Abstract
J774.1 cells, a mouse-derived macrophage-like tumour cell line, were voltage clamped using whole-cell patch-clamp techniques. Cells were maintained in suspension cultures and plated at varying times before recording. The average zero-current potential of long-term adherent (greater than 24 h) cells was -77.6 mV. A tenfold increase in [K]o produced a 49 mV shift in zero-current potential. Freshly plated cells (less than 24 h) expressed two voltage-dependent currents: an outward current expressed transiently from 1 to 12 h post-plating and an inward current expressed 2-4 h post-plating which persisted in 100% of long-term adherent cells. Inward current was dependent upon voltage, time and [K]o 1/2, similar to the anomalous rectifier of other tissues. The conductance activated at potentials negative to -50 mV and plateaued at potentials negative to -110 mV. Inactivation was evident at potentials negative to -100 mV. Both the rate and extent of inactivation increased with hyperpolarization. Inward rectification was blocked by external BaCl2 or CsCl. The outward current was time- and voltage-dependent. The instantaneous I/V curves derived from tail experiments reversed at the potassium equilibrium potential (EK). A tenfold change of [K]o shifted the reversal potential 52 mV, indicating that the current was carried by potassium. This conductance activated at potentials positive to -50 mV, plateaued at potentials positive to -10 mV and inactivated completely with an exponential time course at all potentials. At voltages positive to -25 mV the rate of inactivation was independent of voltage. The outward current was blocked by 4-aminopyridine or D600. During the first 10 min after attaining a whole-cell recording, the conductance/voltage relation of the