Abstract
1. heat production and high-energy phosphate hydrolysis by frog sartorius muscles in 6 s isometric tetani were measured to test the hypothesis that the isometric unexplained enthalpy (u.e.) and labile maintenance heat (l.m.h.) were of similar origin. Muscles were first given a conditioning tetanus to deplete the u.e. and l.m.h. A second (test) 6 s tetanus was given 6-300 s later to ascertain the extent to which l.m.h. and u.e. had recovered (reprimed). 2. The labile maintenance heat repriming was biphasic: 42% of the conditioning l.m.h. reprimed with a time constant of 10 s, the remainder with a time constant of 500 s. 3. The u.e. produced in the test tetanus 6 s after the conditioning tetanus was reduced to 18% of its conditioning value. By 30 s, u.e. had returned to conditioning values even though the amount of high-energy phosphate splitting was 17% less than that in the conditioning tetanus. 4. This observation is supported by measurements revealing that during the 30 s following a 6 s tetanus an amount of enthalpy was absorbed (less heat produced than expected from the measured metabolic changes) whose absolute value (after correction for oxygen consumption) was not different from the amount of unexplained enthalpy liberated during the tetanus. 5. The difference in repriming time course shows that l.m.h. and u.e. are not produced by the same reactions. The data are consistent with the hypothesis that calcium binding to troponin and parvalbumin produce u.e. while calcium binding to troponin and a non-linear time course of ATP hydrolysis produce l.m.h.
Full text
PDF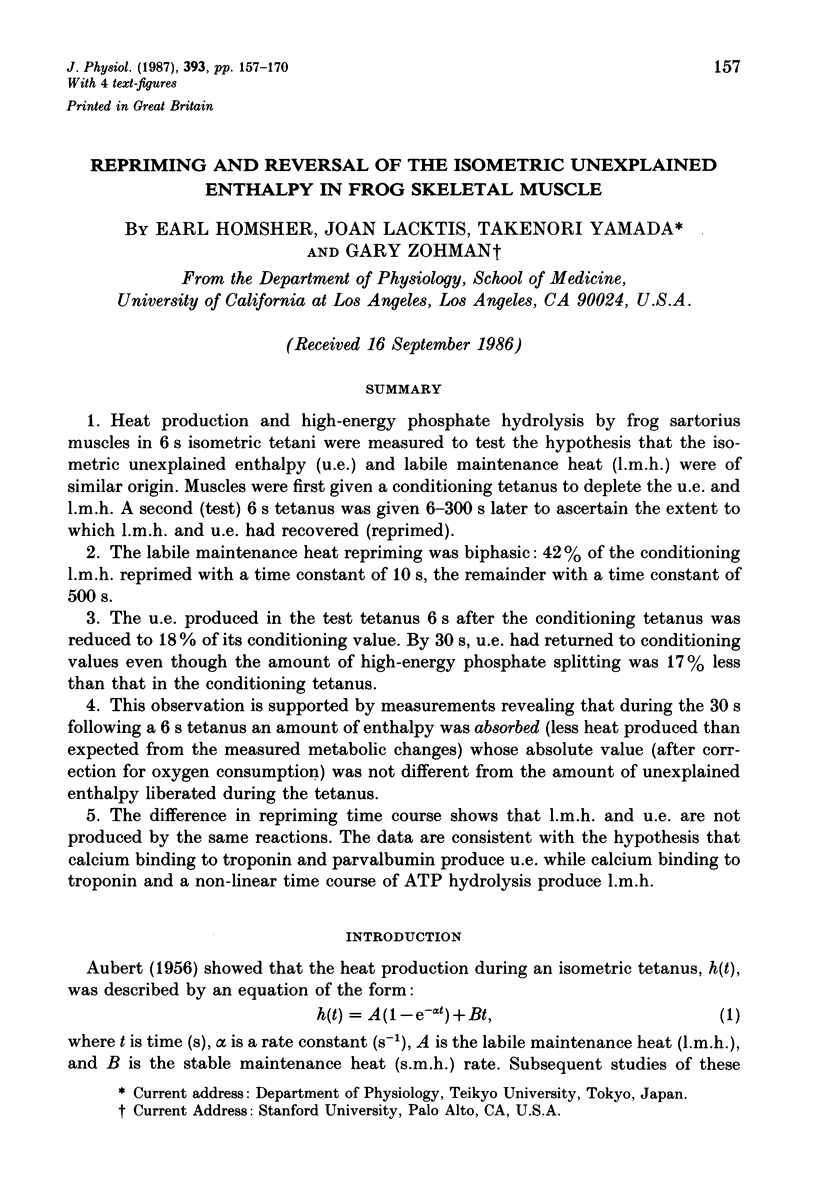
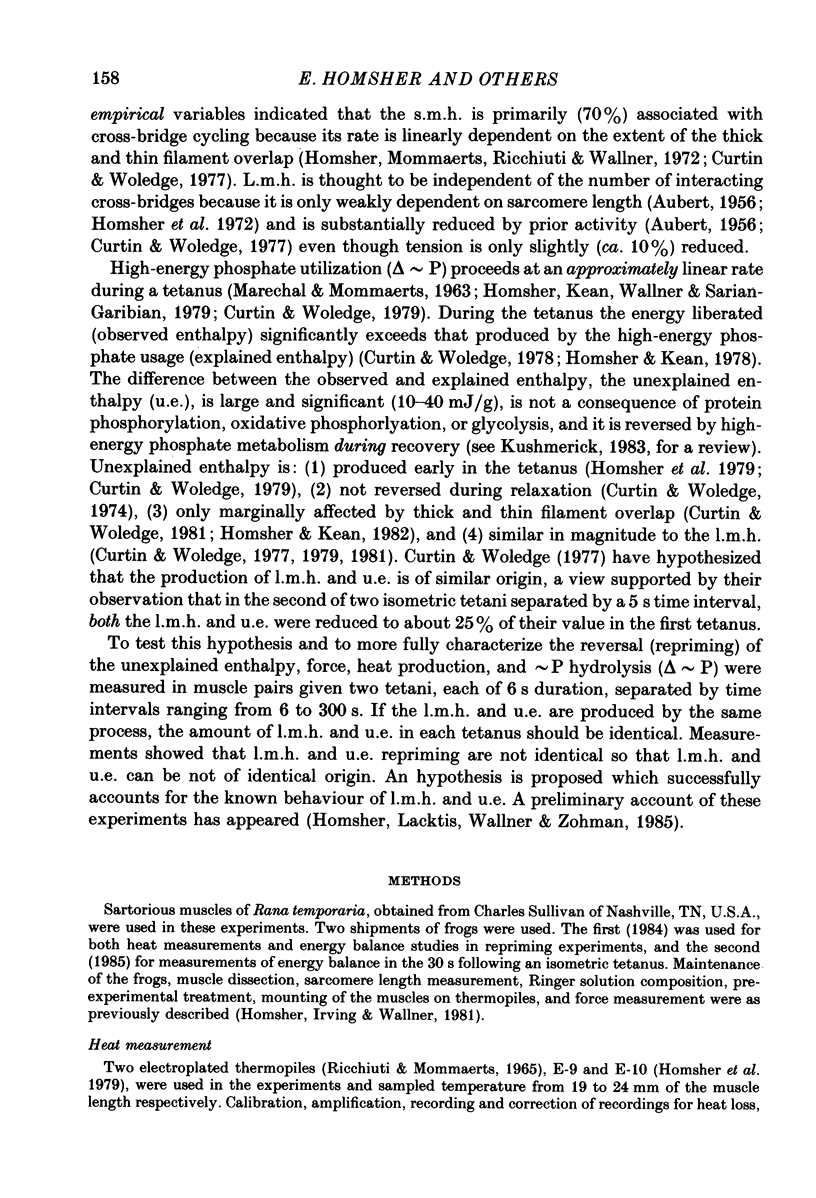
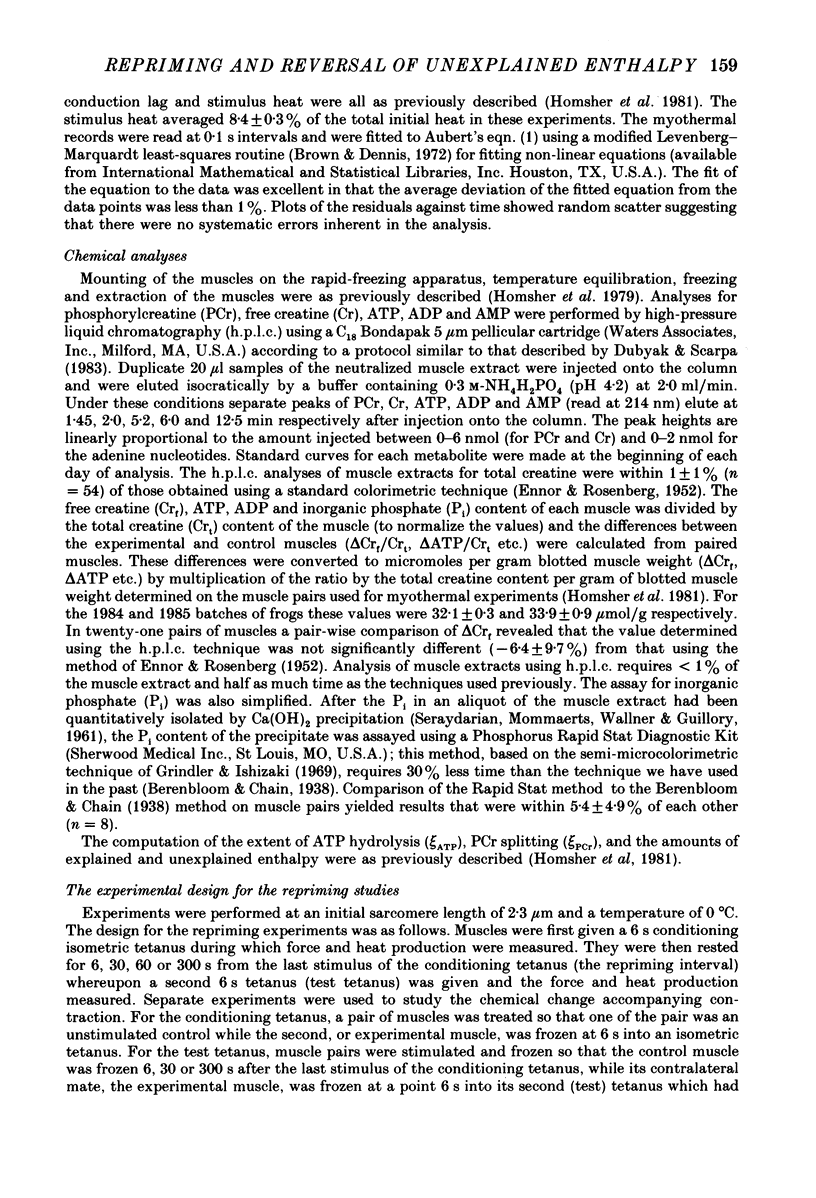
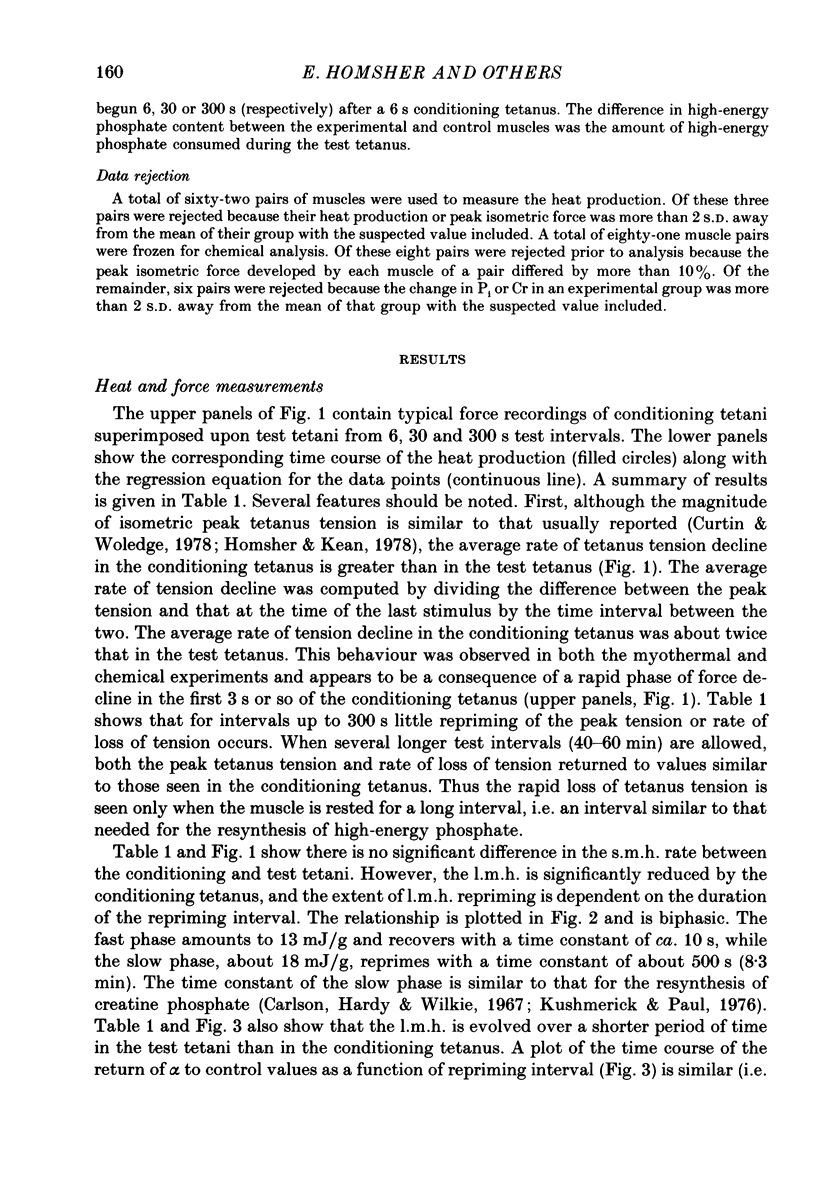
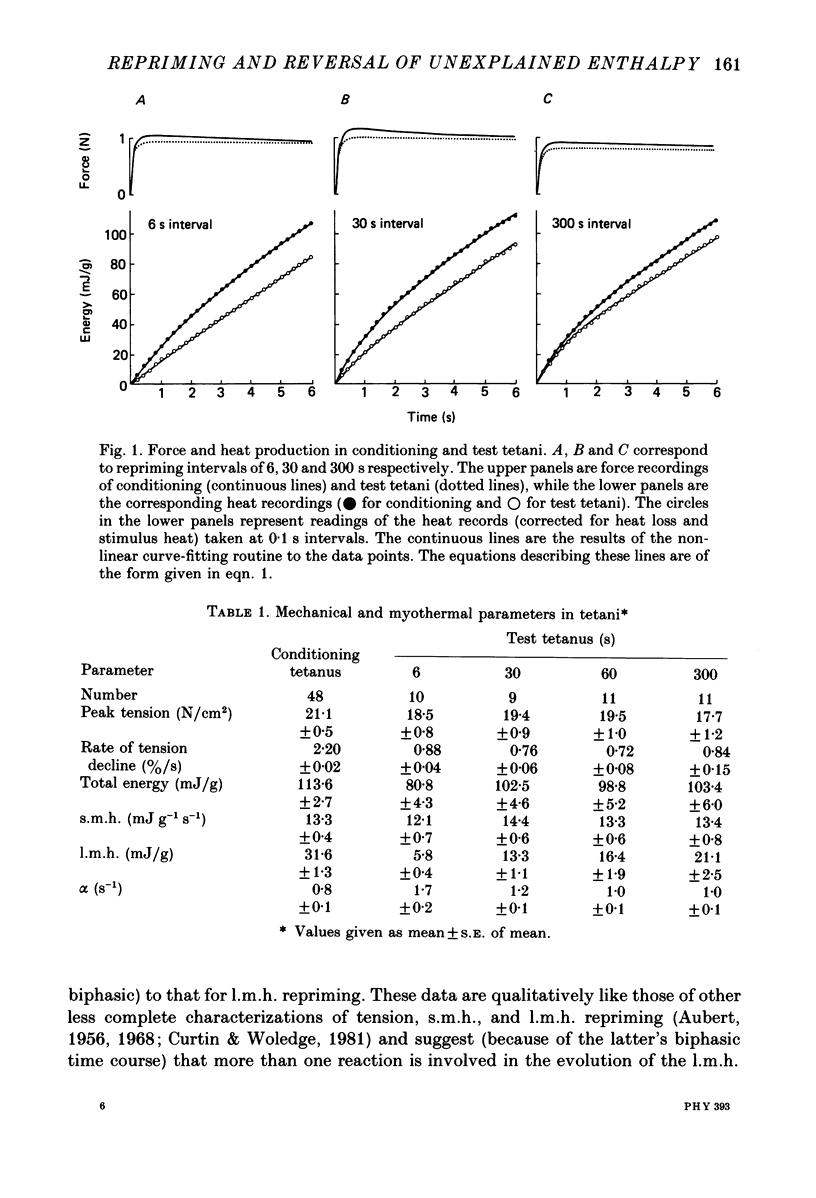
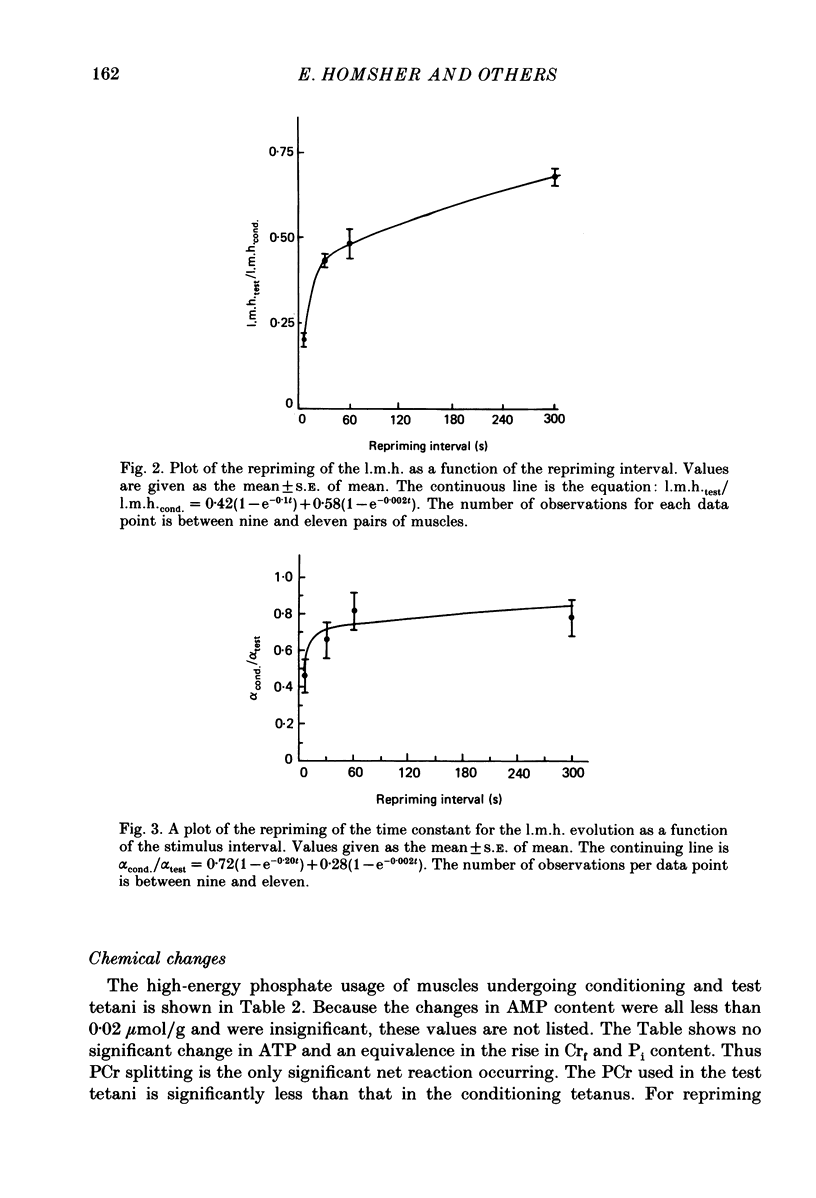
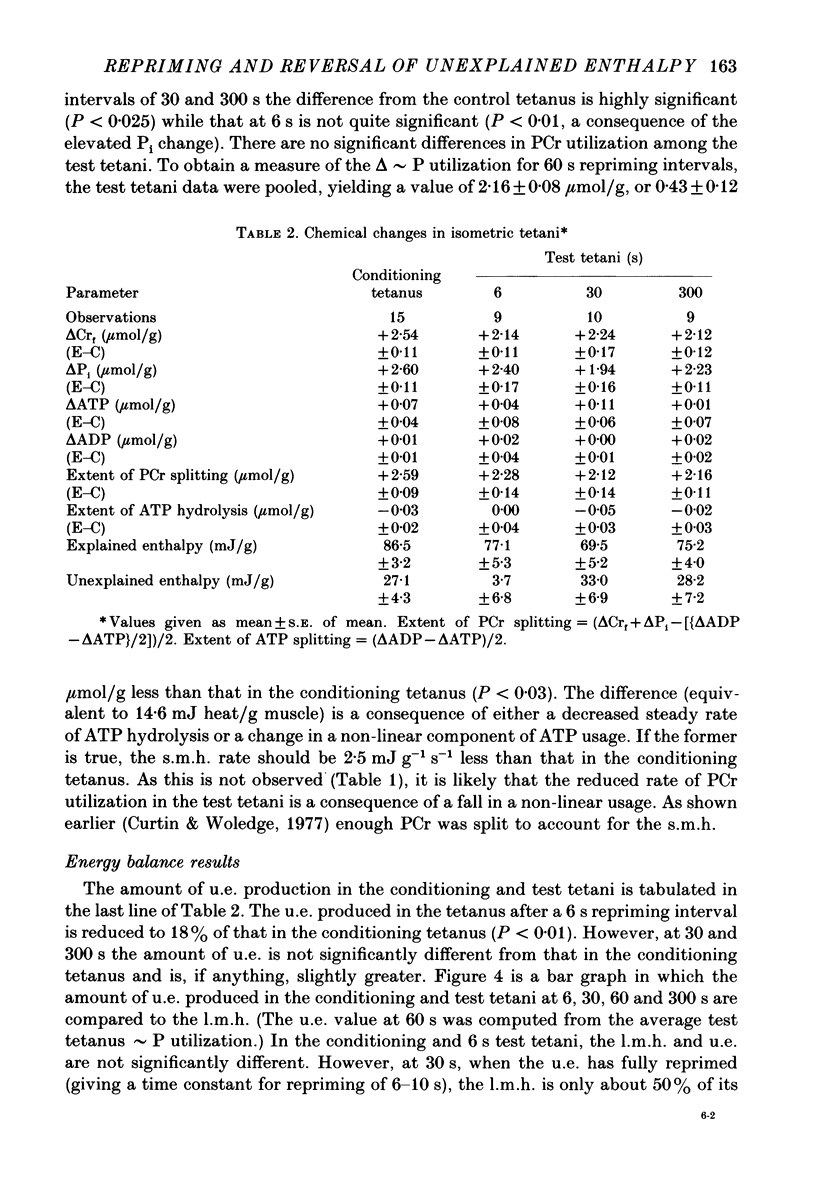
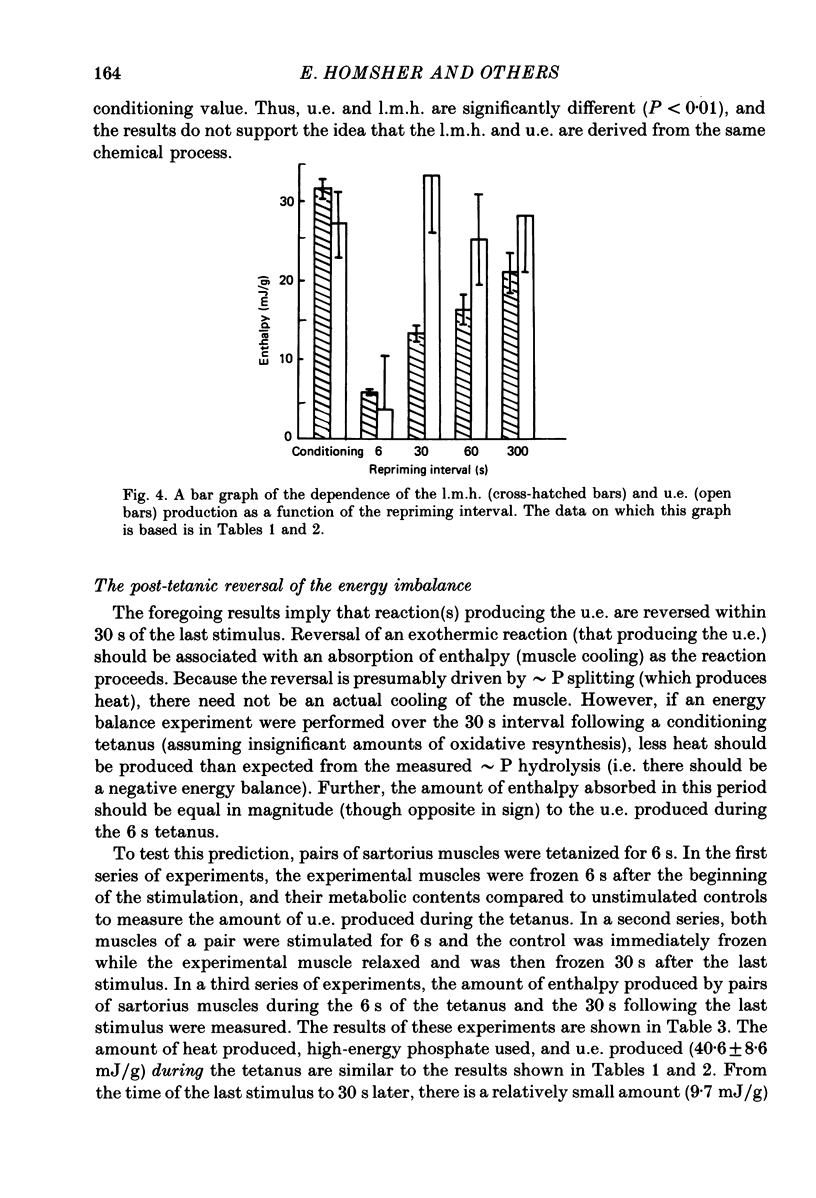
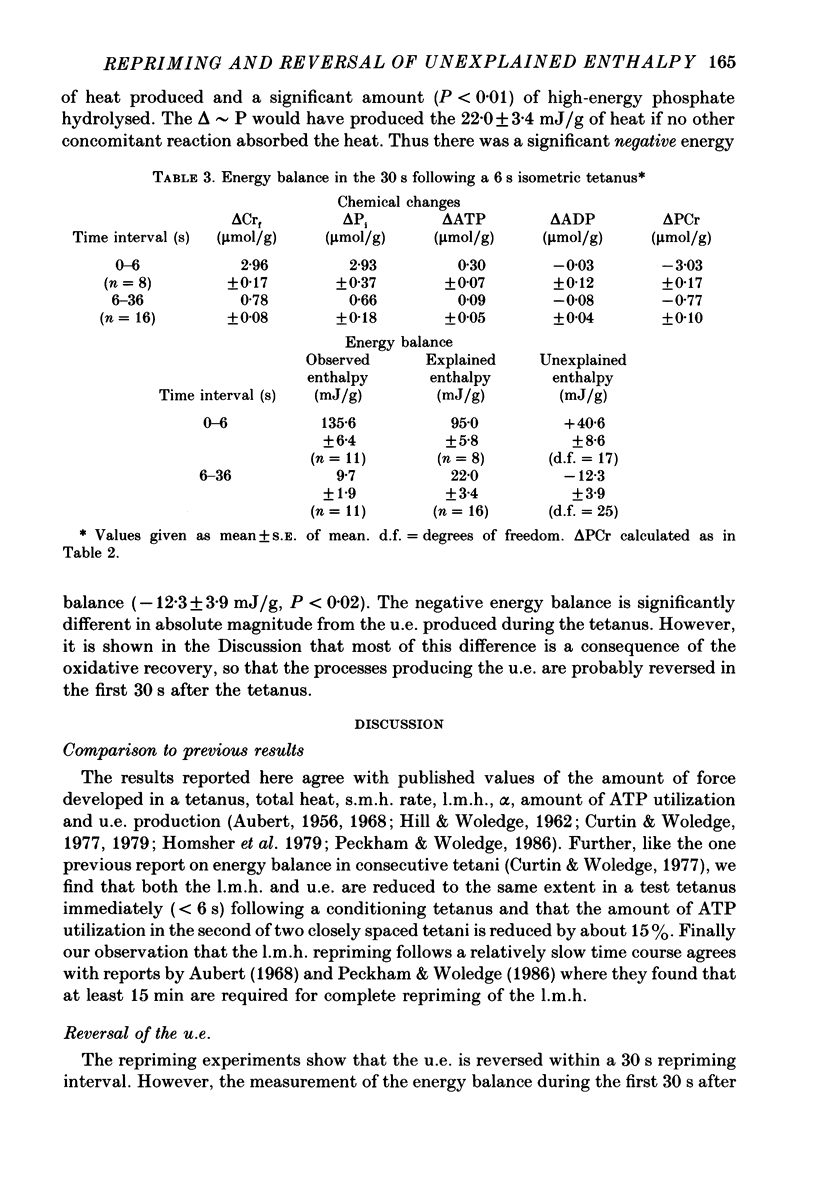
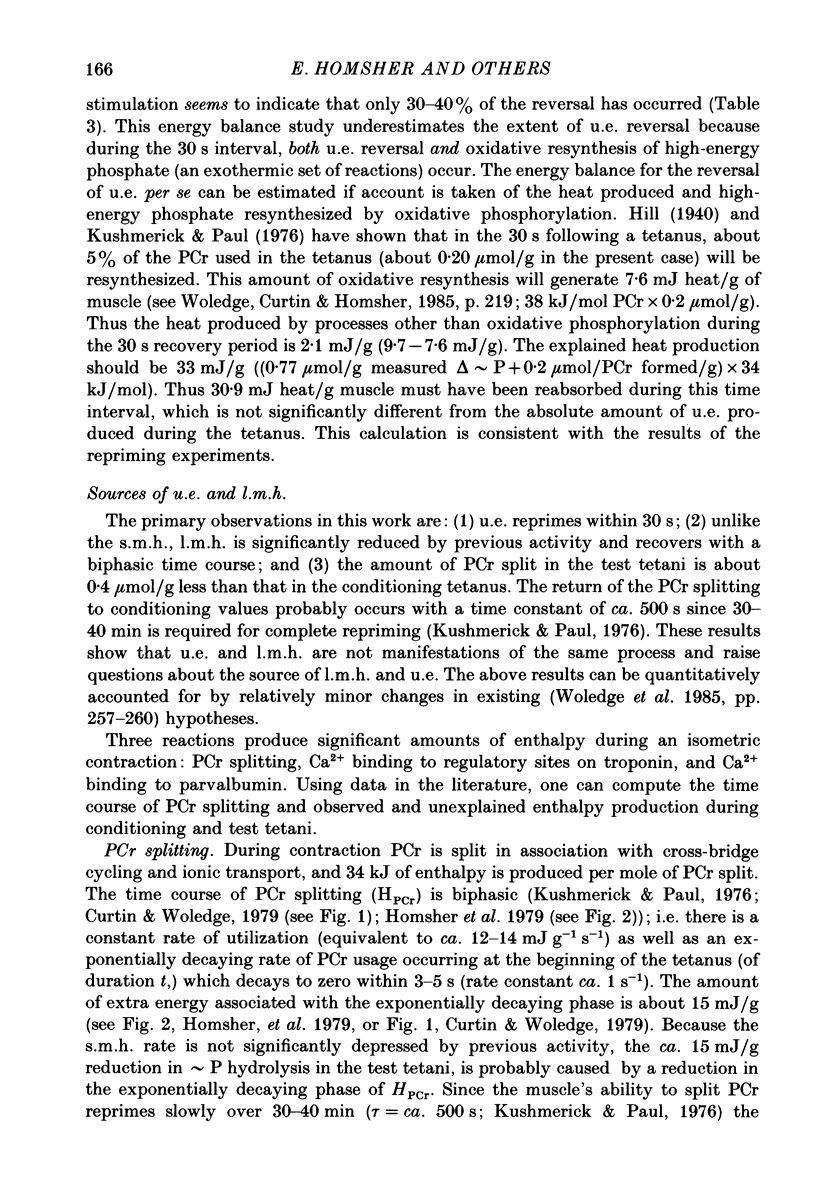
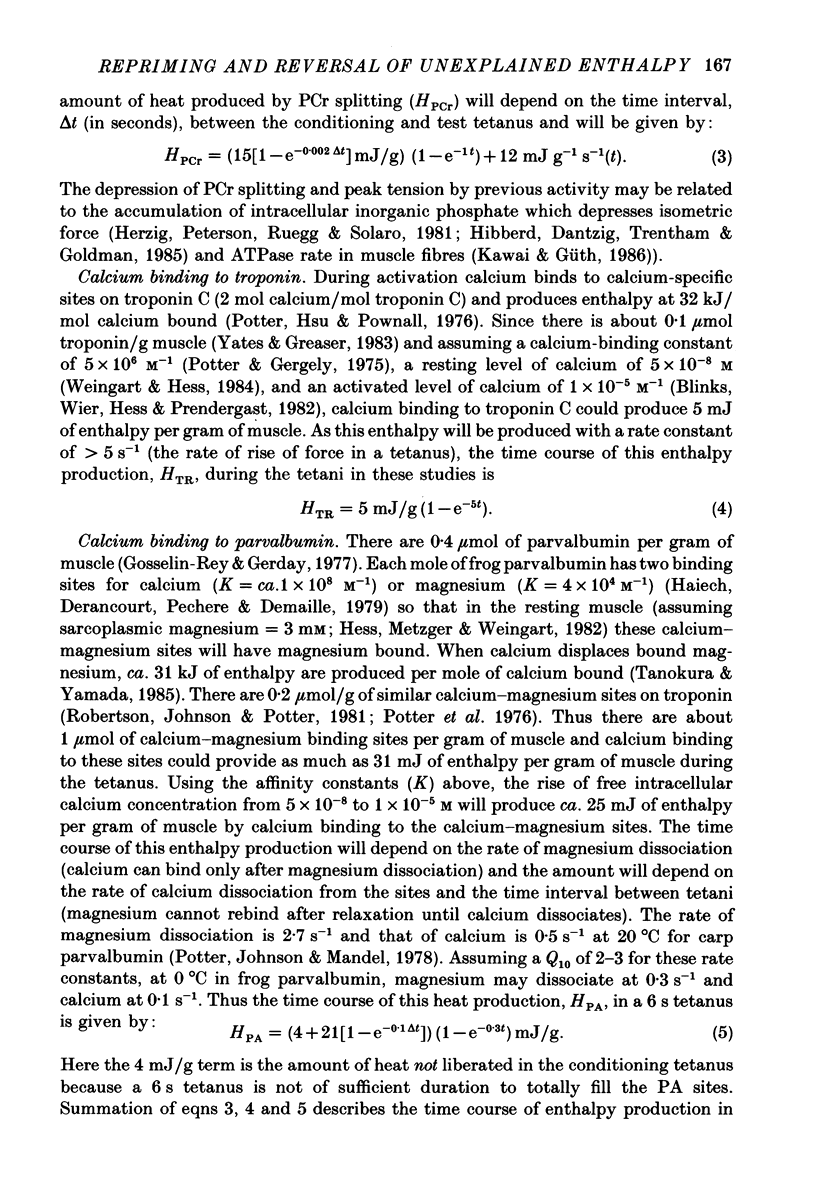
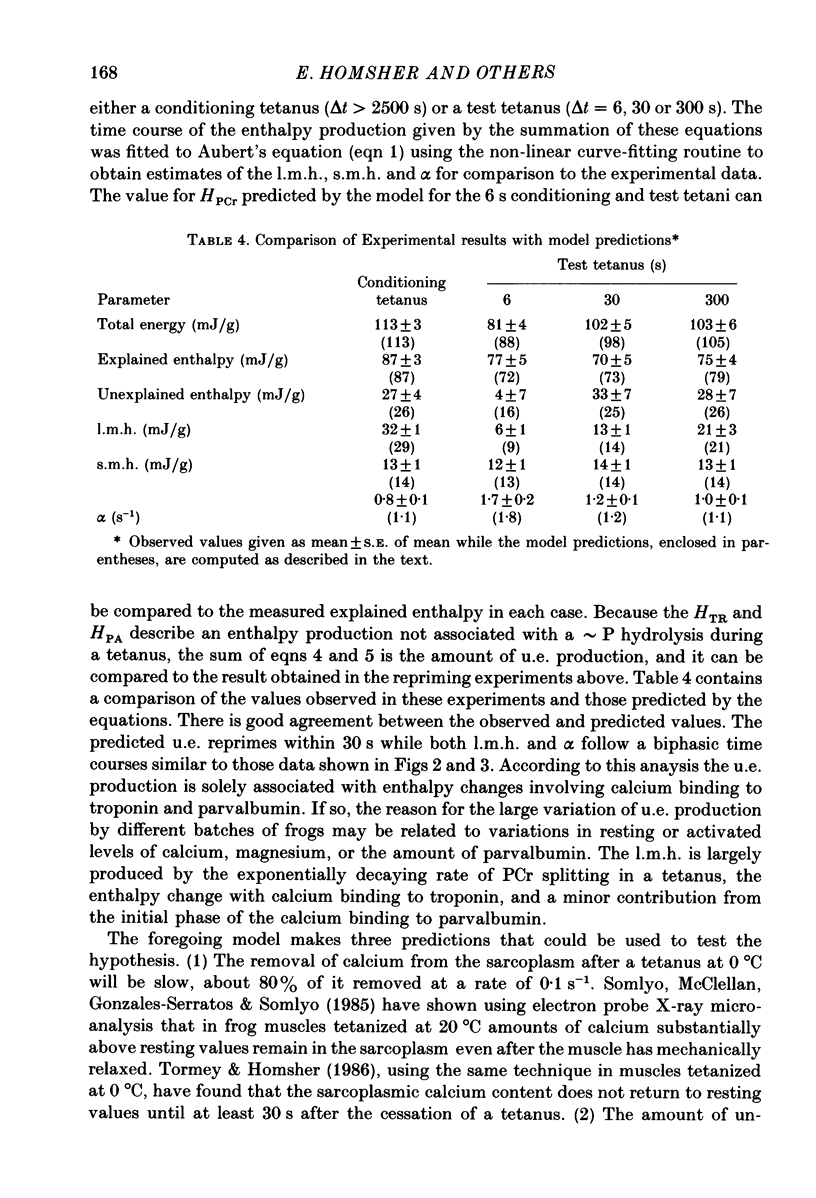
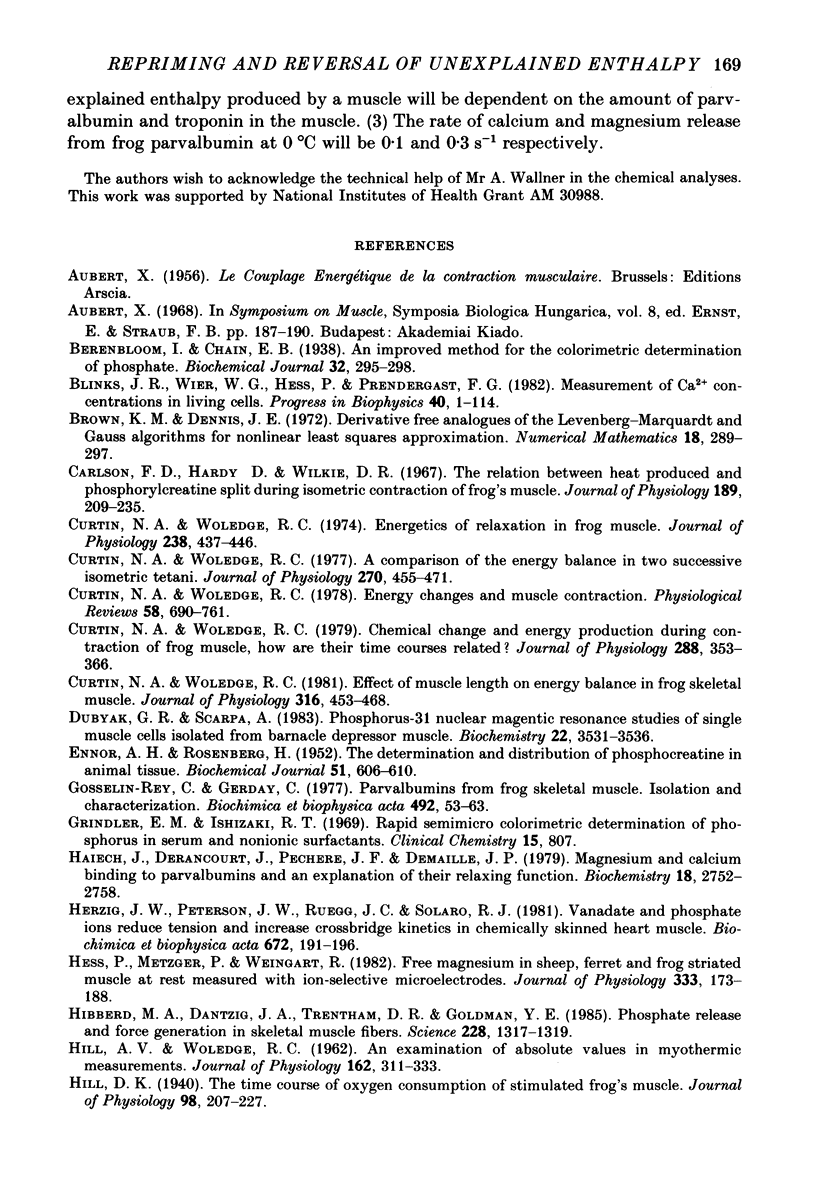
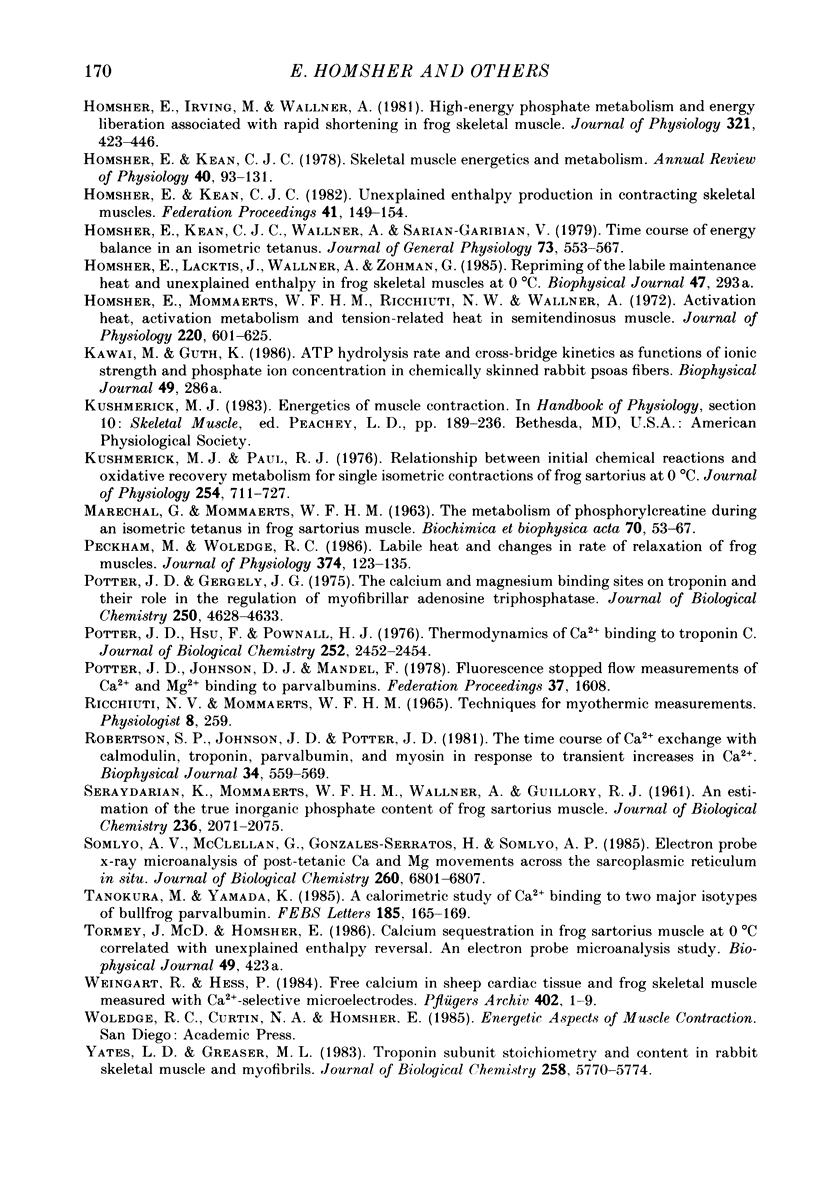
Selected References
These references are in PubMed. This may not be the complete list of references from this article.
- Berenblum I., Chain E. An improved method for the colorimetric determination of phosphate. Biochem J. 1938 Feb;32(2):295–298. doi: 10.1042/bj0320295. [DOI] [PMC free article] [PubMed] [Google Scholar]
