Abstract
1. The membrane potential (Em) of cultured chick embryonic heart cells depolarized to -36 mV after inhibition of the Na+-K+ pump by 0.1 mM-ouabain in a [K+]o of 24 mM: this was accompanied by a rise in Na+ content of approximately 65% in 3 min. Lowering [Na+]o to 27 mM then caused a fall in Na+ content, a rise in Ca2+ content and a small hyperpolarization of approximately 5 mV. The fall in Na+ content indicated a movement of Na+ which was in the opposite direction to the Na+ electrochemical gradient (a countergradient movement). 2. In the presence of 10 mM-Cs+ or 1 mM-Ba2+ the hyperpolarization was approximately 10 or approximately 30 mV, respectively. A 30 mV hyperpolarization took Em negative to the reversal potentials for K+, and Cl- as measured by ion-selective micro-electrodes. 3. The decay of the intracellular Na+ activity alpha iNa, in an [Na+]o of 27 mM followed a simple exponential time course (time constant, 36 s). The initial rate depended on the value to which [Na+]o was lowered in a manner suggesting a simple competitive inhibition of the exchange by external Na+. 4. The low-[Na+]o hyperpolarization was unaffected by amiloride (0.1 or 1 mM) or verapamil (20 microM). Both La3+ (1 mM) and Mn2+ (20 mM) blocked the hyperpolarization sufficiently to prevent Em hyperpolarizing negative to the reversal potentials for K+, Na+ and Cl-. 5. Re-establishing [Na+]o caused a rise in Na+ content and a countergradient drop in Ca2+ content. The effects of verapamil (20 microM), amiloride (0.1 and 1 mM), dichlorobenzamil (0.1 mM), quinidine (1 mM), Mn2+ (20 mM) and La3+ (1 mM) were tested on the movements of Na+ and Ca2+ both during exposure to an [Na+]o of 27 mM and on re-establishing [Na+]o. The only consistent and substantial effects were the attenuation by La3+ and Mn2+ and Ca2+ movements during exposure to an [Na+]o of 27 mM. However, neither La3+ nor Mn2+ affected the movements of Na+ and Ca2+ on re-establishing [Na+]o. 6. We conclude that cultured embryonic chick heart cells contain a Na+-Ca2+ exchange evidenced by the ability to cause movements of Na+ and Ca2+ which are counter to their respective electrochemical gradient and which are accompanied by downhill movements of the counter ion.(ABSTRACT TRUNCATED AT 400 WORDS)
