Abstract
1. When cardiac muscle is stimulated after a rest there is a gradual increase in force development over several minutes. The origin of this 'force staircase' was investigated in experiments on sheep and dog Purkinje fibres. Particular attention was paid to the possible role of changes in the intracellular Na+ activity (aNAi). 2. The first line of evidence for a role for aiNa came from a comparison of sheep and dog Purkinje fibres generating action potentials: after a change in the stimulus rate the slow changes of both aNai and force were monophasic in sheep but biphasic in dog preparations. 3. In the remaining experiments changes in aNai and force in sheep preparations were measured during 4 min trains of voltage-clamp pulses at a frequency of 2.5 Hz. 4. A number of these voltage-clamp experiments also indicated that changes in aNai are involved. Depending on the preparation and the duration of the pulses aNai rose or fell during a train-a rise in aNai was always associated with a gradual rise in force, whereas a fall in aNai was usually accompanied by a gradual fall in force. The addition of tetrodotoxin (TTX) or the use of a low holding potential reduced the progressive rises of both aNai and force, whereas the inclusion of a 10 mV hyperpolarization between pulses potentiated the progressive rises of both. 5. The effect of TTX on the staircase was more marked the longer the pulses during the train; this possibly indicates that the effect of aNai on the force staircase is complex and is more marked with longer pulses. 6. A rise in aNai was shown not to be the only factor underlying the progressive increase in force, because in many preparations a gradual rise in force occurred in spite of no change or even a fall of aNai. 7. It is concluded that an increase in aNai is involved in the slow increase in force during the staircase