- Blinks J. R., Wier W. G., Hess P., Prendergast F. G. Measurement of Ca2+ concentrations in living cells. Prog Biophys Mol Biol. 1982;40(1-2):1–114. doi: 10.1016/0079-6107(82)90011-6. [DOI] [PubMed] [Google Scholar]
- Carlson F. D., Hardy D., Wilkie D. R. The relation between heat produced and phosphorylcreatine split during isometric contraction of frog's muscle. J Physiol. 1967 Apr;189(2):209–235. doi: 10.1113/jphysiol.1967.sp008164. [DOI] [PMC free article] [PubMed] [Google Scholar]
- Curtin N. A., Woledge R. C. A comparison of the energy balance in two successive isometric tetani of frog muscle. J Physiol. 1977 Sep;270(2):455–471. doi: 10.1113/jphysiol.1977.sp011962. [DOI] [PMC free article] [PubMed] [Google Scholar]
- Curtin N. A., Woledge R. C. Chemical change and energy production during contraction of frog muscle: how are their time courses related? J Physiol. 1979 Mar;288:353–366. [PMC free article] [PubMed] [Google Scholar]
- Curtin N. A., Woledge R. C. Effect of muscle length on energy balance in frog skeletal muscle. J Physiol. 1981 Jul;316:453–468. doi: 10.1113/jphysiol.1981.sp013800. [DOI] [PMC free article] [PubMed] [Google Scholar]