Full text
PDF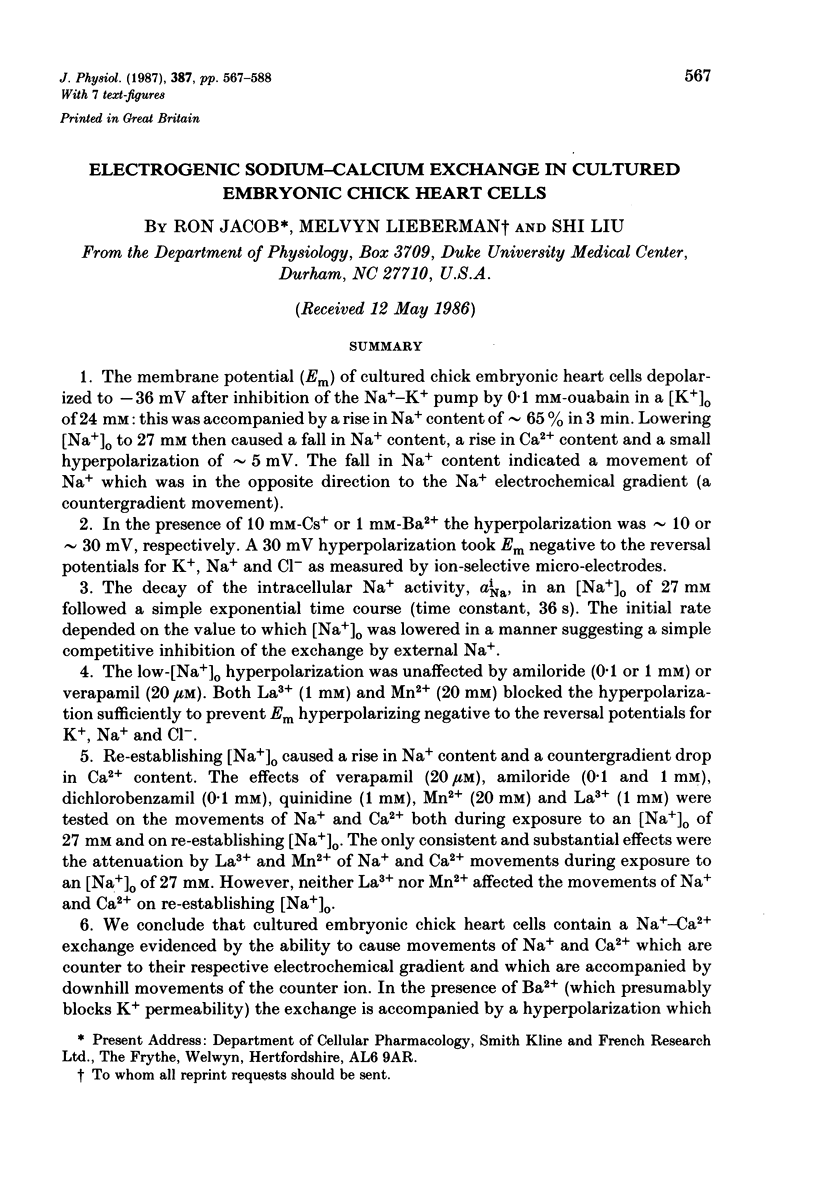
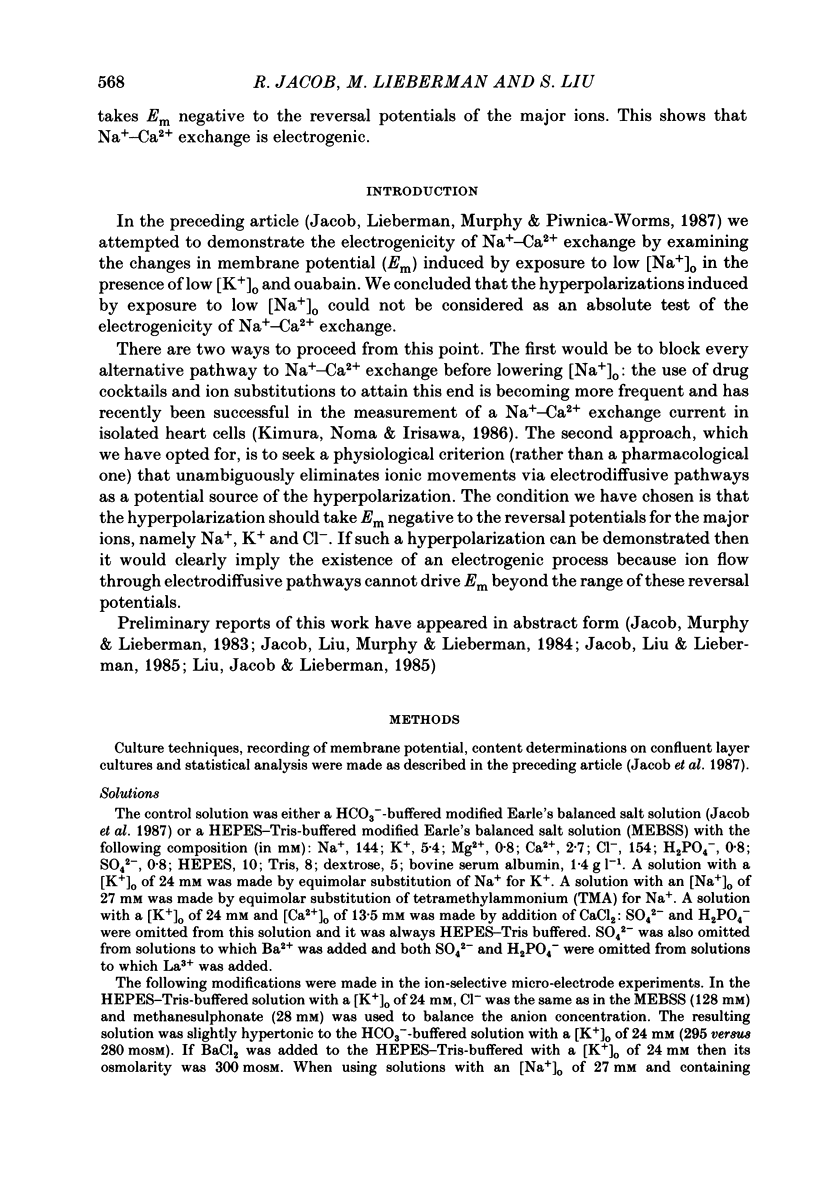
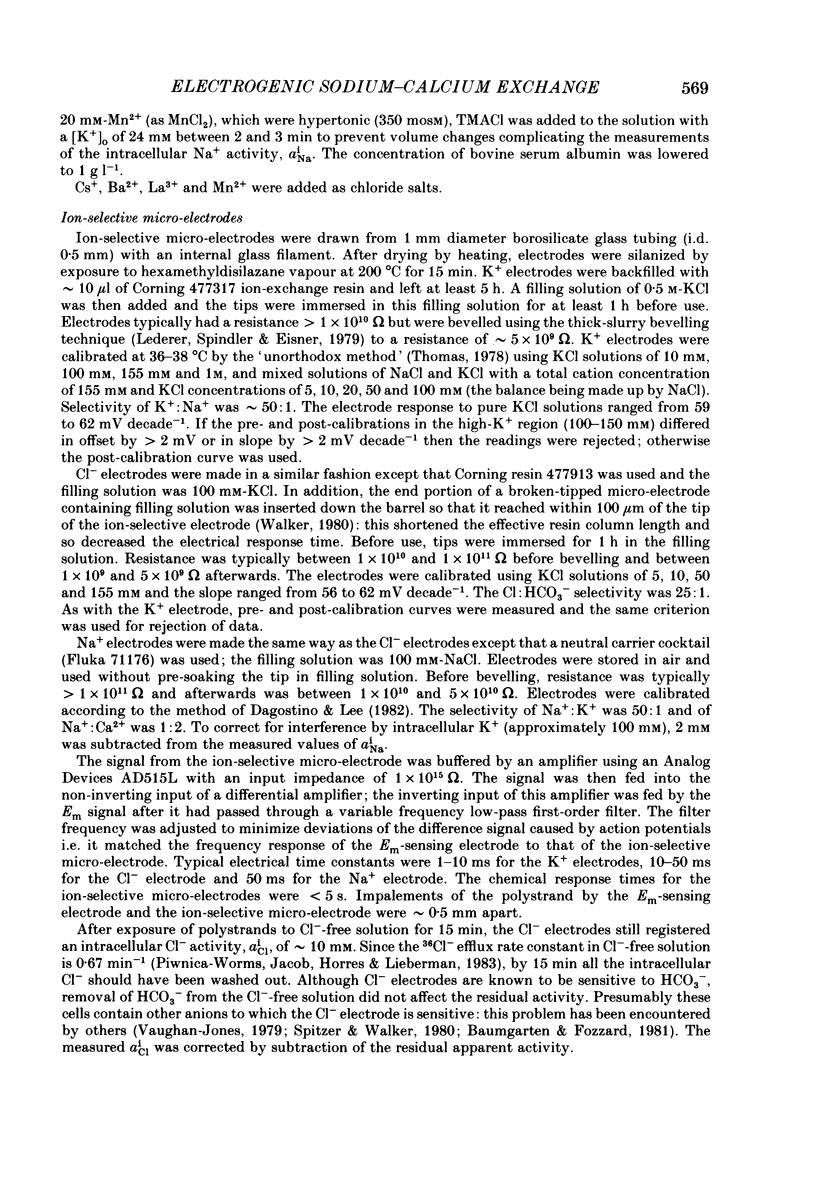
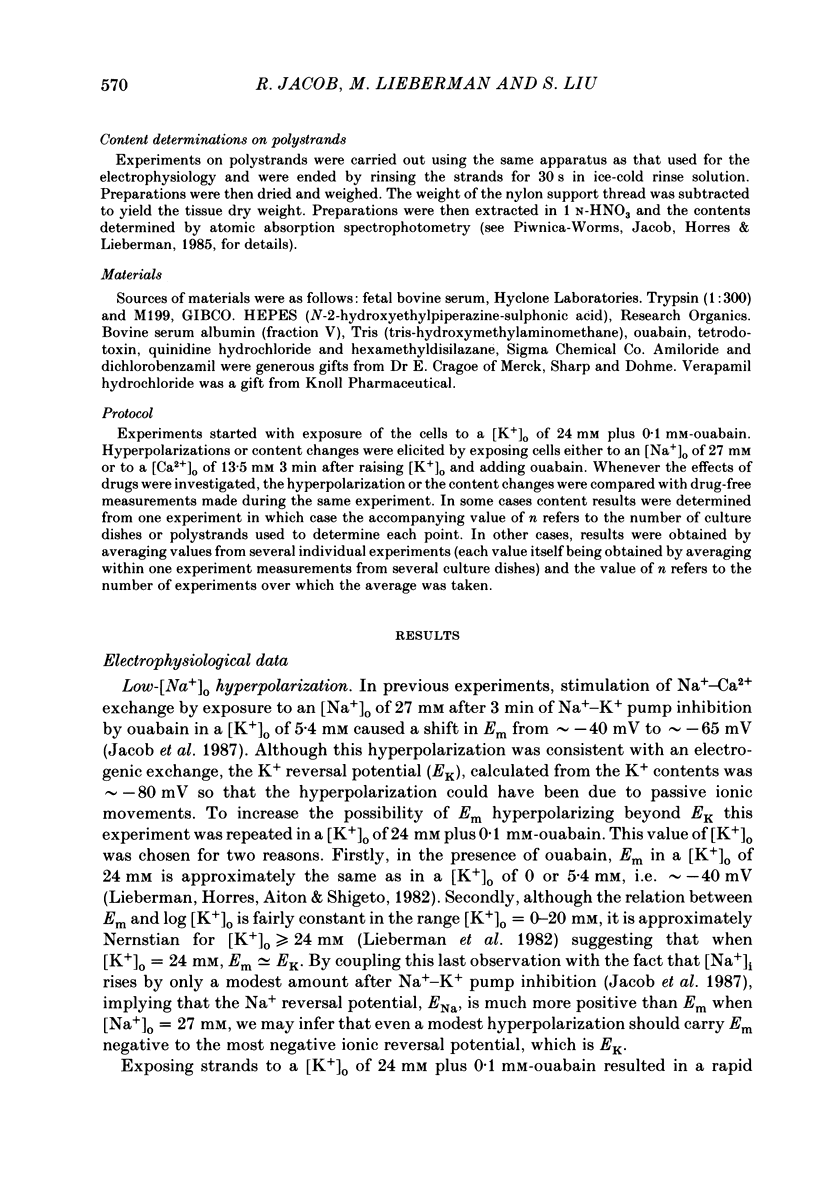
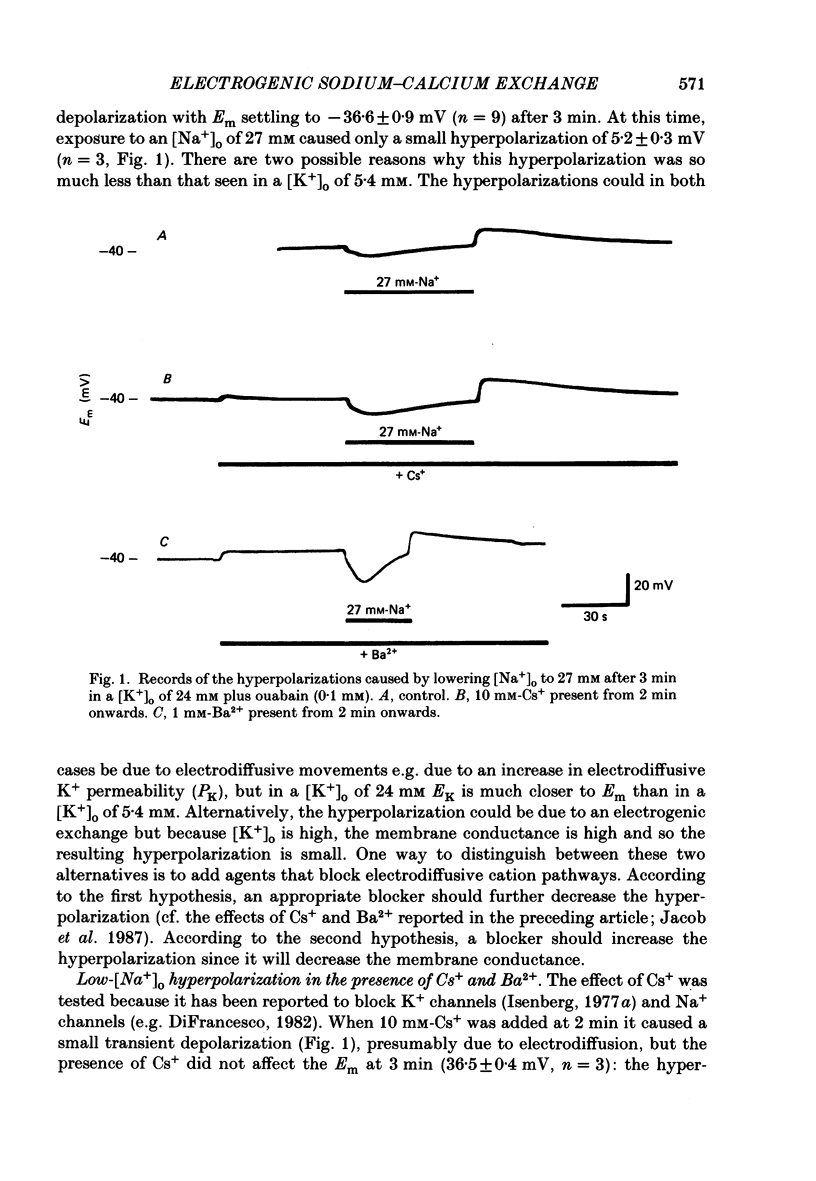
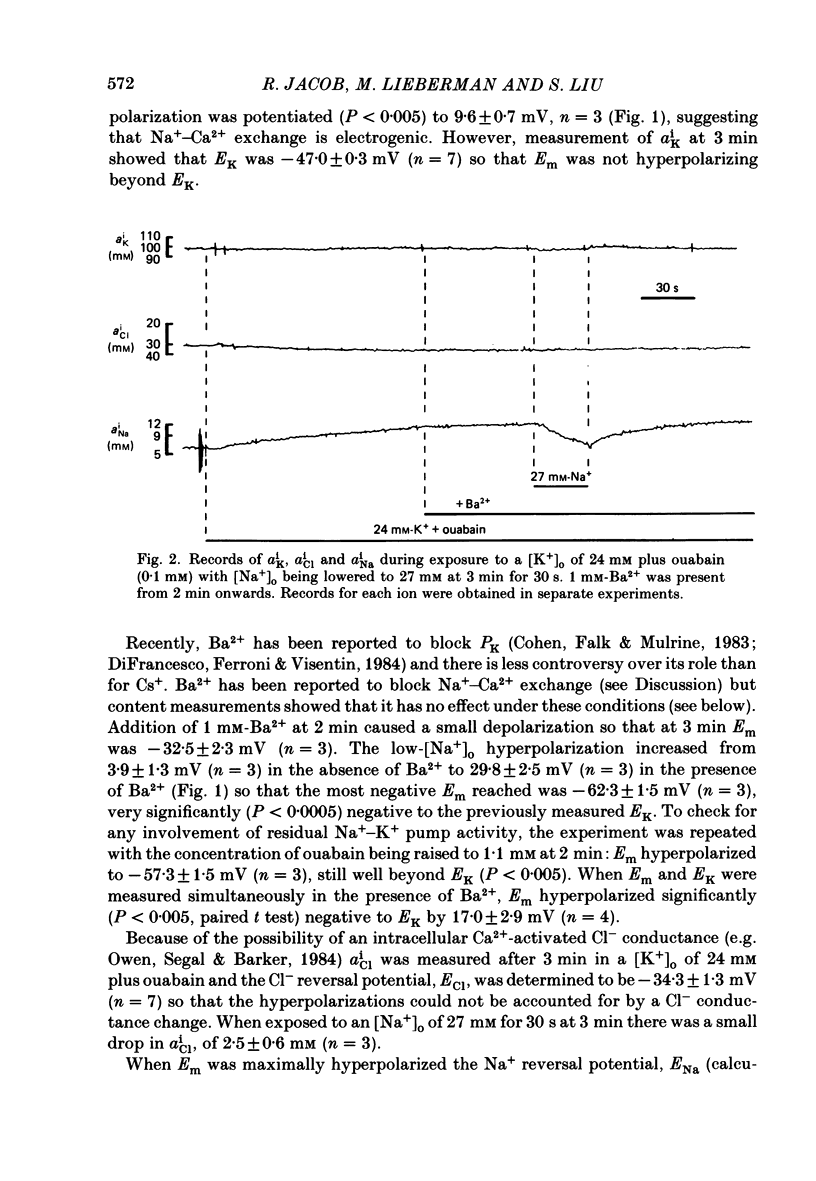
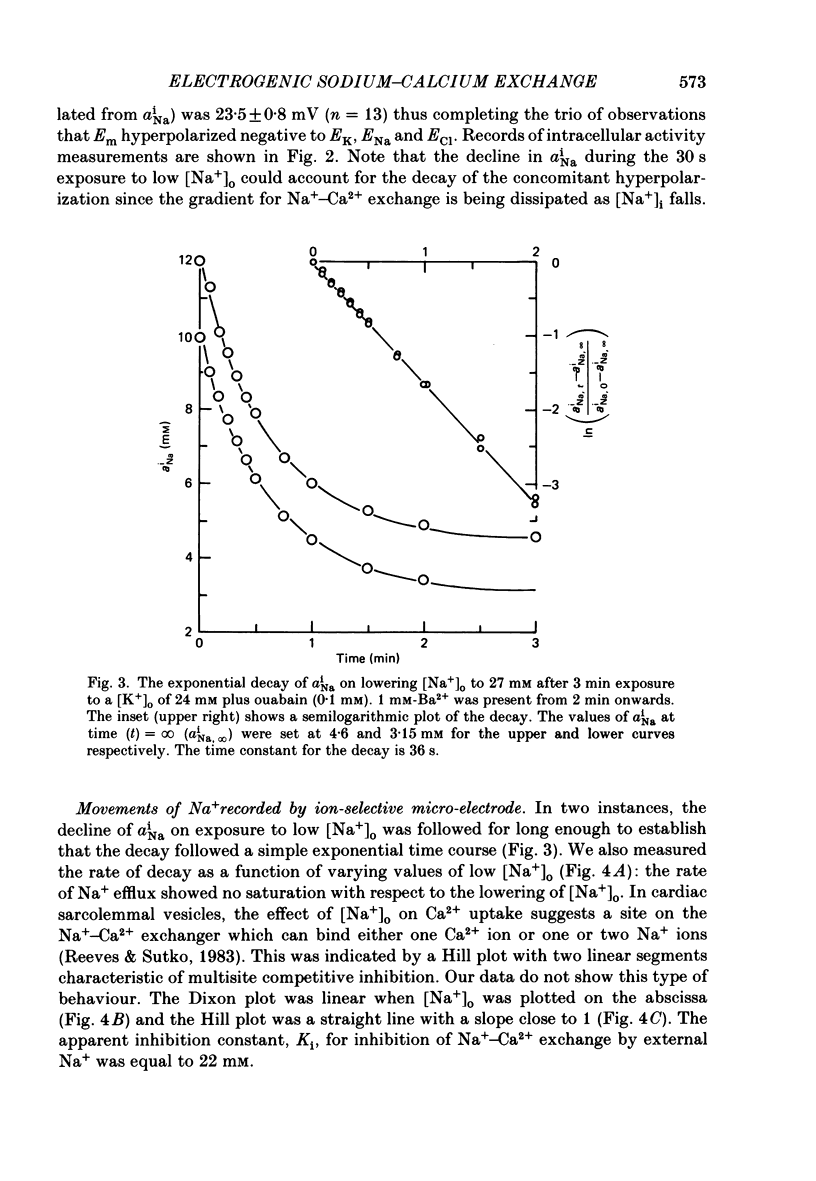
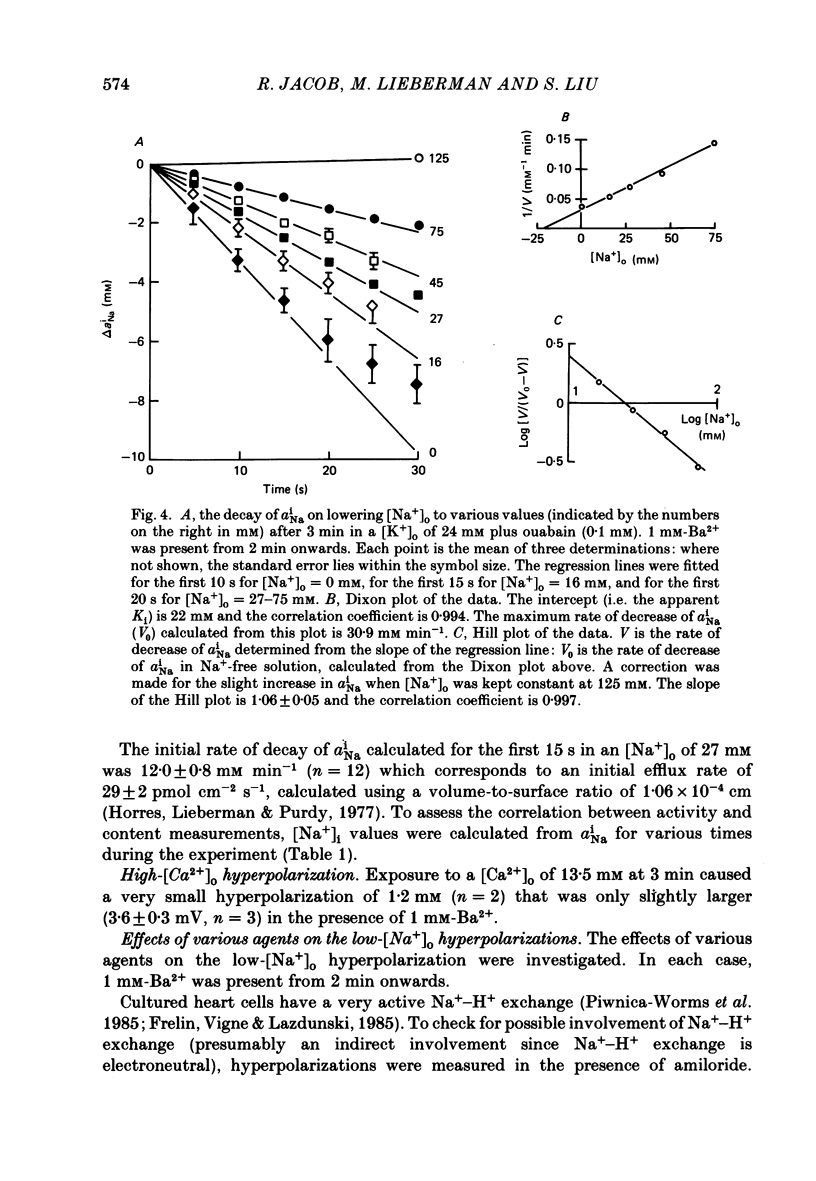
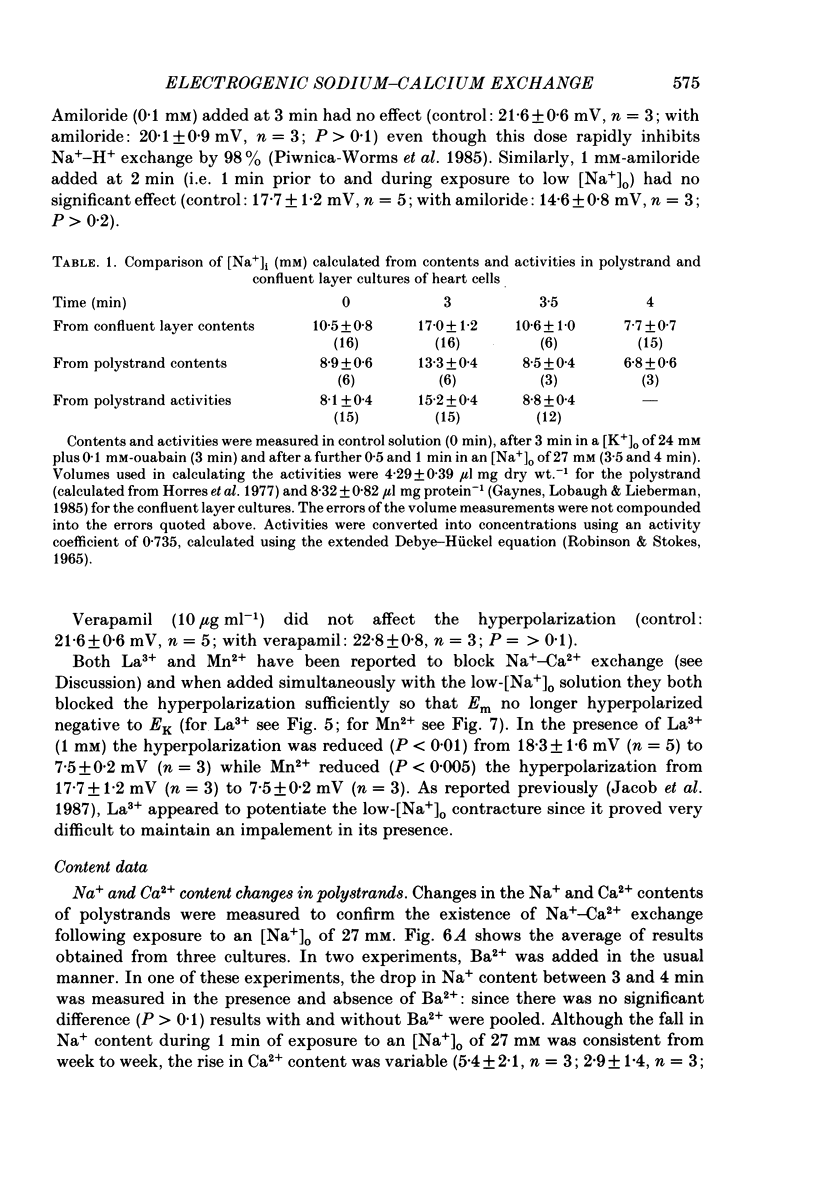
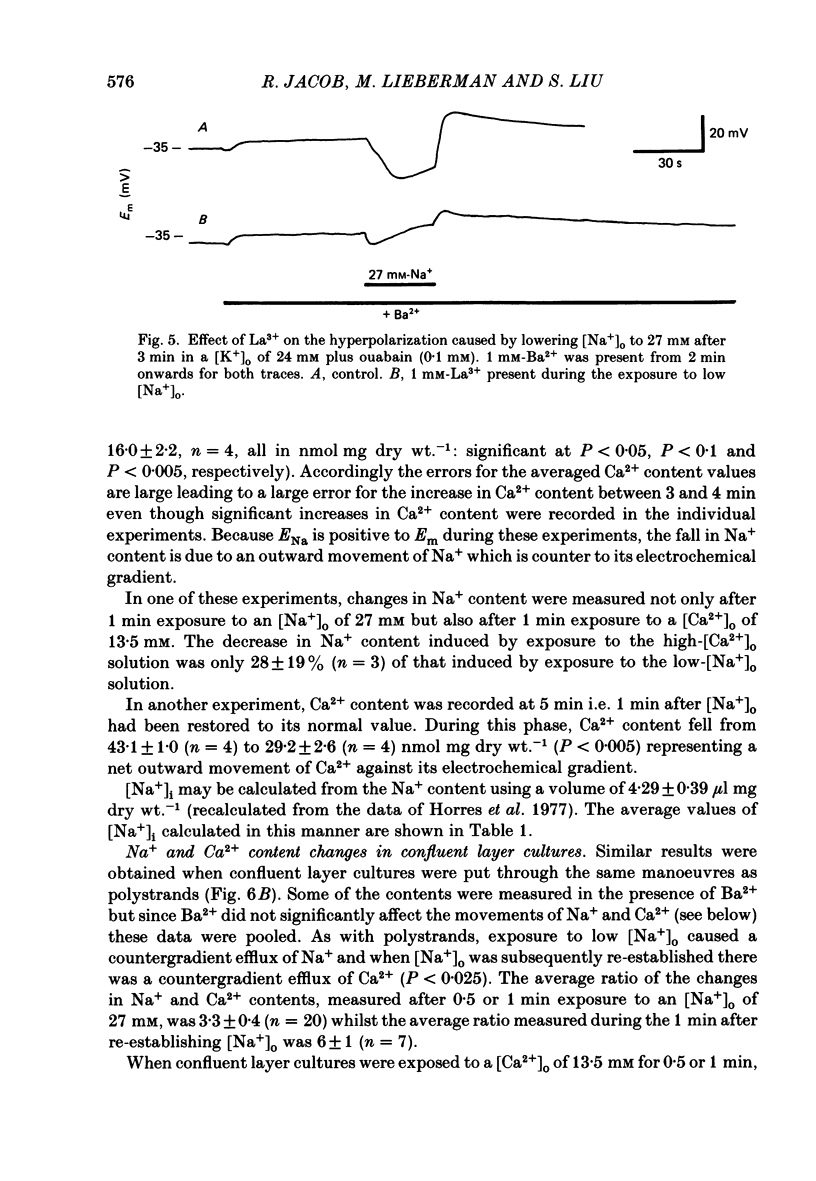
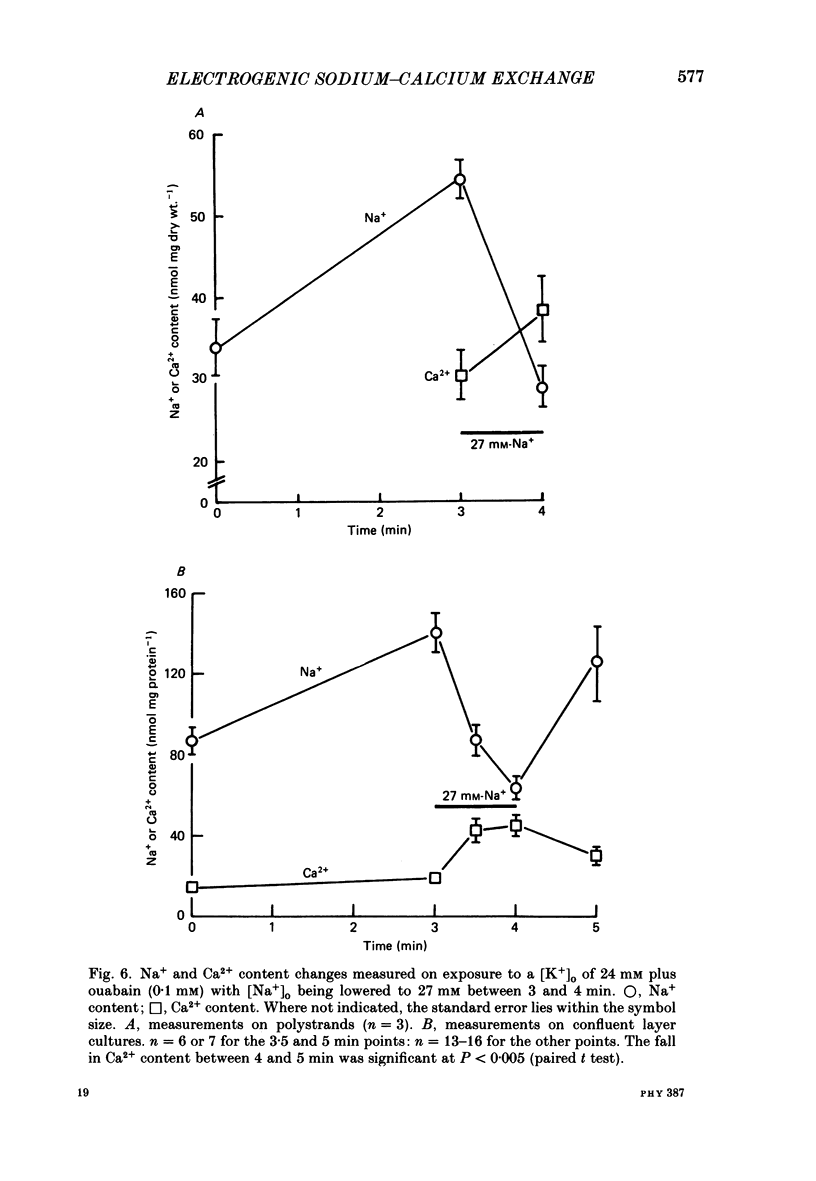
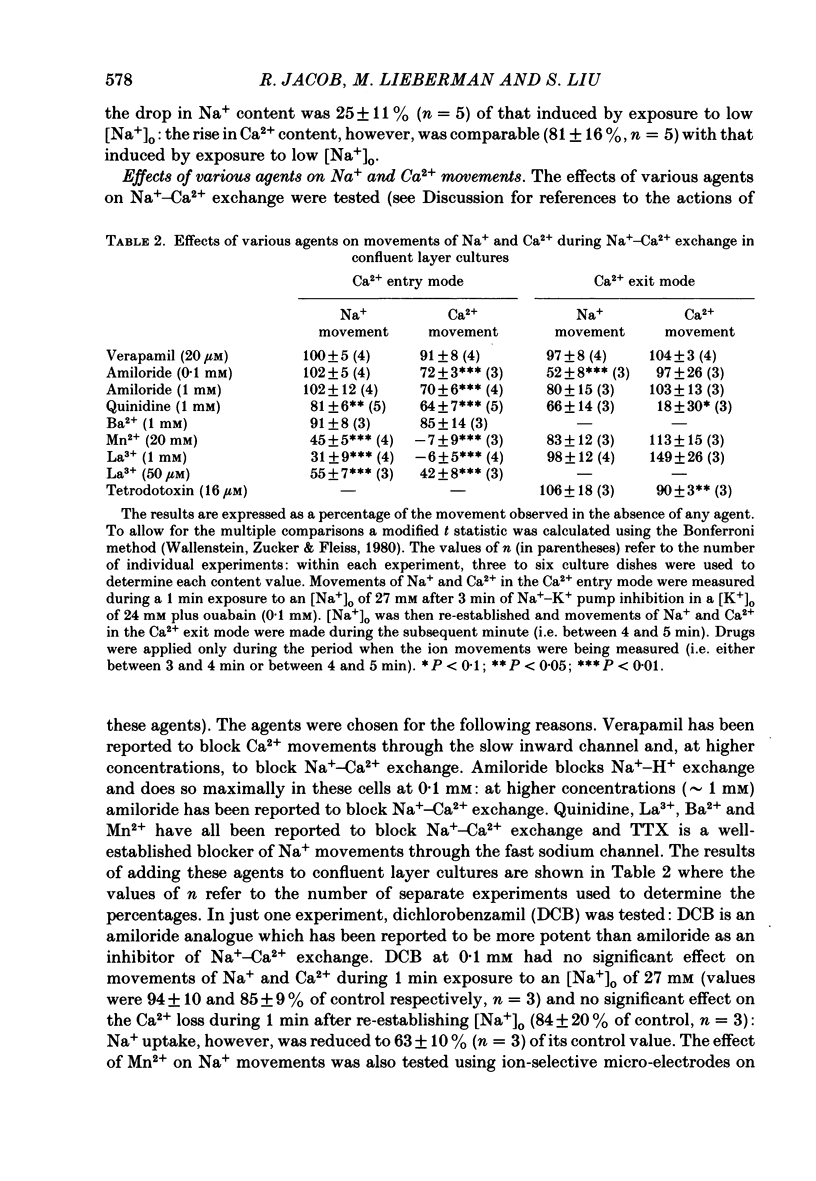
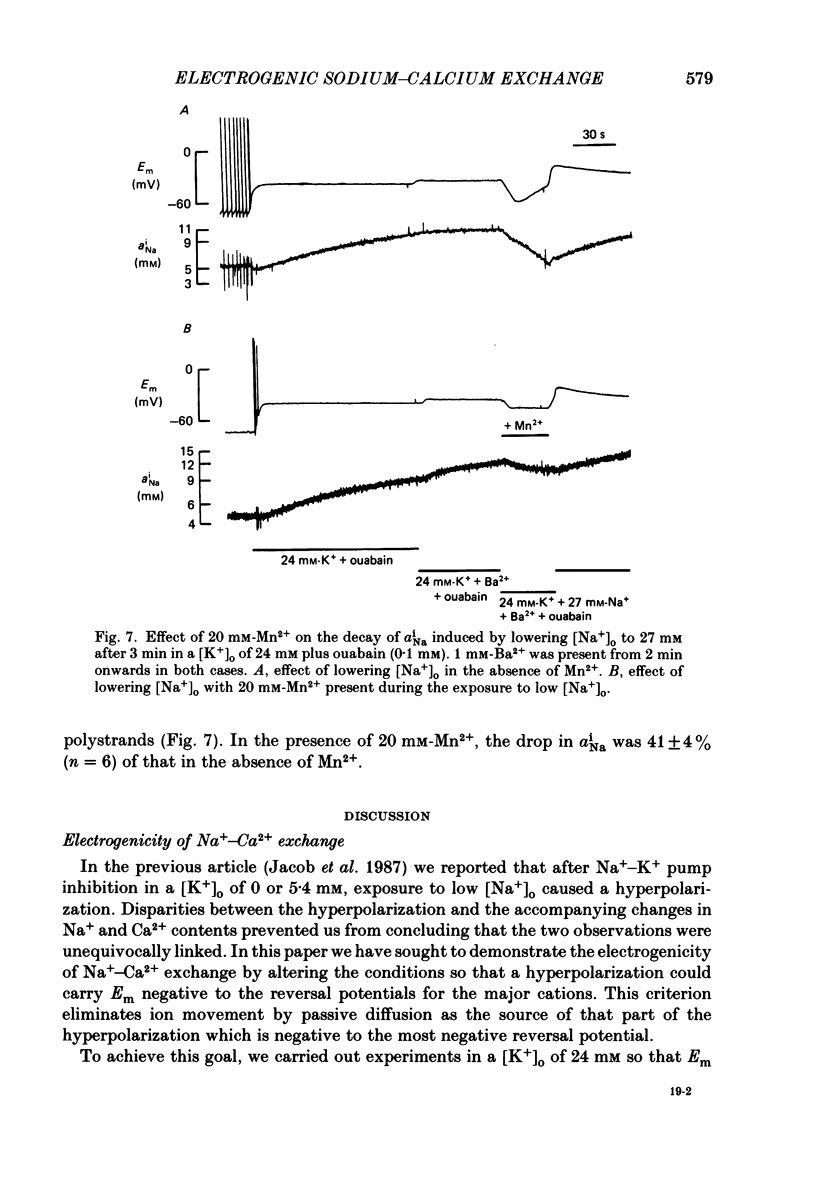
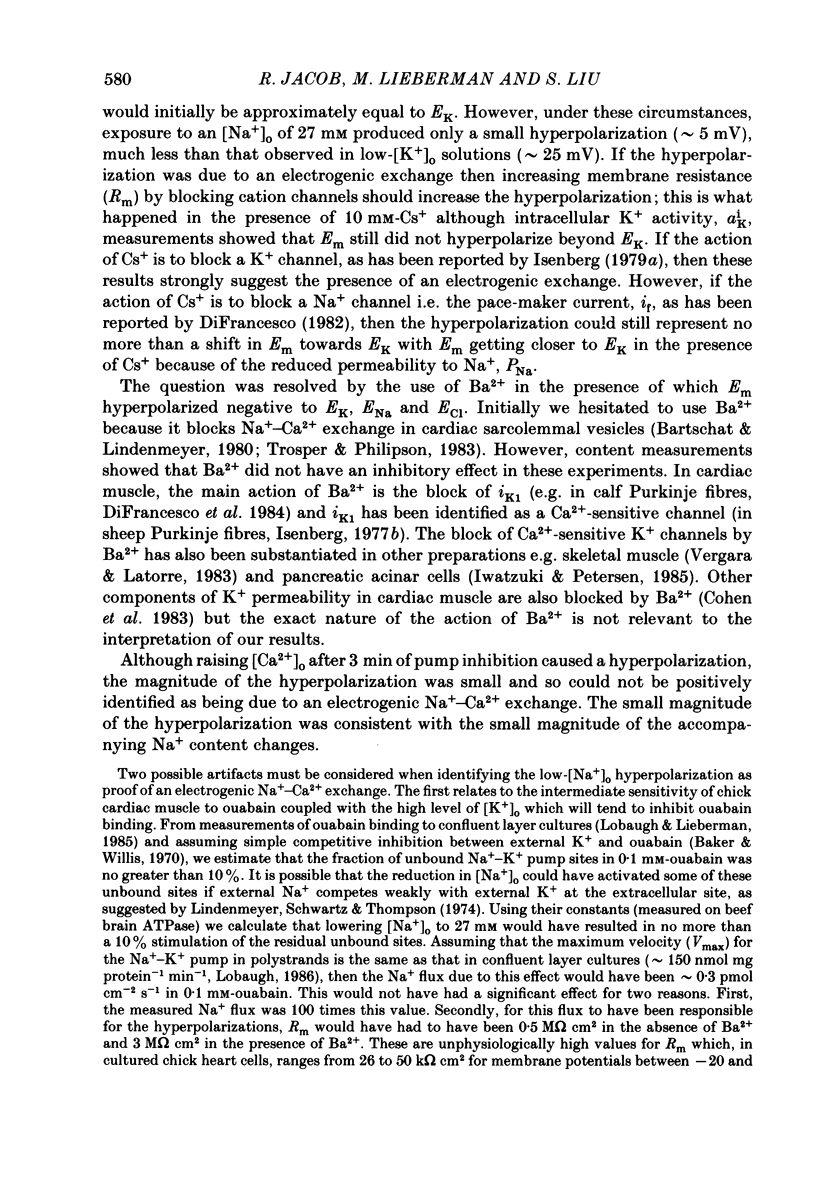
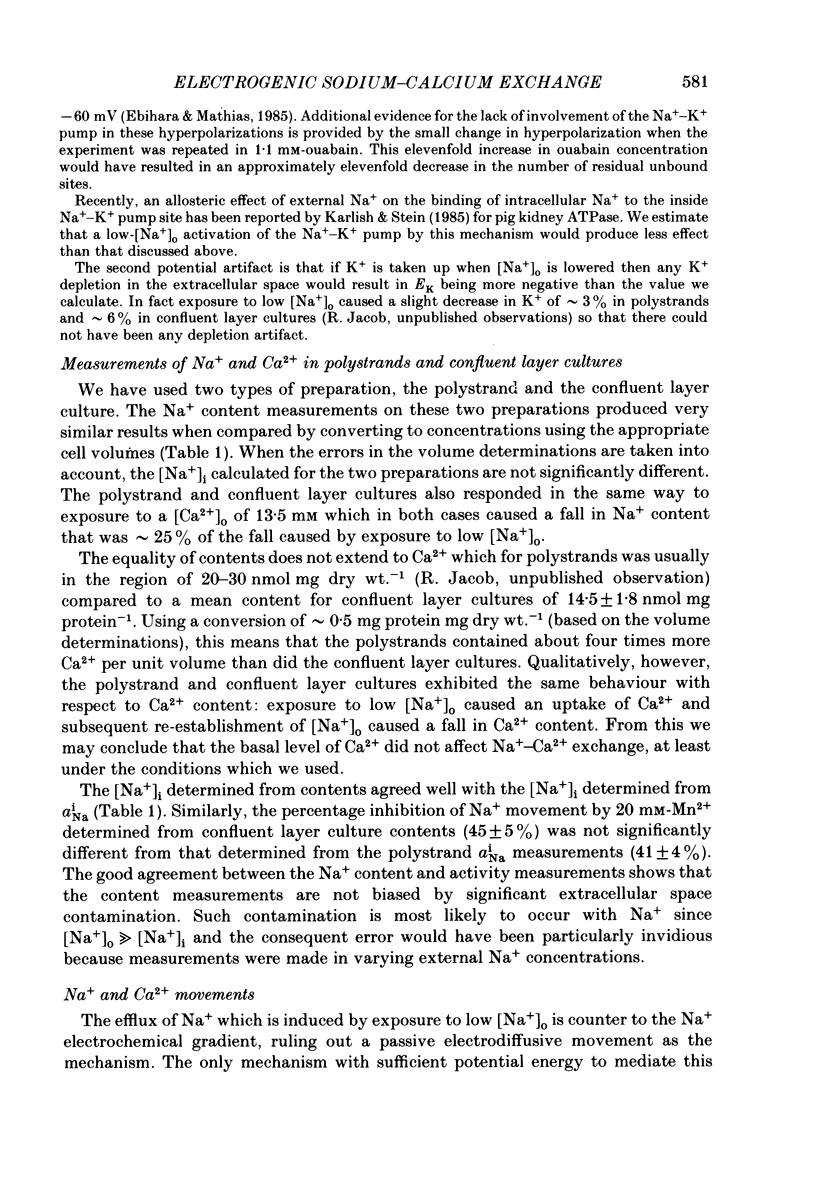
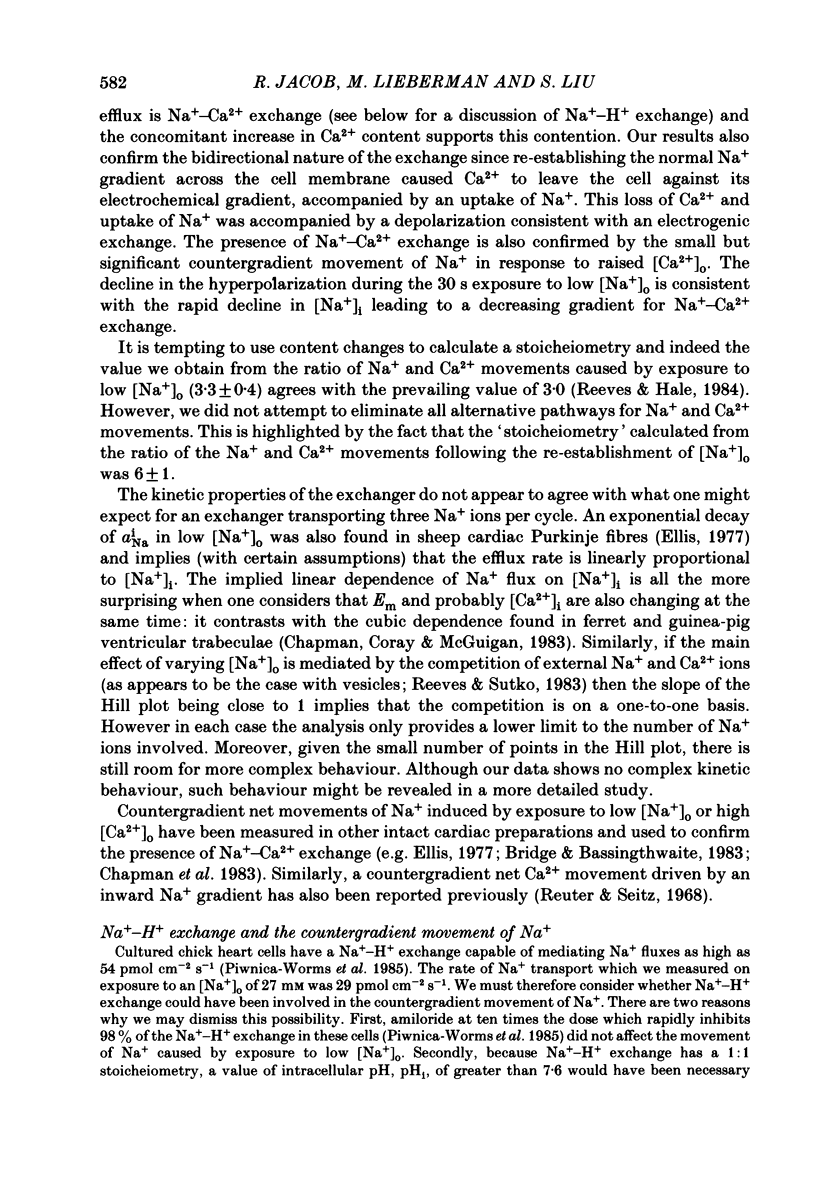
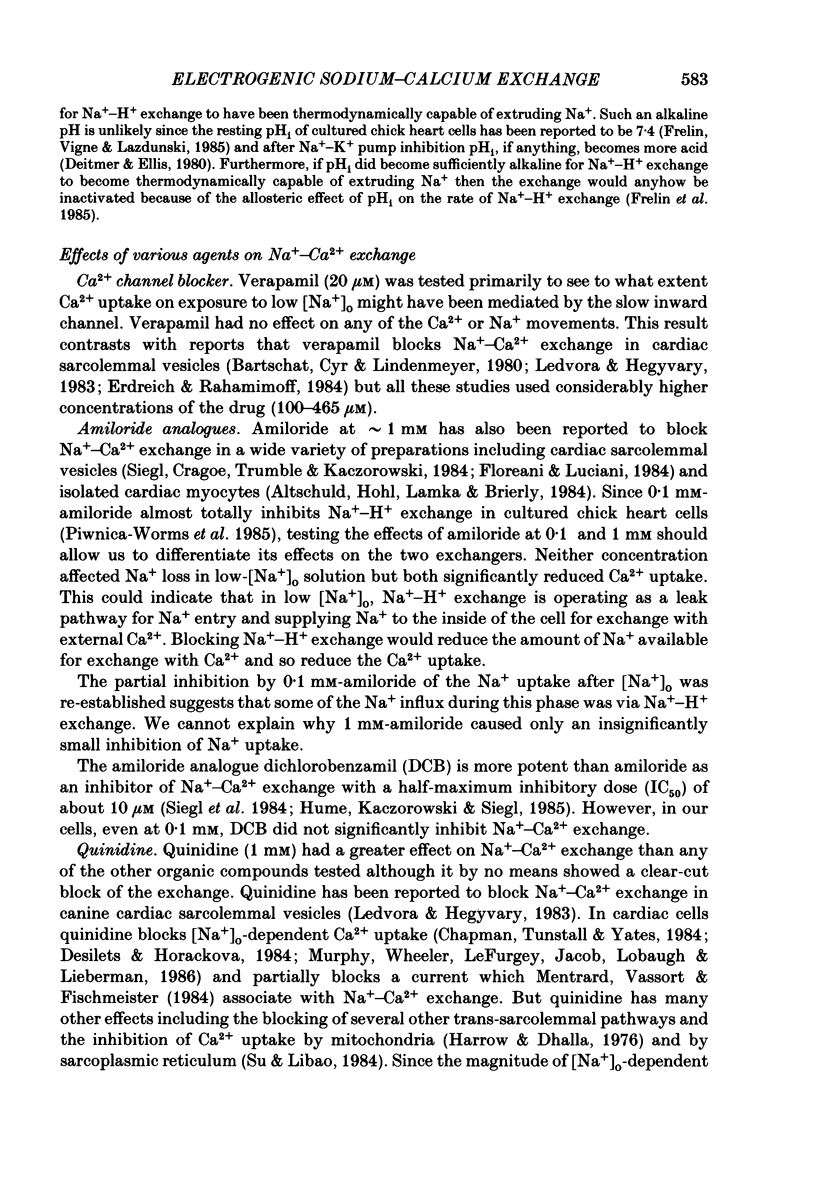
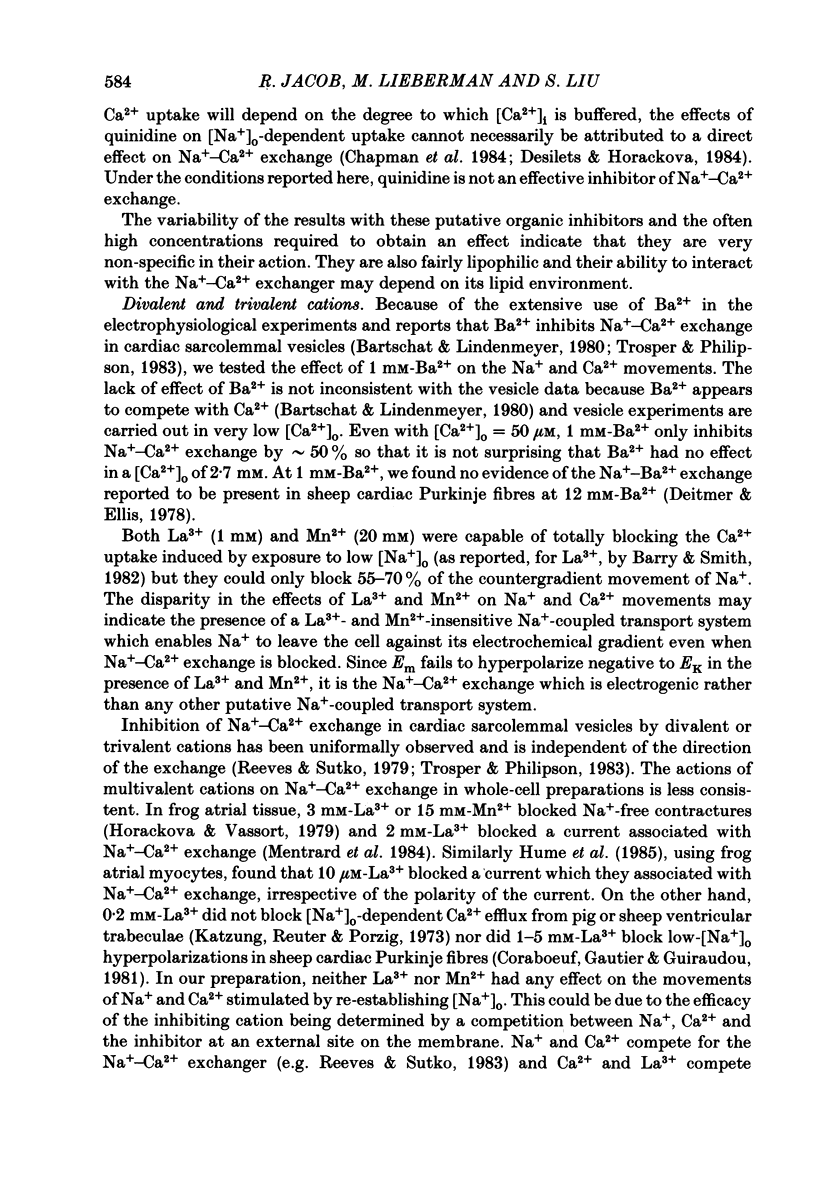
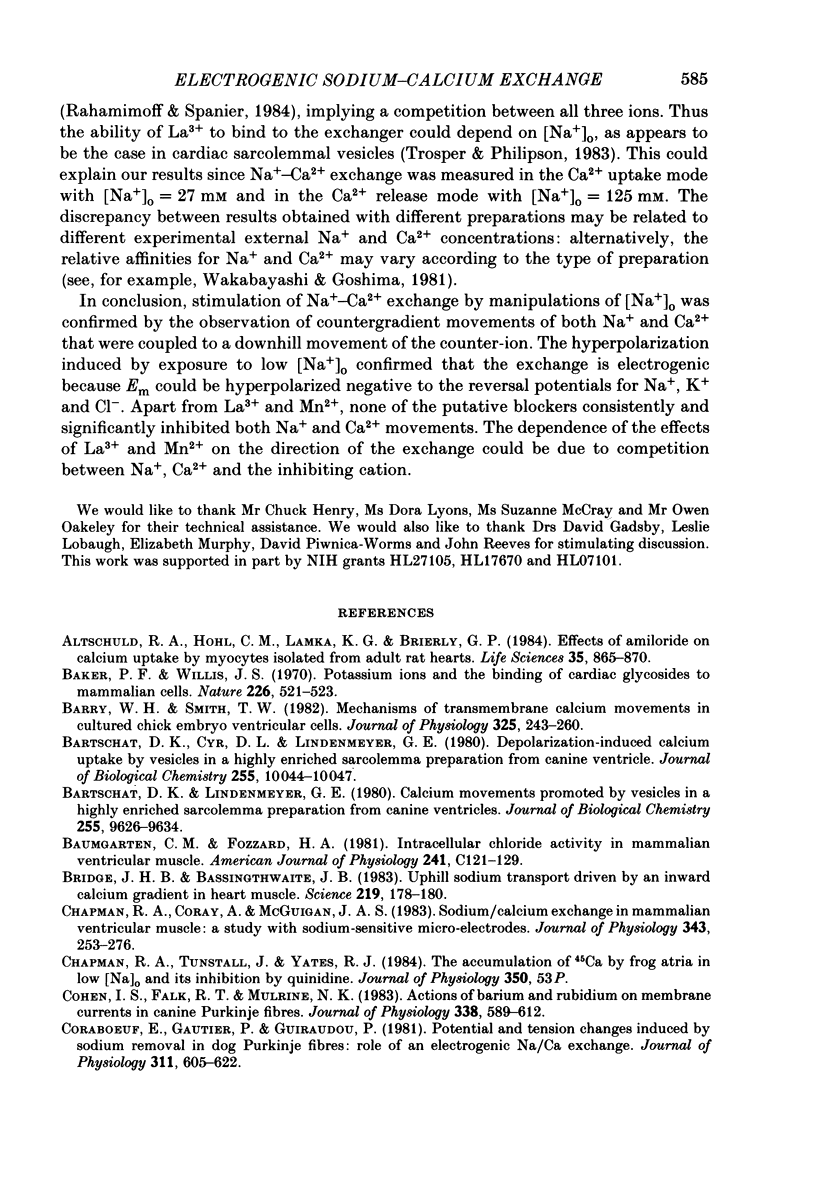
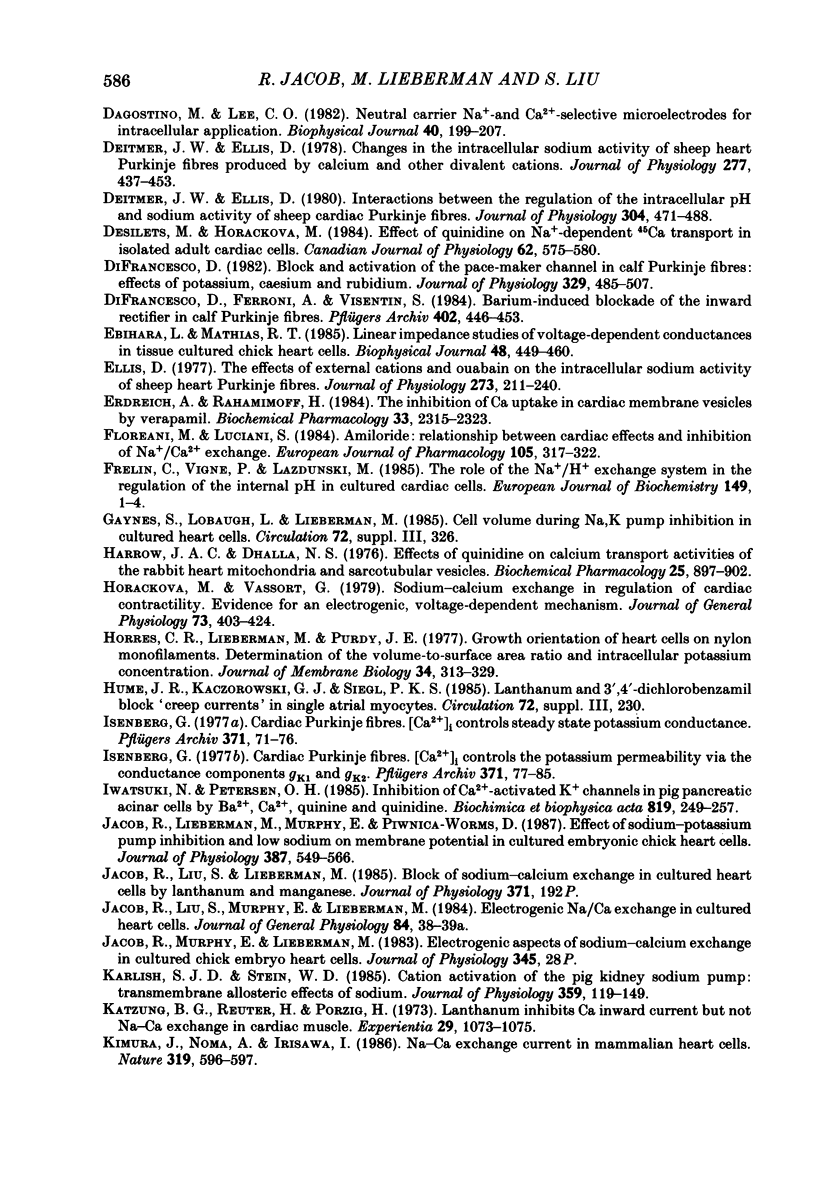
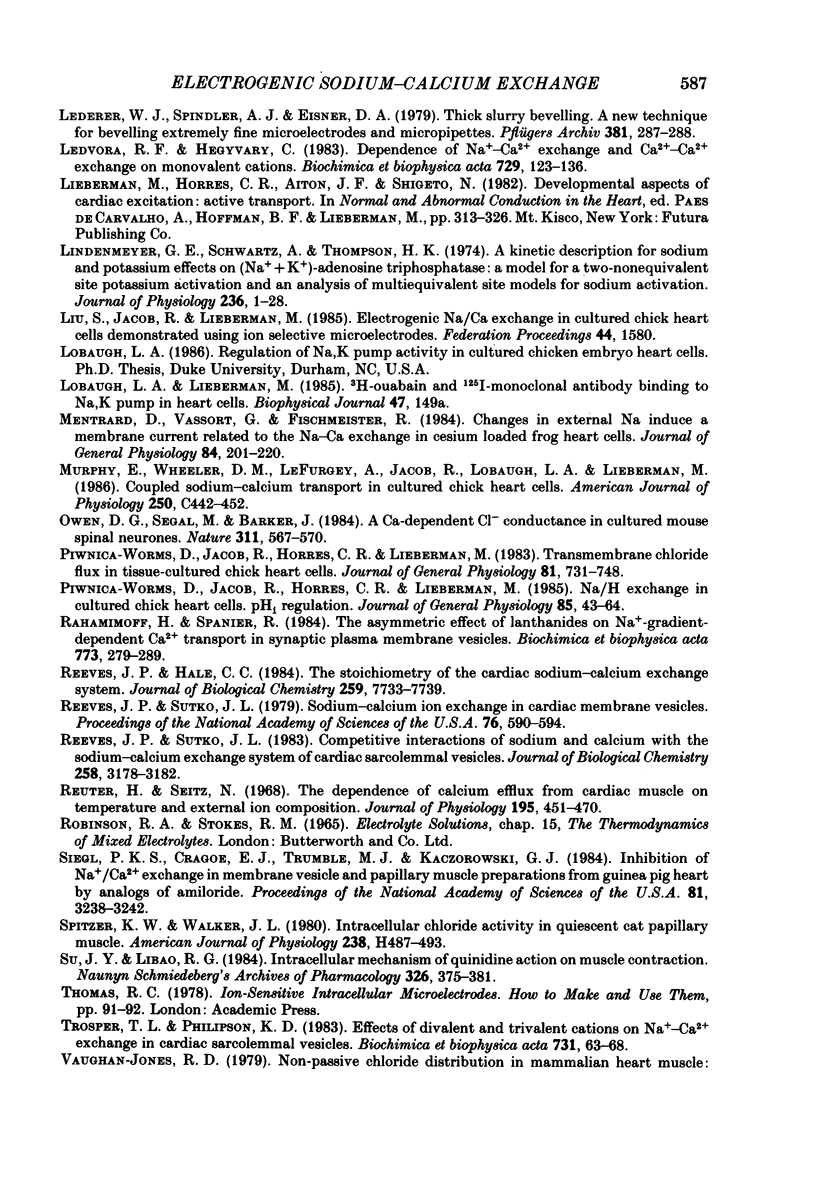
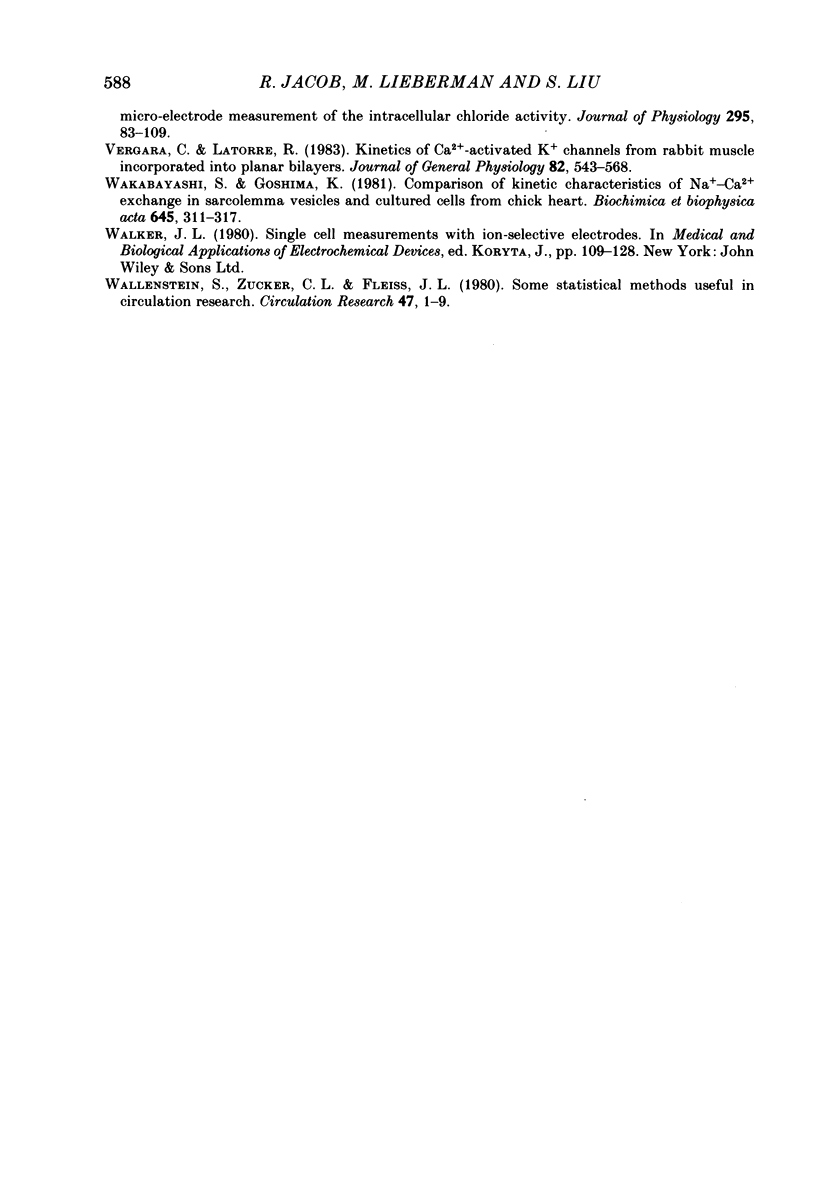
Selected References
These references are in PubMed. This may not be the complete list of references from this article.
- Altschuld R. A., Hohl C. M., Lamka K. G., Brierley G. P. Effects of amiloride on calcium uptake by myocytes isolated from adult rat hearts. Life Sci. 1984 Aug 20;35(8):865–870. doi: 10.1016/0024-3205(84)90412-0. [DOI] [PubMed] [Google Scholar]
- Baker P. F., Willis J. S. Potassium ions and the binding of cardiac glycosides to mammalian cells. Nature. 1970 May 9;226(5245):521–523. doi: 10.1038/226521a0. [DOI] [PubMed] [Google Scholar]
- Barry W. H., Smith T. W. Mechanisms of transmembrane calcium movement in cultured chick embryo ventricular cells. J Physiol. 1982 Apr;325:243–260. doi: 10.1113/jphysiol.1982.sp014148. [DOI] [PMC free article] [PubMed] [Google Scholar]