accompanying a train of action potentials, and that other factors are also involved; various possibilities are discussed.
Full text
PDF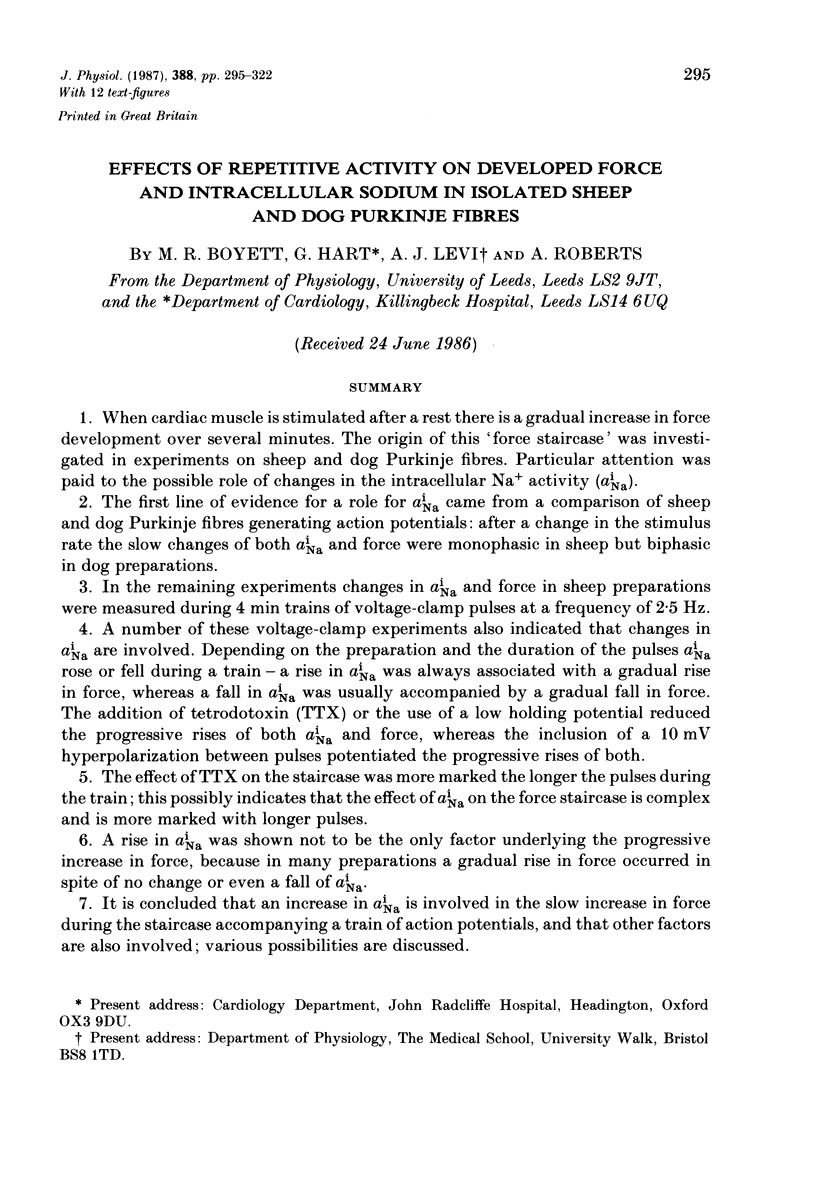
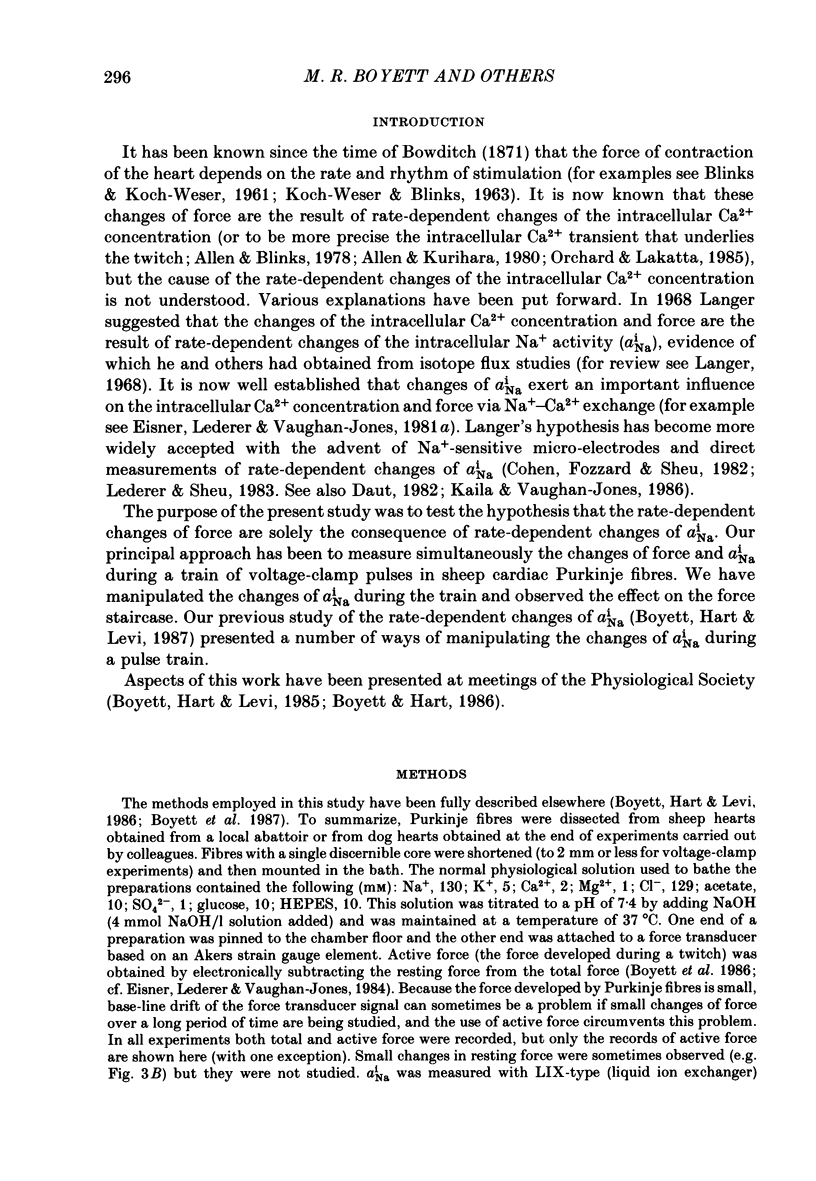
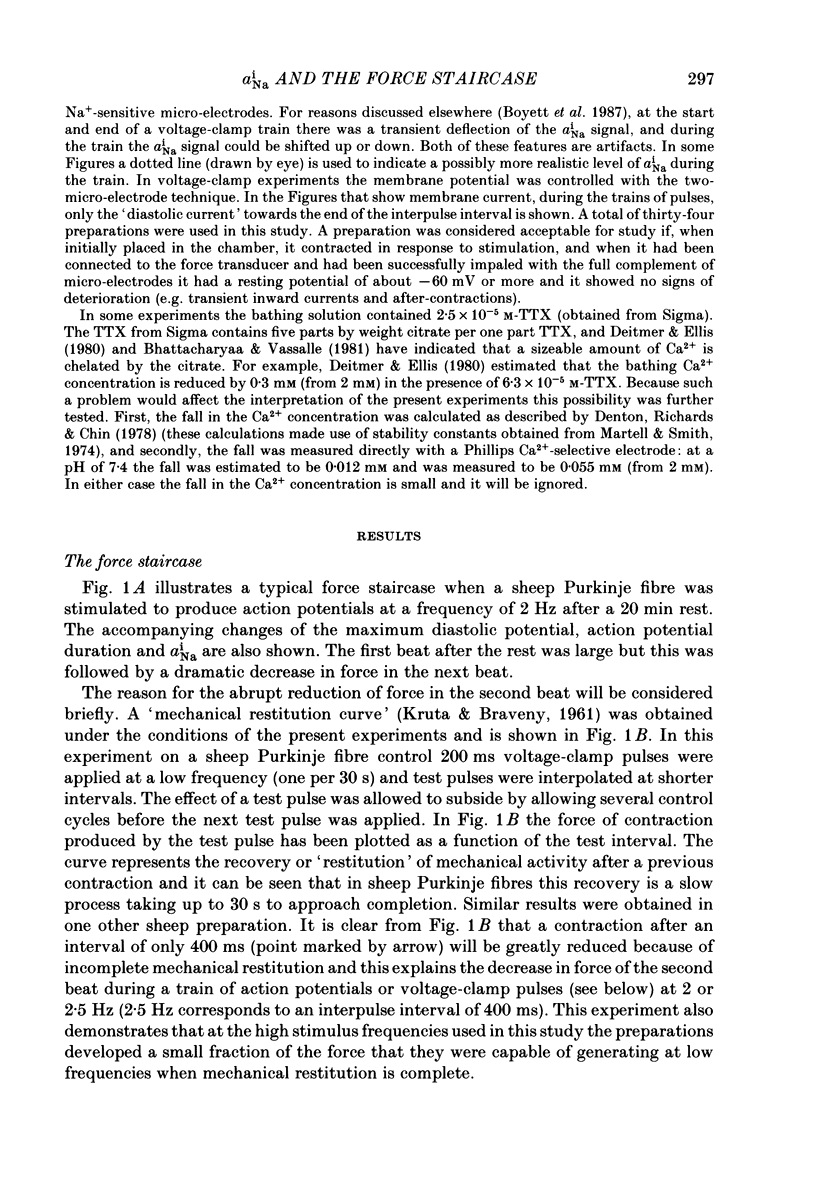
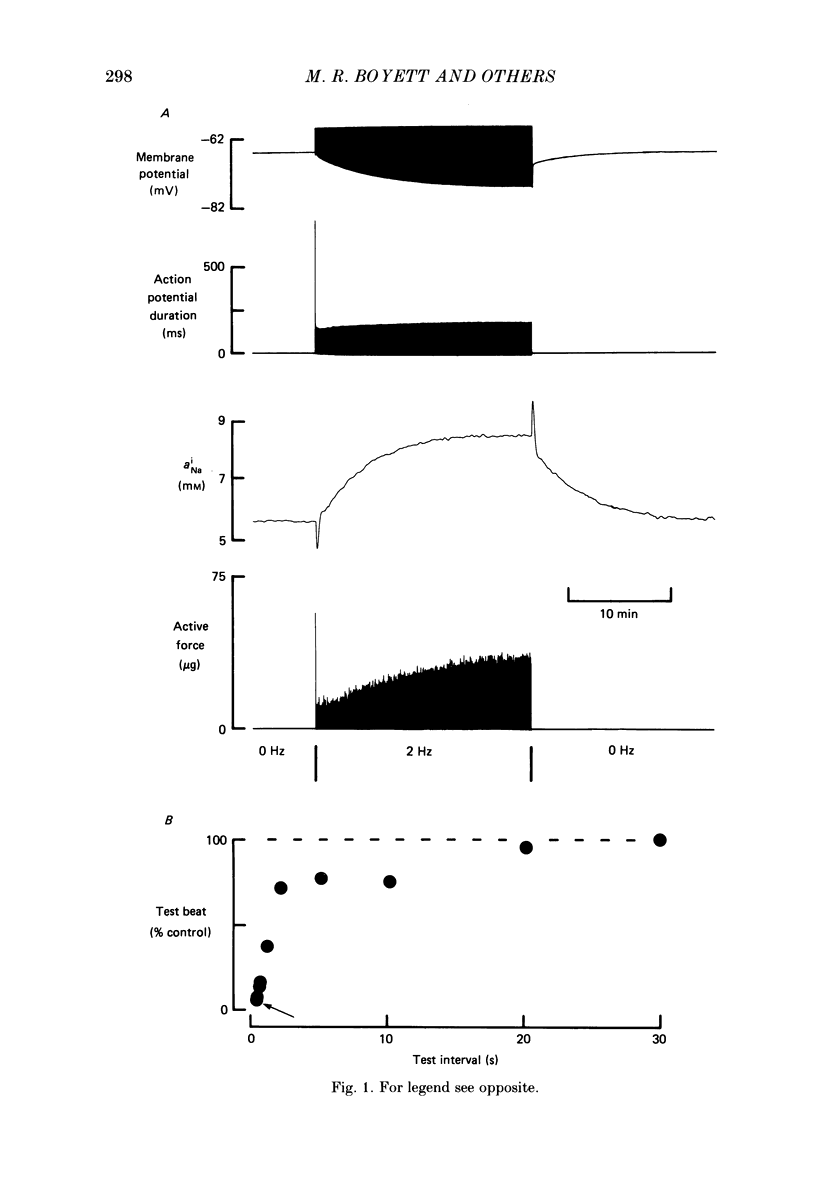
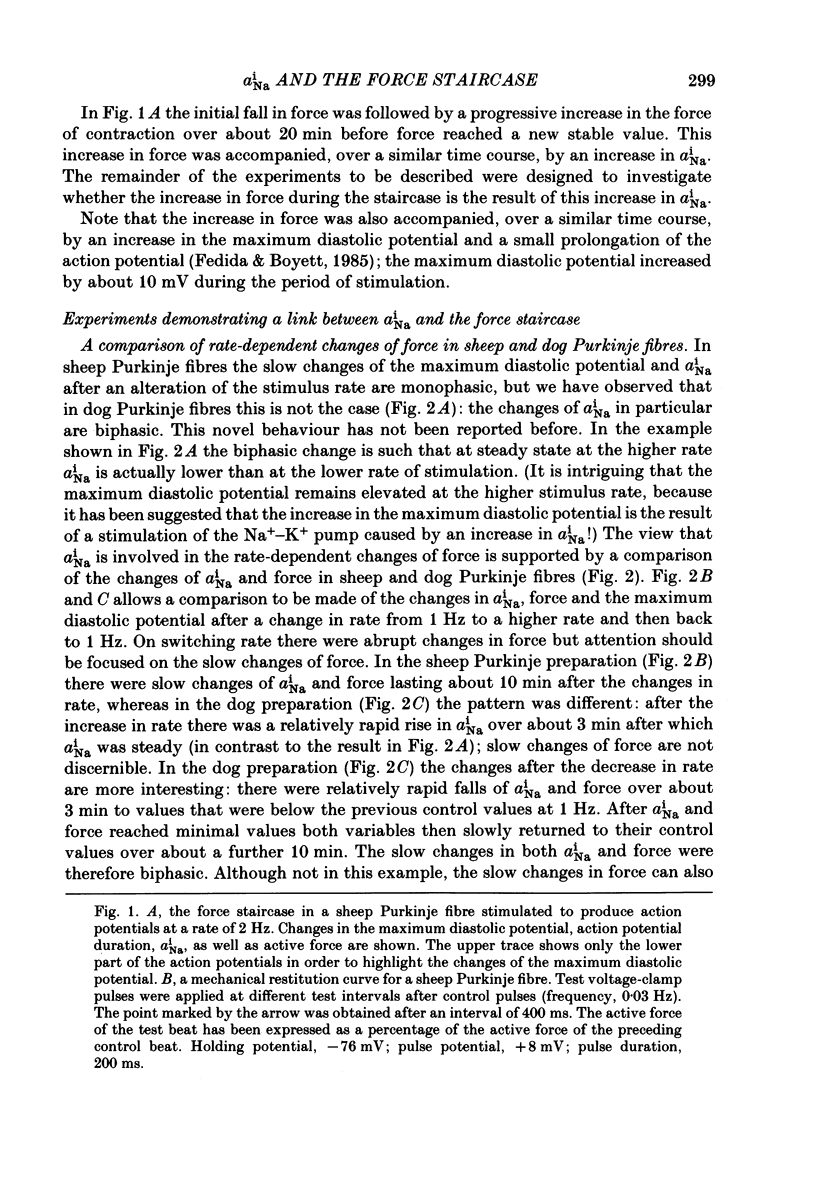
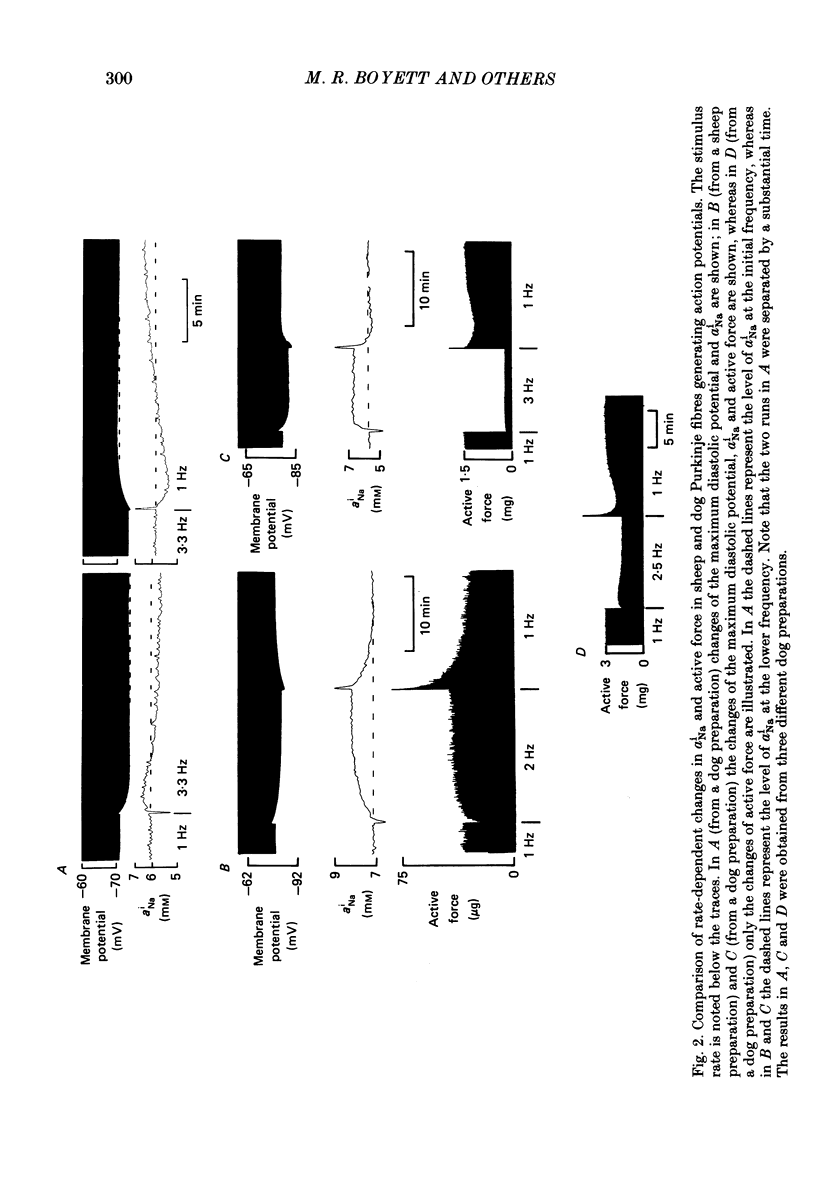
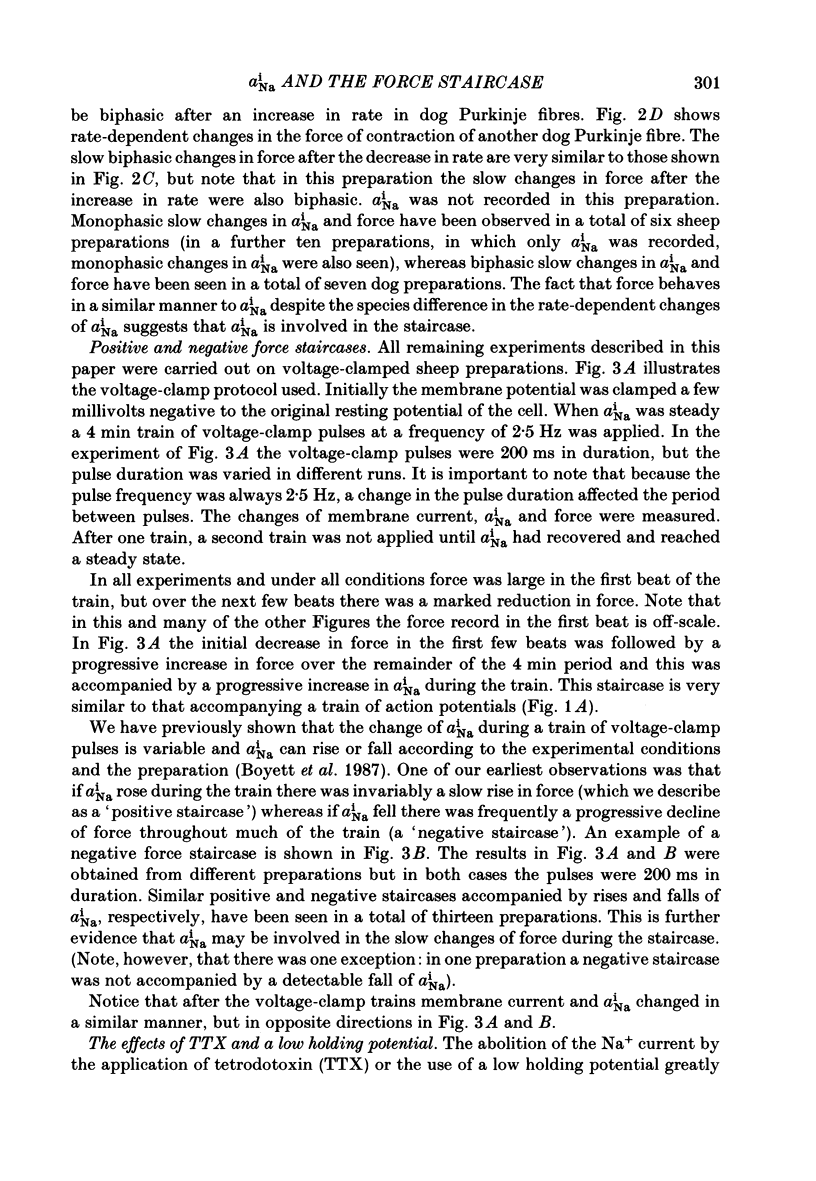
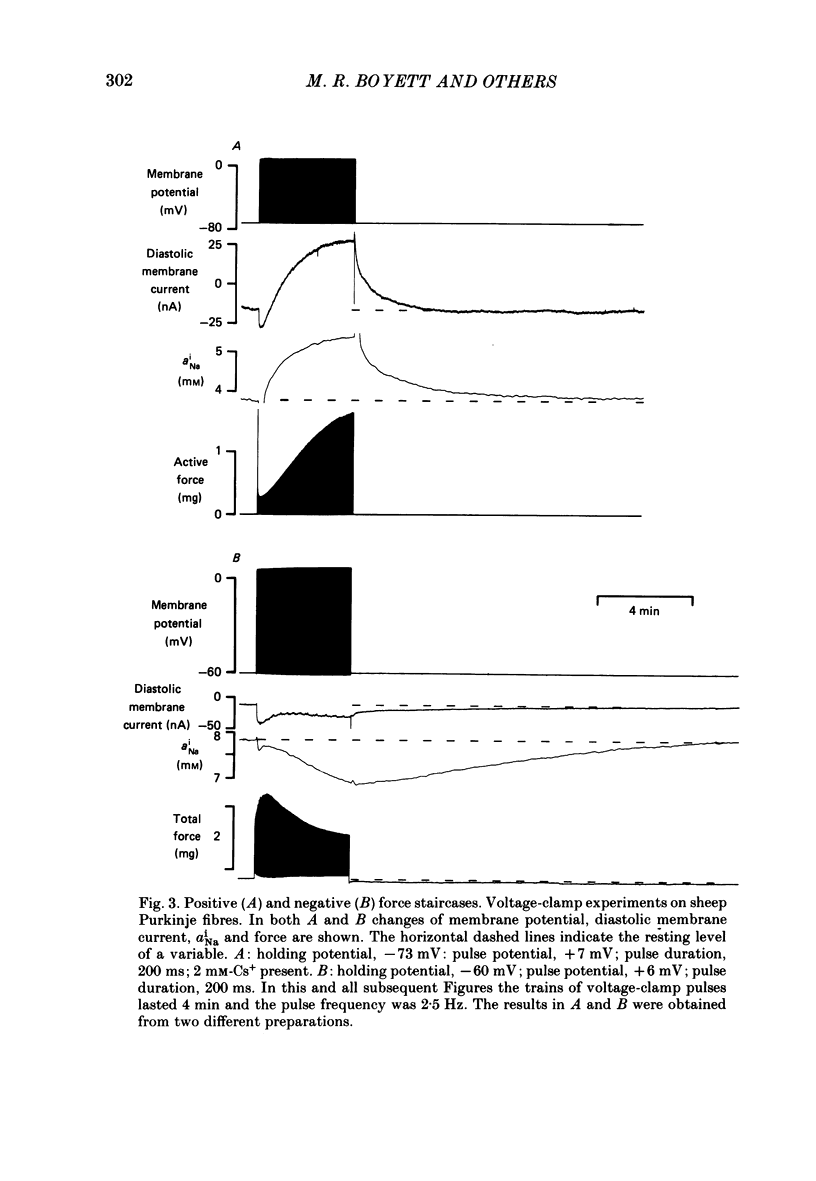