- Curtin N. A., Woledge R. C. Energetics of relaxation in frog muscle. J Physiol. 1974 Apr;238(2):437–446. doi: 10.1113/jphysiol.1974.sp010535. [DOI] [PMC free article] [PubMed] [Google Scholar]
- Curtin N. A., Woledge R. C. Energy changes and muscular contraction. Physiol Rev. 1978 Jul;58(3):690–761. doi: 10.1152/physrev.1978.58.3.690. [DOI] [PubMed] [Google Scholar]
- Dubyak G. R., Scarpa A. Phosphorus-31 nuclear magnetic resonance studies of single muscle cells isolated from barnacle depressor muscle. Biochemistry. 1983 Jul 5;22(14):3531–3536. doi: 10.1021/bi00283a035. [DOI] [PubMed] [Google Scholar]
- ENNOR A. H., ROSENBERG H. The determination and distribution of phosphocreatine in animal tissues. Biochem J. 1952 Aug;51(5):606–610. doi: 10.1042/bj0510606. [DOI] [PMC free article] [PubMed] [Google Scholar]
- Gosselin-rey C., Gerday C. Parvalbumins from frog skeletal muscle (Rana temporaria L.). Isolation and characterization. Structural modifications associated with calcium binding. Biochim Biophys Acta. 1977 May 27;492(1):53–63. doi: 10.1016/0005-2795(77)90213-6. [DOI] [PubMed] [Google Scholar]