- Bartschat D. K., Cyr D. L., Lindenmayer G. E. Depolarization-induced calcium uptake by vesicles in a highly enriched sarcolemma preparation from canine ventricle. J Biol Chem. 1980 Nov 10;255(21):10044–10047. [PubMed] [Google Scholar]
- Bartschat D. K., Lindenmayer G. E. Calcium movements promoted by vesicles in a highly enriched sarcolemma preparation from canine ventricle. Calcium-calcium countertransport. J Biol Chem. 1980 Oct 25;255(20):9626–9634. [PubMed] [Google Scholar]
- Baumgarten C. M., Fozzard H. A. Intracellular chloride activity in mammalian ventricular muscle. Am J Physiol. 1981 Sep;241(3):C121–C129. doi: 10.1152/ajpcell.1981.241.3.C121. [DOI] [PubMed] [Google Scholar]
- Bridge J. H., Bassingthwaighte J. B. Uphill sodium transport driven by an inward calcium gradient in heart muscle. Science. 1983 Jan 14;219(4581):178–180. doi: 10.1126/science.6849128. [DOI] [PMC free article] [PubMed] [Google Scholar]
- Chapman R. A., Coray A., McGuigan J. A. Sodium/calcium exchange in mammalian ventricular muscle: a study with sodium-sensitive micro-electrodes. J Physiol. 1983 Oct;343:253–276. doi: 10.1113/jphysiol.1983.sp014891. [DOI] [PMC free article] [PubMed] [Google Scholar]
- Cohen I. S., Falk R. T., Mulrine N. K. Actions of barium and rubidium on membrane currents in canine Purkinje fibres. J Physiol. 1983 May;338:589–612. doi: 10.1113/jphysiol.1983.sp014691. [DOI] [PMC free article] [PubMed] [Google Scholar]
- Croaboeuf E., Gautier P., Giuraudou P. Potential and tension changes induced by sodium removal in dog Purkinje fibres: role of an electrogenic sodium-calcium exchange. J Physiol. 1981 Feb;311:605–622. doi: 10.1113/jphysiol.1981.sp013607. [DOI] [PMC free article] [PubMed] [Google Scholar]
- Dagostino M., Lee C. O. Neutral carrier Na+- and Ca2+-selective microelectrodes for intracellular application. Biophys J. 1982 Dec;40(3):199–207. doi: 10.1016/S0006-3495(82)84475-5. [DOI] [PMC free article] [PubMed] [Google Scholar]