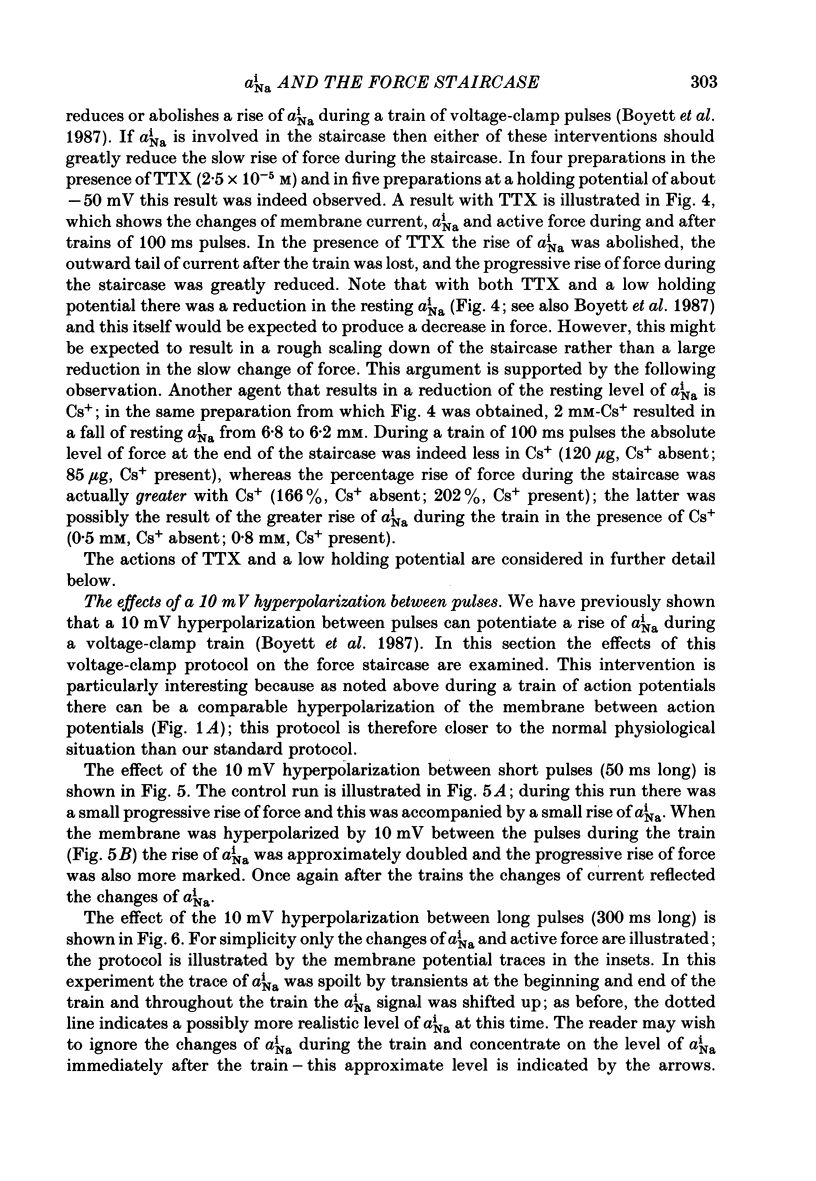
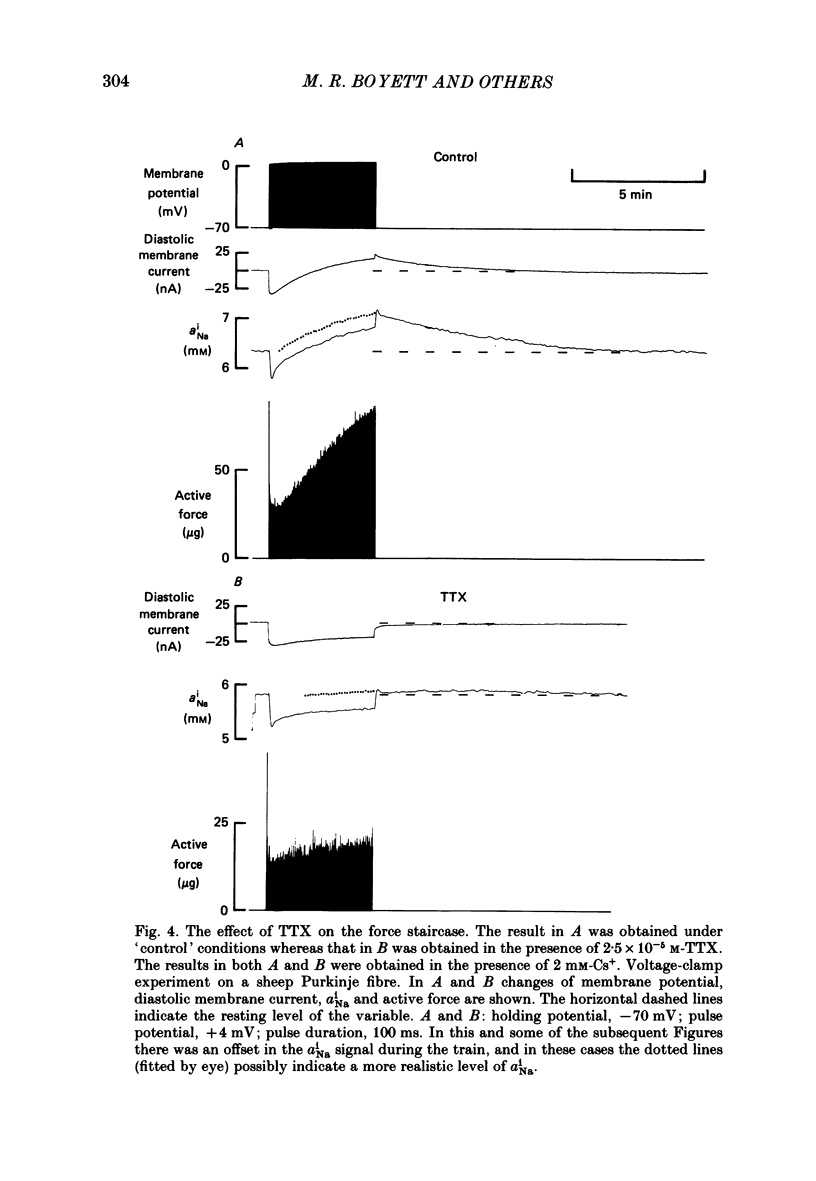
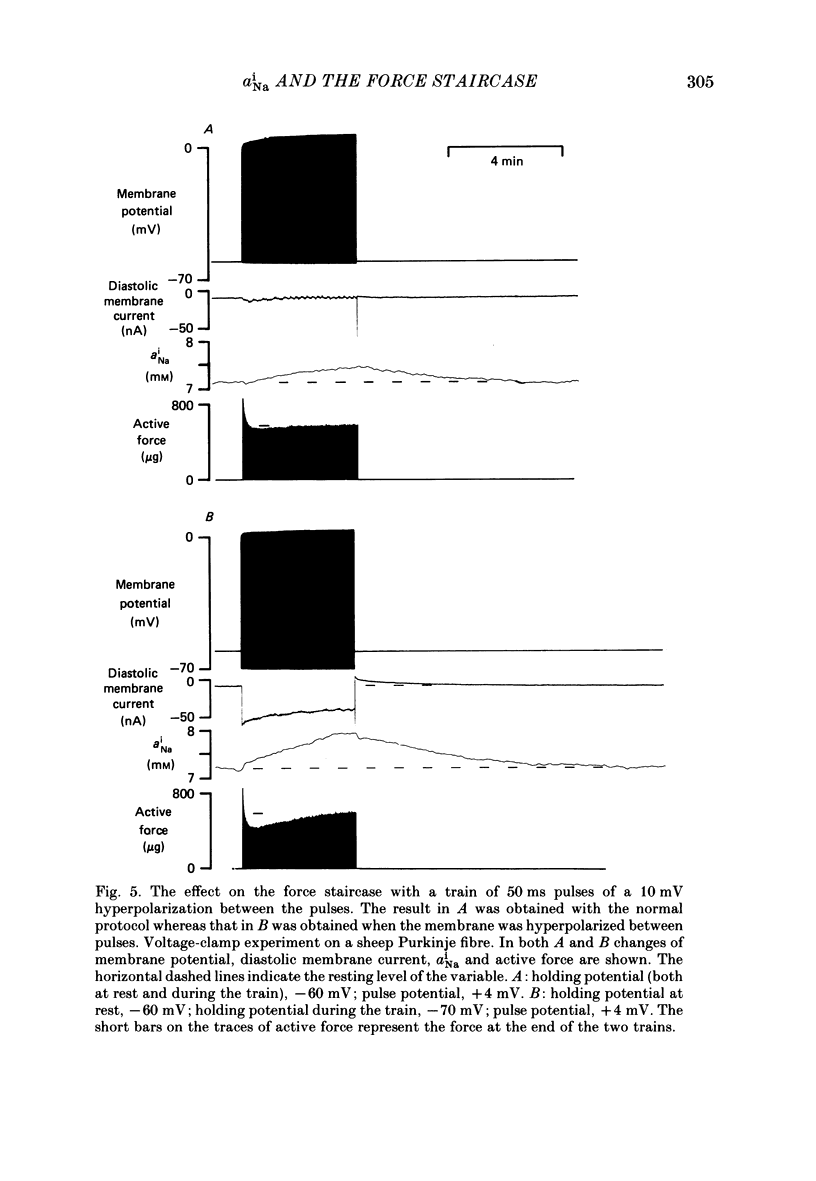
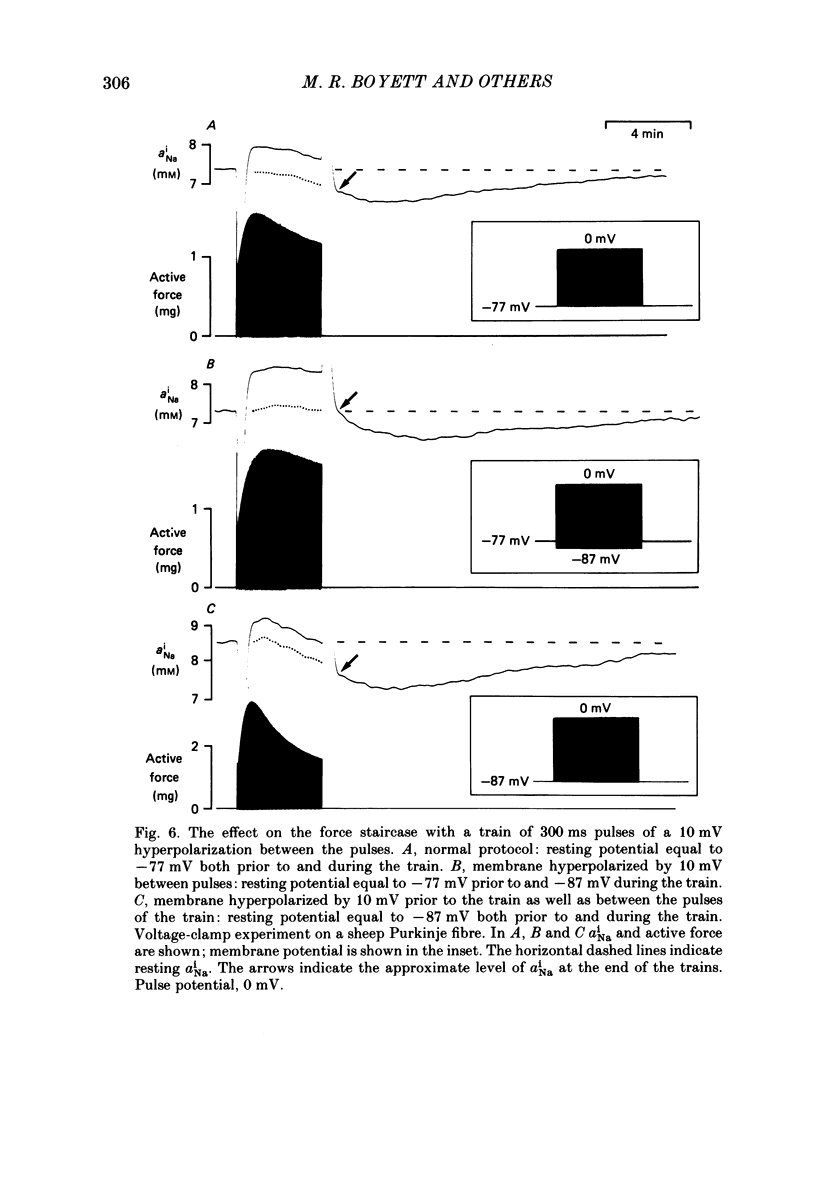
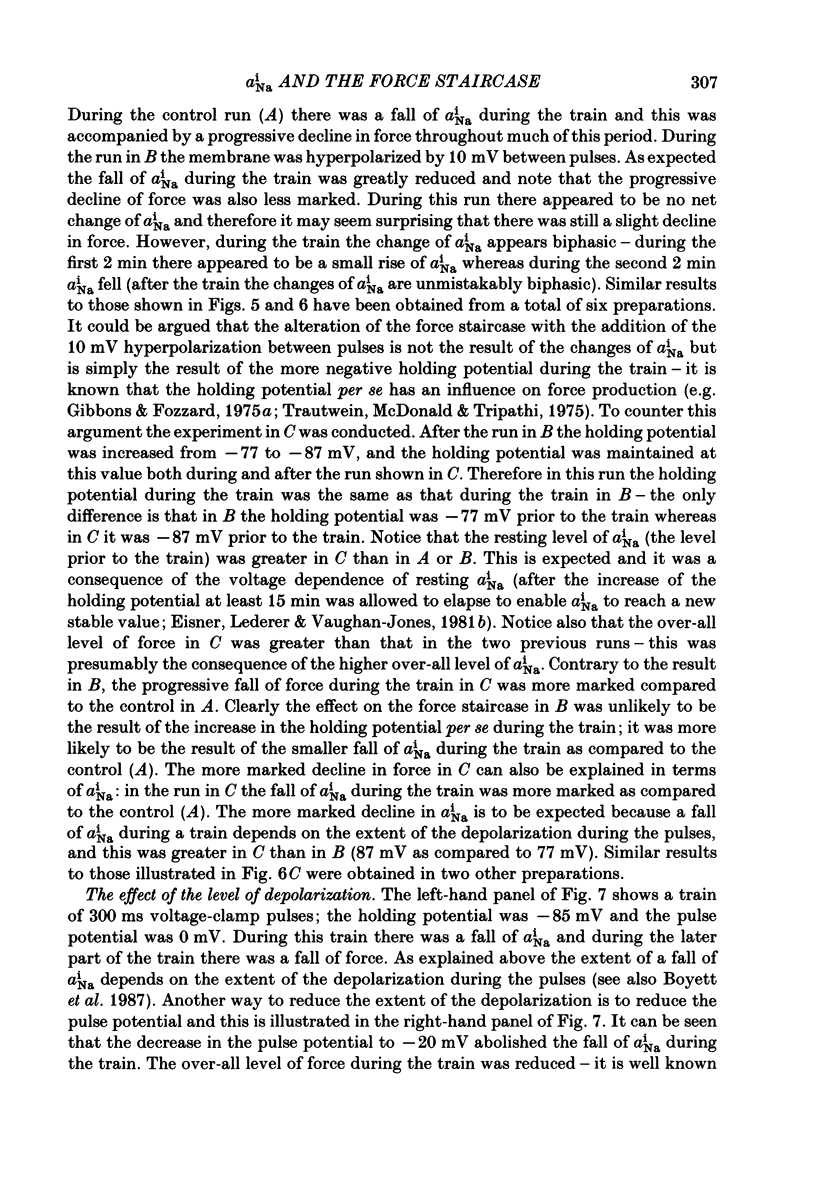
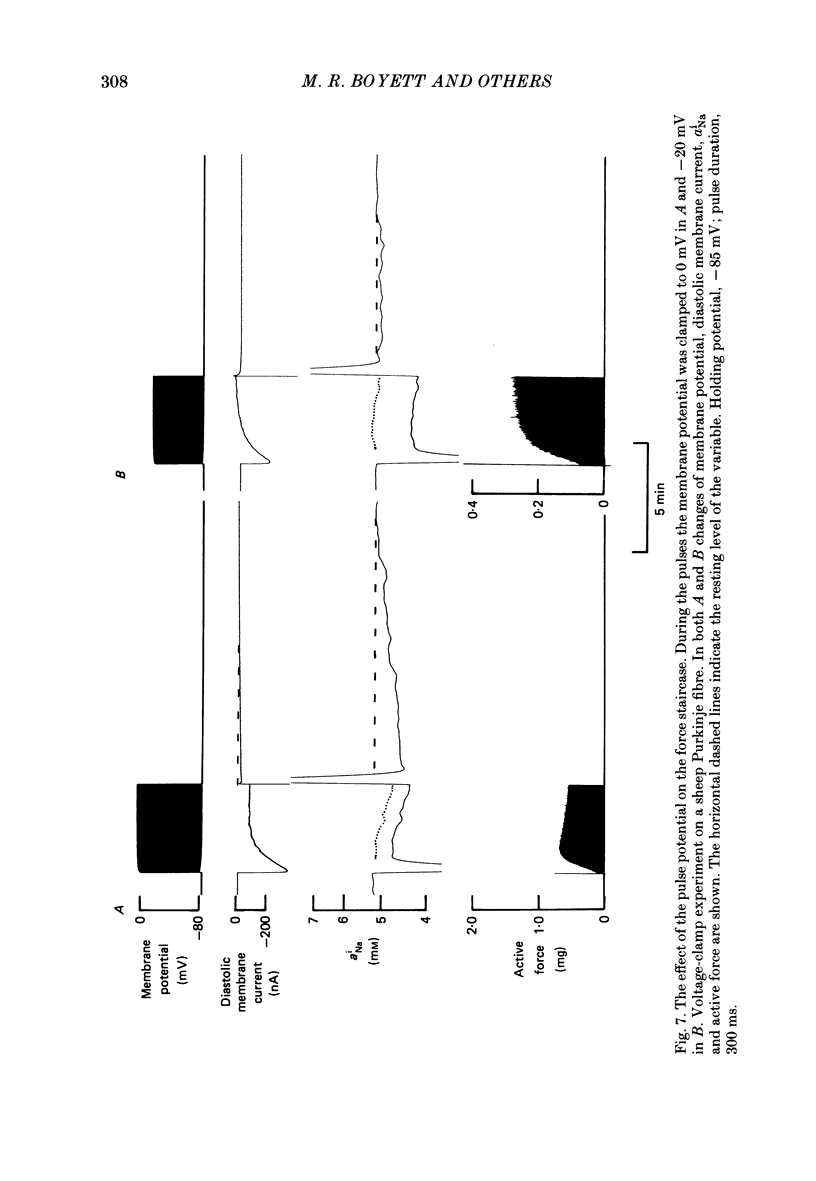
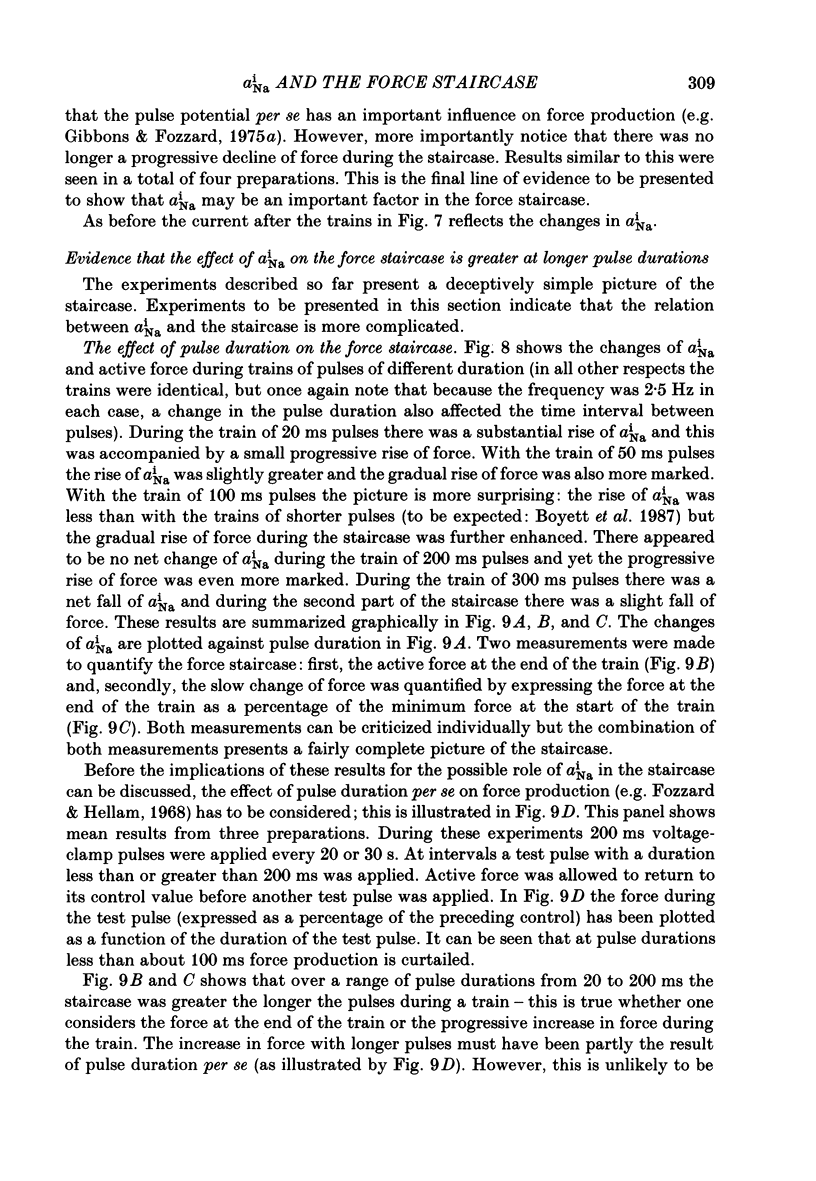
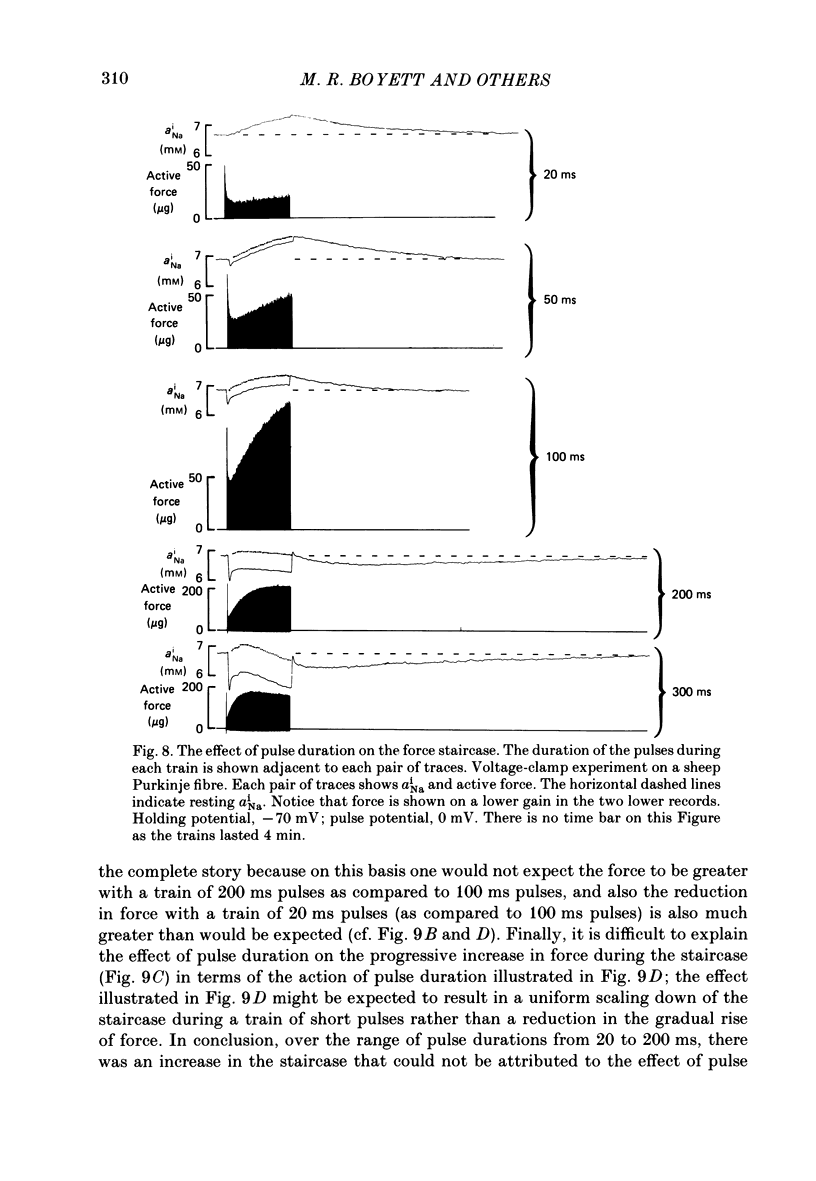
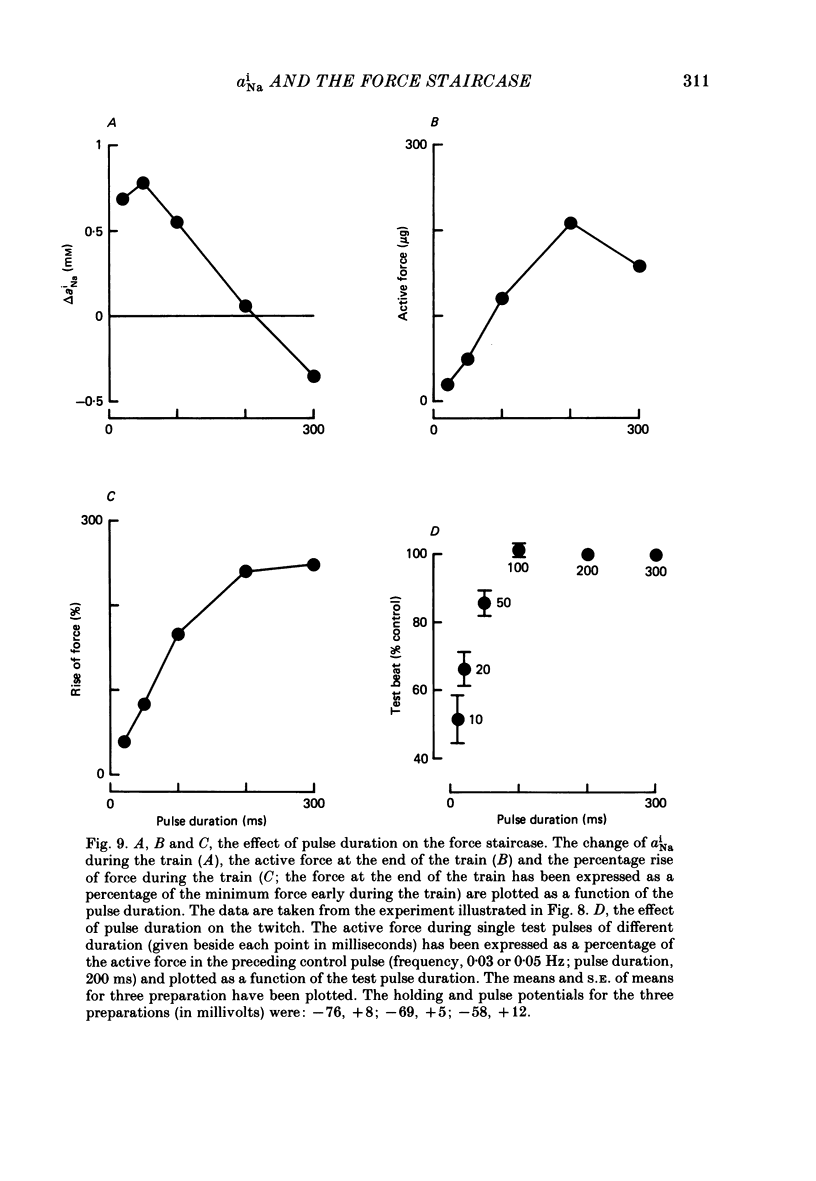
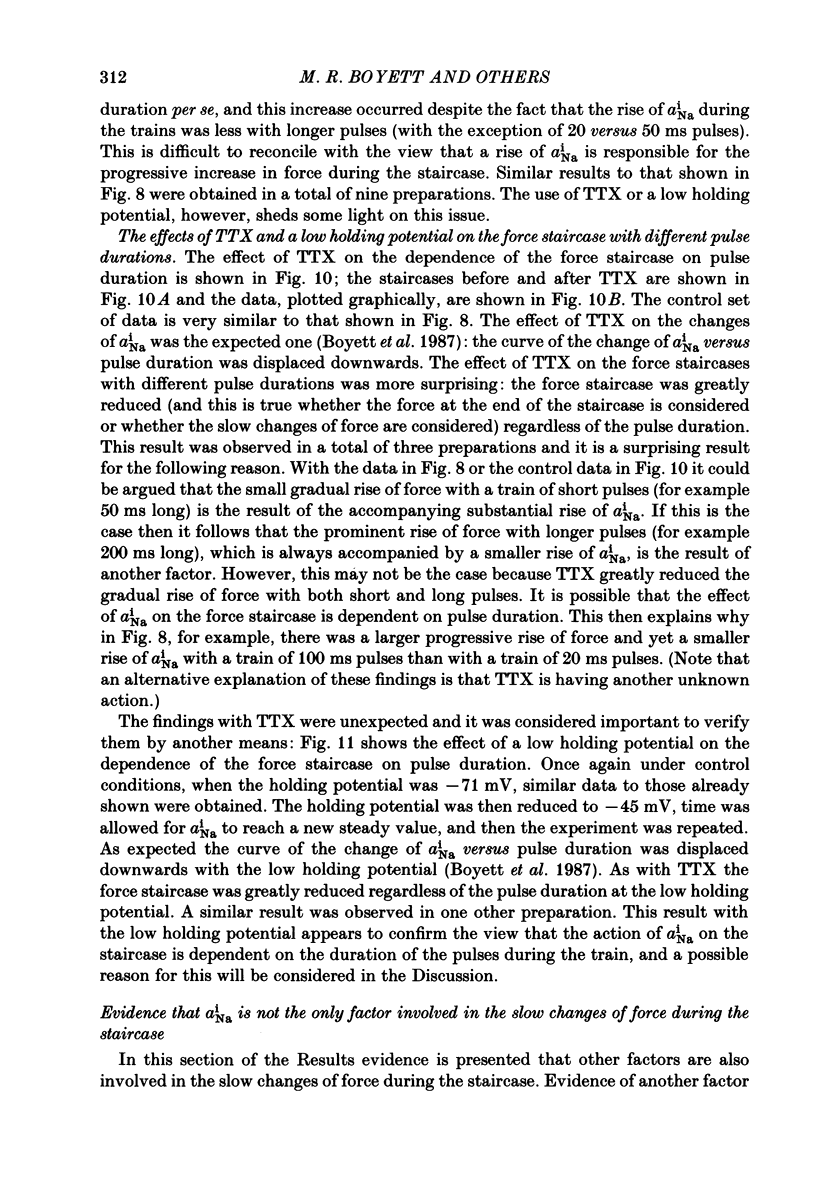
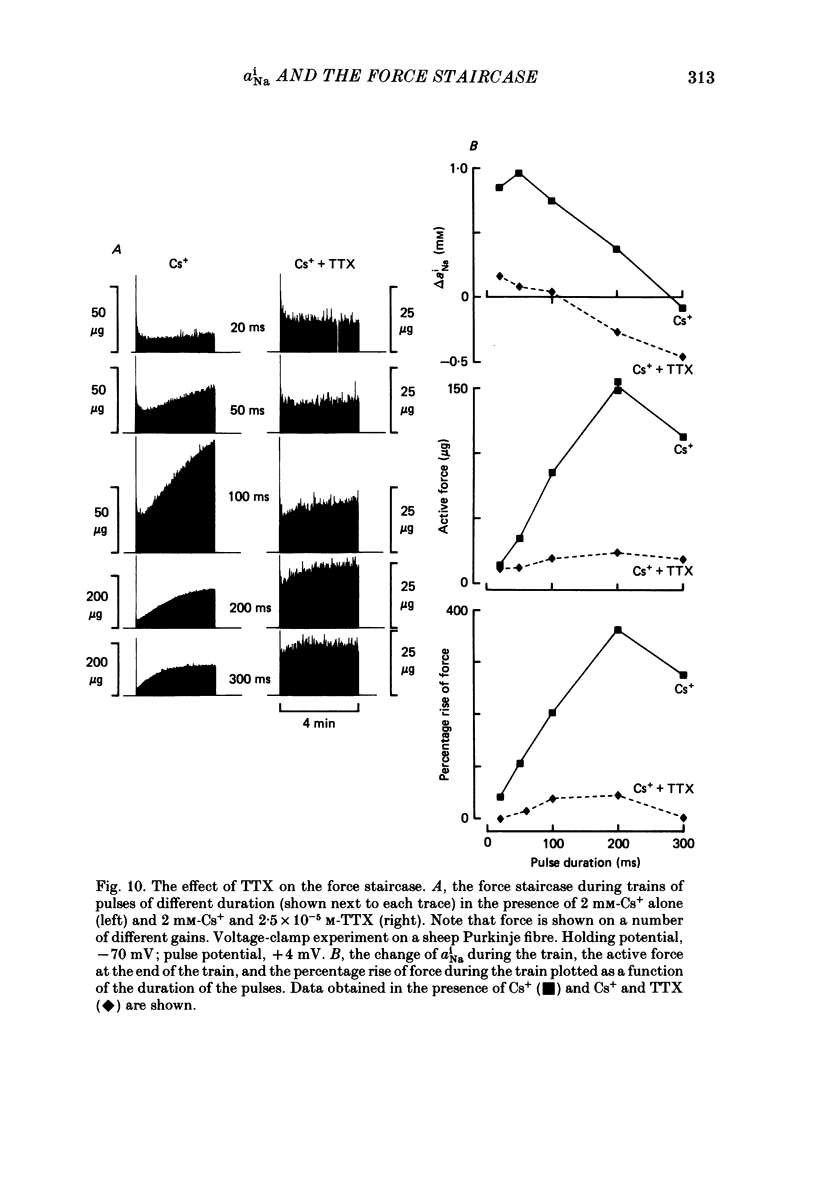
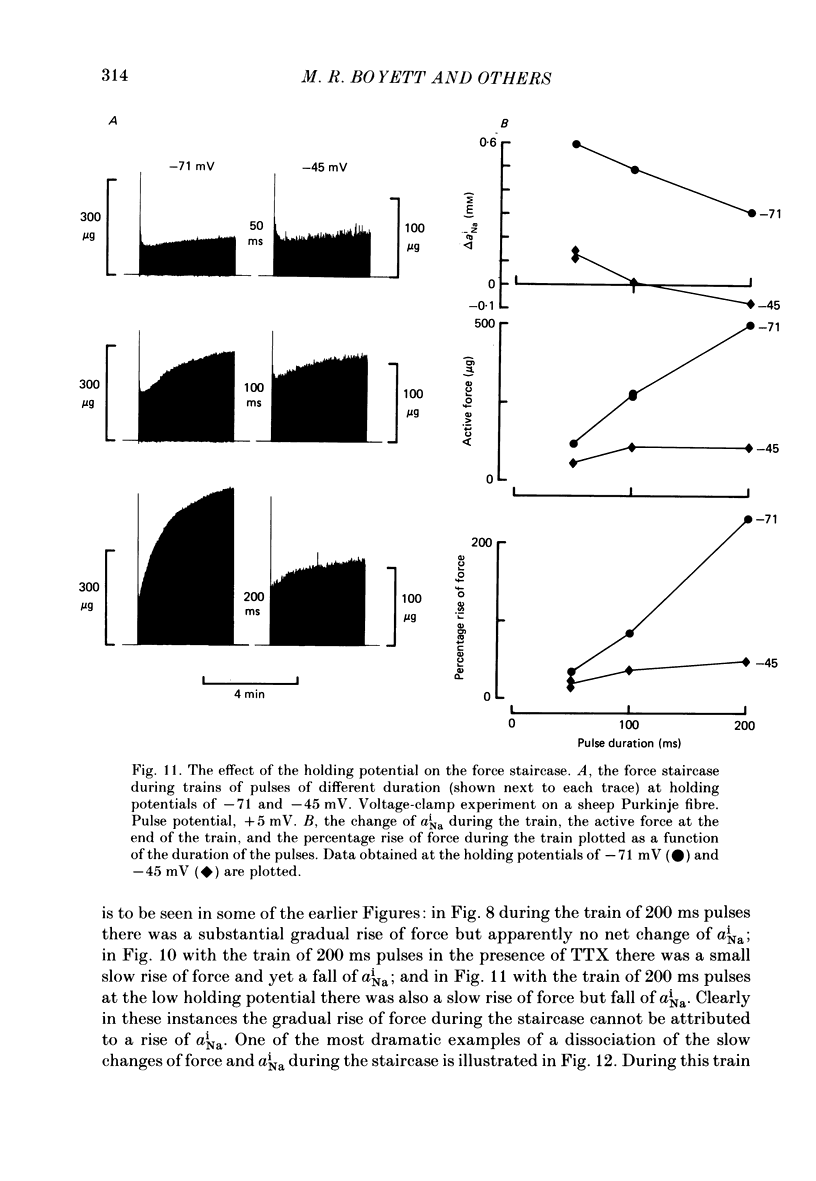
