Abstract
1. Herbivores have higher rates of intestinal sugar transport and lower rates of amino acid transport than carnivores, if each are studied while eating their respective natural diets. It was unclear whether these species differences involve a genetic contribution, since when omnivores are switched from a high-protein to a high-carbohydrate diet they reversibly increase sugar transport and suppress amino acid transport. Hence we studied eight fish species of differing natural diets while all were eating the same manufactured diet. 2. Na+-dependent L-proline uptake and active D-glucose uptake, measured in vitro by the everted intestinal sleeve technique, followed Michaelis-Menten kinetics. Values of the apparent Michaelis-Menten constant increased with values of the maximal transport rate, probably as a result of unstirred layer effects. 3. The ratio of proline to glucose uptake decreased in the sequence: carnivores greater than omnivores greater than herbivores. The intestine's uptake capacity for the non-essential nutrient glucose was much higher in herbivores than in carnivores, correlated with species differences in carbohydrate content of the natural diet. Proline uptake varied much less among species, since species with different natural diets still have similar protein requirements. 4. Since all species were studied while eating the same diet, these species differences in uptake are not phenotypic but genetic adaptations to the different natural diets. 5. In two fish species which normally switch from carnivory towards herbivory or omnivory as they mature, we observe a 'hard-wired' developmental change in intestinal uptake. Larger animals had lower proline uptake relative to glucose uptake than did smaller animals, even though both were being maintained on the same diet in the laboratory. 6. Carnivorous fish tend to allocate absorptive tissue to pyloric caeca or a thick mucosa, while herbivorous fish tend towards a long thin intestine.
Full text
PDF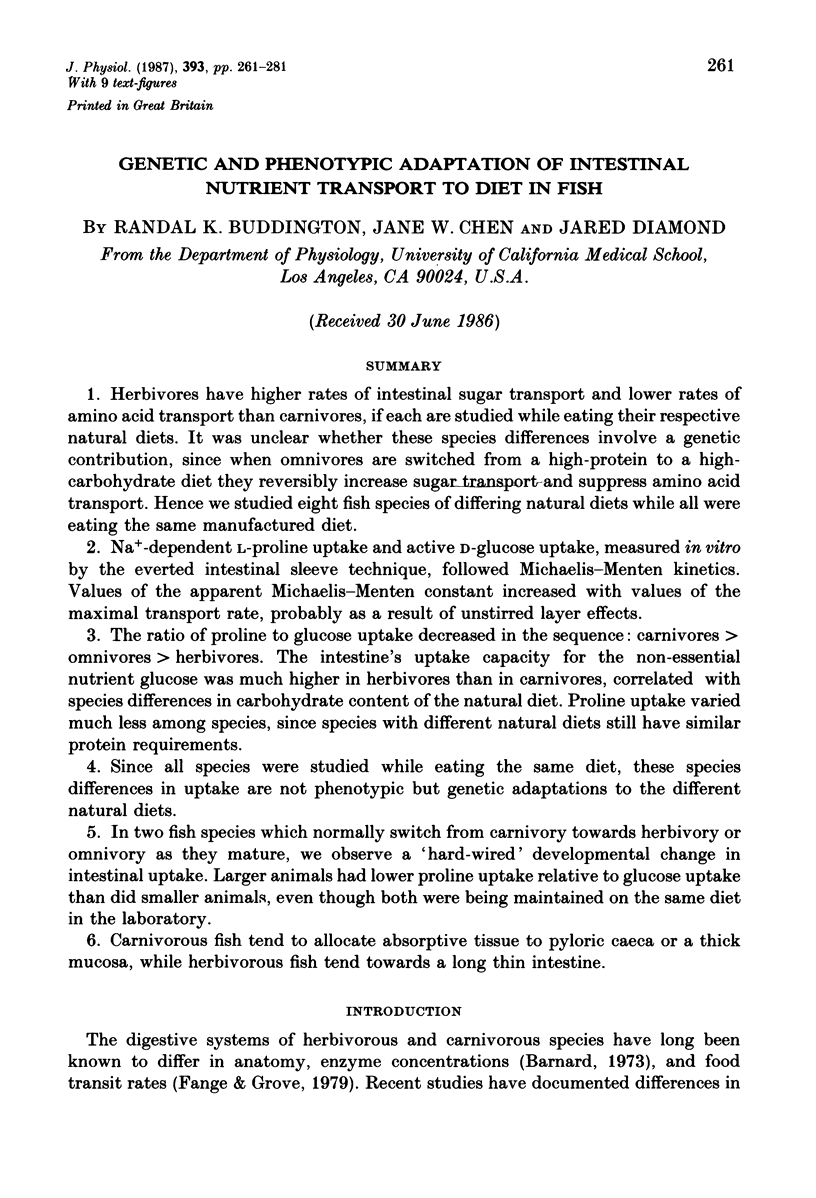
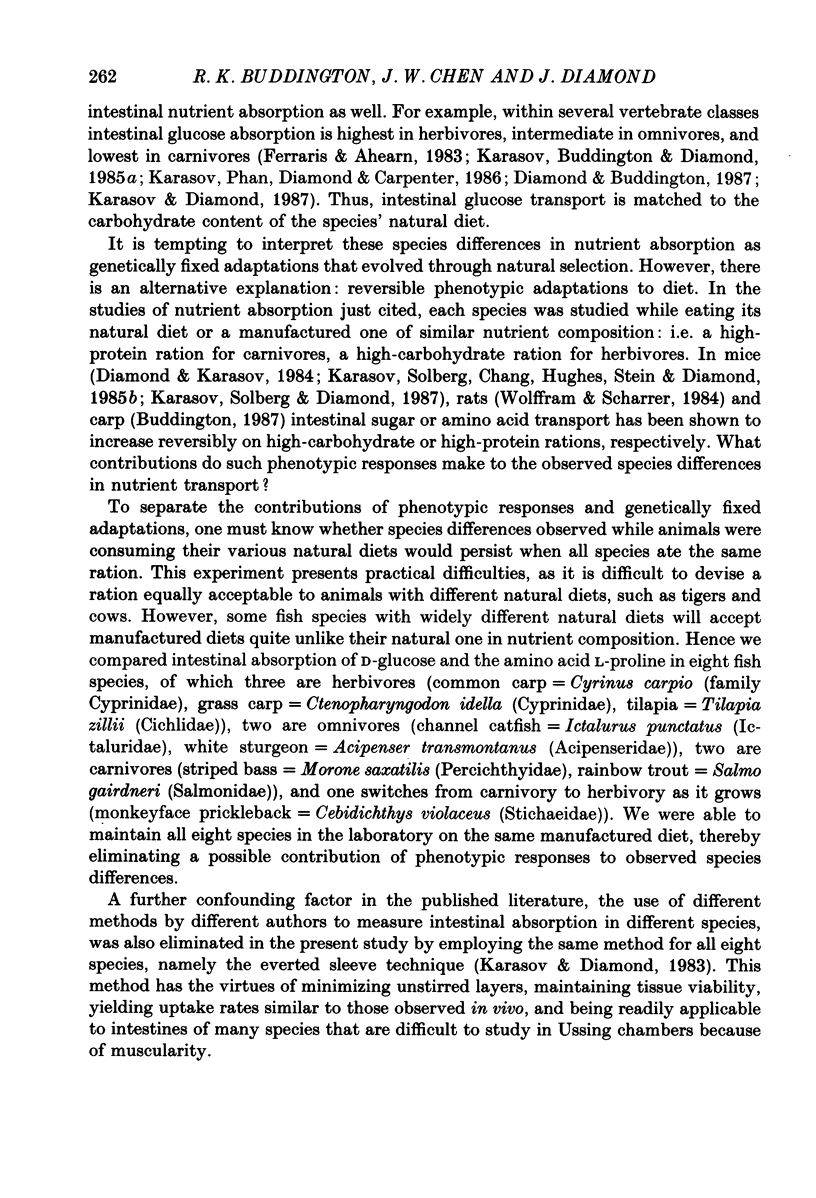
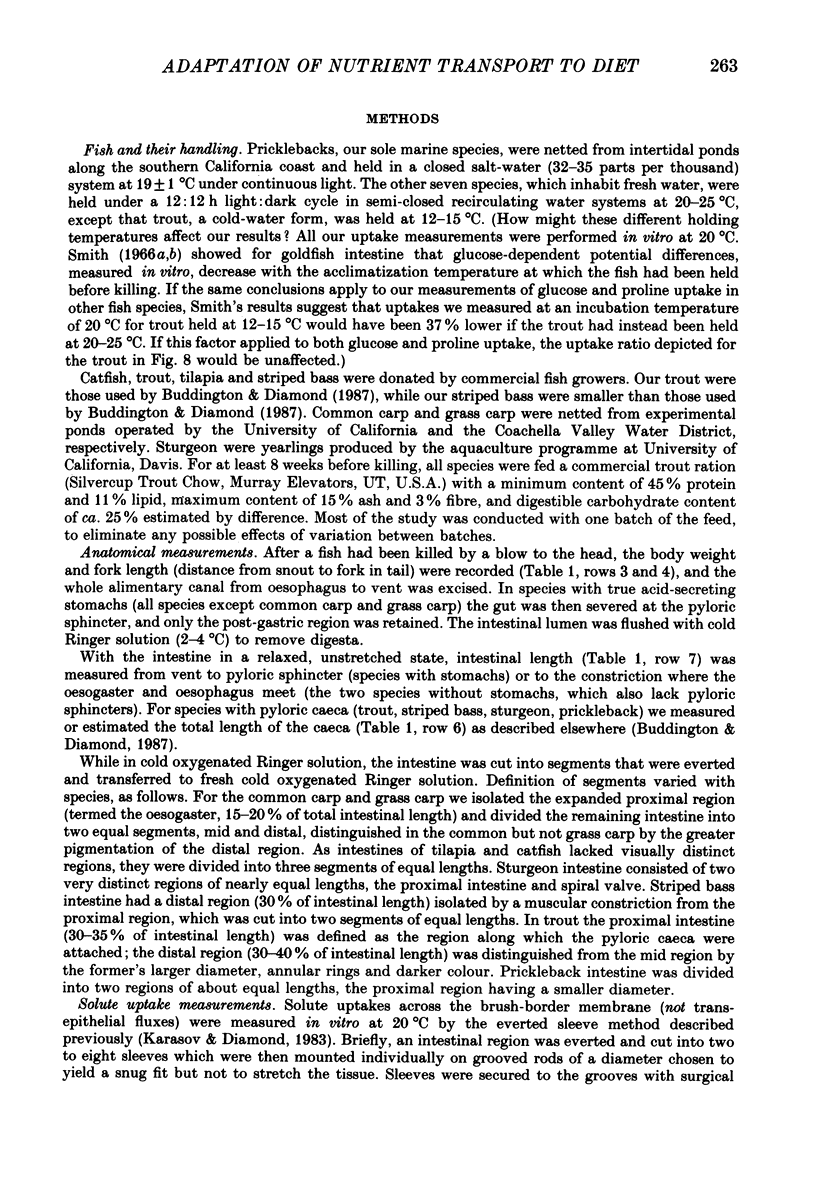
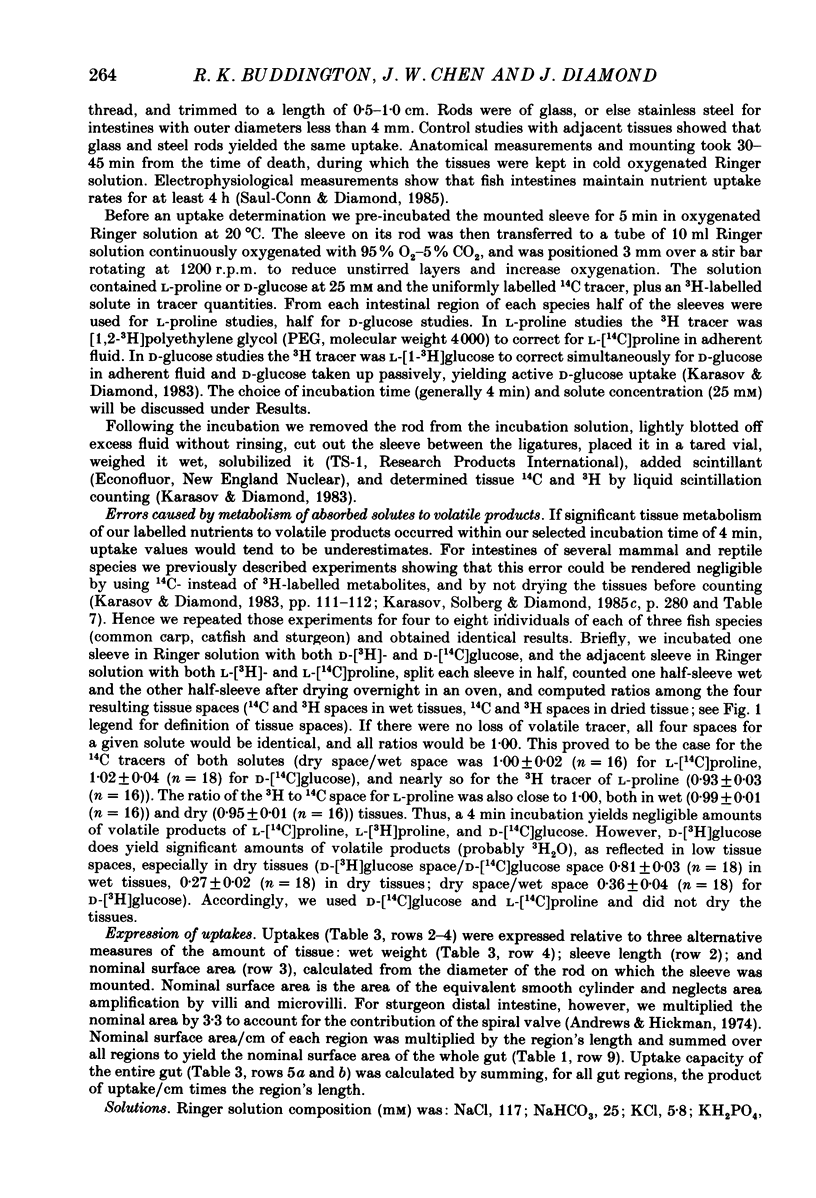