- Deitmer J. W., Ellis D. Changes in the intracellular sodium activity of sheep heart Purkinje fibres produced by calcium and other divalent cations. J Physiol. 1978 Apr;277:437–453. doi: 10.1113/jphysiol.1978.sp012283. [DOI] [PMC free article] [PubMed] [Google Scholar]
- Deitmer J. W., Ellis D. Interactions between the regulation of the intracellular pH and sodium activity of sheep cardiac Purkinje fibres. J Physiol. 1980 Jul;304:471–488. doi: 10.1113/jphysiol.1980.sp013337. [DOI] [PMC free article] [PubMed] [Google Scholar]
- DiFrancesco D. Block and activation of the pace-maker channel in calf purkinje fibres: effects of potassium, caesium and rubidium. J Physiol. 1982 Aug;329:485–507. doi: 10.1113/jphysiol.1982.sp014315. [DOI] [PMC free article] [PubMed] [Google Scholar]
- DiFrancesco D., Ferroni A., Visentin S. Barium-induced blockade of the inward rectifier in calf Purkinje fibres. Pflugers Arch. 1984 Dec;402(4):446–453. doi: 10.1007/BF00583946. [DOI] [PubMed] [Google Scholar]
- Désilets M., Horackova M. Effect of quinidine on Na-dependent 45Ca transport in isolated adult cardiac cells. Can J Physiol Pharmacol. 1984 May;62(5):575–580. doi: 10.1139/y84-092. [DOI] [PubMed] [Google Scholar]
- Ebihara L., Mathias R. T. Linear impedance studies of voltage-dependent conductances in tissue cultured chick heart cells. Biophys J. 1985 Sep;48(3):449–460. doi: 10.1016/S0006-3495(85)83800-5. [DOI] [PMC free article] [PubMed] [Google Scholar]
- Ellis D. The effects of external cations and ouabain on the intracellular sodium activity of sheep heart Purkinje fibres. J Physiol. 1977 Dec;273(1):211–240. doi: 10.1113/jphysiol.1977.sp012090. [DOI] [PMC free article] [PubMed] [Google Scholar]
- Erdreich A., Rahamimoff H. The inhibition of Ca uptake in cardiac membrane vesicles by verapamil. Biochem Pharmacol. 1984 Jul 15;33(14):2315–2323. doi: 10.1016/0006-2952(84)90672-5. [DOI] [PubMed] [Google Scholar]
- Floreani M., Luciani S. Amiloride: relationship between cardiac effects and inhibition of Na+/Ca2+ exchange. Eur J Pharmacol. 1984 Oct 15;105(3-4):317–322. doi: 10.1016/0014-2999(84)90624-1. [DOI] [PubMed] [Google Scholar]
