Abstract
Intracellular pressure responses were recorded from squid giant axons after the axoplasm was removed by the intracellular perfusion technique. A glass tube was inserted into the axon and the movement of the air-water interface formed on the end of the tube was observed with a Y-shaped fibrescope. The intracellular pressure increased and decreased rapidly when an action potential was induced by electrical stimulation. The amplitude of the response was about 10 mPa (or 1 X 10(-3) mmH2O), which was very large in comparison with that observed in unperfused axons. It was sensitive to extracellular Ca2+. The pressure response appeared in an all-or-none manner and could be suppressed by tetrodotoxin. This excluded physicochemical processes on the stimulating electrode or current-supplying electrode as sources of the response. Various other sources of artifacts were also excluded. An extensive removal of the axoplasm by intracellular perfusion with a protease-containing solution and a KCl solution did not eliminate the pressure response. The intracellular pressure was membrane potential dependent, increasing upon depolarization and decreasing upon hyperpolarization of the membrane. Under voltage clamp, the relationship between the membrane potential and the pressure response was parabolic with a maximum at +109 mV (in reference to the resting level). The response did not depend on the membrane current. A much slower response due to electro-osmotic water flow was also detected. The pressure response induced by hyperpolarization of the membrane was suppressed by extracellular application of a lidocaine-containing solution, but not by a tetrodotoxin-containing solution. These results suggest that the pressure responses arise either from a change in electrostriction across the axolemma or from a change in charge-dependent tension along the axolemma.
Full text
PDF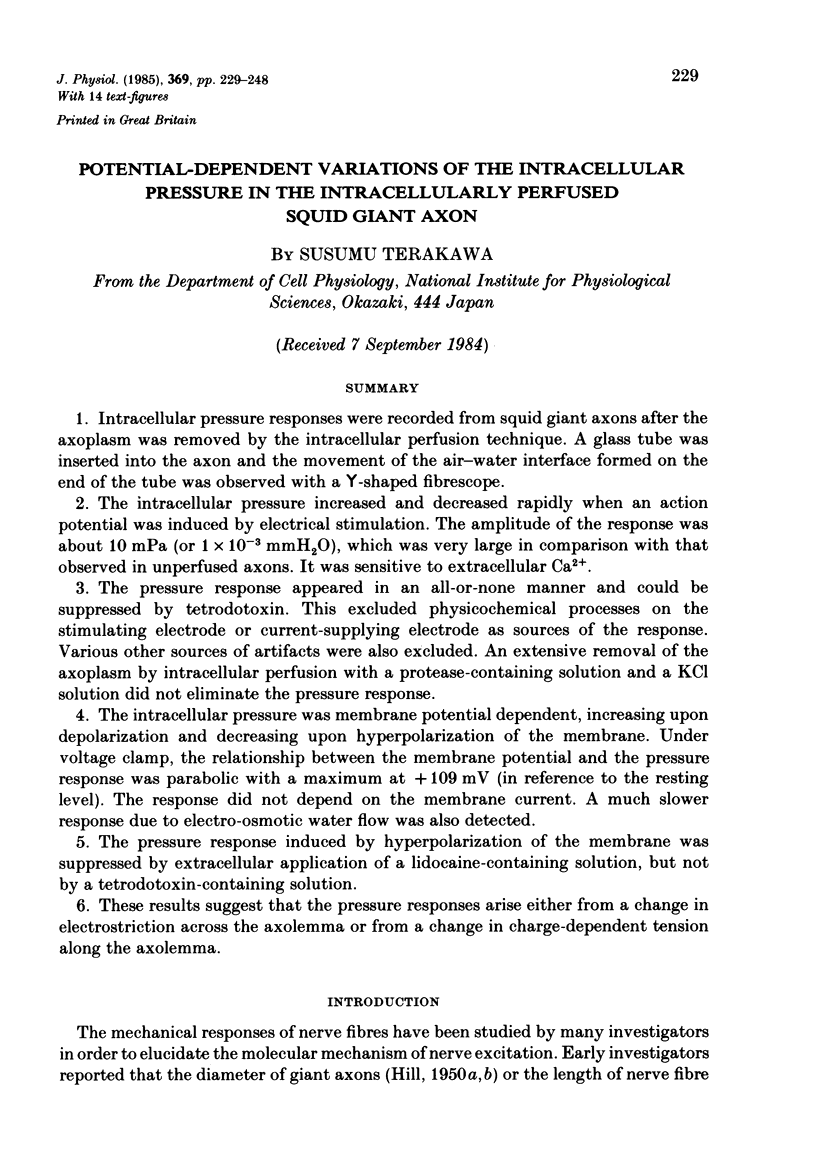
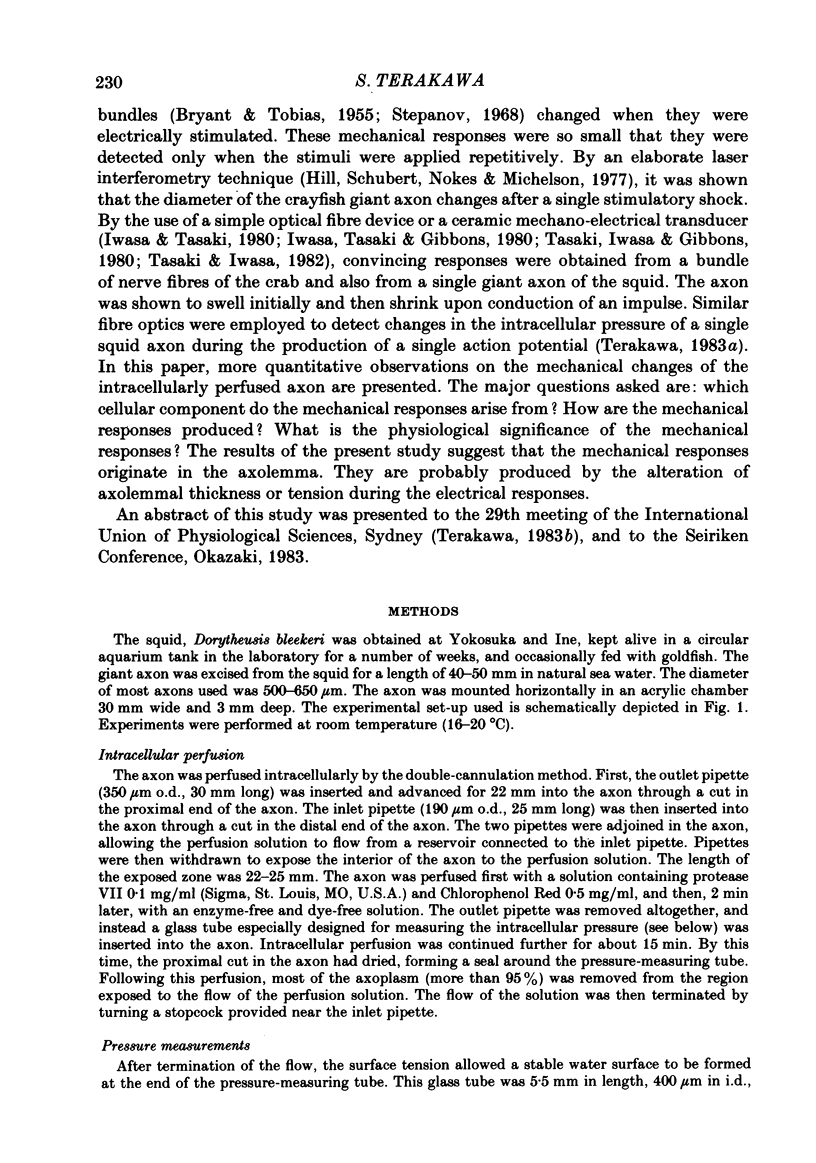
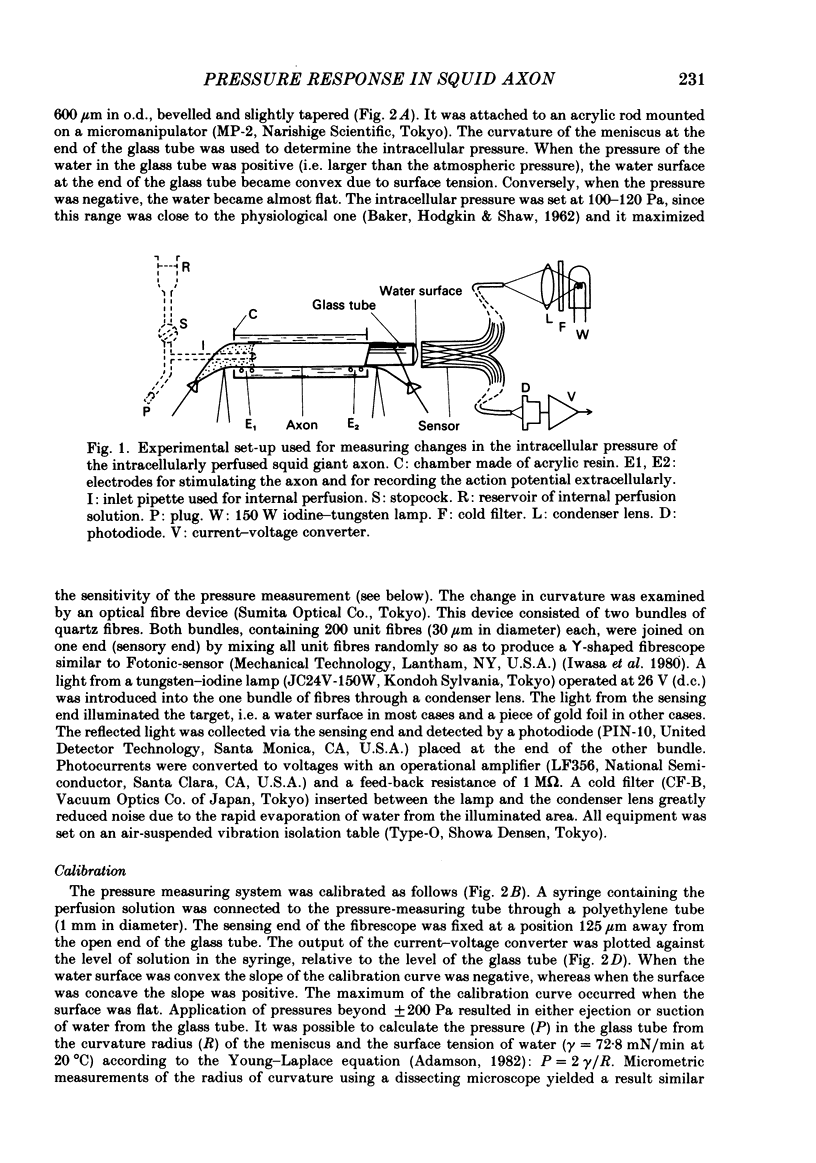