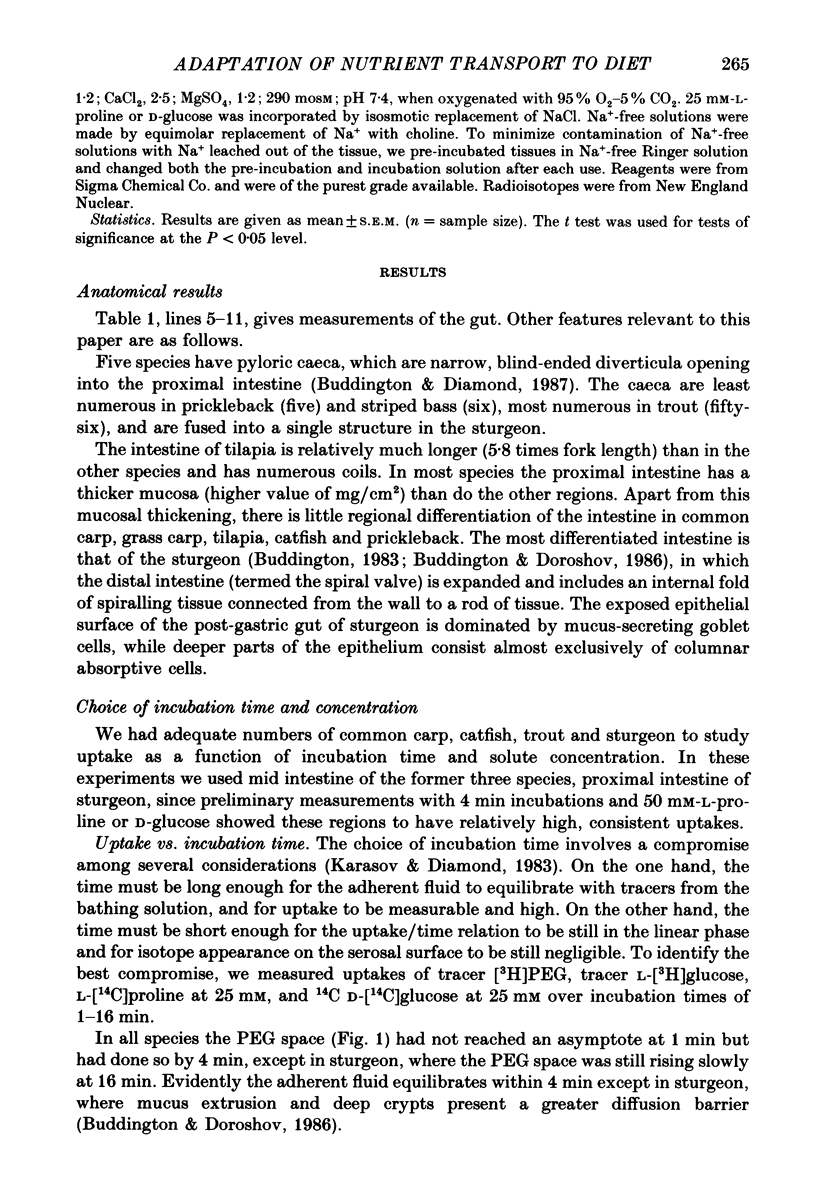
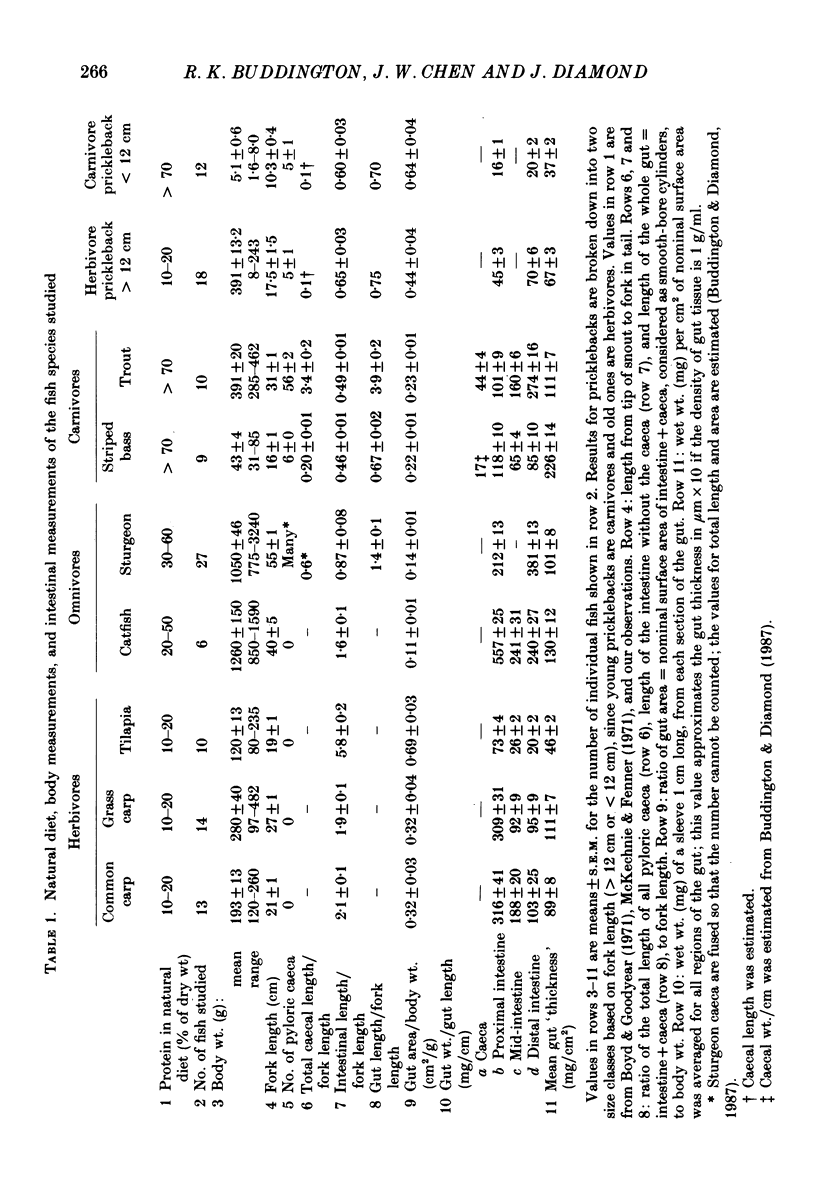
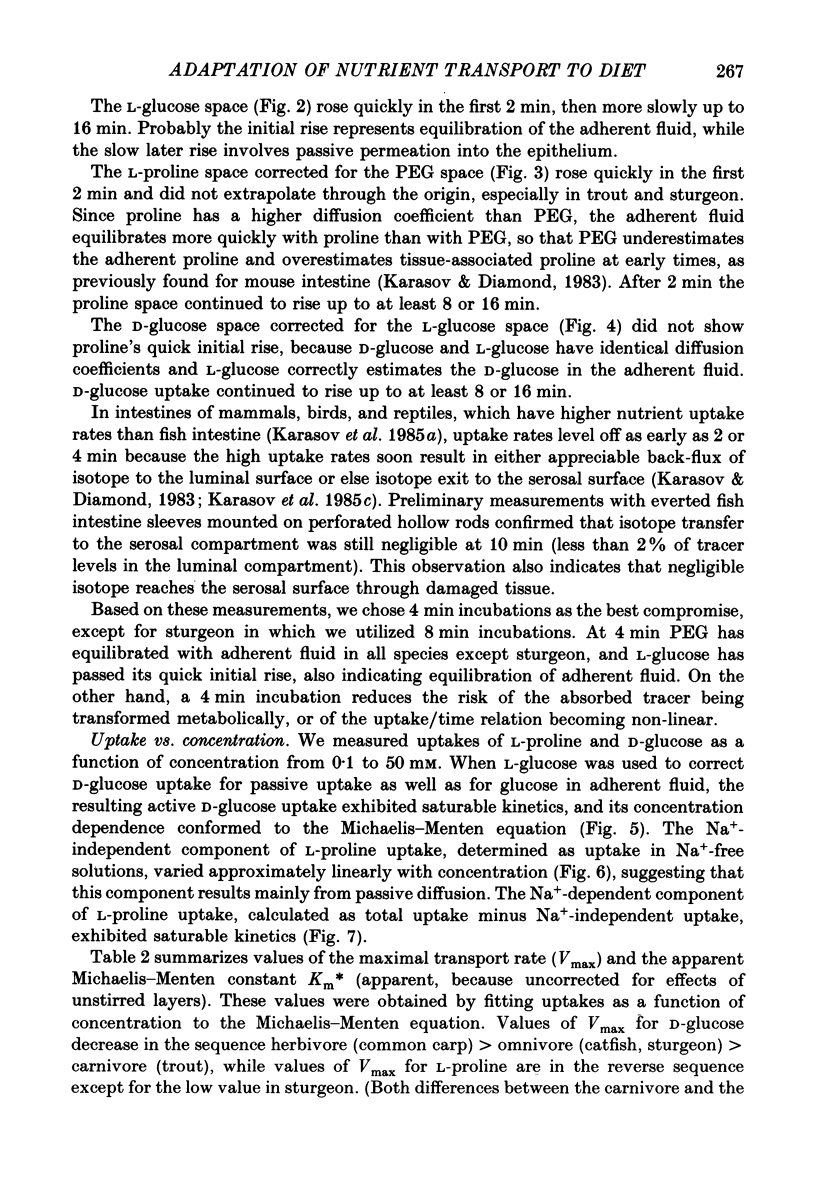
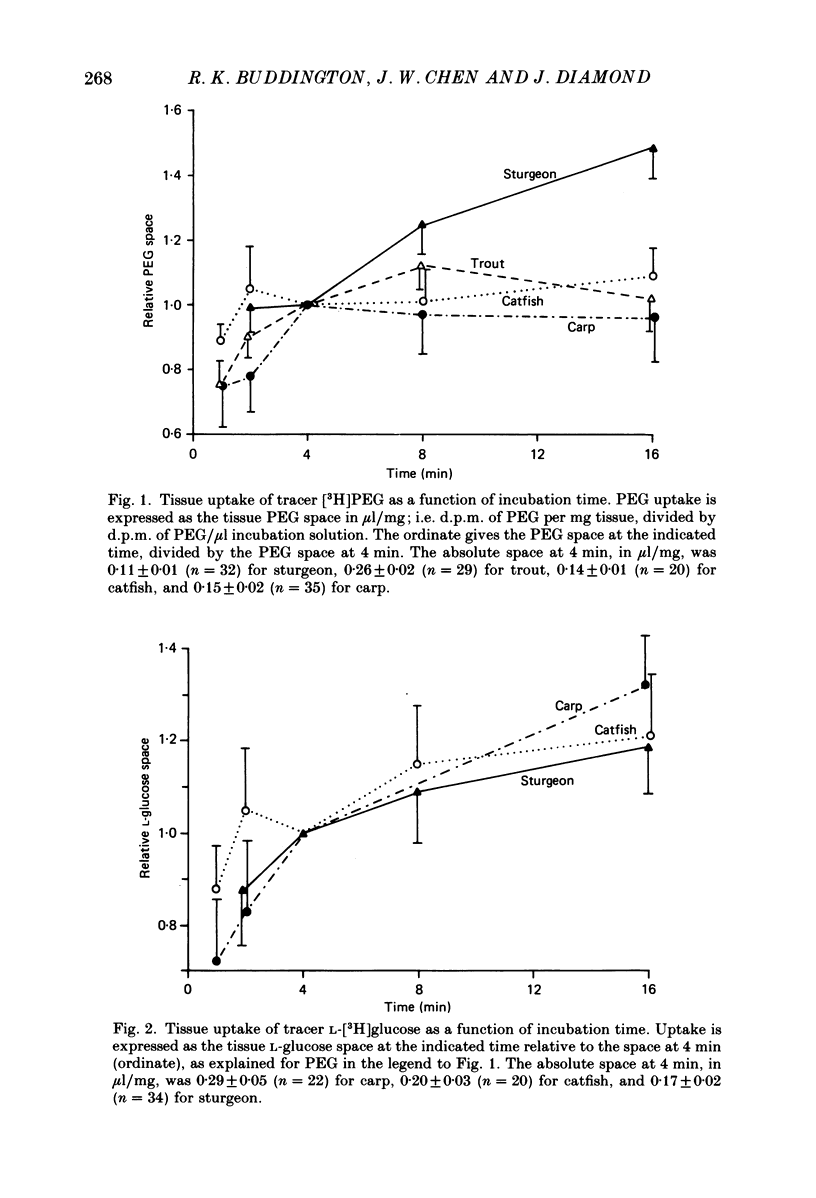
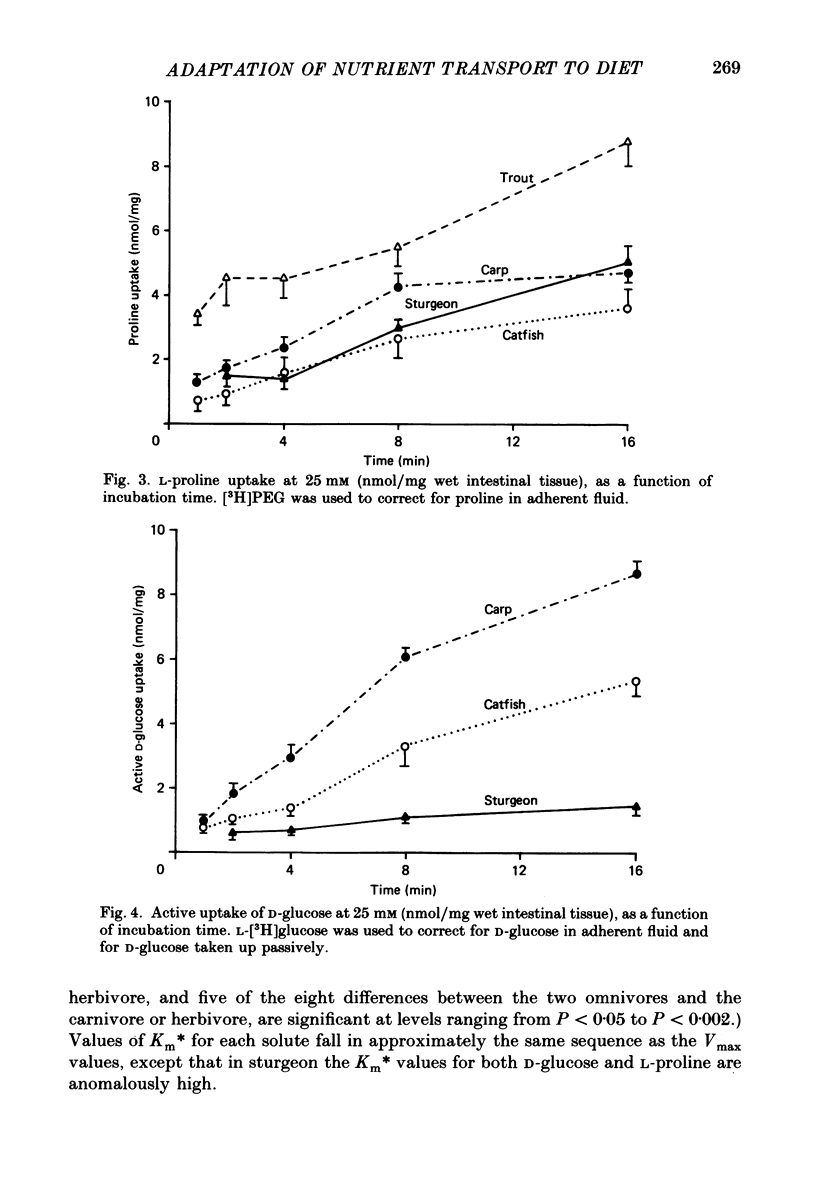
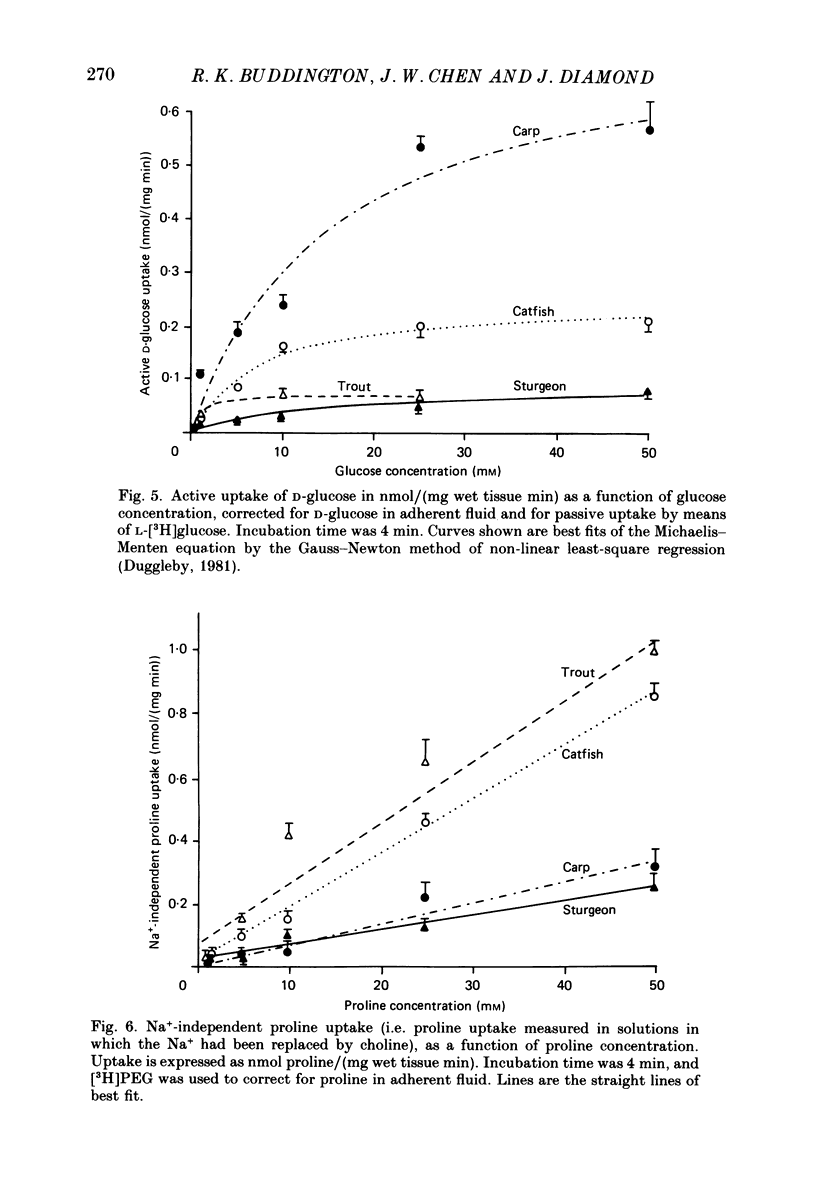
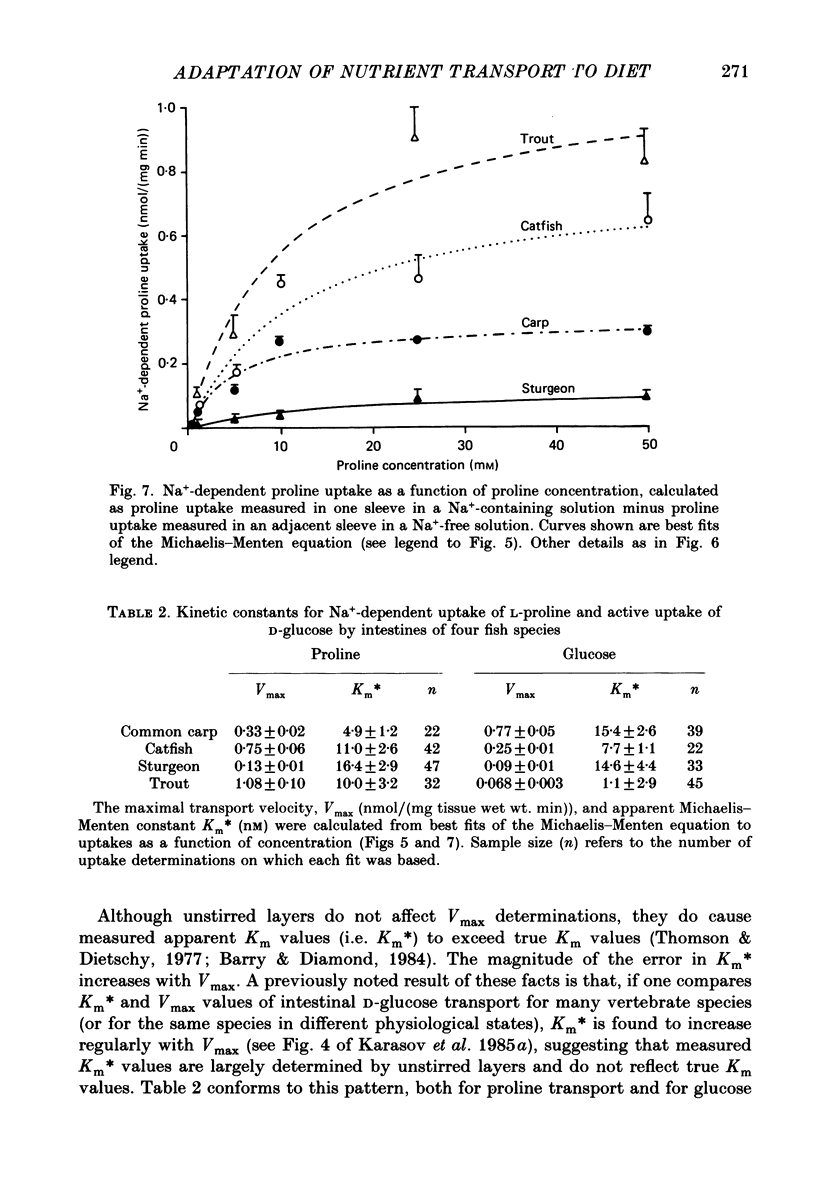
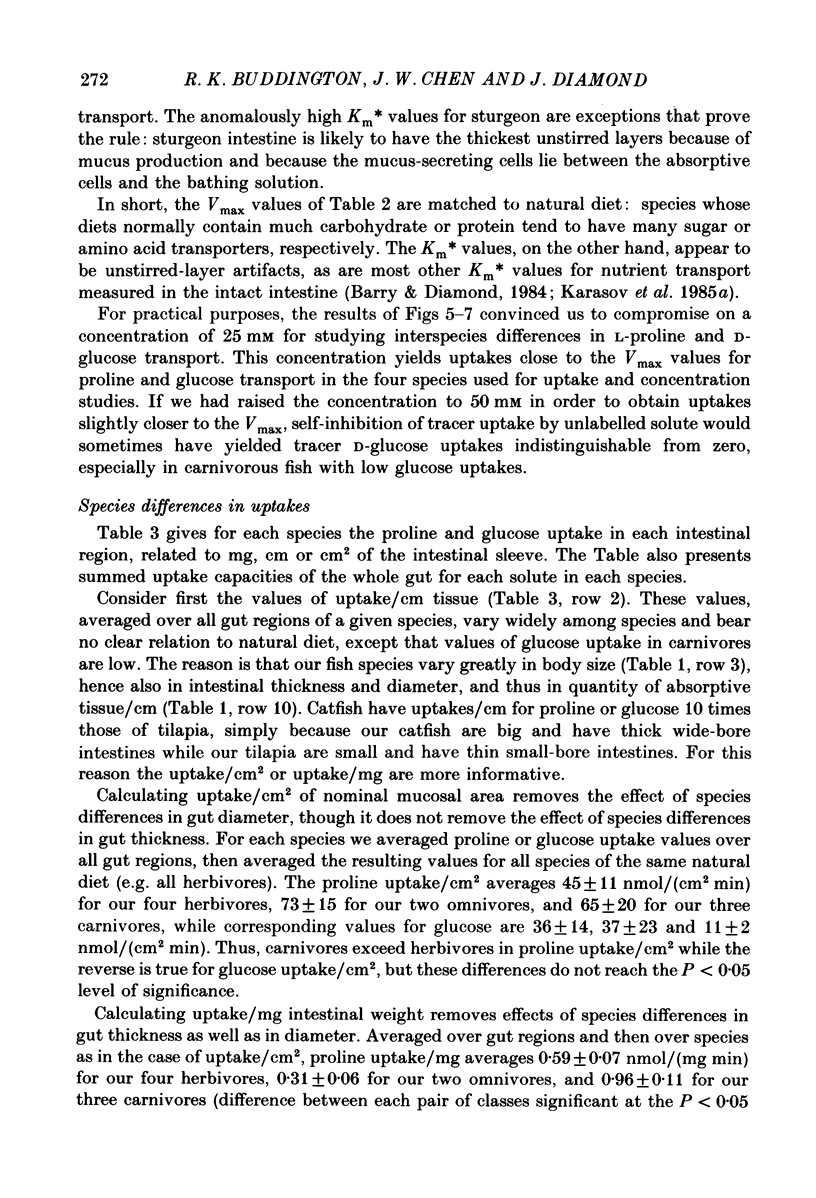
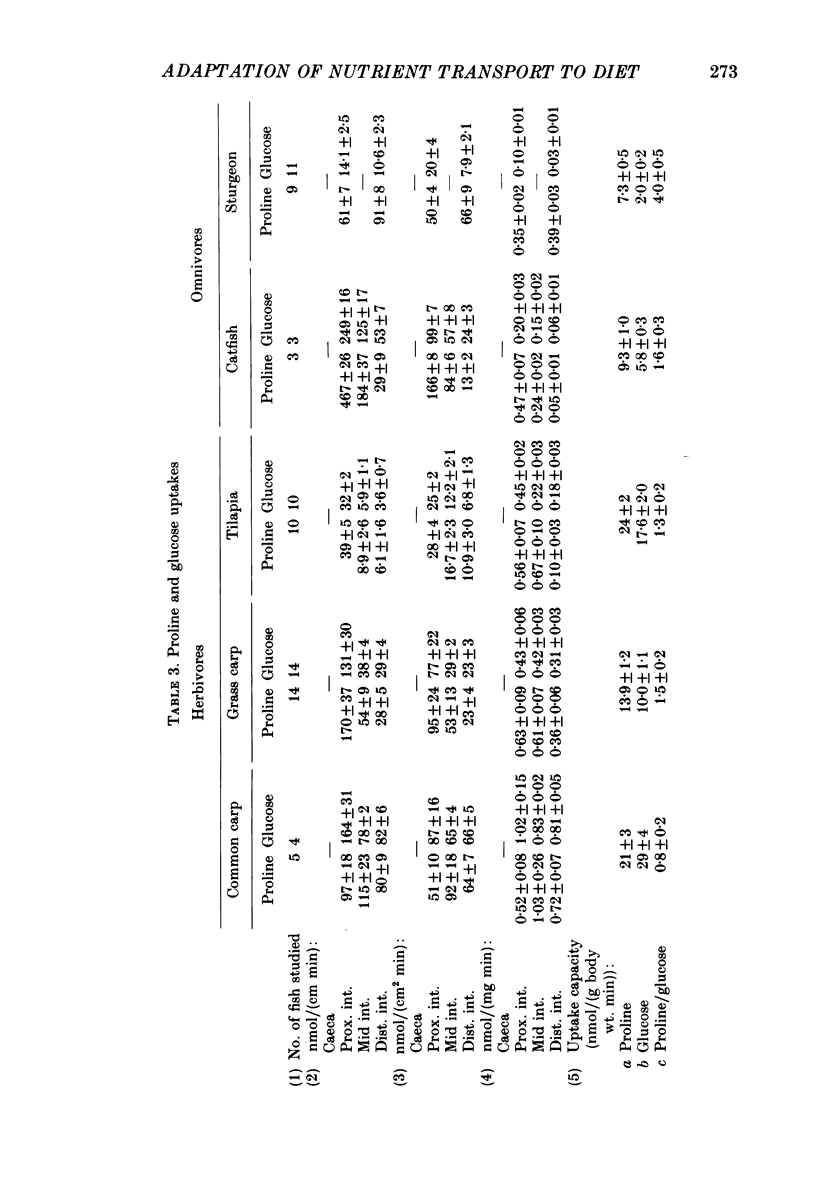
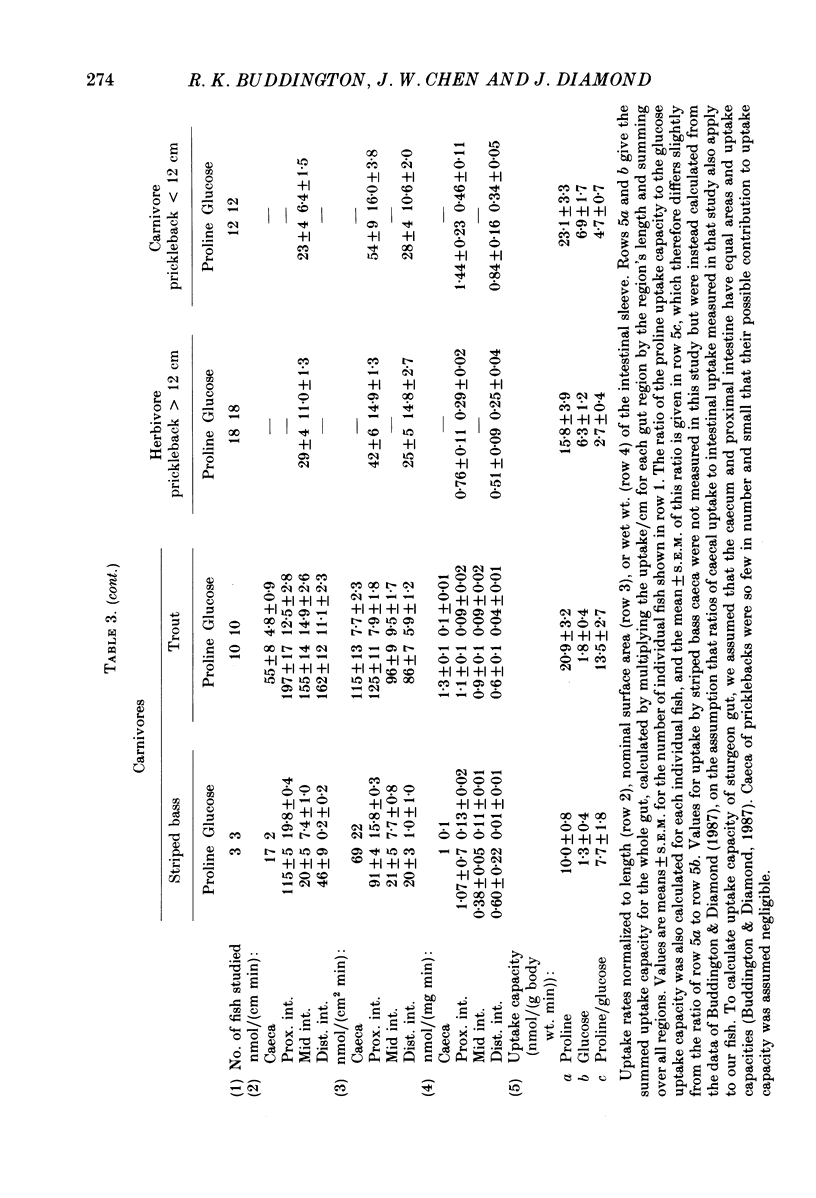
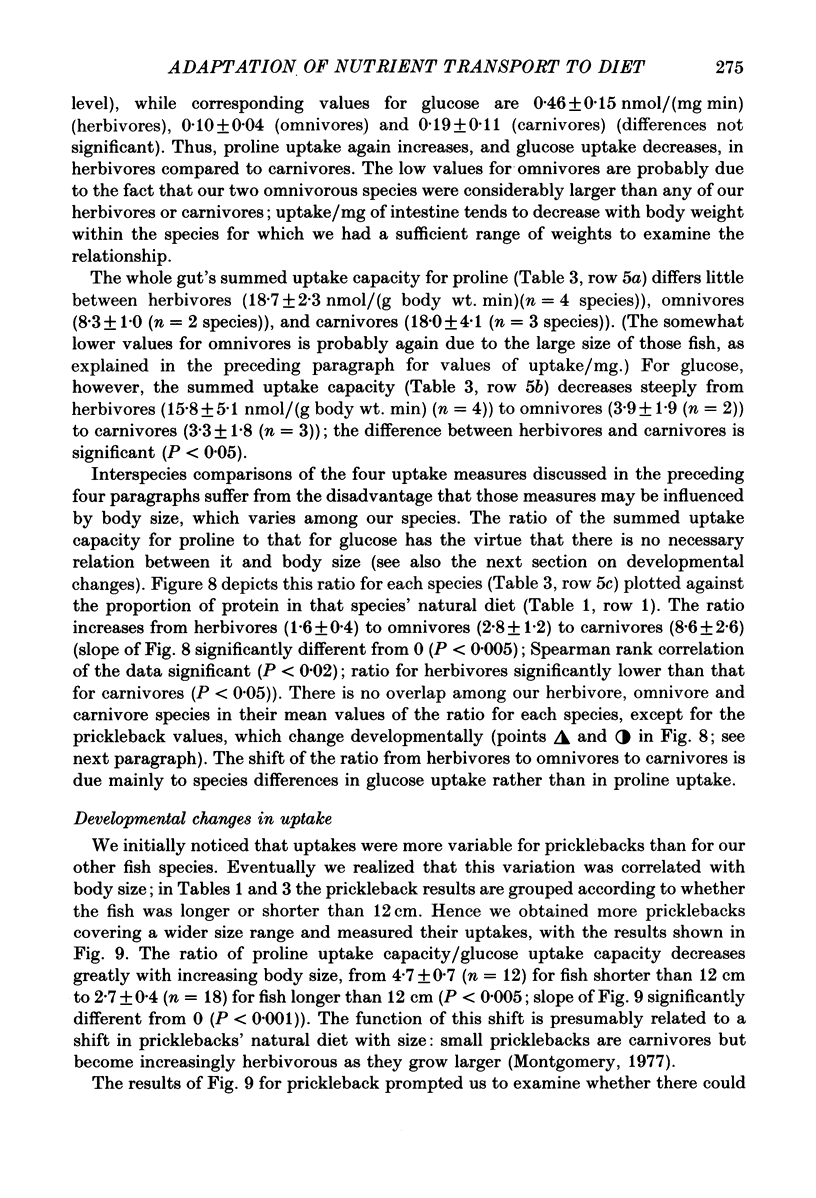
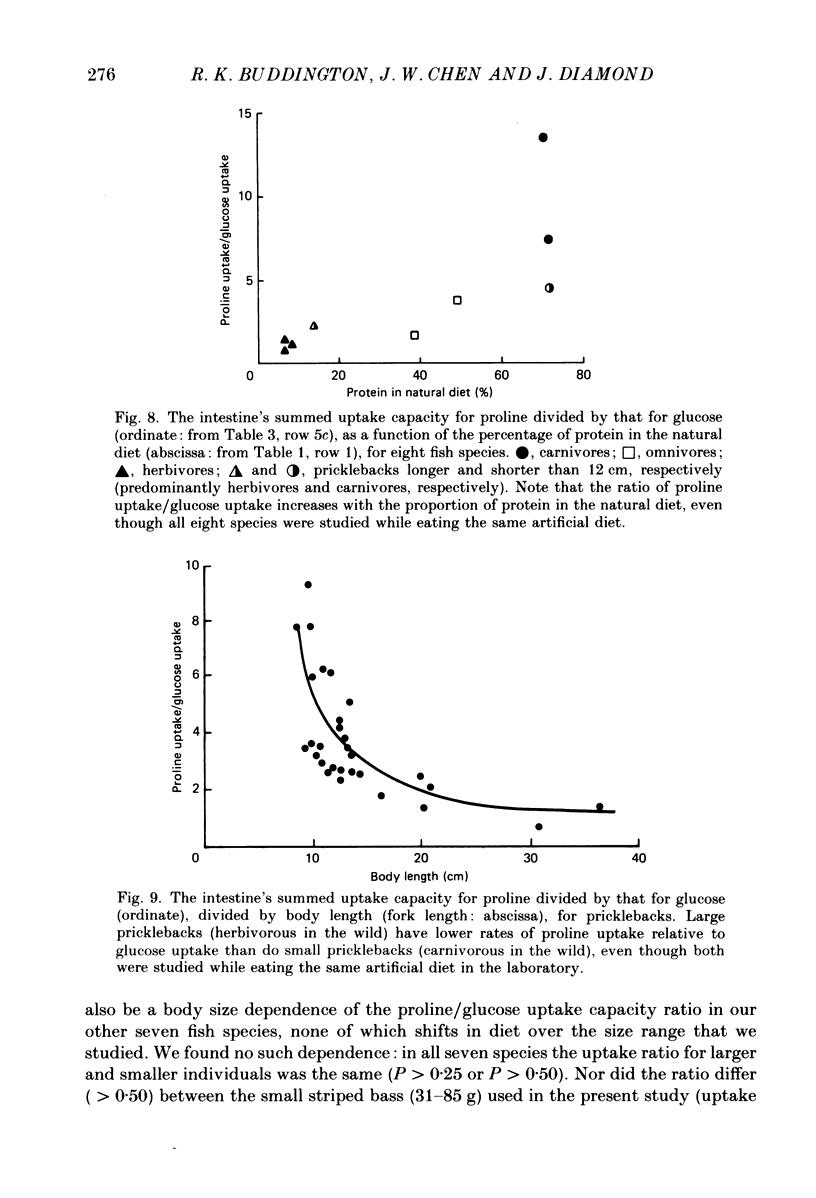
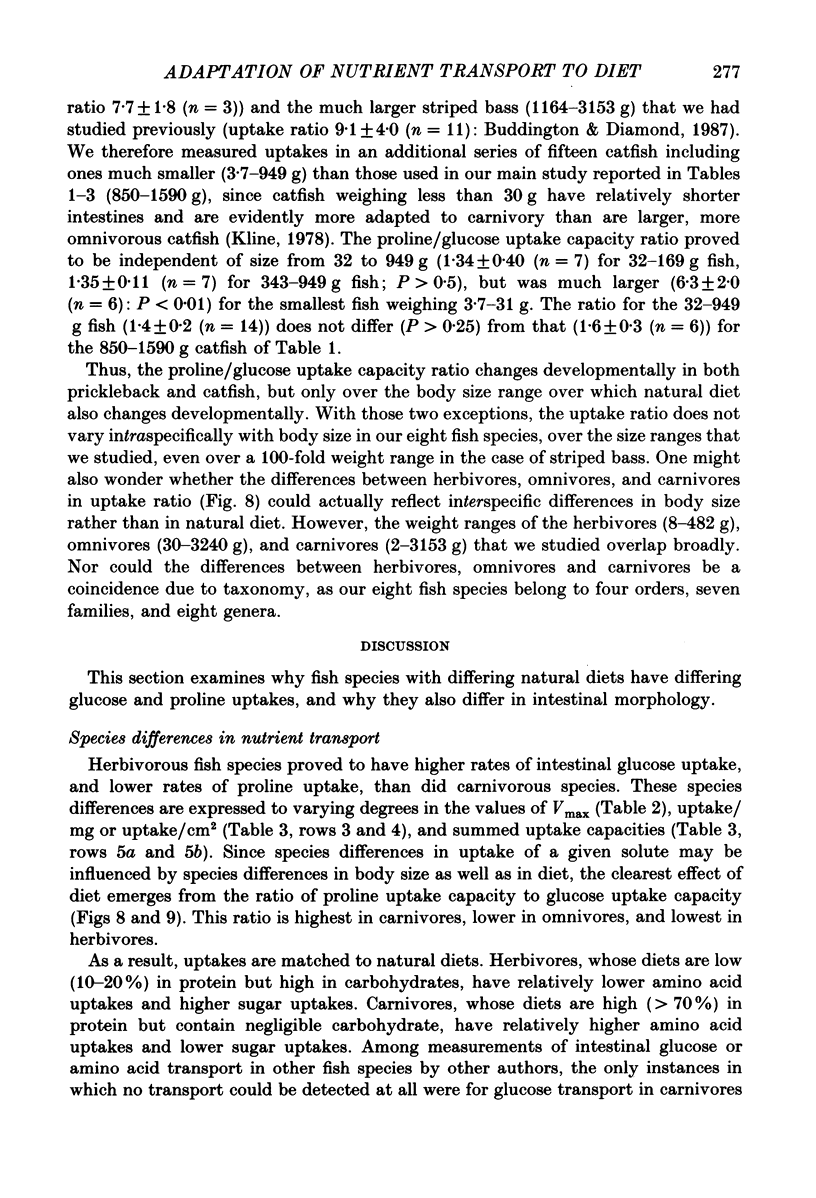
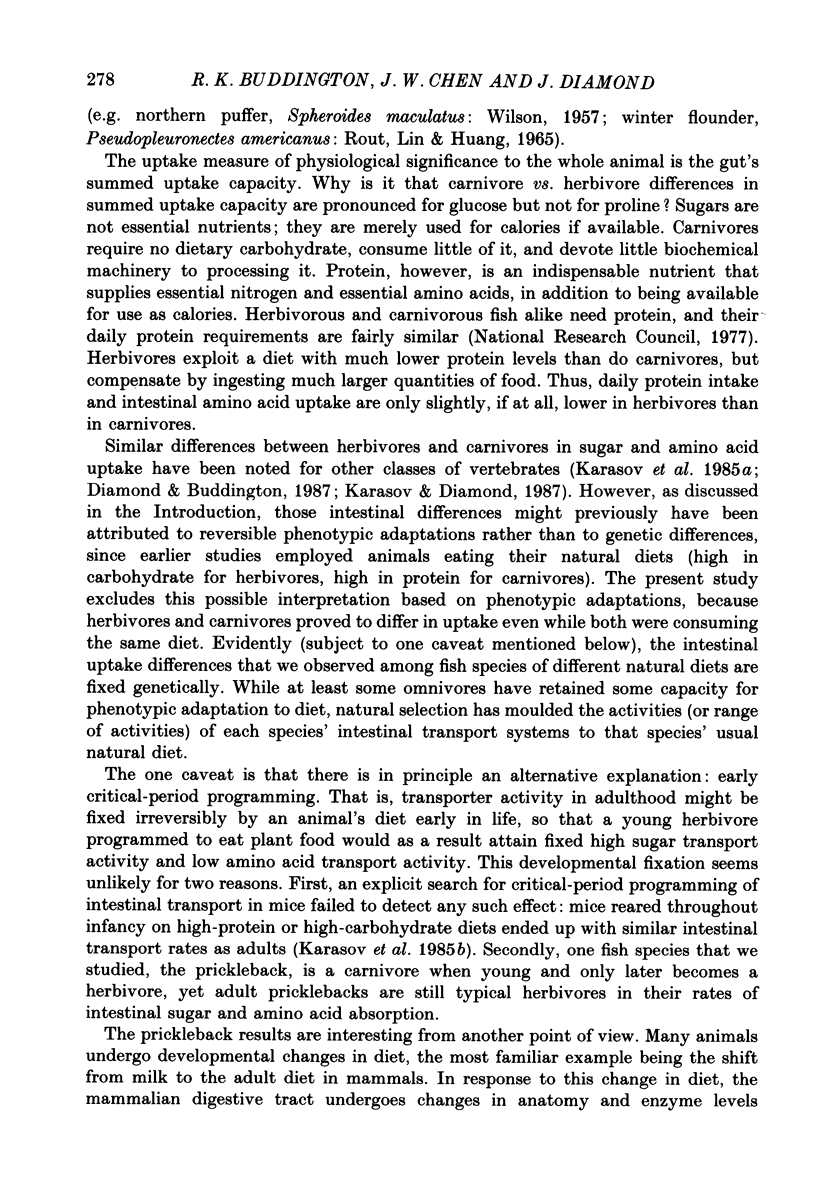
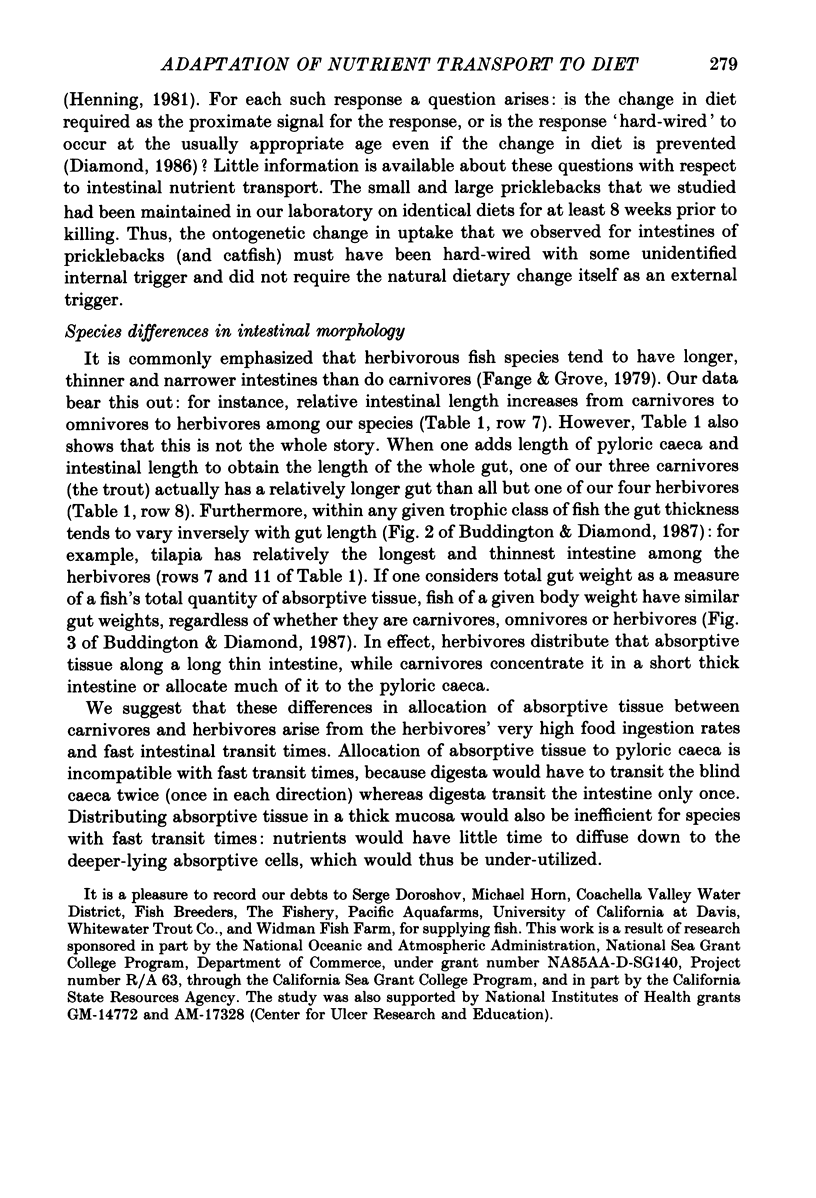
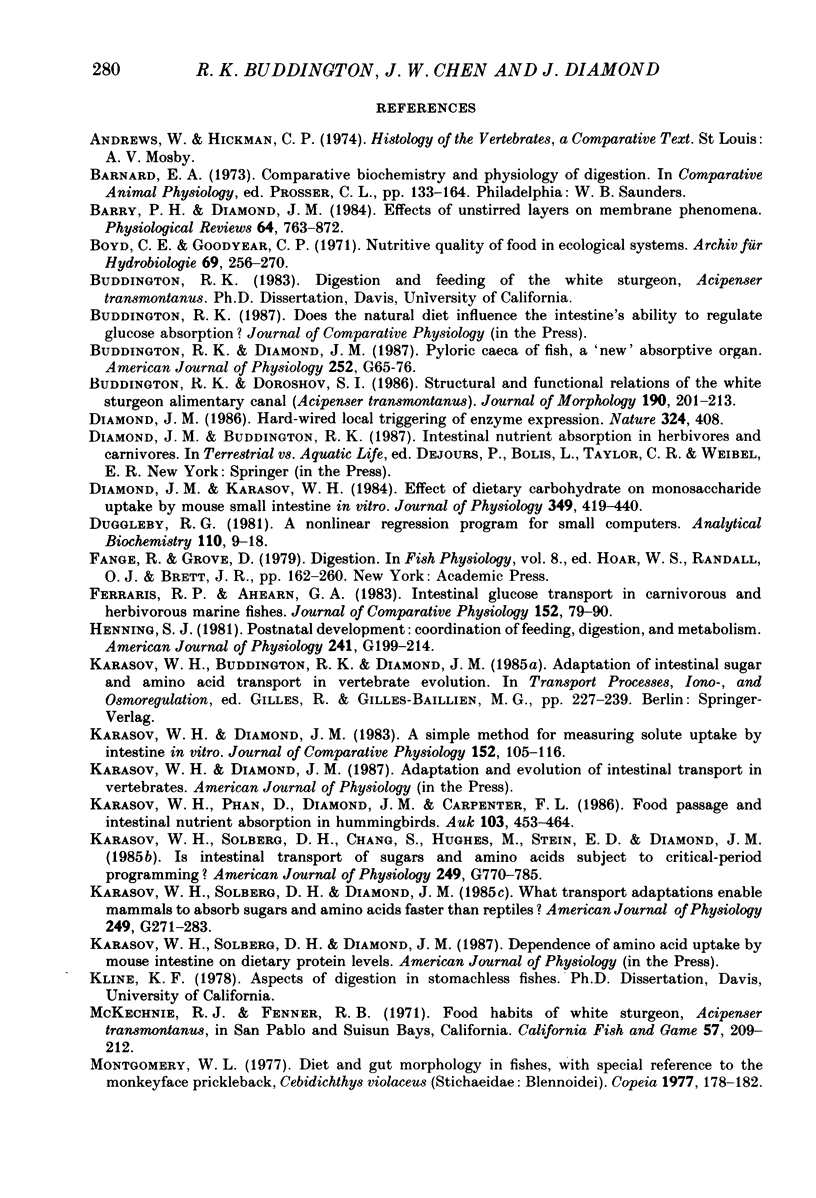
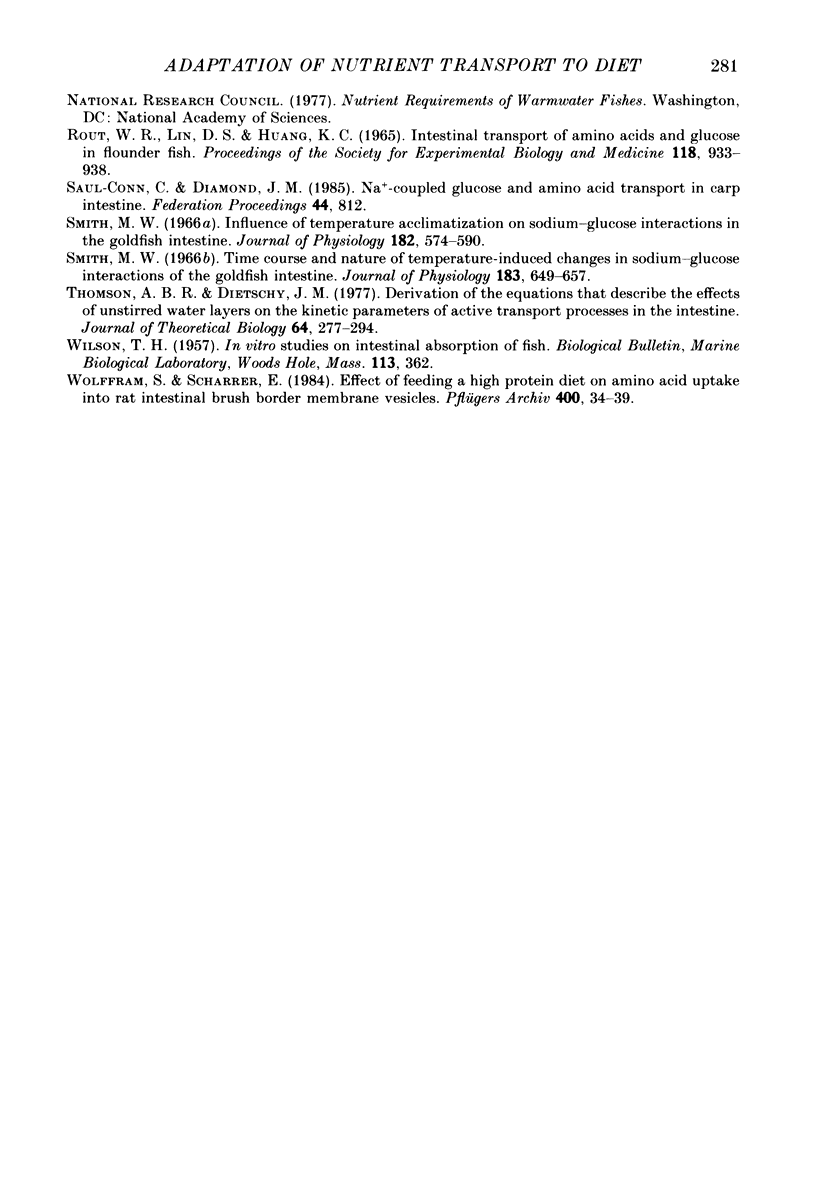
Selected References