- Frelin C., Vigne P., Lazdunski M. The role of the Na+/H+ exchange system in the regulation of the internal pH in cultured cardiac cells. Eur J Biochem. 1985 May 15;149(1):1–4. doi: 10.1111/j.1432-1033.1985.tb08884.x. [DOI] [PubMed] [Google Scholar]
- Harrow J. A., Dhalla N. S. Effects of quinidine on calcium transport activities of the rabbit heart mitochondria and sarcotubular vesicles. Biochem Pharmacol. 1976 Apr 15;25(8):897–902. doi: 10.1016/0006-2952(76)90311-7. [DOI] [PubMed] [Google Scholar]
- Horackova M., Vassort G. Sodium-calcium exchange in regulation of cardiac contractility. Evidence for an electrogenic, voltage-dependent mechanism. J Gen Physiol. 1979 Apr;73(4):403–424. doi: 10.1085/jgp.73.4.403. [DOI] [PMC free article] [PubMed] [Google Scholar]
- Horres C. R., Lieberman M., Purdy J. E. Growth orientation of heart cells on nylon monofilament. Determination of the volume-to-surface area ratio and intracellular potassium concentration. J Membr Biol. 1977 Jun 15;34(4):313–329. doi: 10.1007/BF01870306. [DOI] [PubMed] [Google Scholar]
- Isenberg G. Cardiac Purkinje fibres: [Ca2+]i controls steady state potassium conductance. Pflugers Arch. 1977 Oct 19;371(1-2):71–76. doi: 10.1007/BF00580774. [DOI] [PubMed] [Google Scholar]
- Isenberg G. Cardiac Purkinje fibres: [Ca2+]i controls the potassium permeability via the conductance components gK1 and gK2. Pflugers Arch. 1977 Oct 19;371(1-2):77–85. doi: 10.1007/BF00580775. [DOI] [PubMed] [Google Scholar]
- Iwatsuki N., Petersen O. H. Inhibition of Ca2+-activated K+ channels in pig pancreatic acinar cells by Ba2+, Ca2+, quinine and quinidine. Biochim Biophys Acta. 1985 Oct 10;819(2):249–257. doi: 10.1016/0005-2736(85)90180-4. [DOI] [PubMed] [Google Scholar]
- Jacob R., Lieberman M., Murphy E., Piwnica-Worms D. Effects of sodium-potassium pump inhibition and low sodium on membrane potential in cultured embryonic chick heart cells. J Physiol. 1987 Jun;387:549–566. doi: 10.1113/jphysiol.1987.sp016588. [DOI] [PMC free article] [PubMed] [Google Scholar]
