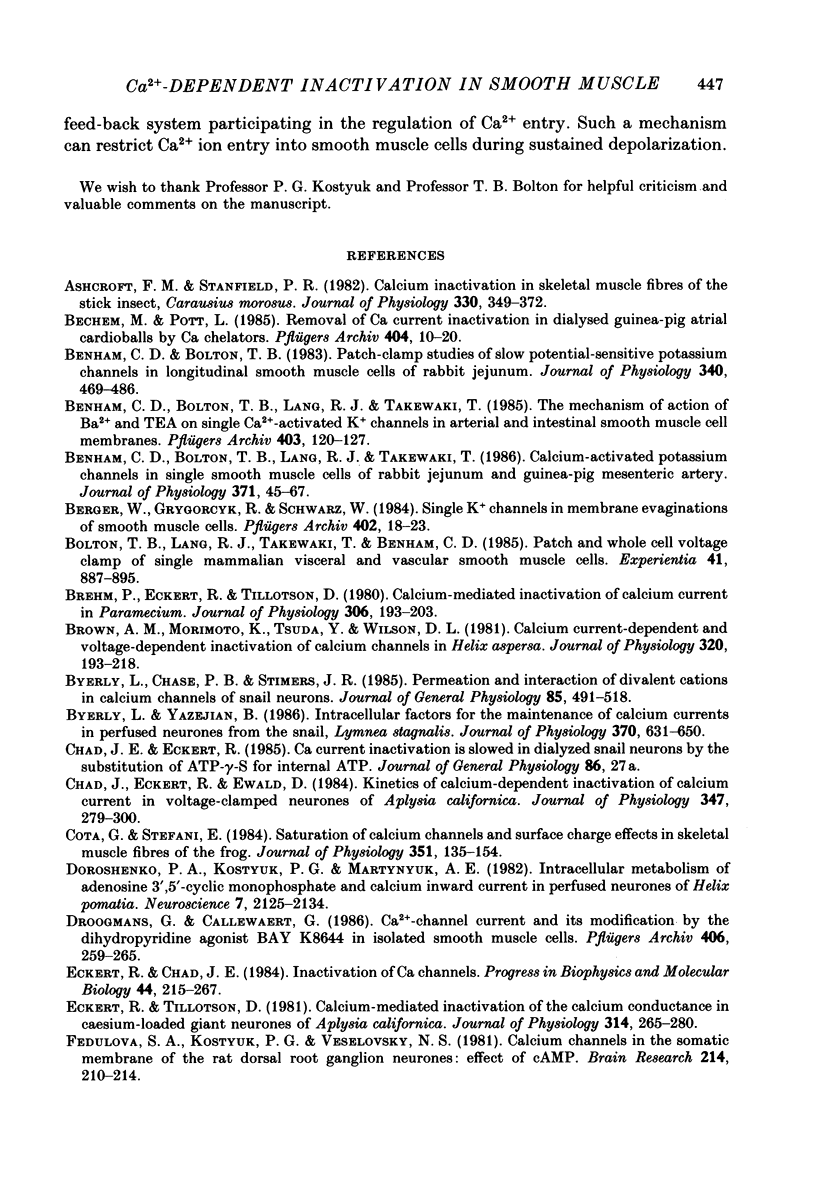
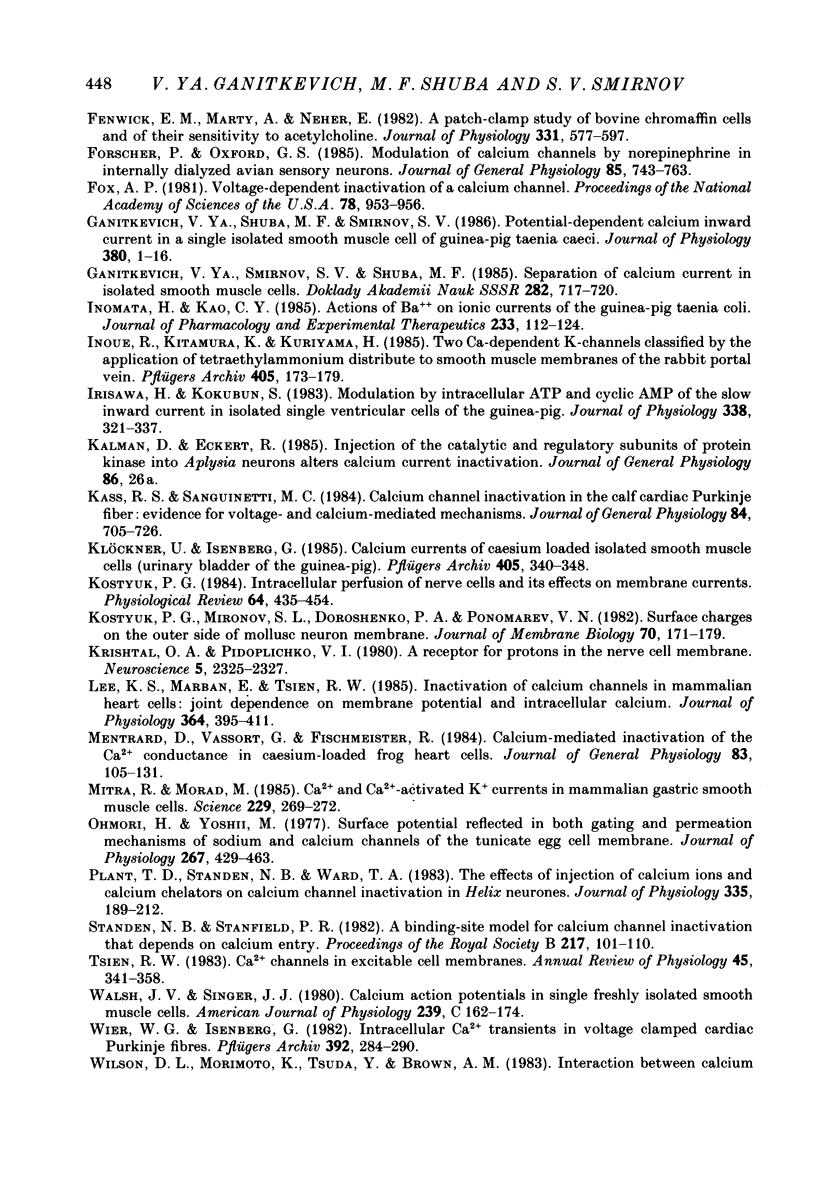
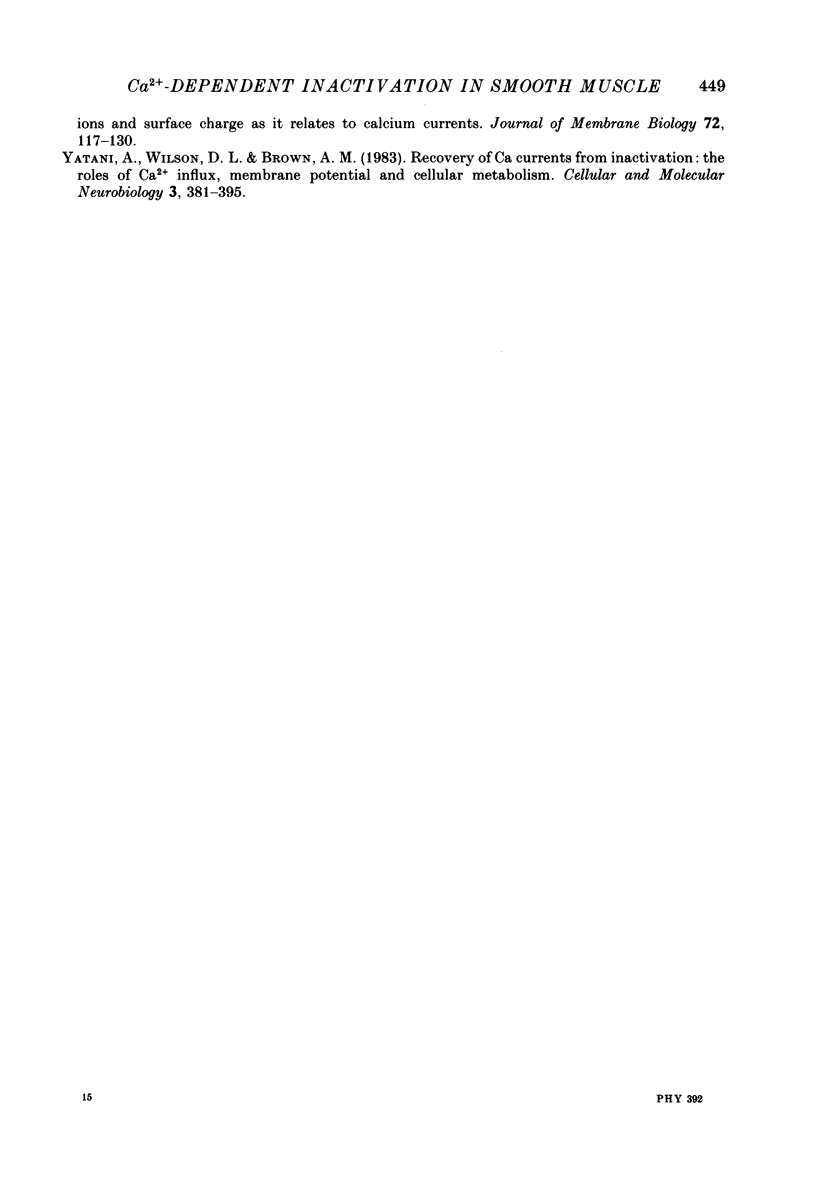
Selected References
These references are in PubMed. This may not be the complete list of references from this article.