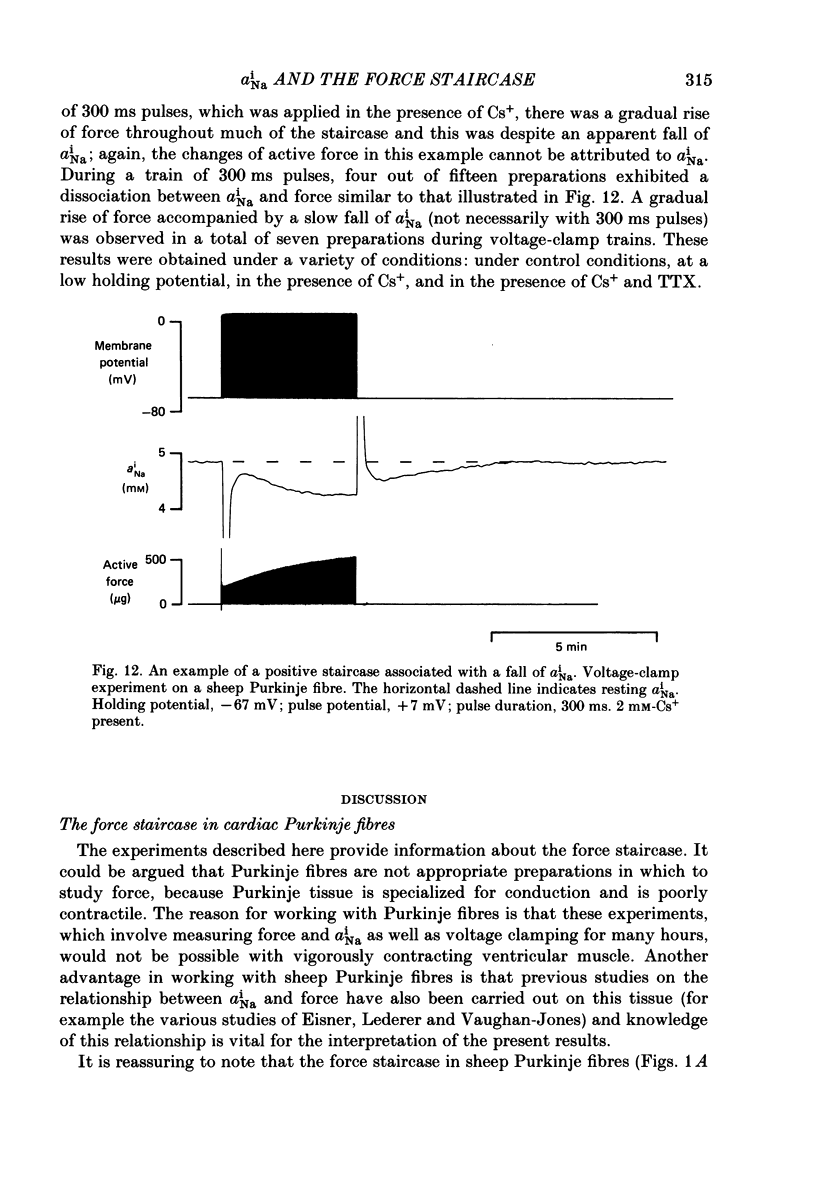
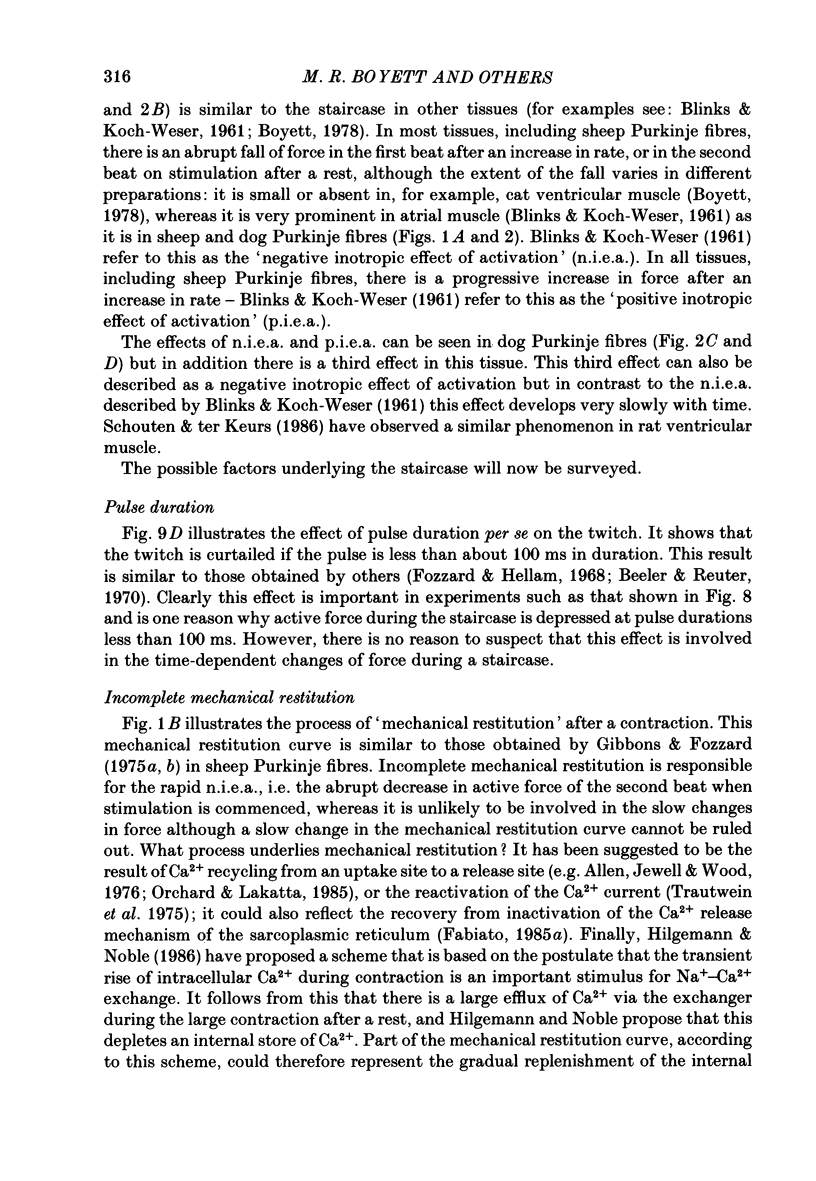
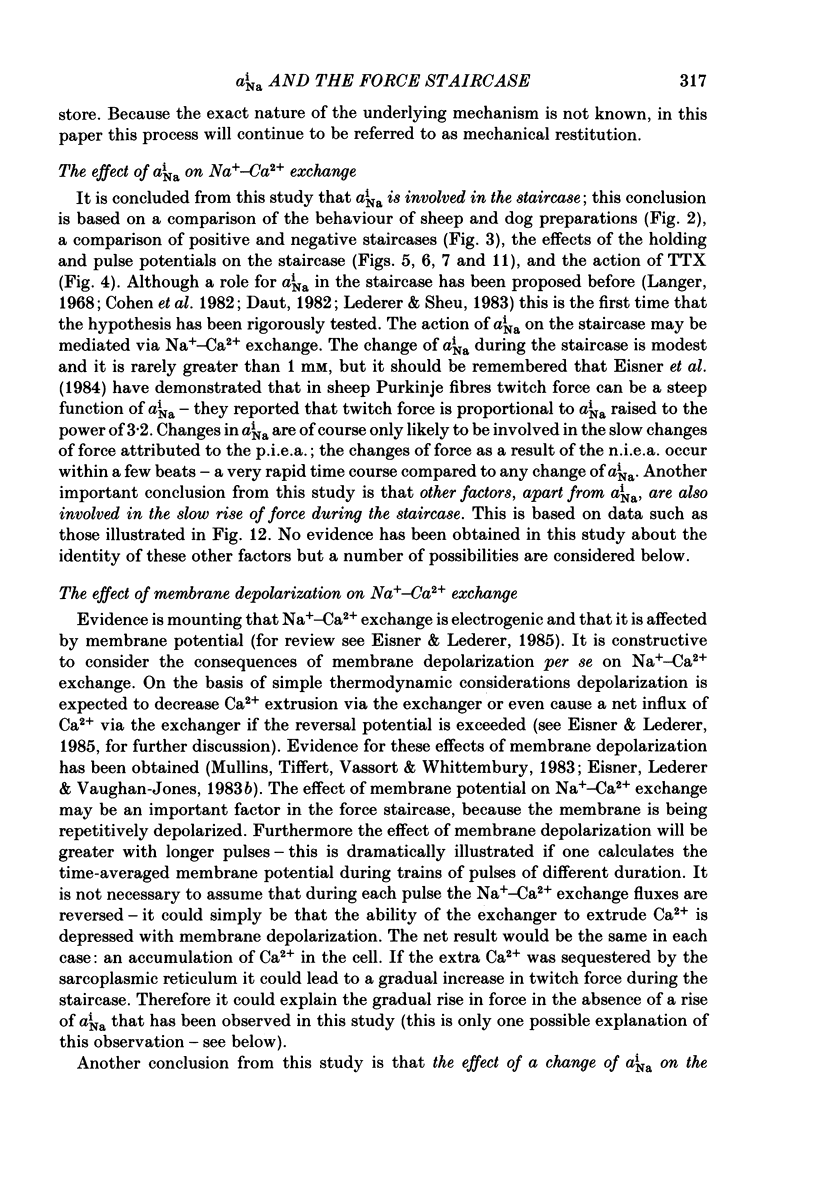
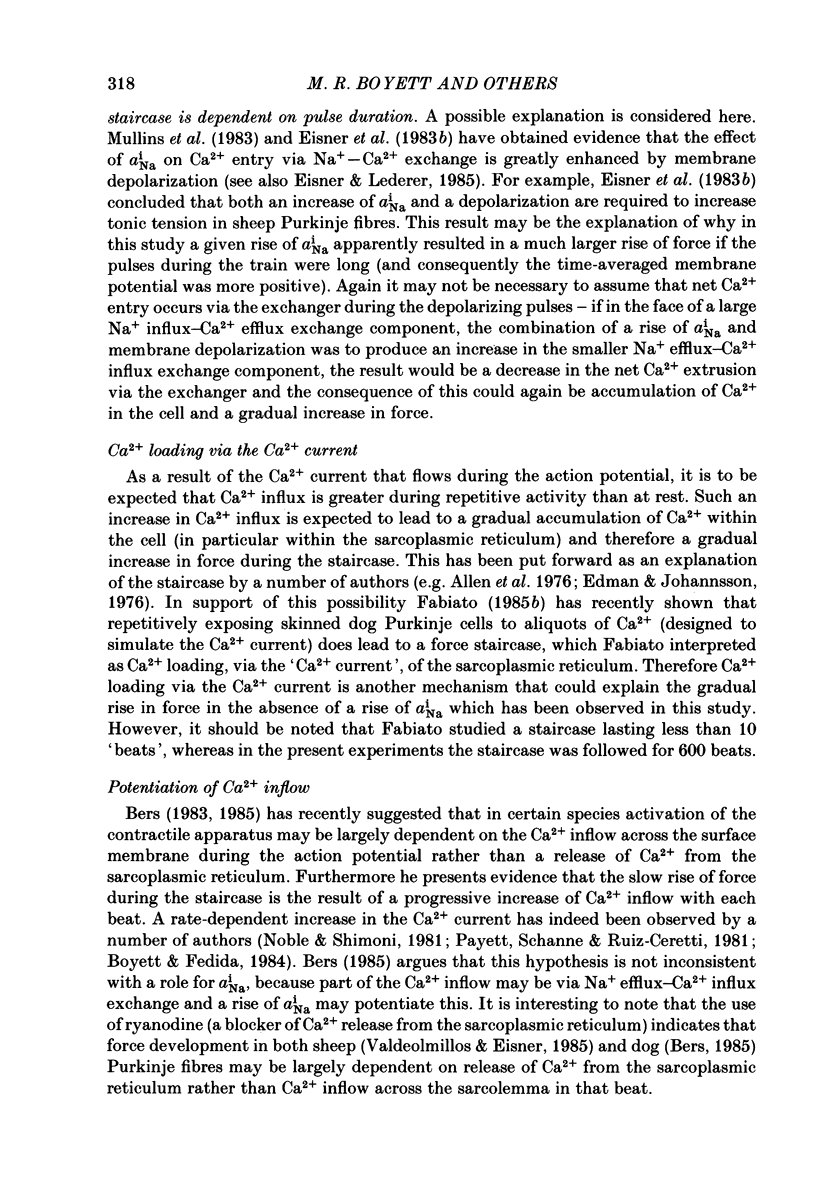
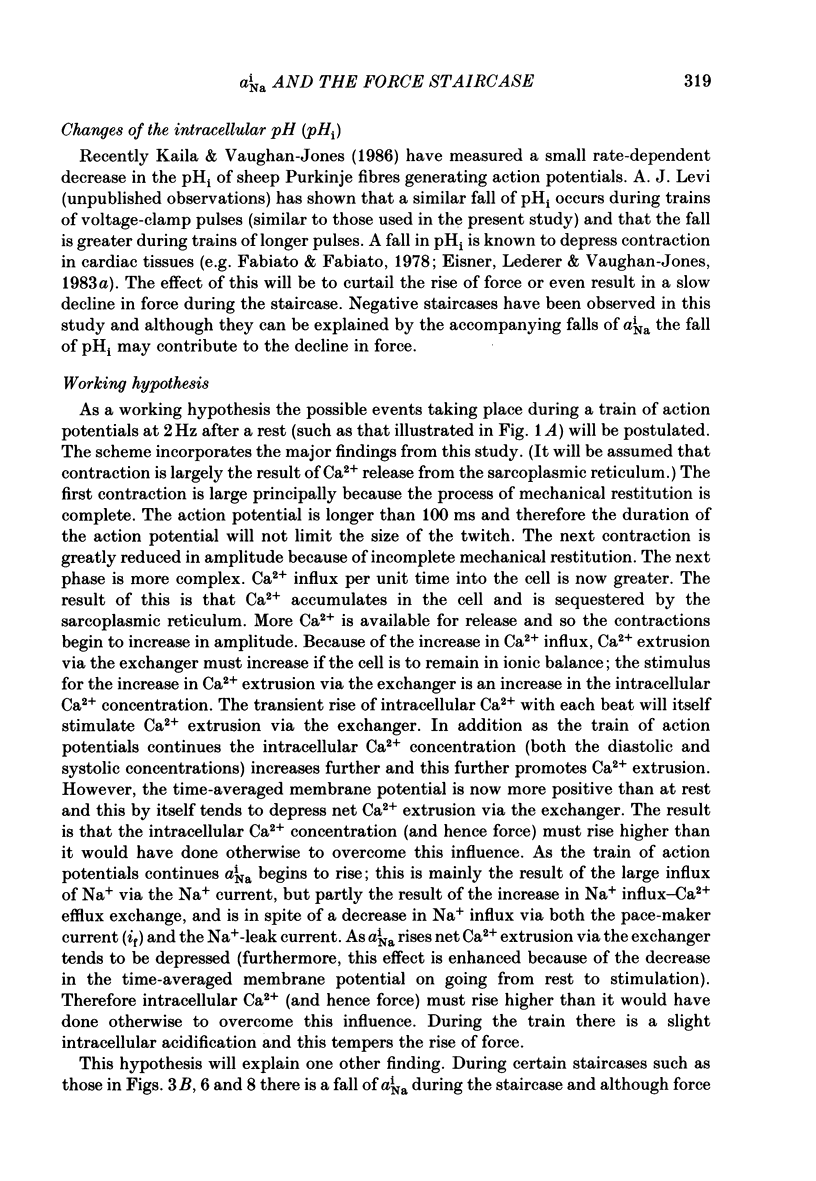
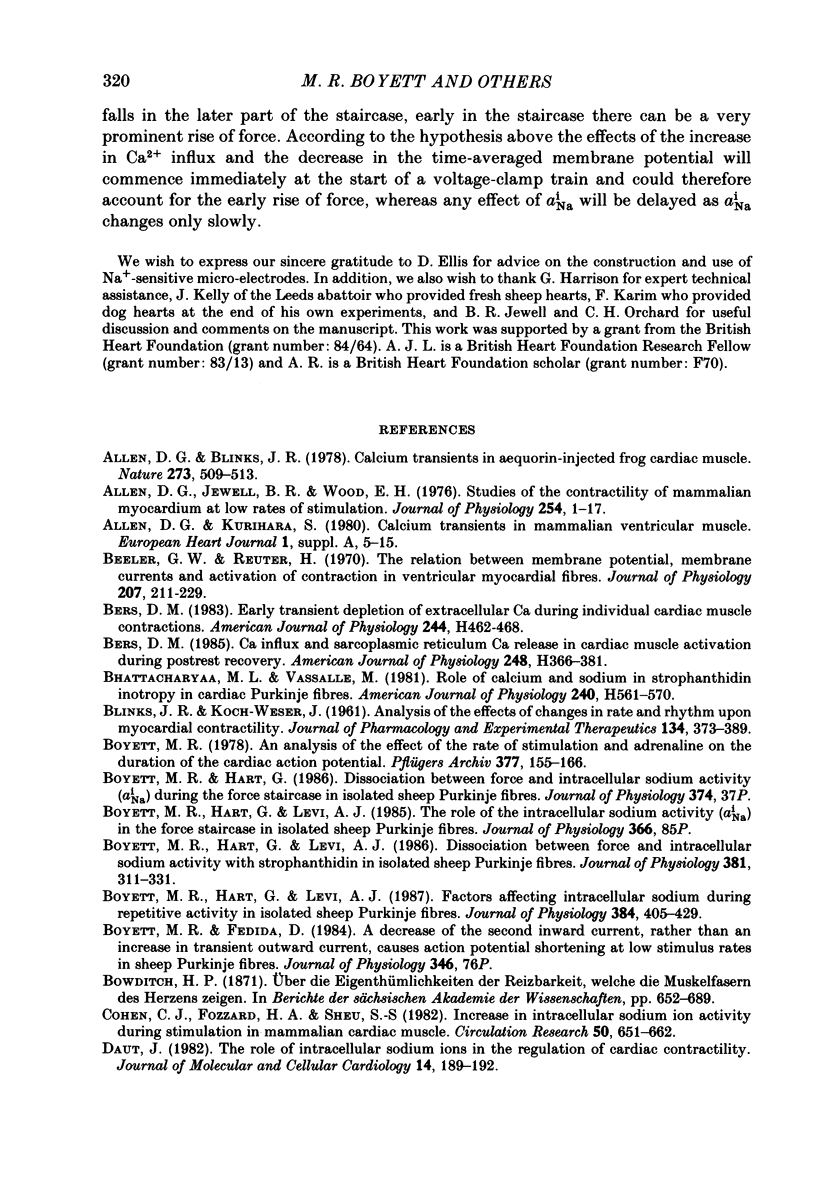
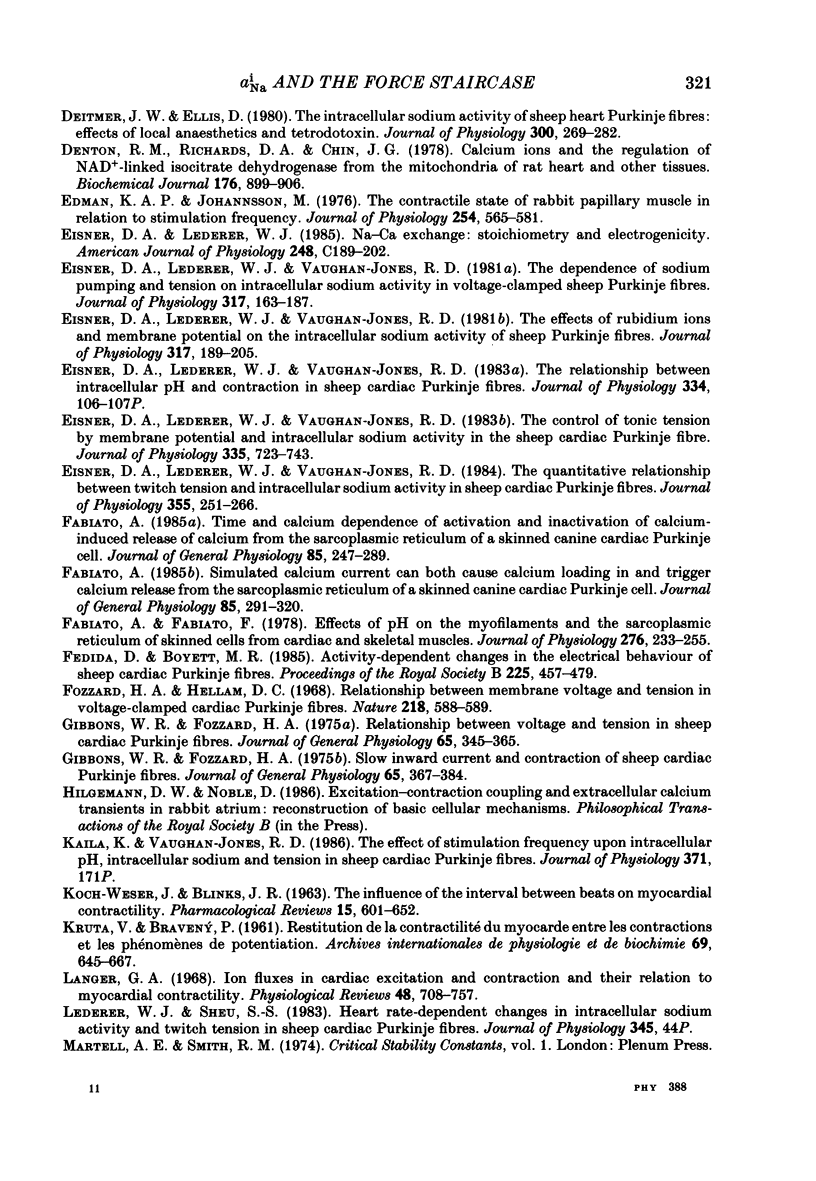
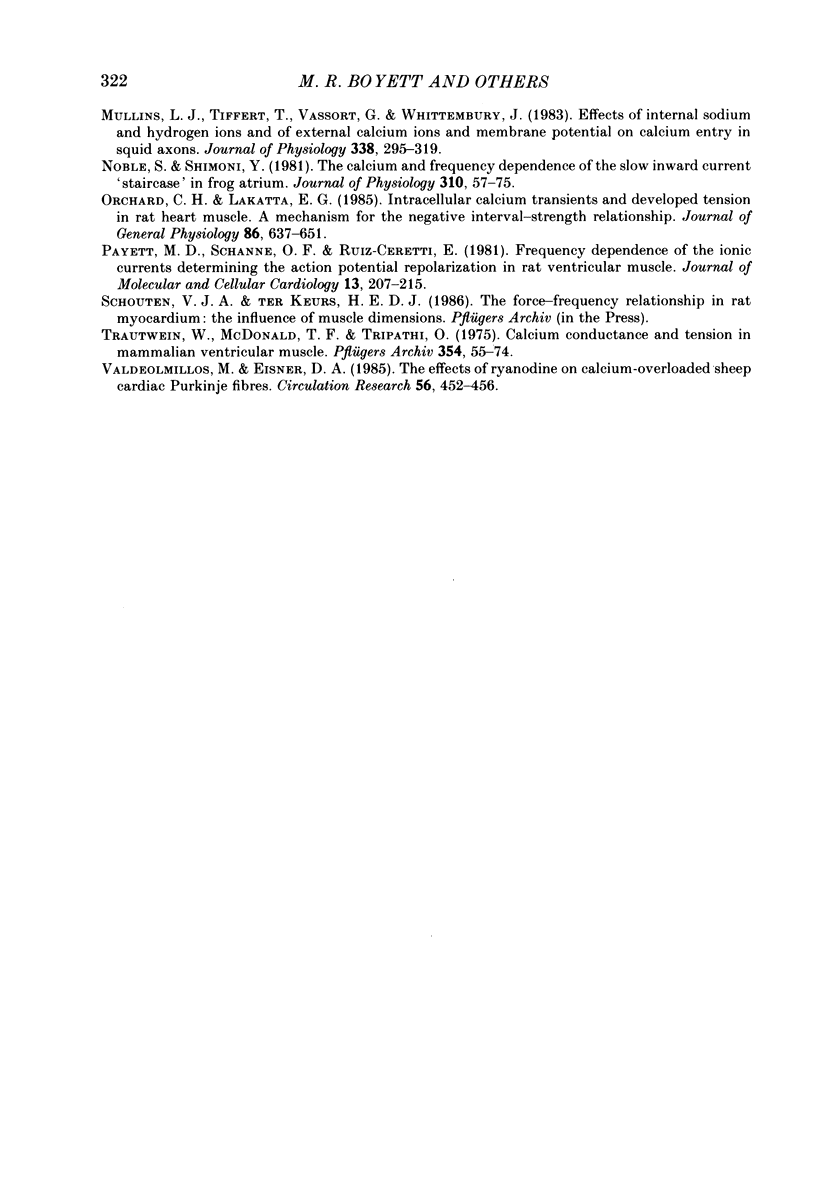
Selected References
These references are in PubMed. This may not be the complete list of references from this article.