- HILL A. V., WOLEDGE R. C. An examination of absolute values in myothermic measurements. J Physiol. 1962 Jul;162:311–333. doi: 10.1113/jphysiol.1962.sp006935. [DOI] [PMC free article] [PubMed] [Google Scholar]
- Haiech J., Derancourt J., Pechère J. F., Demaille J. G. Magnesium and calcium binding to parvalbumins: evidence for differences between parvalbumins and an explanation of their relaxing function. Biochemistry. 1979 Jun 26;18(13):2752–2758. doi: 10.1021/bi00580a010. [DOI] [PubMed] [Google Scholar]
- Herzig J. W., Peterson J. W., Rüegg J. C., Solaro R. J. Vanadate and phosphate ions reduce tension and increase cross-bridge kinetics in chemically skinned heart muscle. Biochim Biophys Acta. 1981 Jan 21;672(2):191–196. doi: 10.1016/0304-4165(81)90392-5. [DOI] [PubMed] [Google Scholar]
- Hess P., Metzger P., Weingart R. Free magnesium in sheep, ferret and frog striated muscle at rest measured with ion-selective micro-electrodes. J Physiol. 1982 Dec;333:173–188. doi: 10.1113/jphysiol.1982.sp014447. [DOI] [PMC free article] [PubMed] [Google Scholar]
- Hibberd M. G., Dantzig J. A., Trentham D. R., Goldman Y. E. Phosphate release and force generation in skeletal muscle fibers. Science. 1985 Jun 14;228(4705):1317–1319. doi: 10.1126/science.3159090. [DOI] [PubMed] [Google Scholar]