- Ashcroft F. M., Stanfield P. R. Calcium inactivation in skeletal muscle fibres of the stick insect, Carausius morosus. J Physiol. 1982 Sep;330:349–372. doi: 10.1113/jphysiol.1982.sp014345. [DOI] [PMC free article] [PubMed] [Google Scholar]
- Bechem M., Pott L. Removal of Ca current inactivation in dialysed guinea-pig atrial cardioballs by Ca chelators. Pflugers Arch. 1985 May;404(1):10–20. doi: 10.1007/BF00581485. [DOI] [PubMed] [Google Scholar]
- Benham C. D., Bolton T. B., Lang R. J., Takewaki T. The mechanism of action of Ba2+ and TEA on single Ca2+-activated K+ -channels in arterial and intestinal smooth muscle cell membranes. Pflugers Arch. 1985 Feb;403(2):120–127. doi: 10.1007/BF00584088. [DOI] [PubMed] [Google Scholar]
- Benham C. D., Bolton T. B. Patch-clamp studies of slow potential-sensitive potassium channels in longitudinal smooth muscle cells of rabbit jejunum. J Physiol. 1983 Jul;340:469–486. doi: 10.1113/jphysiol.1983.sp014774. [DOI] [PMC free article] [PubMed] [Google Scholar]
- Berger W., Grygorcyk R., Schwarz W. Single K+ channels in membrane evaginations of smooth muscle cells. Pflugers Arch. 1984 Sep;402(1):18–23. doi: 10.1007/BF00584826. [DOI] [PubMed] [Google Scholar]
- Bolton T. B., Lang R. J., Takewaki T., Benham C. D. Patch and whole-cell voltage clamp of single mammalian visceral and vascular smooth muscle cells. Experientia. 1985 Jul 15;41(7):887–894. doi: 10.1007/BF01970006. [DOI] [PubMed] [Google Scholar]
- Brehm P., Eckert R., Tillotson D. Calcium-mediated inactivation of calcium current in Paramecium. J Physiol. 1980 Sep;306:193–203. doi: 10.1113/jphysiol.1980.sp013391. [DOI] [PMC free article] [PubMed] [Google Scholar]
- Brown A. M., Morimoto K., Tsuda Y., wilson D. L. Calcium current-dependent and voltage-dependent inactivation of calcium channels in Helix aspersa. J Physiol. 1981 Nov;320:193–218. doi: 10.1113/jphysiol.1981.sp013944. [DOI] [PMC free article] [PubMed] [Google Scholar]
- Byerly L., Chase P. B., Stimers J. R. Permeation and interaction of divalent cations in calcium channels of snail neurons. J Gen Physiol. 1985 Apr;85(4):491–518. doi: 10.1085/jgp.85.4.491. [DOI] [PMC free article] [PubMed] [Google Scholar]
- Byerly L., Yazejian B. Intracellular factors for the maintenance of calcium currents in perfused neurones from the snail, Lymnaea stagnalis. J Physiol. 1986 Jan;370:631–650. doi: 10.1113/jphysiol.1986.sp015955. [DOI] [PMC free article] [PubMed] [Google Scholar]
- Chad J., Eckert R., Ewald D. Kinetics of calcium-dependent inactivation of calcium current in voltage-clamped neurones of Aplysia californica. J Physiol. 1984 Feb;347:279–300. doi: 10.1113/jphysiol.1984.sp015066. [DOI] [PMC free article] [PubMed] [Google Scholar]
- Cota G., Stefani E. Saturation of calcium channels and surface charge effects in skeletal muscle fibres of the frog. J Physiol. 1984 Jun;351:135–154. doi: 10.1113/jphysiol.1984.sp015238. [DOI] [PMC free article] [PubMed] [Google Scholar]