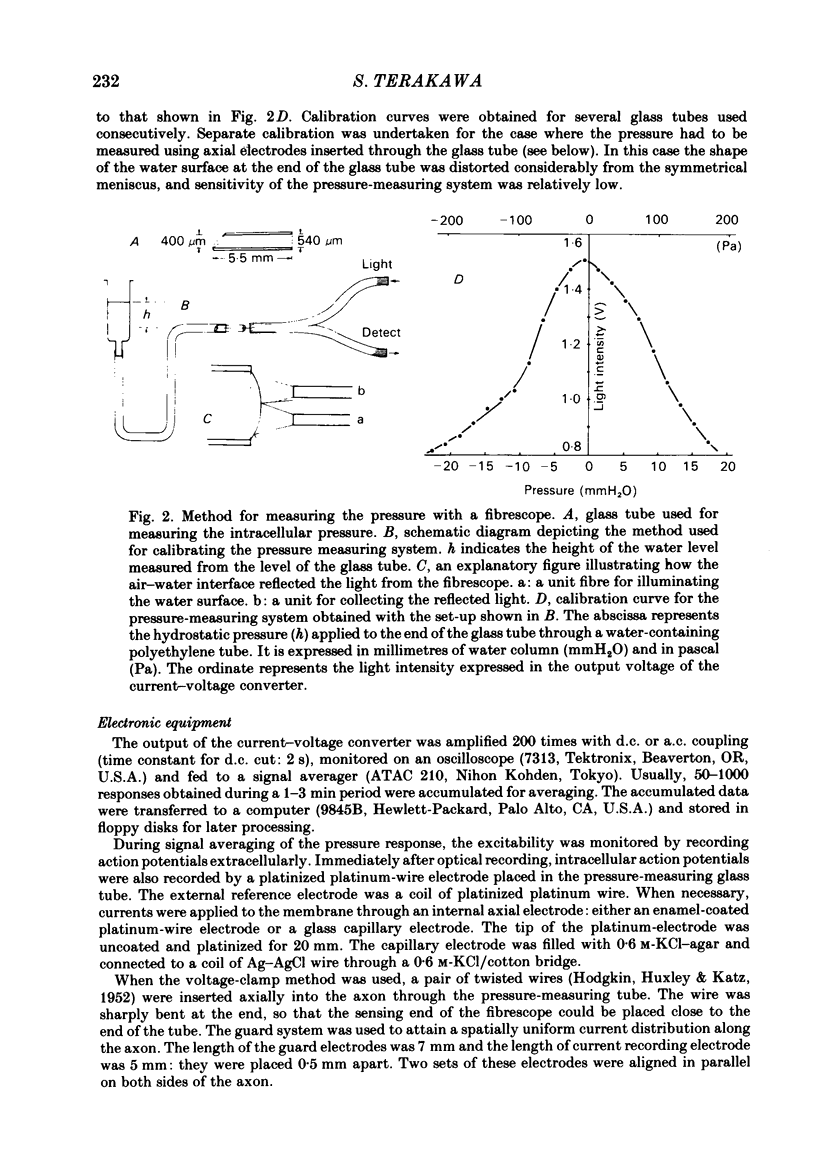
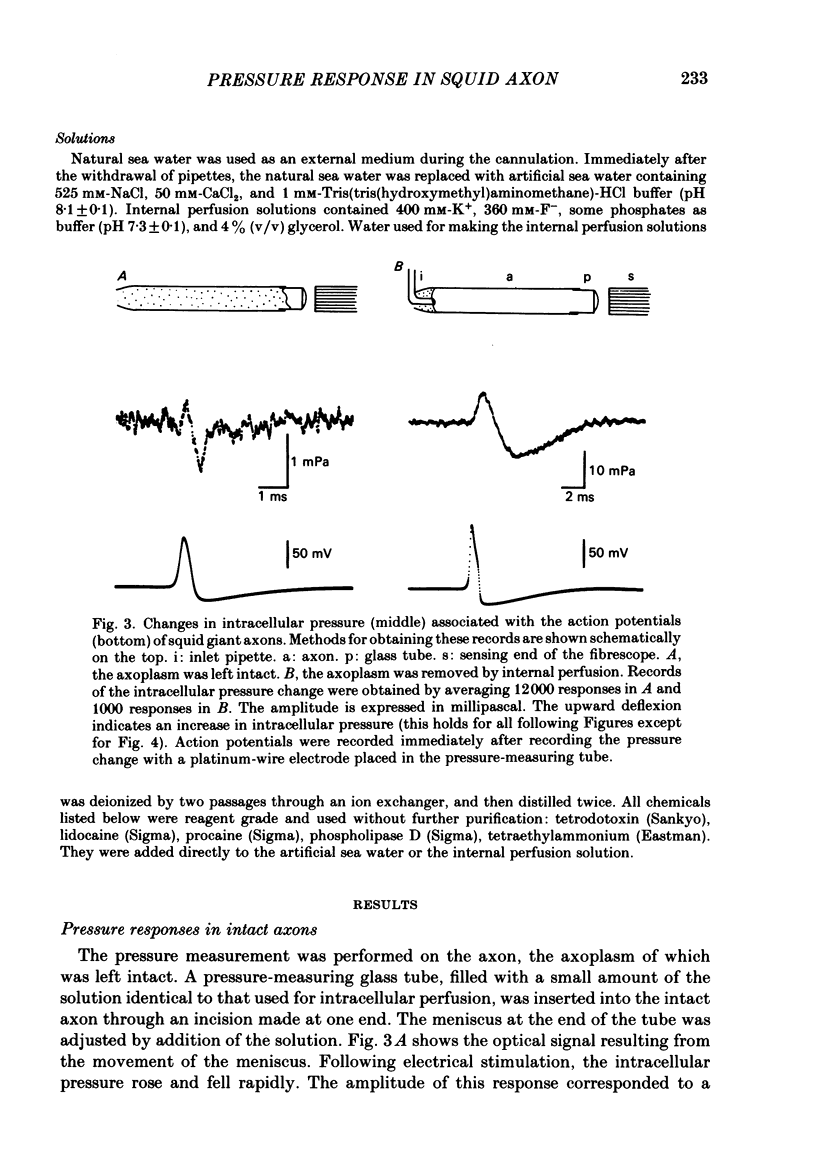
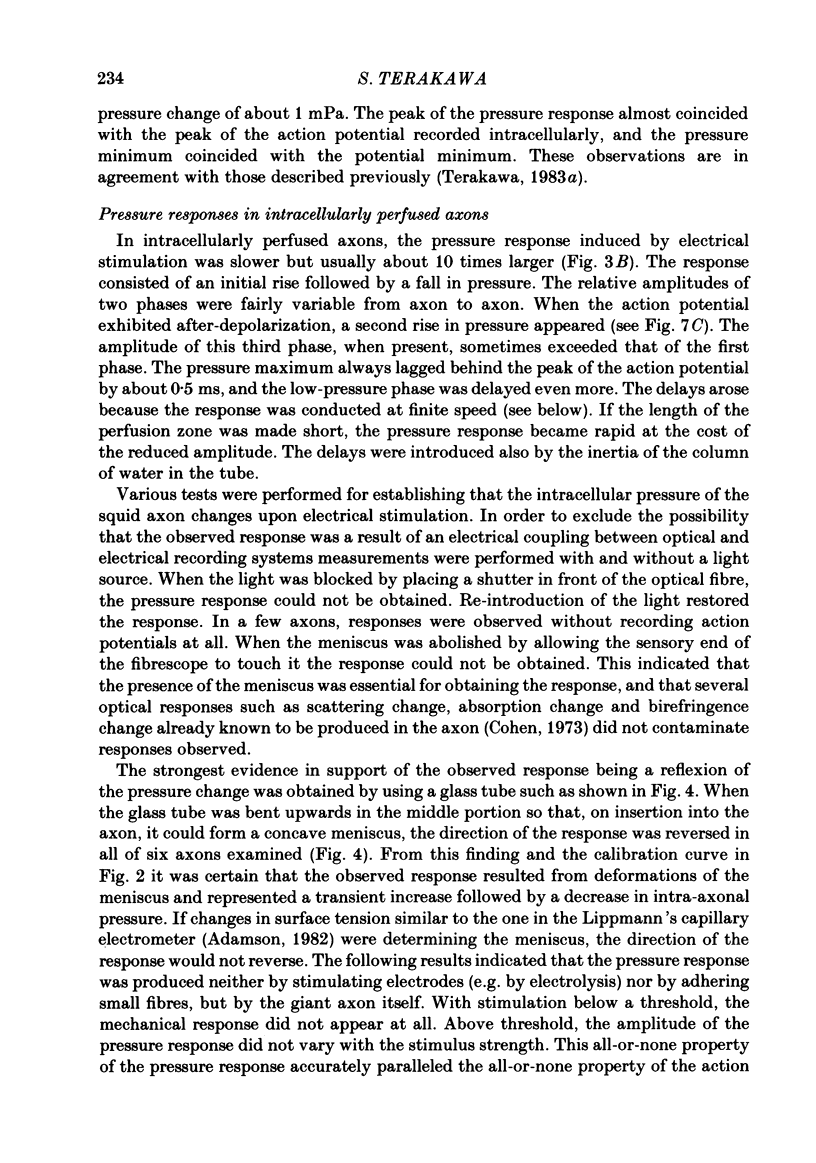
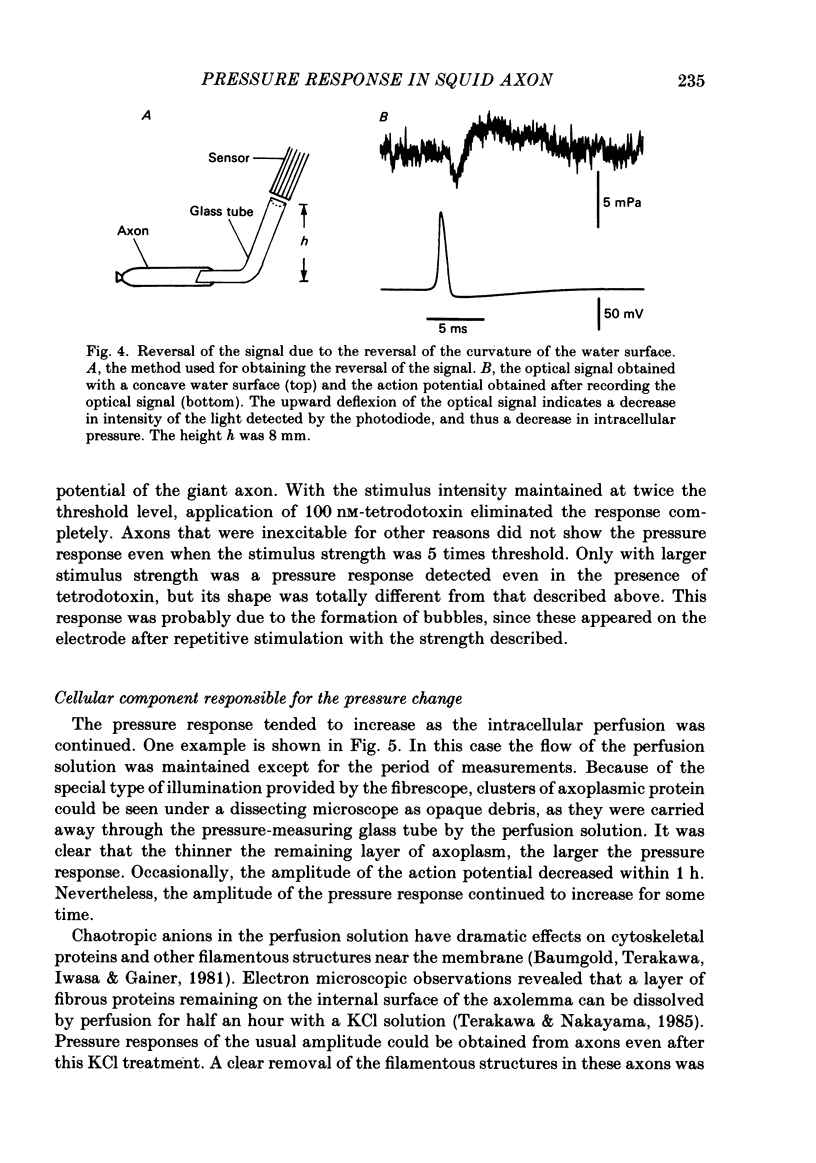
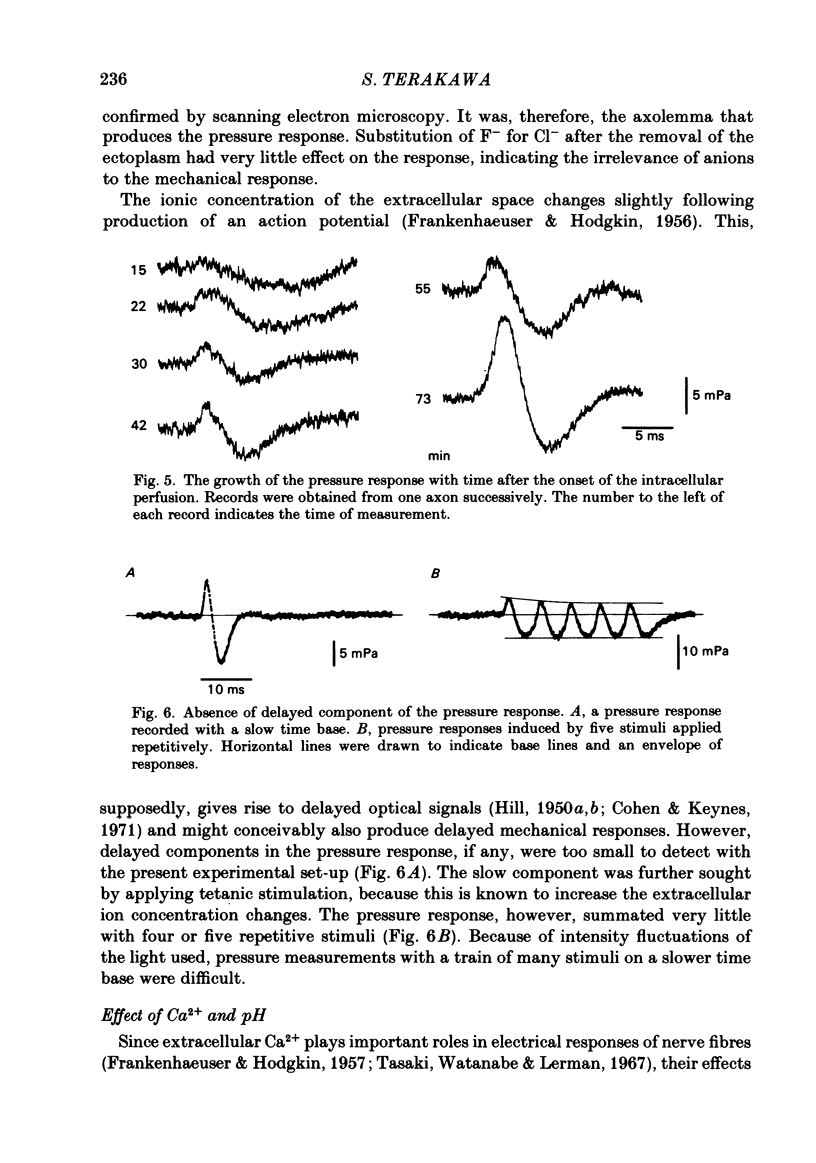
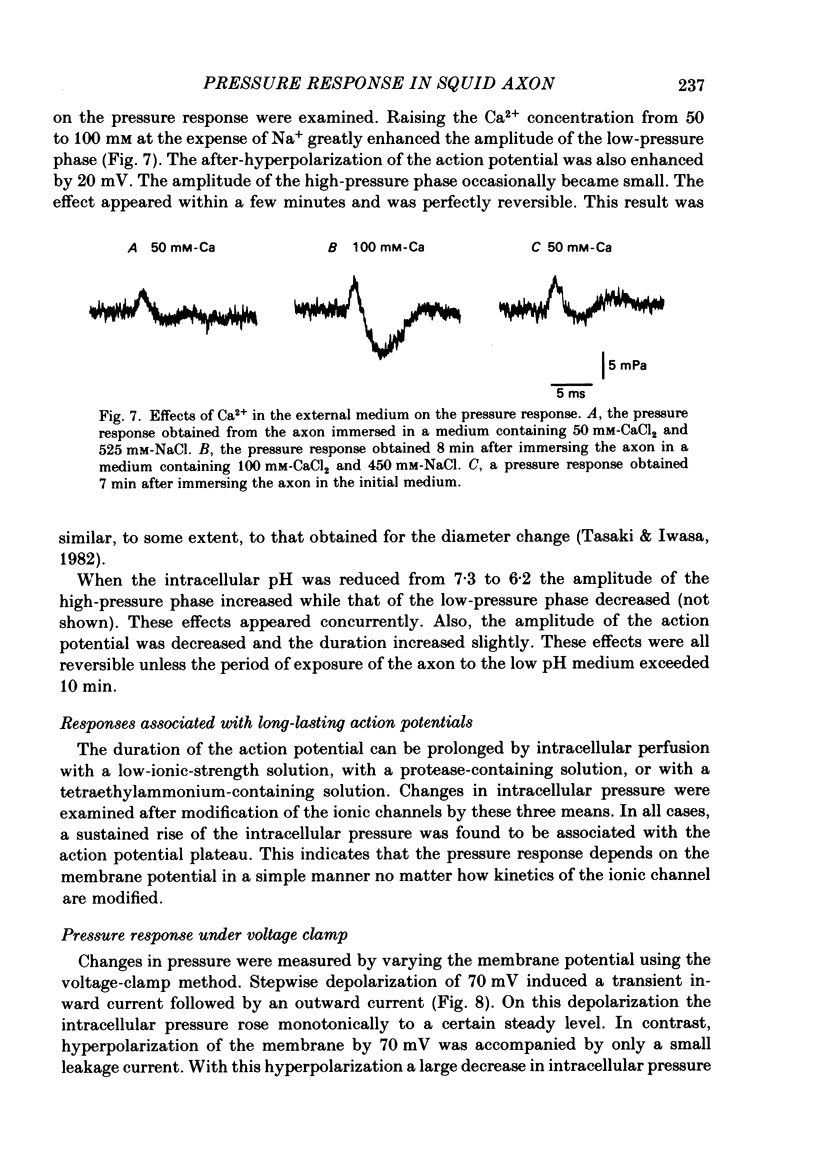
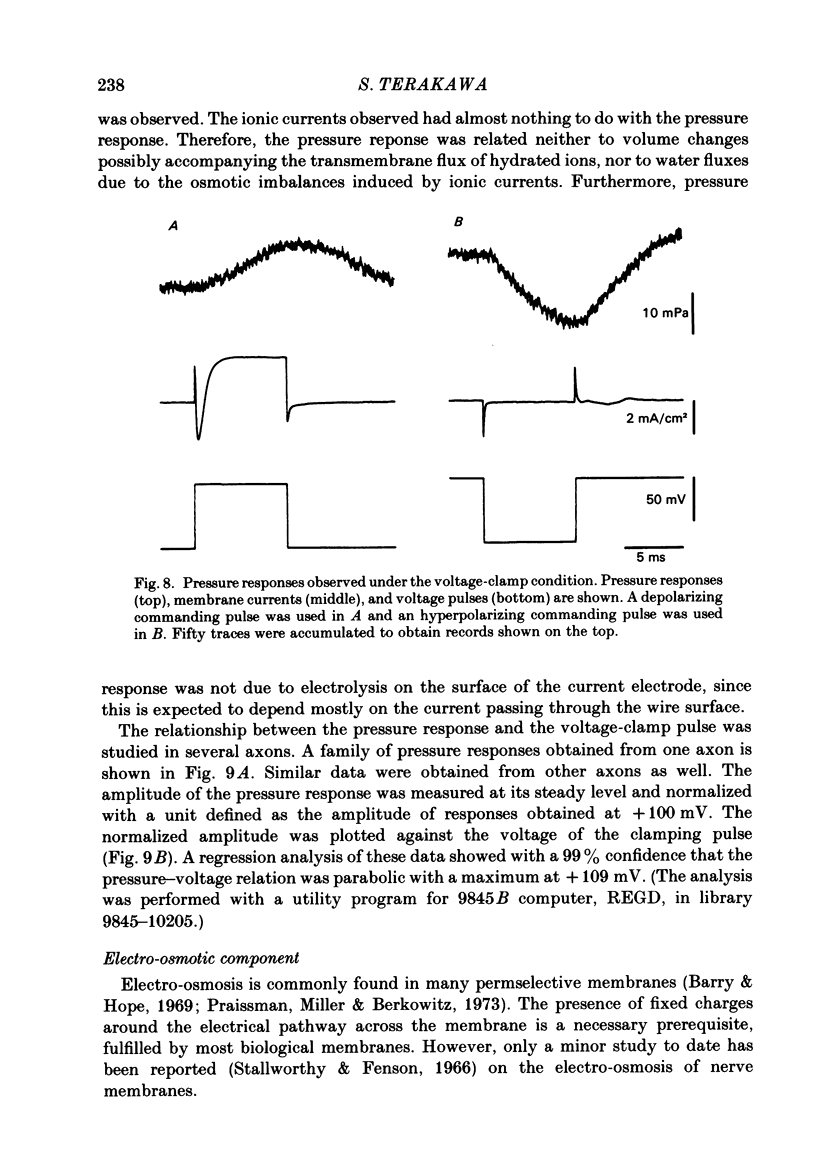
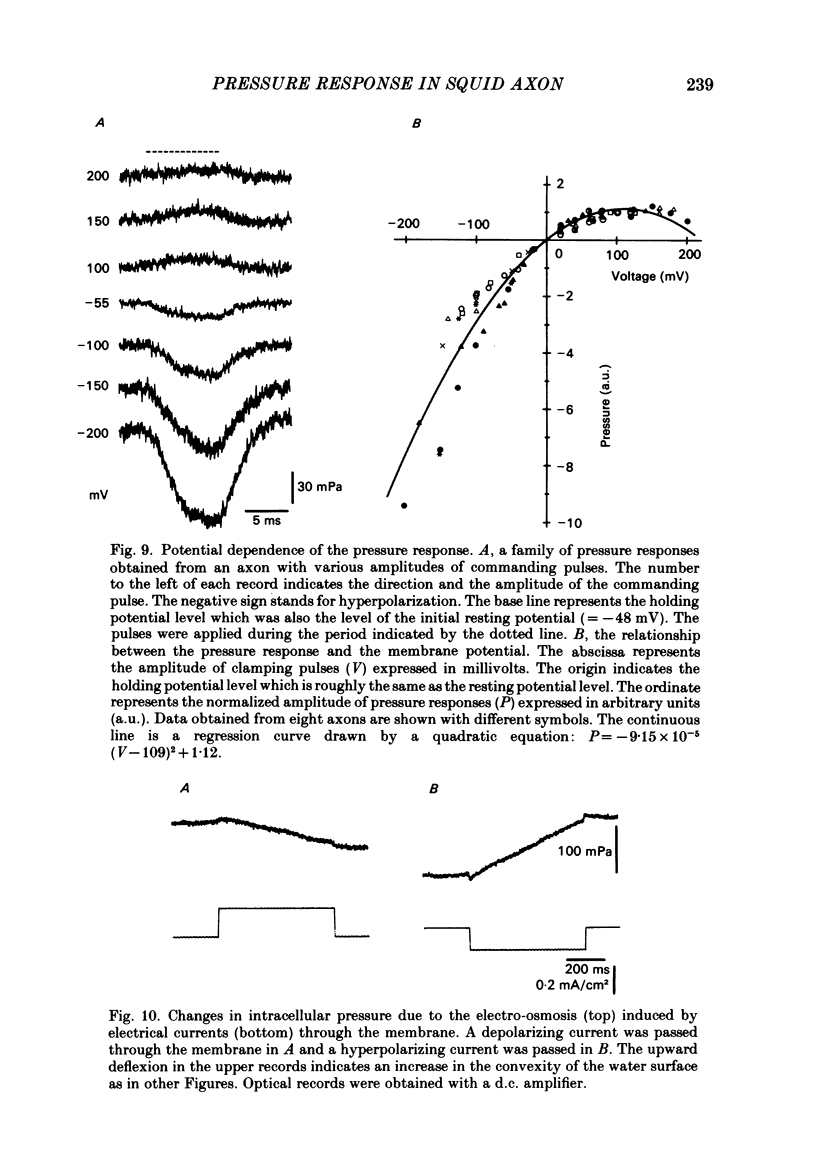
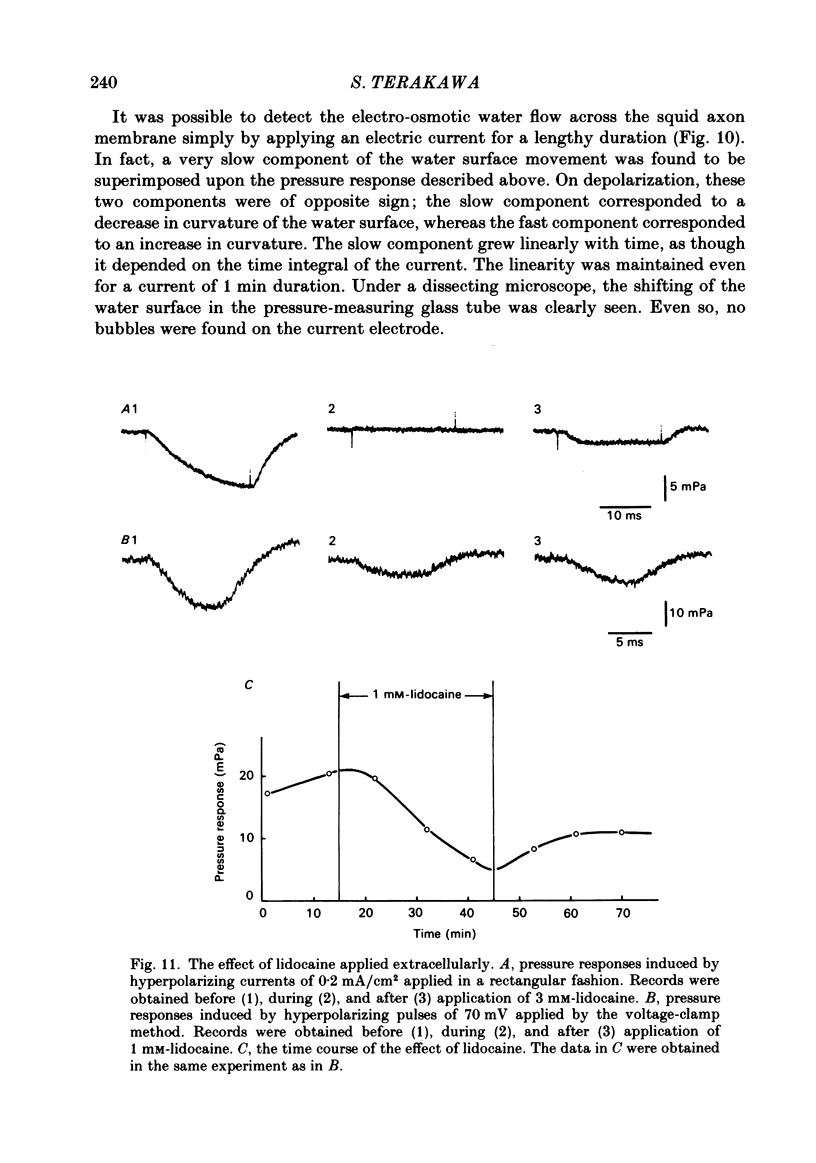
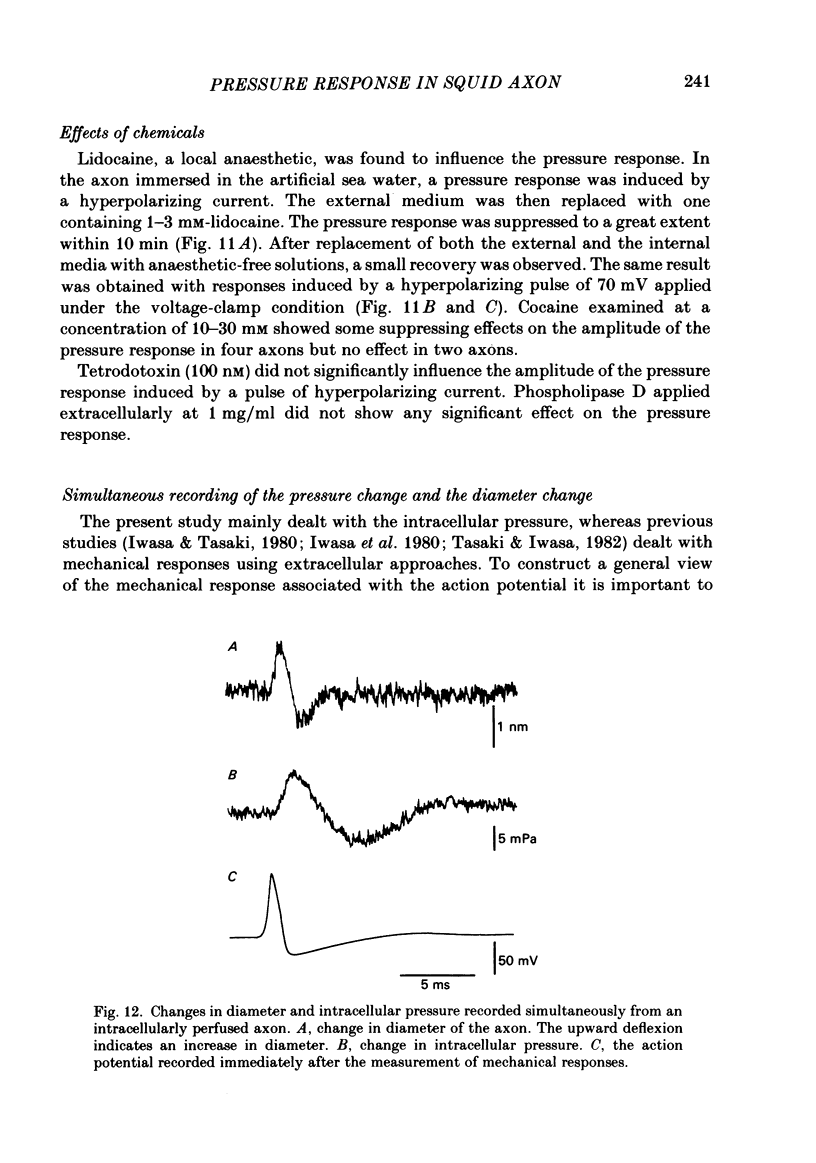