These references are in PubMed. This may not be the complete list of references from this article.
- Barry P. H., Diamond J. M. Effects of unstirred layers on membrane phenomena. Physiol Rev. 1984 Jul;64(3):763–872. doi: 10.1152/physrev.1984.64.3.763. [DOI] [PubMed] [Google Scholar]
- Diamond J. M. Developmental physiology. Hard-wired local triggering of intestinal enzyme expression. Nature. 1986 Dec 4;324(6096):408–408. doi: 10.1038/324408a0. [DOI] [PubMed] [Google Scholar]
- Diamond J. M., Karasov W. H., Cary C., Enders D., Yung R. Effect of dietary carbohydrate on monosaccharide uptake by mouse small intestine in vitro. J Physiol. 1984 Apr;349:419–440. doi: 10.1113/jphysiol.1984.sp015165. [DOI] [PMC free article] [PubMed] [Google Scholar]
- Duggleby R. G. A nonlinear regression program for small computers. Anal Biochem. 1981 Jan 1;110(1):9–18. doi: 10.1016/0003-2697(81)90104-4. [DOI] [PubMed] [Google Scholar]
- Henning S. J. Postnatal development: coordination of feeding, digestion, and metabolism. Am J Physiol. 1981 Sep;241(3):G199–G214. doi: 10.1152/ajpgi.1981.241.3.G199. [DOI] [PubMed] [Google Scholar]
- Karasov W. H., Solberg D. H., Chang S. D., Hughes M., Stein E. D., Diamond J. M. Is intestinal transport of sugars and amino acids subject to critical-period programming? Am J Physiol. 1985 Dec;249(6 Pt 1):G770–G785. doi: 10.1152/ajpgi.1985.249.6.G770. [DOI] [PubMed] [Google Scholar]