- Karlish S. J., Stein W. D. Cation activation of the pig kidney sodium pump: transmembrane allosteric effects of sodium. J Physiol. 1985 Feb;359:119–149. doi: 10.1113/jphysiol.1985.sp015578. [DOI] [PMC free article] [PubMed] [Google Scholar]
- Katzung B. G., Reuter H., Porzig H. Lanthanum inhibits Ca inward current but not Na-Ca exchange in cardiac muscle. Experientia. 1973 Sep 15;29(9):1073–1075. doi: 10.1007/BF01946727. [DOI] [PubMed] [Google Scholar]
- Kimura J., Noma A., Irisawa H. Na-Ca exchange current in mammalian heart cells. Nature. 1986 Feb 13;319(6054):596–597. doi: 10.1038/319596a0. [DOI] [PubMed] [Google Scholar]
- Lederer W. J., Spindler A. J., Eisner D. A. Thick slurry bevelling: a new technique for bevelling extremely fine microelectrodes and micropipettes. Pflugers Arch. 1979 Sep;381(3):287–288. doi: 10.1007/BF00583261. [DOI] [PubMed] [Google Scholar]
- Ledvora R. F., Hegyvary C. Dependence of Na+-Ca2+ exchange and Ca2+-Ca2+ exchange on monovalent cations. Biochim Biophys Acta. 1983 Mar 23;729(1):123–136. doi: 10.1016/0005-2736(83)90463-7. [DOI] [PubMed] [Google Scholar]
- Lindenmayer G. E., Schwartz A., Thompson H. K., Jr A kinetic description for sodium and potassium effects on (Na+ plus K+)-adenosine triphosphatase: a model for a two-nonequivalent site potassium activation and an analysis of multiequivalent site models for sodium activation. J Physiol. 1974 Jan;236(1):1–28. doi: 10.1113/jphysiol.1974.sp010419. [DOI] [PMC free article] [PubMed] [Google Scholar]
- Mentrard D., Vassort G., Fischmeister R. Changes in external Na induce a membrane current related to the Na-Ca exchange in cesium-loaded frog heart cells. J Gen Physiol. 1984 Aug;84(2):201–220. doi: 10.1085/jgp.84.2.201. [DOI] [PMC free article] [PubMed] [Google Scholar]