- Hill D. K. The time course of the oxygen consumption of stimulated frog's muscle. J Physiol. 1940 May 14;98(2):207–227. doi: 10.1113/jphysiol.1940.sp003845. [DOI] [PMC free article] [PubMed] [Google Scholar]
- Homsher E., Irving M., Wallner A. High-energy phosphate metabolism and energy liberation associated with rapid shortening in frog skeletal muscle. J Physiol. 1981 Dec;321:423–436. doi: 10.1113/jphysiol.1981.sp013994. [DOI] [PMC free article] [PubMed] [Google Scholar]
- Homsher E., Kean C. J. Skeletal muscle energetics and metabolism. Annu Rev Physiol. 1978;40:93–131. doi: 10.1146/annurev.ph.40.030178.000521. [DOI] [PubMed] [Google Scholar]
- Homsher E., Kean C. J. Unexplained enthalpy production in contracting skeletal muscles. Fed Proc. 1982 Feb;41(2):149–154. [PubMed] [Google Scholar]
- Homsher E., Kean C. J., Wallner A., Garibian-Sarian V. The time-course of energy balance in an isometric tetanus. J Gen Physiol. 1979 May;73(5):553–567. doi: 10.1085/jgp.73.5.553. [DOI] [PMC free article] [PubMed] [Google Scholar]