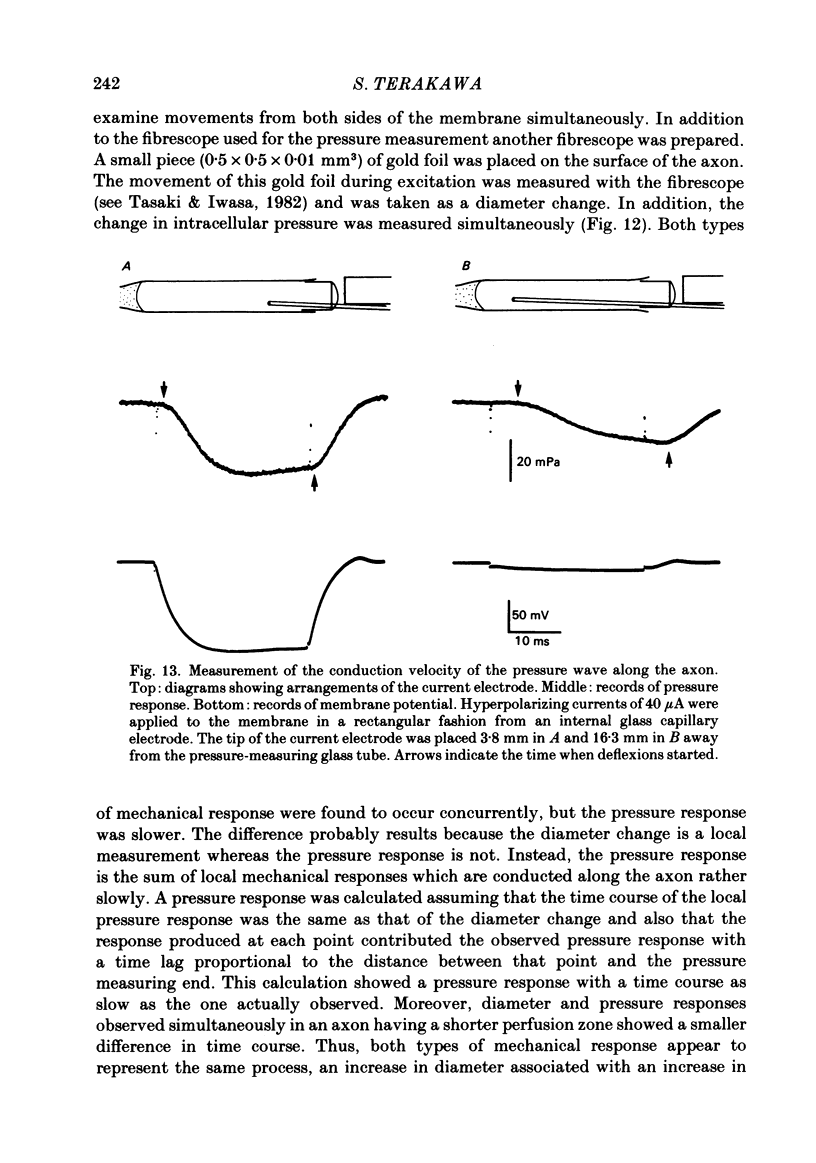
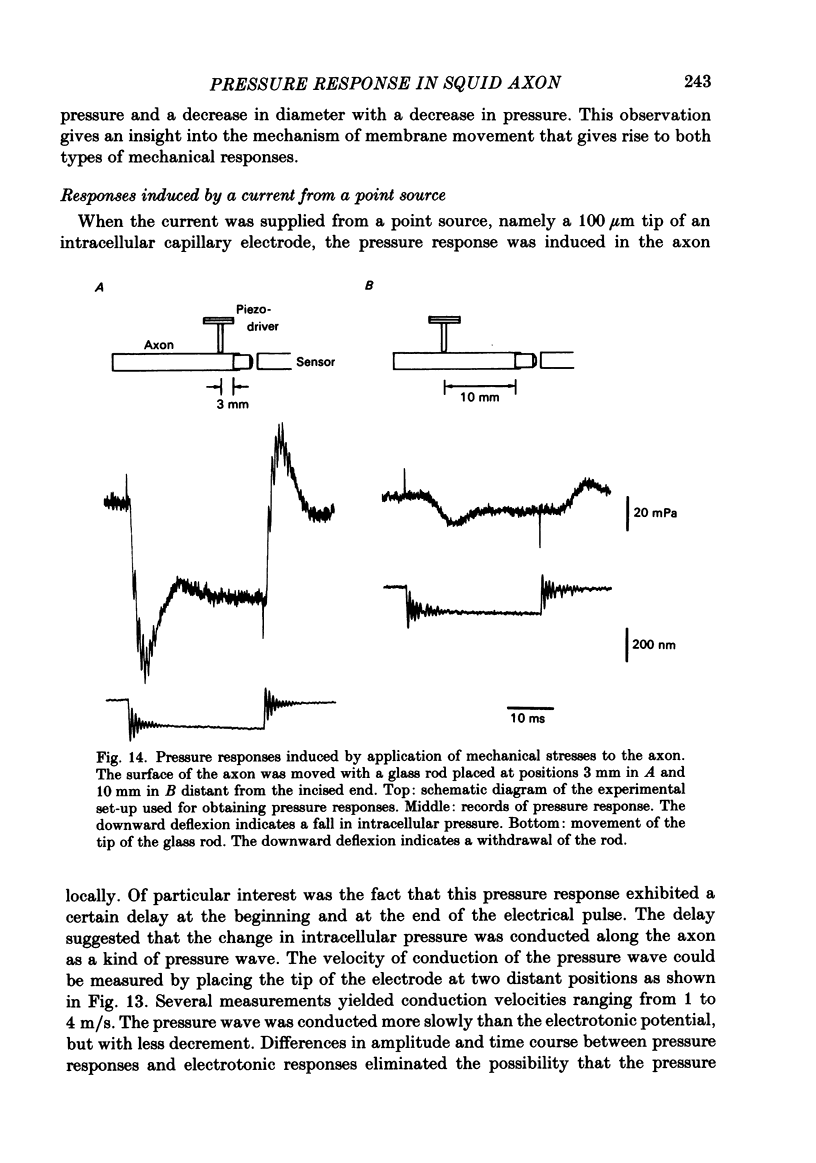
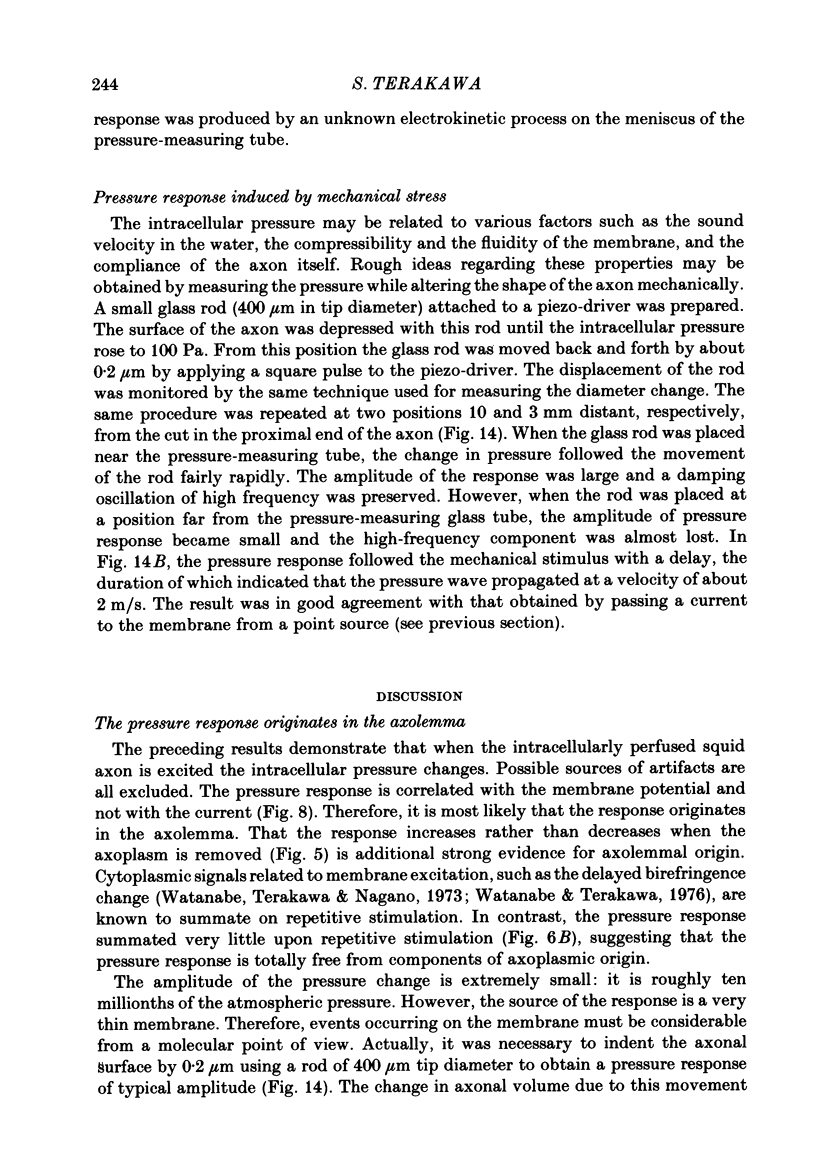
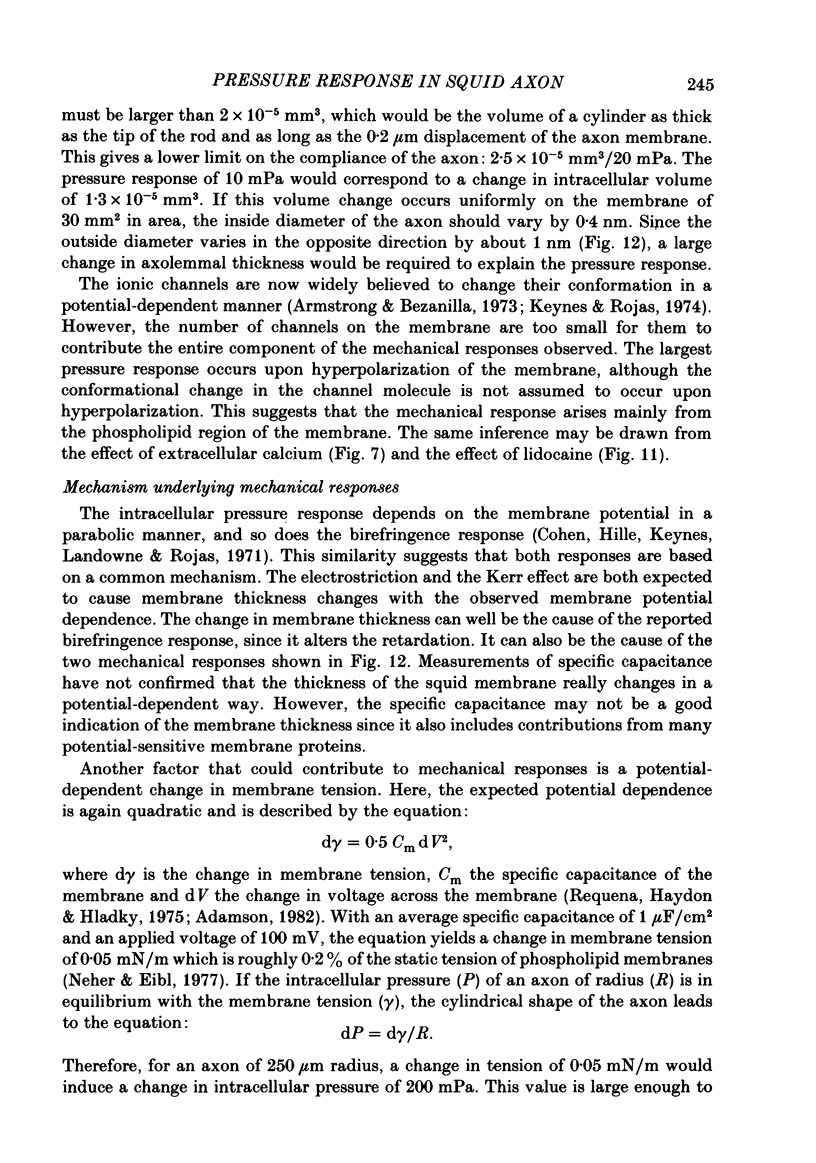
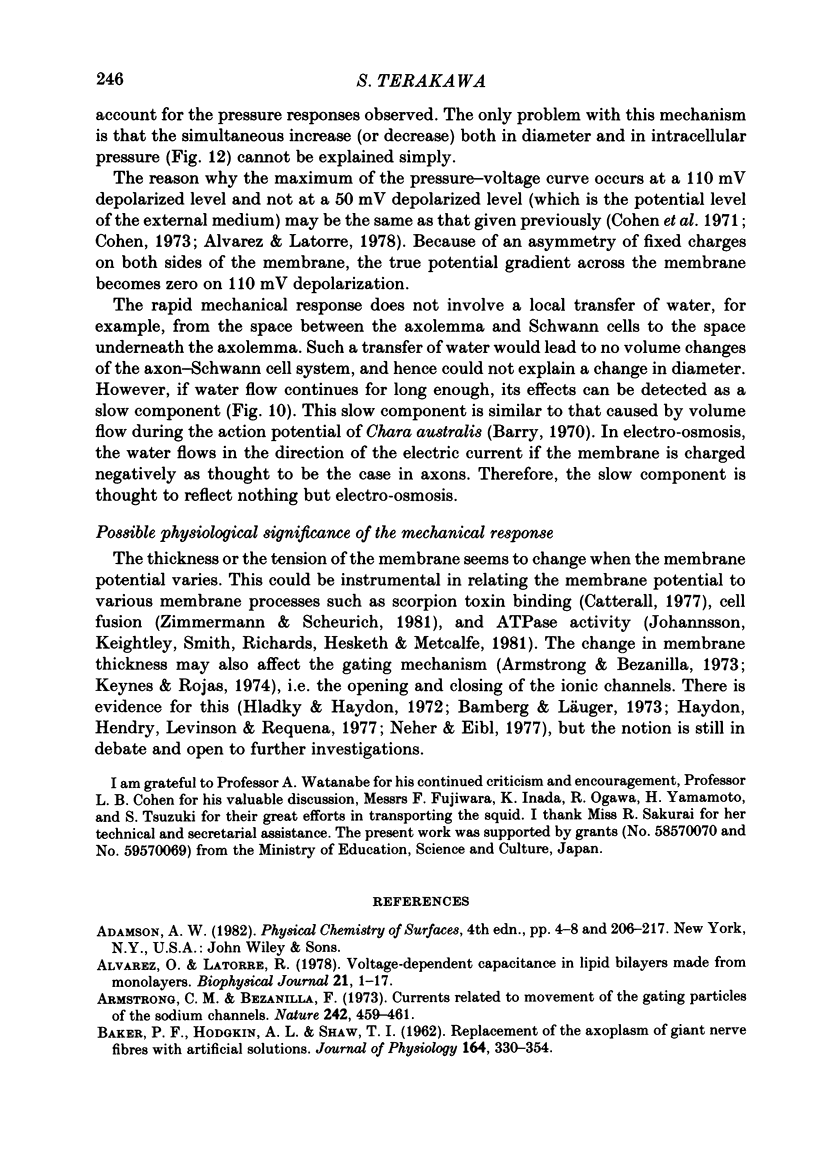
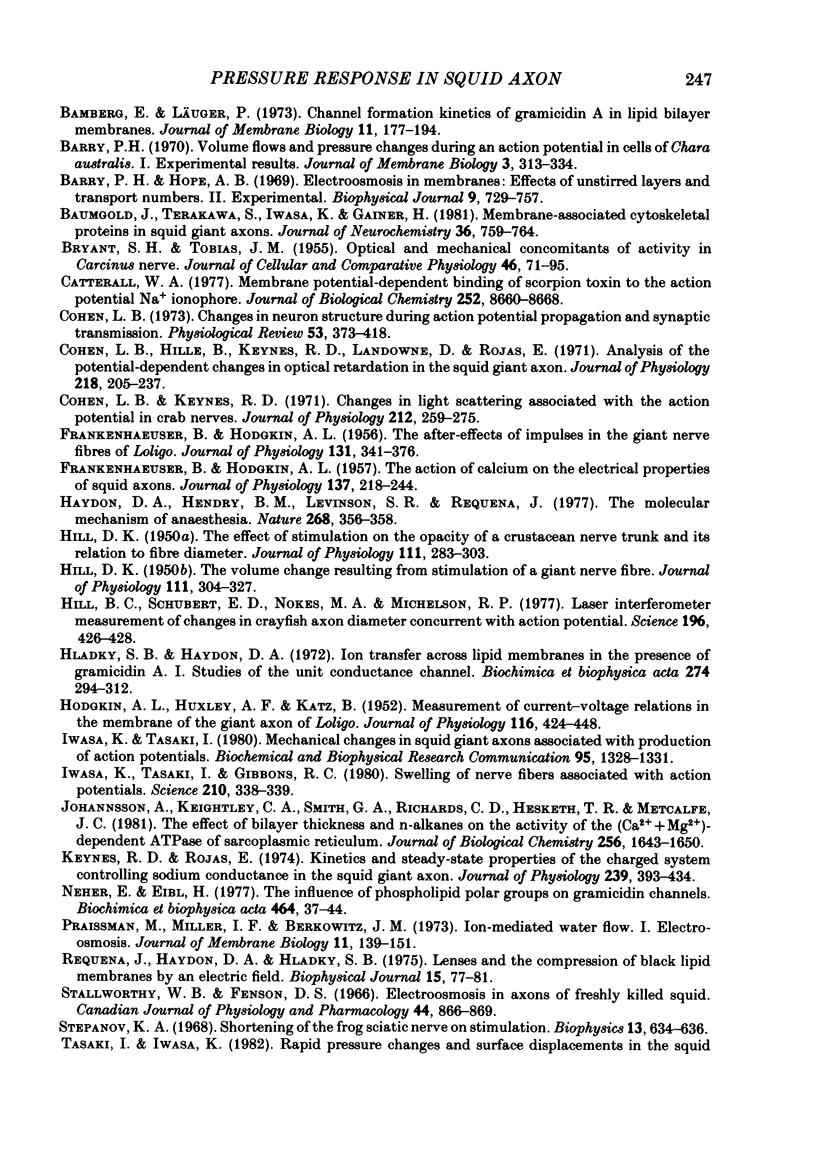
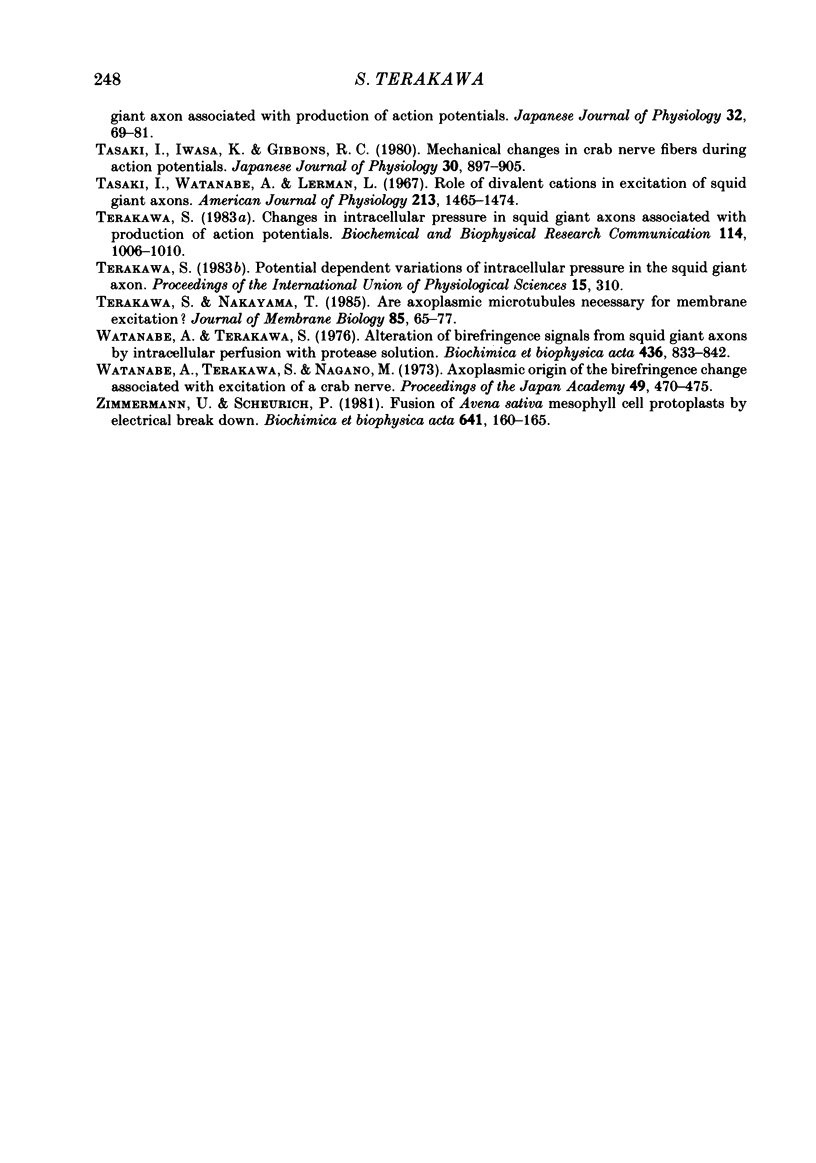
Selected References
These references are in PubMed. This may not be the complete list of references from this article.