- Murphy E., Wheeler D. M., LeFurgey A., Jacob R., Lobaugh L. A., Lieberman M. Coupled sodium-calcium transport in cultured chick heart cells. Am J Physiol. 1986 Mar;250(3 Pt 1):C442–C452. doi: 10.1152/ajpcell.1986.250.3.C442. [DOI] [PubMed] [Google Scholar]
- Owen D. G., Segal M., Barker J. L. A Ca-dependent Cl- conductance in cultured mouse spinal neurones. Nature. 1984 Oct 11;311(5986):567–570. doi: 10.1038/311567a0. [DOI] [PubMed] [Google Scholar]
- Piwnica-Worms D., Jacob R., Horres C. R., Lieberman M. Na/H exchange in cultured chick heart cells. pHi regulation. J Gen Physiol. 1985 Jan;85(1):43–64. doi: 10.1085/jgp.85.1.43. [DOI] [PMC free article] [PubMed] [Google Scholar]
- Piwnica-Worms D., Jacob R., Horres C. R., Lieberman M. Transmembrane chloride flux in tissue-cultured chick heart cells. J Gen Physiol. 1983 May;81(5):731–748. doi: 10.1085/jgp.81.5.731. [DOI] [PMC free article] [PubMed] [Google Scholar]
- Rahamimoff H., Spanier R. The asymmetric effect of lanthanides on Na+-gradient-dependent Ca2+ transport in synaptic plasma membrane vesicles. Biochim Biophys Acta. 1984 Jun 27;773(2):279–289. doi: 10.1016/0005-2736(84)90092-0. [DOI] [PubMed] [Google Scholar]
- Reeves J. P., Hale C. C. The stoichiometry of the cardiac sodium-calcium exchange system. J Biol Chem. 1984 Jun 25;259(12):7733–7739. [PubMed] [Google Scholar]
- Reeves J. P., Sutko J. L. Competitive interactions of sodium and calcium with the sodium-calcium exchange system of cardiac sarcolemmal vesicles. J Biol Chem. 1983 Mar 10;258(5):3178–3182. [PubMed] [Google Scholar]
- Reeves J. P., Sutko J. L. Sodium-calcium ion exchange in cardiac membrane vesicles. Proc Natl Acad Sci U S A. 1979 Feb;76(2):590–594. doi: 10.1073/pnas.76.2.590. [DOI] [PMC free article] [PubMed] [Google Scholar]
- Reuter H., Seitz N. The dependence of calcium efflux from cardiac muscle on temperature and external ion composition. J Physiol. 1968 Mar;195(2):451–470. doi: 10.1113/jphysiol.1968.sp008467. [DOI] [PMC free article] [PubMed] [Google Scholar]