- Homsher E., Mommaerts W. F., Ricchiuti N. V., Wallner A. Activation heat, activation metabolism and tension-related heat in frog semitendinosus muscles. J Physiol. 1972 Feb;220(3):601–625. doi: 10.1113/jphysiol.1972.sp009725. [DOI] [PMC free article] [PubMed] [Google Scholar]
- Kushmerick M. J., Paul R. J. Relationship between initial chemical reactions and oxidative recovery metabolism for single isometric contractions of frog sartorius at 0 degrees C. J Physiol. 1976 Jan;254(3):711–727. doi: 10.1113/jphysiol.1976.sp011254. [DOI] [PMC free article] [PubMed] [Google Scholar]
- Peckham M., Woledge R. C. Labile heat and changes in rate of relaxation of frog muscles. J Physiol. 1986 May;374:123–135. doi: 10.1113/jphysiol.1986.sp016070. [DOI] [PMC free article] [PubMed] [Google Scholar]
- Potter J. D., Gergely J. The calcium and magnesium binding sites on troponin and their role in the regulation of myofibrillar adenosine triphosphatase. J Biol Chem. 1975 Jun 25;250(12):4628–4633. [PubMed] [Google Scholar]
- Potter J. D., Hsu F. J., Pownall H. J. Thermodynamics of Ca2+ binding to troponin-C. J Biol Chem. 1977 Apr 10;252(7):2452–2454. [PubMed] [Google Scholar]
- Robertson S. P., Johnson J. D., Potter J. D. The time-course of Ca2+ exchange with calmodulin, troponin, parvalbumin, and myosin in response to transient increases in Ca2+. Biophys J. 1981 Jun;34(3):559–569. doi: 10.1016/S0006-3495(81)84868-0. [DOI] [PMC free article] [PubMed] [Google Scholar]