- Alvarez O., Latorre R. Voltage-dependent capacitance in lipid bilayers made from monolayers. Biophys J. 1978 Jan;21(1):1–17. doi: 10.1016/S0006-3495(78)85505-2. [DOI] [PMC free article] [PubMed] [Google Scholar]
- Armstrong C. M., Bezanilla F. Currents related to movement of the gating particles of the sodium channels. Nature. 1973 Apr 13;242(5398):459–461. doi: 10.1038/242459a0. [DOI] [PubMed] [Google Scholar]
- BAKER P. F., HODGKIN A. L., SHAW T. I. Replacement of the axoplasm of giant nerve fibres with artificial solutions. J Physiol. 1962 Nov;164:330–354. doi: 10.1113/jphysiol.1962.sp007025. [DOI] [PMC free article] [PubMed] [Google Scholar]
- BRYANT S. H., TOBIAS J. M. Optical and mechanical concomitants of activity in Carcinus nerve. I. Effect of sodium azide on the optical response. II. Shortening of the nerve with activity. J Cell Physiol. 1955 Aug;46(1):71–95. doi: 10.1002/jcp.1030460105. [DOI] [PubMed] [Google Scholar]
- Bamberg E., Läuger P. Channel formation kinetics of gramicidin A in lipid bilayer membranes. J Membr Biol. 1973;11(2):177–194. doi: 10.1007/BF01869820. [DOI] [PubMed] [Google Scholar]
- Barry P. H., Hope A. B. Electroosmosis in membranes: effects of unstirred layers and transport numbers. II. Experimental. Biophys J. 1969 May;9(5):729–757. doi: 10.1016/S0006-3495(69)86414-3. [DOI] [PMC free article] [PubMed] [Google Scholar]
- Baumgold J., Ierakawa S., Iwasa K., Gainer H. Membrane-associated cytoskeletal proteins in squid giant axons. J Neurochem. 1981 Feb;36(2):759–764. doi: 10.1111/j.1471-4159.1981.tb01653.x. [DOI] [PubMed] [Google Scholar]
- Catterall W. A. Membrane potential-dependent binding of scorpion toxin to the action potential Na+ ionophore. Studies with a toxin derivative prepared by lactoperoxidase-catalyzed iodination. J Biol Chem. 1977 Dec 10;252(23):8660–8668. [PubMed] [Google Scholar]
- Cohen L. B. Changes in neuron structure during action potential propagation and synaptic transmission. Physiol Rev. 1973 Apr;53(2):373–418. doi: 10.1152/physrev.1973.53.2.373. [DOI] [PubMed] [Google Scholar]
- Cohen L. B., Hille B., Keynes R. D., Landowne D., Rojas E. Analysis of the potential-dependent changes in optical retardation in the squid giant axon. J Physiol. 1971 Oct;218(1):205–237. doi: 10.1113/jphysiol.1971.sp009611. [DOI] [PMC free article] [PubMed] [Google Scholar]
- Cohen L. B., Keynes R. D. Changes in light scattering associated with the action potential in crab nerves. J Physiol. 1971 Jan;212(1):259–275. doi: 10.1113/jphysiol.1971.sp009321. [DOI] [PMC free article] [PubMed] [Google Scholar]
- FRANKENHAEUSER B., HODGKIN A. L. The action of calcium on the electrical properties of squid axons. J Physiol. 1957 Jul 11;137(2):218–244. doi: 10.1113/jphysiol.1957.sp005808. [DOI] [PMC free article] [PubMed] [Google Scholar]
- FRANKENHAEUSER B., HODGKIN A. L. The after-effects of impulses in the giant nerve fibres of Loligo. J Physiol. 1956 Feb 28;131(2):341–376. doi: 10.1113/jphysiol.1956.sp005467. [DOI] [PMC free article] [PubMed] [Google Scholar]
- HILL D. K. The effect of stimulation on the opacity of a crustacean nerve trunk and its relation to fibre diameter. J Physiol. 1950 Oct 16;111(3-4):283–303. doi: 10.1113/jphysiol.1950.sp004480. [DOI] [PMC free article] [PubMed] [Google Scholar]
- HILL D. K. The volume change resulting from stimulation of a giant nerve fibre. J Physiol. 1950 Oct 16;111(3-4):304–327. doi: 10.1113/jphysiol.1950.sp004481. [DOI] [PMC free article] [PubMed] [Google Scholar]
- HODGKIN A. L., HUXLEY A. F., KATZ B. Measurement of current-voltage relations in the membrane of the giant axon of Loligo. J Physiol. 1952 Apr;116(4):424–448. doi: 10.1113/jphysiol.1952.sp004716. [DOI] [PMC free article] [PubMed] [Google Scholar]
- Haydon D. A., Hendry B. M., Levinson S. R., Requena J. The molecular mechanisms of anaesthesia. Nature. 1977 Jul 28;268(5618):356–358. doi: 10.1038/268356a0. [DOI] [PubMed] [Google Scholar]
- Hill B. C., Schubert E. D., Nokes M. A., Michelson R. P. Laser interferometer measurement of changes in crayfish axon diameter concurrent with action potential. Science. 1977 Apr 22;196(4288):426–428. doi: 10.1126/science.850785. [DOI] [PubMed] [Google Scholar]
- Hladky S. B., Haydon D. A. Ion transfer across lipid membranes in the presence of gramicidin A. I. Studies of the unit conductance channel. Biochim Biophys Acta. 1972 Aug 9;274(2):294–312. doi: 10.1016/0005-2736(72)90178-2. [DOI] [PubMed] [Google Scholar]
- Iwasa K., Tasaki I., Gibbons R. C. Swelling of nerve fibers associated with action potentials. Science. 1980 Oct 17;210(4467):338–339. doi: 10.1126/science.7423196. [DOI] [PubMed] [Google Scholar]
- Iwasa K., Tasaki I. Mechanical changes in squid giant axons associated with production of action potentials. Biochem Biophys Res Commun. 1980 Aug 14;95(3):1328–1331. doi: 10.1016/0006-291x(80)91619-8. [DOI] [PubMed] [Google Scholar]
- Johannsson A., Keightley C. A., Smith G. A., Richards C. D., Hesketh T. R., Metcalfe J. C. The effect of bilayer thickness and n-alkanes on the activity of the (Ca2+ + Mg2+)-dependent ATPase of sarcoplasmic reticulum. J Biol Chem. 1981 Feb 25;256(4):1643–1650. [PubMed] [Google Scholar]
- Keynes R. D., Rojas E. Kinetics and steady-state properties of the charged system controlling sodium conductance in the squid giant axon. J Physiol. 1974 Jun;239(2):393–434. doi: 10.1113/jphysiol.1974.sp010575. [DOI] [PMC free article] [PubMed] [Google Scholar]
- Letter: Lenses and the compression of black lipid membranes by an electric field. Biophys J. 1975 Jan;15(1):77–81. doi: 10.1016/S0006-3495(75)85793-6. [DOI] [PMC free article] [PubMed] [Google Scholar]
- Neher E., Eibl H. The influence of phospholipid polar groups on gramicidin channels. Biochim Biophys Acta. 1977 Jan 4;464(1):37–44. doi: 10.1016/0005-2736(77)90368-6. [DOI] [PubMed] [Google Scholar]
- Praissman M., Miller I. F., Berkowitz J. M. Ion-mediated water flow. I. Electroosmosis. J Membr Biol. 1973;11(2):139–151. doi: 10.1007/BF01869817. [DOI] [PubMed] [Google Scholar]
- Stallworthy W. B., Fensom D. S. Electroosmosis in axons of freshly killed squid. Can J Physiol Pharmacol. 1966 Sep;44(5):866–870. doi: 10.1139/y66-106. [DOI] [PubMed] [Google Scholar]
- Tasaki I., Iwasa K., Gibbons R. C. Mechanical changes in crab nerve fibers during action potentials. Jpn J Physiol. 1980;30(6):897–905. doi: 10.2170/jjphysiol.30.897. [DOI] [PubMed] [Google Scholar]
- Tasaki I., Iwasa K. Rapid pressure changes and surface displacements in the squid giant axon associated with production of action potentials. Jpn J Physiol. 1982;32(1):69–81. doi: 10.2170/jjphysiol.32.69. [DOI] [PubMed] [Google Scholar]
- Tasaki I., Watanabe A., Lerman L. Role of divalent cations in excitation of squid giant axons. Am J Physiol. 1967 Dec;213(6):1465–1474. doi: 10.1152/ajplegacy.1967.213.6.1465. [DOI] [PubMed] [Google Scholar]
- Terakawa S. Changes in intracellular pressure in squid giant axons associated with production of action potentials. Biochem Biophys Res Commun. 1983 Aug 12;114(3):1006–1010. doi: 10.1016/0006-291x(83)90661-7. [DOI] [PubMed] [Google Scholar]
- Terakawa S., Nakayama T. Are axoplasmic microtubules necessary for membrane excitation? J Membr Biol. 1985;85(1):65–77. doi: 10.1007/BF01872006. [DOI] [PubMed] [Google Scholar]
- Watanabe A., Terakawa S. Alteration of birefringence signals from squid giant axons by intracellular perfusion with protease solution. Biochim Biophys Acta. 1976 Jul 15;436(4):833–842. doi: 10.1016/0005-2736(76)90410-7. [DOI] [PubMed] [Google Scholar]
- Zimmermann U., Scheurich P. Fusion of Avena sativa mesophyll cell protoplasts by electrical breakdown. Biochim Biophys Acta. 1981 Feb 20;641(1):160–165. doi: 10.1016/0005-2736(81)90579-4. [DOI] [PubMed] [Google Scholar]