- Siegl P. K., Cragoe E. J., Jr, Trumble M. J., Kaczorowski G. J. Inhibition of Na+/Ca2+ exchange in membrane vesicle and papillary muscle preparations from guinea pig heart by analogs of amiloride. Proc Natl Acad Sci U S A. 1984 May;81(10):3238–3242. doi: 10.1073/pnas.81.10.3238. [DOI] [PMC free article] [PubMed] [Google Scholar]
- Spitzer K. W., Walker J. L. Intracellular chloride activity in quiescent cat papillary muscle. Am J Physiol. 1980 Apr;238(4):H487–H493. doi: 10.1152/ajpheart.1980.238.4.H487. [DOI] [PubMed] [Google Scholar]
- Su J. Y., Libao R. G. Intracellular mechanism of quinidine action on muscle contraction. A comparison between rabbit cardiac and skeletal muscle. Naunyn Schmiedebergs Arch Pharmacol. 1984 Jul;326(4):375–381. doi: 10.1007/BF00501446. [DOI] [PubMed] [Google Scholar]
- Trosper T. L., Philipson K. D. Effects of divalent and trivalent cations on Na+-Ca2+ exchange in cardiac sarcolemmal vesicles. Biochim Biophys Acta. 1983 May 26;731(1):63–68. doi: 10.1016/0005-2736(83)90398-x. [DOI] [PubMed] [Google Scholar]
- Vaughan-Jones R. D. Non-passive chloride distribution in mammalian heart muscle: micro-electrode measurement of the intracellular chloride activity. J Physiol. 1979 Oct;295:83–109. doi: 10.1113/jphysiol.1979.sp012956. [DOI] [PMC free article] [PubMed] [Google Scholar]
- Vergara C., Latorre R. Kinetics of Ca2+-activated K+ channels from rabbit muscle incorporated into planar bilayers. Evidence for a Ca2+ and Ba2+ blockade. J Gen Physiol. 1983 Oct;82(4):543–568. doi: 10.1085/jgp.82.4.543. [DOI] [PMC free article] [PubMed] [Google Scholar]
- Wakabayashi S., Goshima K. Comparison of kinetic characteristics of Na+-Ca2+ exchange in sarcolemma vesicles and cultured cells from chick heart. Biochim Biophys Acta. 1981 Jul 20;645(2):311–317. doi: 10.1016/0005-2736(81)90202-9. [DOI] [PubMed] [Google Scholar]
- Wallenstein S., Zucker C. L., Fleiss J. L. Some statistical methods useful in circulation research. Circ Res. 1980 Jul;47(1):1–9. doi: 10.1161/01.res.47.1.1. [DOI] [PubMed] [Google Scholar]