- SERAYDARIAN K., MOMMAERTS W. F., WALLNER A., GUILLORY R. J. An estimation of the true inorganic phosphate content of frog sartorius muscle. J Biol Chem. 1961 Jul;236:2071–2075. [PubMed] [Google Scholar]
- Somlyo A. V., McClellan G., Gonzalez-Serratos H., Somlyo A. P. Electron probe X-ray microanalysis of post-tetanic Ca2+ and Mg2+ movements across the sarcoplasmic reticulum in situ. J Biol Chem. 1985 Jun 10;260(11):6801–6807. [PubMed] [Google Scholar]
- Tanokura M., Yamada K. A calorimetric study of Ca2+ binding to two major isotypes of bullfrog parvalbumin. FEBS Lett. 1985 Jun 3;185(1):165–169. doi: 10.1016/0014-5793(85)80763-8. [DOI] [PubMed] [Google Scholar]
- Weingart R., Hess P. Free calcium in sheep cardiac tissue and frog skeletal muscle measured with Ca2+-selective microelectrodes. Pflugers Arch. 1984 Sep;402(1):1–9. doi: 10.1007/BF00584824. [DOI] [PubMed] [Google Scholar]
- Yates L. D., Greaser M. L. Troponin subunit stoichiometry and content in rabbit skeletal muscle and myofibrils. J Biol Chem. 1983 May 10;258(9):5770–5774. [PubMed] [Google Scholar]