- Karasov W. H., Solberg D. H., Diamond J. M. What transport adaptations enable mammals to absorb sugars and amino acids faster than reptiles? Am J Physiol. 1985 Aug;249(2 Pt 1):G271–G283. doi: 10.1152/ajpgi.1985.249.2.G271. [DOI] [PubMed] [Google Scholar]
- ROUT W. R., LIN D. S., HUANG K. C. INTESTINAL TRANSPORT OF AMINO ACIDS AND GLUCOSE IN FLOUNDER FISH. Proc Soc Exp Biol Med. 1965 Apr;118:933–938. doi: 10.3181/00379727-118-30010. [DOI] [PubMed] [Google Scholar]
- Smith M. W. Influence of temperature acclimatization on sodium--glucose interactions in the goldfish intestine. J Physiol. 1966 Feb;182(3):574–590. doi: 10.1113/jphysiol.1966.sp007838. [DOI] [PMC free article] [PubMed] [Google Scholar]
- Smith M. W. Time course and nature of temperature-induced changes in sodium-glucose interactions of the goldfish intestine. J Physiol. 1966 Apr;183(3):649–657. doi: 10.1113/jphysiol.1966.sp007890. [DOI] [PMC free article] [PubMed] [Google Scholar]
- Thomson A. B., Dietschy J. M. Derivation of the equations that describe the effects of unstirred water layers on the kinetic parameters of active transport processes in the intestine. J Theor Biol. 1977 Jan 21;64(2):277–294. doi: 10.1016/0022-5193(77)90357-5. [DOI] [PubMed] [Google Scholar]
- Wolffram S., Scharrer E. Effect of feeding a high protein diet on amino acid uptake into rat intestinal brush border membrane vesicles. Pflugers Arch. 1984 Jan;400(1):34–39. doi: 10.1007/BF00670533. [DOI] [PubMed] [Google Scholar]