- Doroshenko P. A., Kostyuk P. G., Martynyuk A. E. Intracellular metabolism of adenosine 3',5'-cyclic monophosphate and calcium inward current in perfused neurones of Helix pomatia. Neuroscience. 1982;7(9):2125–2134. doi: 10.1016/0306-4522(82)90124-5. [DOI] [PubMed] [Google Scholar]
- Droogmans G., Callewaert G. Ca2+-channel current and its modification by the dihydropyridine agonist BAY k 8644 in isolated smooth muscle cells. Pflugers Arch. 1986 Mar;406(3):259–265. doi: 10.1007/BF00640911. [DOI] [PubMed] [Google Scholar]
- Eckert R., Chad J. E. Inactivation of Ca channels. Prog Biophys Mol Biol. 1984;44(3):215–267. doi: 10.1016/0079-6107(84)90009-9. [DOI] [PubMed] [Google Scholar]
- Eckert R., Tillotson D. L. Calcium-mediated inactivation of the calcium conductance in caesium-loaded giant neurones of Aplysia californica. J Physiol. 1981 May;314:265–280. doi: 10.1113/jphysiol.1981.sp013706. [DOI] [PMC free article] [PubMed] [Google Scholar]
- Fedulova S. A., Kostyuk P. G., Veselovsky N. S. Calcium channels in the somatic membrane of the rat dorsal root ganglion neurons, effect of cAMP. Brain Res. 1981 Jun 9;214(1):210–214. doi: 10.1016/0006-8993(81)90457-1. [DOI] [PubMed] [Google Scholar]
- Fenwick E. M., Marty A., Neher E. A patch-clamp study of bovine chromaffin cells and of their sensitivity to acetylcholine. J Physiol. 1982 Oct;331:577–597. doi: 10.1113/jphysiol.1982.sp014393. [DOI] [PMC free article] [PubMed] [Google Scholar]
- Forscher P., Oxford G. S. Modulation of calcium channels by norepinephrine in internally dialyzed avian sensory neurons. J Gen Physiol. 1985 May;85(5):743–763. doi: 10.1085/jgp.85.5.743. [DOI] [PMC free article] [PubMed] [Google Scholar]
- Fox A. P. Voltage-dependent inactivation of a calcium channel. Proc Natl Acad Sci U S A. 1981 Feb;78(2):953–956. doi: 10.1073/pnas.78.2.953. [DOI] [PMC free article] [PubMed] [Google Scholar]
- Ganitkevich VYa, Shuba M. F., Smirnov S. V. Potential-dependent calcium inward current in a single isolated smooth muscle cell of the guinea-pig taenia caeci. J Physiol. 1986 Nov;380:1–16. doi: 10.1113/jphysiol.1986.sp016268. [DOI] [PMC free article] [PubMed] [Google Scholar]
- Ganitkevich V. Ia, Smirnov S. V., Shuba M. F. Vydelenie kal'tsievogo toka v izolirovannykh gladkomyshechnykh kletkakh. Dokl Akad Nauk SSSR. 1985;282(3):717–720. [PubMed] [Google Scholar]
- Inomata H., Kao C. Y. Actions of Ba++ on ionic currents of the guinea-pig taenia coli. J Pharmacol Exp Ther. 1985 Apr;233(1):112–124. [PubMed] [Google Scholar]
- Inoue R., Kitamura K., Kuriyama H. Two Ca-dependent K-channels classified by the application of tetraethylammonium distribute to smooth muscle membranes of the rabbit portal vein. Pflugers Arch. 1985 Oct;405(3):173–179. doi: 10.1007/BF00582557. [DOI] [PubMed] [Google Scholar]
- Irisawa H., Kokubun S. Modulation by intracellular ATP and cyclic AMP of the slow inward current in isolated single ventricular cells of the guinea-pig. J Physiol. 1983 May;338:321–337. doi: 10.1113/jphysiol.1983.sp014675. [DOI] [PMC free article] [PubMed] [Google Scholar]
- Kass R. S., Sanguinetti M. C. Inactivation of calcium channel current in the calf cardiac Purkinje fiber. Evidence for voltage- and calcium-mediated mechanisms. J Gen Physiol. 1984 Nov;84(5):705–726. doi: 10.1085/jgp.84.5.705. [DOI] [PMC free article] [PubMed] [Google Scholar]