- Allen D. G., Blinks J. R. Calcium transients in aequorin-injected frog cardiac muscle. Nature. 1978 Jun 15;273(5663):509–513. doi: 10.1038/273509a0. [DOI] [PubMed] [Google Scholar]
- Allen D. G., Jewell B. R., Wood E. H. Studies of the contractility of mammalian myocardium at low rates of stimulation. J Physiol. 1976 Jan;254(1):1–17. doi: 10.1113/jphysiol.1976.sp011217. [DOI] [PMC free article] [PubMed] [Google Scholar]
- Allen D. G., Kurihara S. Calcium transients in mammalian ventricular muscle. Eur Heart J. 1980;Suppl A:5–15. doi: 10.1093/eurheartj/1.suppl_1.5. [DOI] [PubMed] [Google Scholar]
- BLINKS J. R., KOCH-WESER J. Analysis of the effects of changes in rate and rhythm upon myocardial contractility. J Pharmacol Exp Ther. 1961 Dec;134:373–389. [PubMed] [Google Scholar]
- Beeler G. W., Jr, Reuter H. The relation between membrane potential, membrane currents and activation of contraction in ventricular myocardial fibres. J Physiol. 1970 Mar;207(1):211–229. doi: 10.1113/jphysiol.1970.sp009057. [DOI] [PMC free article] [PubMed] [Google Scholar]
- Bers D. M. Ca influx and sarcoplasmic reticulum Ca release in cardiac muscle activation during postrest recovery. Am J Physiol. 1985 Mar;248(3 Pt 2):H366–H381. doi: 10.1152/ajpheart.1985.248.3.H366. [DOI] [PubMed] [Google Scholar]
- Bers D. M. Early transient depletion of extracellular Ca during individual cardiac muscle contractions. Am J Physiol. 1983 Mar;244(3):H462–H468. doi: 10.1152/ajpheart.1983.244.3.H462. [DOI] [PubMed] [Google Scholar]
- Bhattacharyya M. L., Vassalle M. Role of calcium and sodium in strophanthidin inotropy in cardiac Purkinje fibers. Am J Physiol. 1981 Apr;240(4):H561–H570. doi: 10.1152/ajpheart.1981.240.4.H561. [DOI] [PubMed] [Google Scholar]
- Boyett M. R. An analysis of the effect of the rate of stimulation and adrenaline on the duration of the cardiac action potential. Pflugers Arch. 1978 Nov 14;377(2):155–166. doi: 10.1007/BF00582846. [DOI] [PubMed] [Google Scholar]
- Boyett M. R., Hart G., Levi A. J. Dissociation between force and intracellular sodium activity with strophanthidin in isolated sheep Purkinje fibres. J Physiol. 1986 Dec;381:311–331. doi: 10.1113/jphysiol.1986.sp016329. [DOI] [PMC free article] [PubMed] [Google Scholar]
- Boyett M. R., Hart G., Levi A. J. Factors affecting intracellular sodium during repetitive activity in isolated sheep Purkinje fibres. J Physiol. 1987 Mar;384:405–429. doi: 10.1113/jphysiol.1987.sp016461. [DOI] [PMC free article] [PubMed] [Google Scholar]
- Cohen C. J., Fozzard H. A., Sheu S. S. Increase in intracellular sodium ion activity during stimulation in mammalian cardiac muscle. Circ Res. 1982 May;50(5):651–662. doi: 10.1161/01.res.50.5.651. [DOI] [PubMed] [Google Scholar]
- Daut J. The role of intracellular sodium ions in the regulation of cardiac contractility. J Mol Cell Cardiol. 1982 Mar;14(3):189–192. doi: 10.1016/0022-2828(82)90119-5. [DOI] [PubMed] [Google Scholar]
- Deitmer J. W., Ellis D. The intracellular sodium activity of sheep heart Purkinje fibres: effects of local anaesthetics and tetrodotoxin. J Physiol. 1980 Mar;300:269–282. doi: 10.1113/jphysiol.1980.sp013161. [DOI] [PMC free article] [PubMed] [Google Scholar]
- Denton R. M., Richards D. A., Chin J. G. Calcium ions and the regulation of NAD+-linked isocitrate dehydrogenase from the mitochondria of rat heart and other tissues. Biochem J. 1978 Dec 15;176(3):899–906. doi: 10.1042/bj1760899. [DOI] [PMC free article] [PubMed] [Google Scholar]
- Edman K. A., Jóhannsson M. The contractile state of rabbit papillary muscle in relation to stimulation frequency. J Physiol. 1976 Jan;254(3):565–581. doi: 10.1113/jphysiol.1976.sp011247. [DOI] [PMC free article] [PubMed] [Google Scholar]
- Eisner D. A., Lederer W. J. Na-Ca exchange: stoichiometry and electrogenicity. Am J Physiol. 1985 Mar;248(3 Pt 1):C189–C202. doi: 10.1152/ajpcell.1985.248.3.C189. [DOI] [PubMed] [Google Scholar]
- Eisner D. A., Lederer W. J., Vaughan-Jones R. D. The control of tonic tension by membrane potential and intracellular sodium activity in the sheep cardiac Purkinje fibre. J Physiol. 1983 Feb;335:723–743. doi: 10.1113/jphysiol.1983.sp014560. [DOI] [PMC free article] [PubMed] [Google Scholar]
- Eisner D. A., Lederer W. J., Vaughan-Jones R. D. The dependence of sodium pumping and tension on intracellular sodium activity in voltage-clamped sheep Purkinje fibres. J Physiol. 1981 Aug;317:163–187. doi: 10.1113/jphysiol.1981.sp013819. [DOI] [PMC free article] [PubMed] [Google Scholar]
- Eisner D. A., Lederer W. J., Vaughan-Jones R. D. The effects of rubidium ions and membrane potentials on the intracellular sodium activity of sheep Purkinje fibres. J Physiol. 1981 Aug;317:189–205. doi: 10.1113/jphysiol.1981.sp013820. [DOI] [PMC free article] [PubMed] [Google Scholar]
- Eisner D. A., Lederer W. J., Vaughan-Jones R. D. The quantitative relationship between twitch tension and intracellular sodium activity in sheep cardiac Purkinje fibres. J Physiol. 1984 Oct;355:251–266. doi: 10.1113/jphysiol.1984.sp015417. [DOI] [PMC free article] [PubMed] [Google Scholar]
- Fabiato A., Fabiato F. Effects of pH on the myofilaments and the sarcoplasmic reticulum of skinned cells from cardiace and skeletal muscles. J Physiol. 1978 Mar;276:233–255. doi: 10.1113/jphysiol.1978.sp012231. [DOI] [PMC free article] [PubMed] [Google Scholar]
- Fabiato A. Simulated calcium current can both cause calcium loading in and trigger calcium release from the sarcoplasmic reticulum of a skinned canine cardiac Purkinje cell. J Gen Physiol. 1985 Feb;85(2):291–320. doi: 10.1085/jgp.85.2.291. [DOI] [PMC free article] [PubMed] [Google Scholar]
- Fabiato A. Time and calcium dependence of activation and inactivation of calcium-induced release of calcium from the sarcoplasmic reticulum of a skinned canine cardiac Purkinje cell. J Gen Physiol. 1985 Feb;85(2):247–289. doi: 10.1085/jgp.85.2.247. [DOI] [PMC free article] [PubMed] [Google Scholar]
- Fozzard H. A., Hellman D. C. Relationship between membrane voltage and tension in voltage-clamped cardiac purkinje fibres. Nature. 1968 May 11;218(5141):588–589. doi: 10.1038/218588a0. [DOI] [PubMed] [Google Scholar]
- Gibbons W. R., Fozzard H. A. Relationships between voltage and tension in sheep cardiac Purkinje fibers. J Gen Physiol. 1975 Mar;65(3):345–365. doi: 10.1085/jgp.65.3.345. [DOI] [PMC free article] [PubMed] [Google Scholar]
- Gibbons W. R., Fozzard H. A. Slow inward current and contraction of sheep cardiac Purkinje fibers. J Gen Physiol. 1975 Mar;65(3):367–384. doi: 10.1085/jgp.65.3.367. [DOI] [PMC free article] [PubMed] [Google Scholar]
- KOCH-WESER J., BLINKS J. R. THE INFLUENCE OF THE INTERVAL BETWEEN BEATS ON MYOCARDIAL CONTRACTILITY. Pharmacol Rev. 1963 Sep;15:601–652. [PubMed] [Google Scholar]
- KRUTA V., BRAVENY P. [Restoration of myocardial contractility between contractions and potentiation phenomena]. Arch Int Physiol Biochim. 1961 Dec;69:645–667. doi: 10.3109/13813456109092827. [DOI] [PubMed] [Google Scholar]
- Langer G. A. Ion fluxes in cardiac excitation and contraction and their relation to myocardial contractility. Physiol Rev. 1968 Oct;48(4):708–757. doi: 10.1152/physrev.1968.48.4.708. [DOI] [PubMed] [Google Scholar]
- Mullins L. J., Tiffert T., Vassort G., Whittembury J. Effects of internal sodium and hydrogen ions and of external calcium ions and membrane potential on calcium entry in squid axons. J Physiol. 1983 May;338:295–319. doi: 10.1113/jphysiol.1983.sp014674. [DOI] [PMC free article] [PubMed] [Google Scholar]
- Noble S., Shimoni Y. The calcium and frequency dependence of the slow inward current 'staircase' in frog atrium. J Physiol. 1981 Jan;310:57–75. doi: 10.1113/jphysiol.1981.sp013537. [DOI] [PMC free article] [PubMed] [Google Scholar]
- Orchard C. H., Lakatta E. G. Intracellular calcium transients and developed tension in rat heart muscle. A mechanism for the negative interval-strength relationship. J Gen Physiol. 1985 Nov;86(5):637–651. doi: 10.1085/jgp.86.5.637. [DOI] [PMC free article] [PubMed] [Google Scholar]
- Payet M. D., Schanne O. F., Ruiz-Ceretti E. Frequency dependence of the ionic currents determining the action potential repolarization in rat ventricular muscle. J Mol Cell Cardiol. 1981 Feb;13(2):207–215. doi: 10.1016/0022-2828(81)90217-0. [DOI] [PubMed] [Google Scholar]
- Trautwein W., McDonald T. F., Tripathi O. Calcium conductance and tension in mammalian ventricular muscle. Pflugers Arch. 1975;354(1):55–74. doi: 10.1007/BF00584503. [DOI] [PubMed] [Google Scholar]
- Valdeolmillos M., Eisner D. A. The effects of ryanodine on calcium-overloaded sheep cardiac Purkinje fibers. Circ Res. 1985 Mar;56(3):452–456. doi: 10.1161/01.res.56.3.452. [DOI] [PubMed] [Google Scholar]