- Klöckner U., Isenberg G. Calcium currents of cesium loaded isolated smooth muscle cells (urinary bladder of the guinea pig). Pflugers Arch. 1985 Dec;405(4):340–348. doi: 10.1007/BF00595686. [DOI] [PubMed] [Google Scholar]
- Kostyuk P. G. Intracellular perfusion of nerve cells and its effects on membrane currents. Physiol Rev. 1984 Apr;64(2):435–454. doi: 10.1152/physrev.1984.64.2.435. [DOI] [PubMed] [Google Scholar]
- Krishtal O. A., Pidoplichko V. I. A receptor for protons in the nerve cell membrane. Neuroscience. 1980;5(12):2325–2327. doi: 10.1016/0306-4522(80)90149-9. [DOI] [PubMed] [Google Scholar]
- Lee K. S., Marban E., Tsien R. W. Inactivation of calcium channels in mammalian heart cells: joint dependence on membrane potential and intracellular calcium. J Physiol. 1985 Jul;364:395–411. doi: 10.1113/jphysiol.1985.sp015752. [DOI] [PMC free article] [PubMed] [Google Scholar]
- Mentrard D., Vassort G., Fischmeister R. Calcium-mediated inactivation of the calcium conductance in cesium-loaded frog heart cells. J Gen Physiol. 1984 Jan;83(1):105–131. doi: 10.1085/jgp.83.1.105. [DOI] [PMC free article] [PubMed] [Google Scholar]
- Mitra R., Morad M. Ca2+ and Ca2+-activated K+ currents in mammalian gastric smooth muscle cells. Science. 1985 Jul 19;229(4710):269–272. doi: 10.1126/science.2409600. [DOI] [PubMed] [Google Scholar]
- Ohmori H., Yoshii M. Surface potential reflected in both gating and permeation mechanisms of sodium and calcium channels of the tunicate egg cell membrane. J Physiol. 1977 May;267(2):429–463. doi: 10.1113/jphysiol.1977.sp011821. [DOI] [PMC free article] [PubMed] [Google Scholar]
- Plant T. D., Standen N. B., Ward T. A. The effects of injection of calcium ions and calcium chelators on calcium channel inactivation in Helix neurones. J Physiol. 1983 Jan;334:189–212. doi: 10.1113/jphysiol.1983.sp014489. [DOI] [PMC free article] [PubMed] [Google Scholar]
- Standen N. B., Stanfield P. R. A binding-site model for calcium channel inactivation that depends on calcium entry. Proc R Soc Lond B Biol Sci. 1982 Dec 22;217(1206):101–110. doi: 10.1098/rspb.1982.0097. [DOI] [PubMed] [Google Scholar]
- Tsien R. W. Calcium channels in excitable cell membranes. Annu Rev Physiol. 1983;45:341–358. doi: 10.1146/annurev.ph.45.030183.002013. [DOI] [PubMed] [Google Scholar]
- Wier W. G., Isenberg G. Intracellular [Ca2+] transients in voltage clamped cardiac Purkinje fibers. Pflugers Arch. 1982 Jan;392(3):284–290. doi: 10.1007/BF00584312. [DOI] [PubMed] [Google Scholar]
- Wilson D. L., Morimoto K., Tsuda Y., Brown A. M. Interaction between calcium ions and surface charge as it relates to calcium currents. J Membr Biol. 1983;72(1-2):117–130. doi: 10.1007/BF01870319. [DOI] [PubMed] [Google Scholar]
- Yatani A., Wilson D. L., Brown A. M. Recovery of Ca currents from inactivation: the roles of Ca influx, membrane potential, and cellular metabolism. Cell Mol Neurobiol. 1983 Dec;3(4):381–395. doi: 10.1007/BF00734718. [DOI] [PubMed] [Google Scholar]