outward current shifted to more negative voltages and peak conductance showed a slight increase; recordings then stabilized. The voltage dependence of the inward current did not shift with time but wash-out of inward current was observed in some cells. The J774.1 cell line can serve as a model for the study of the role of voltage-dependent ionic conductances in macrophages.
Full text
PDF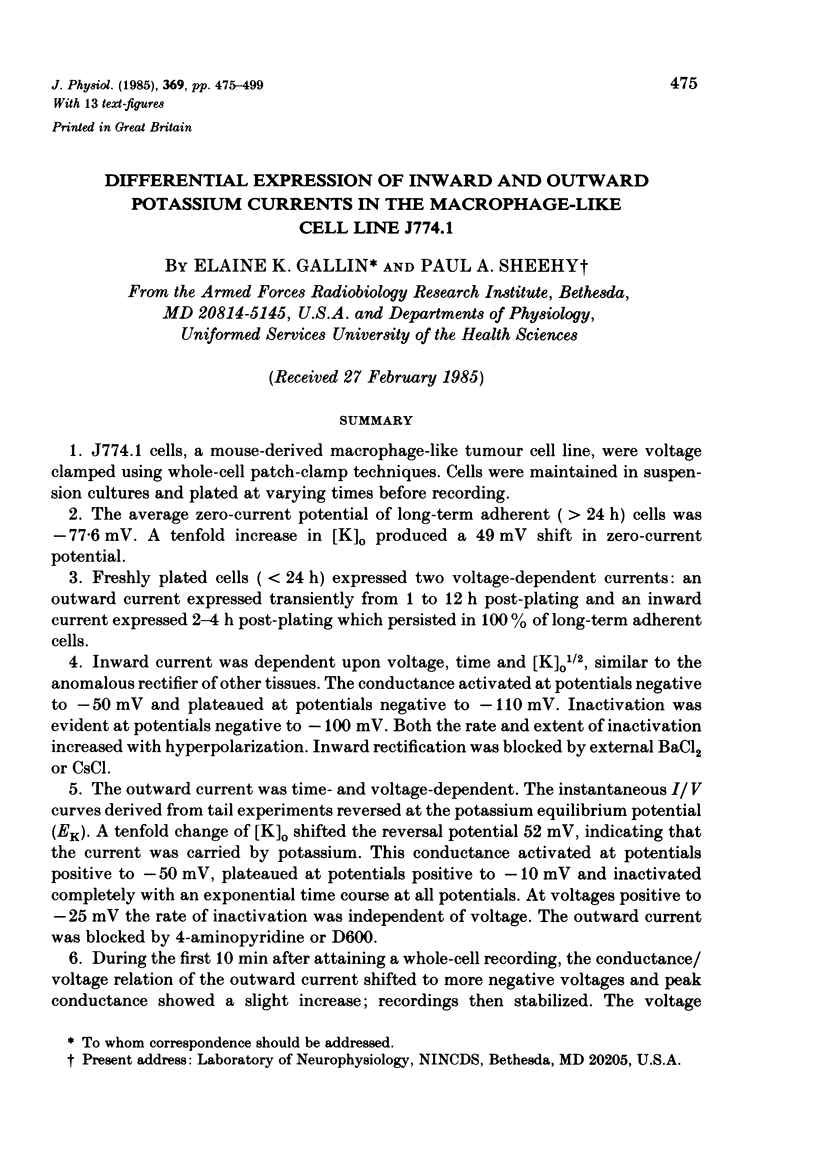
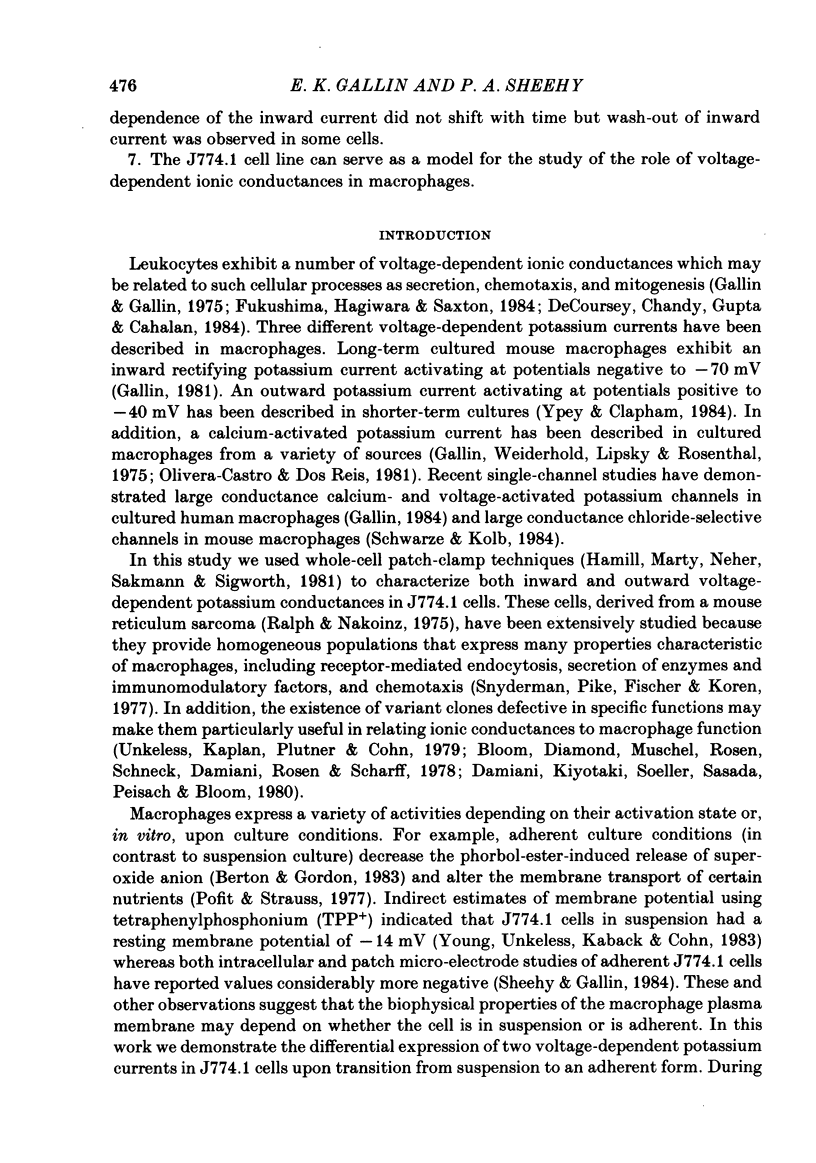
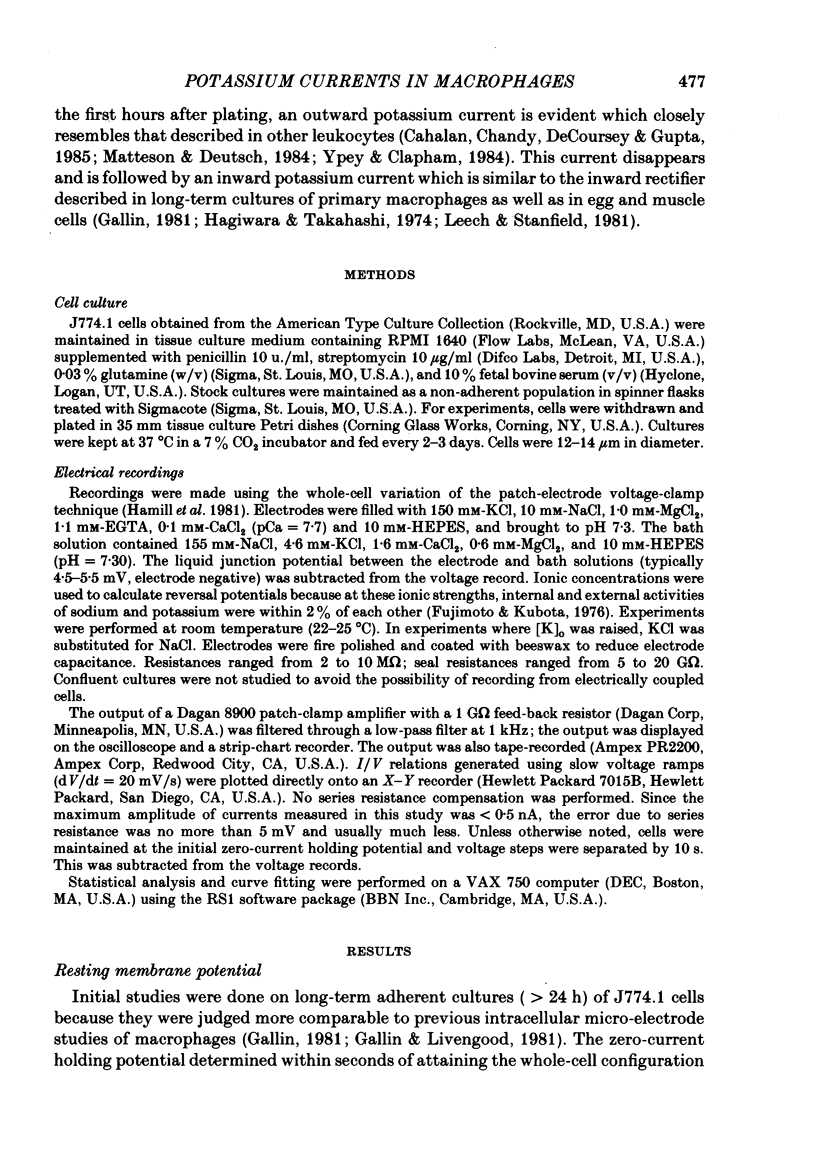
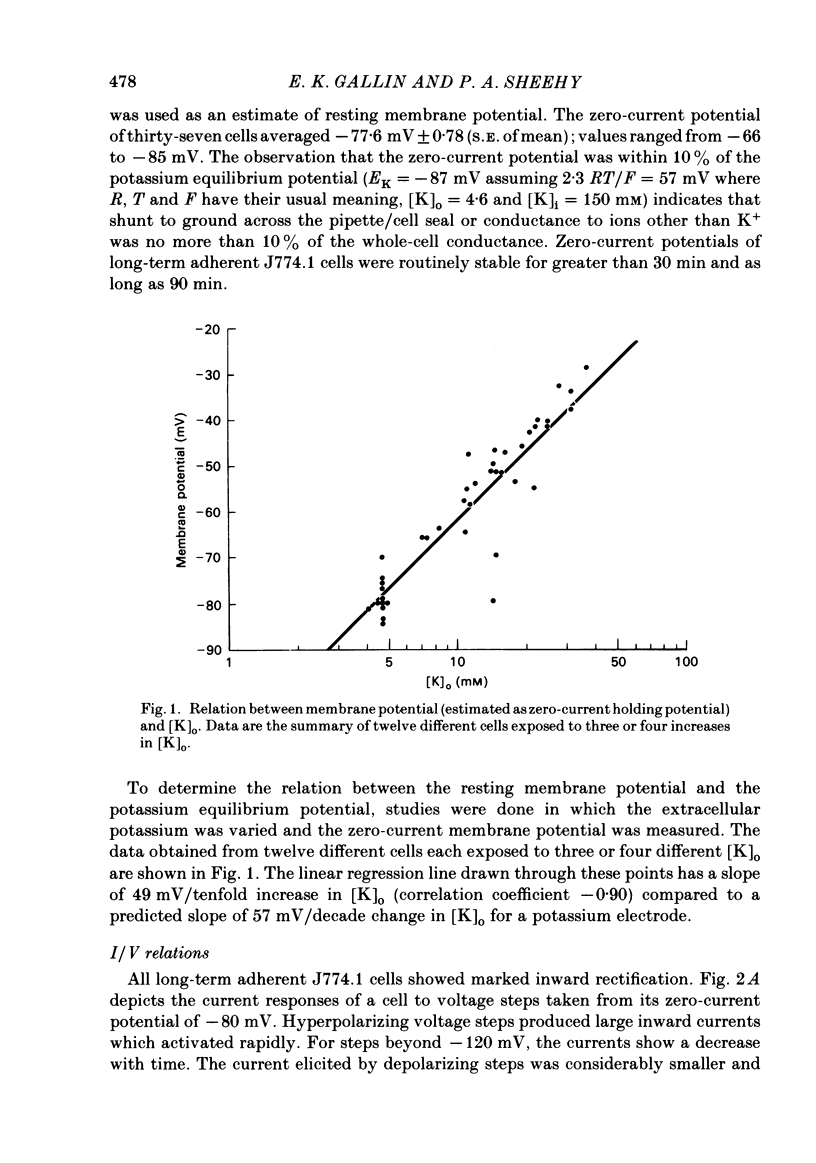
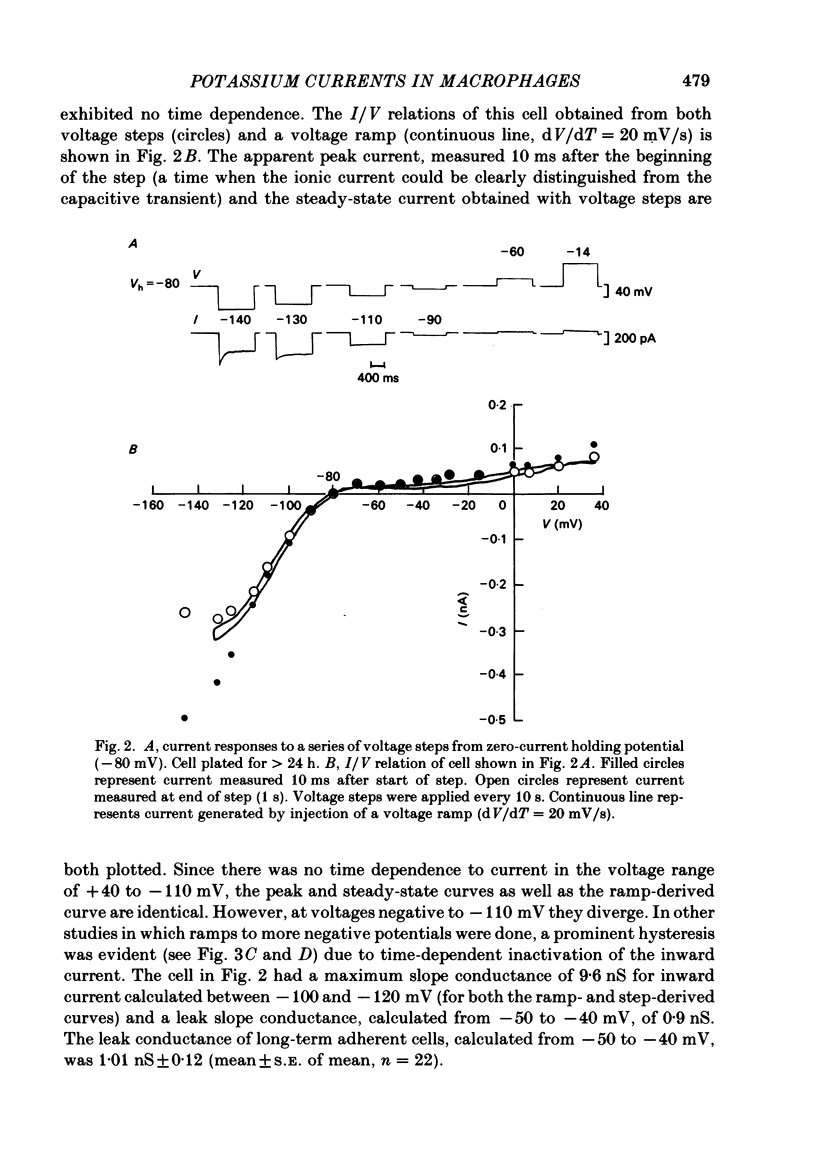
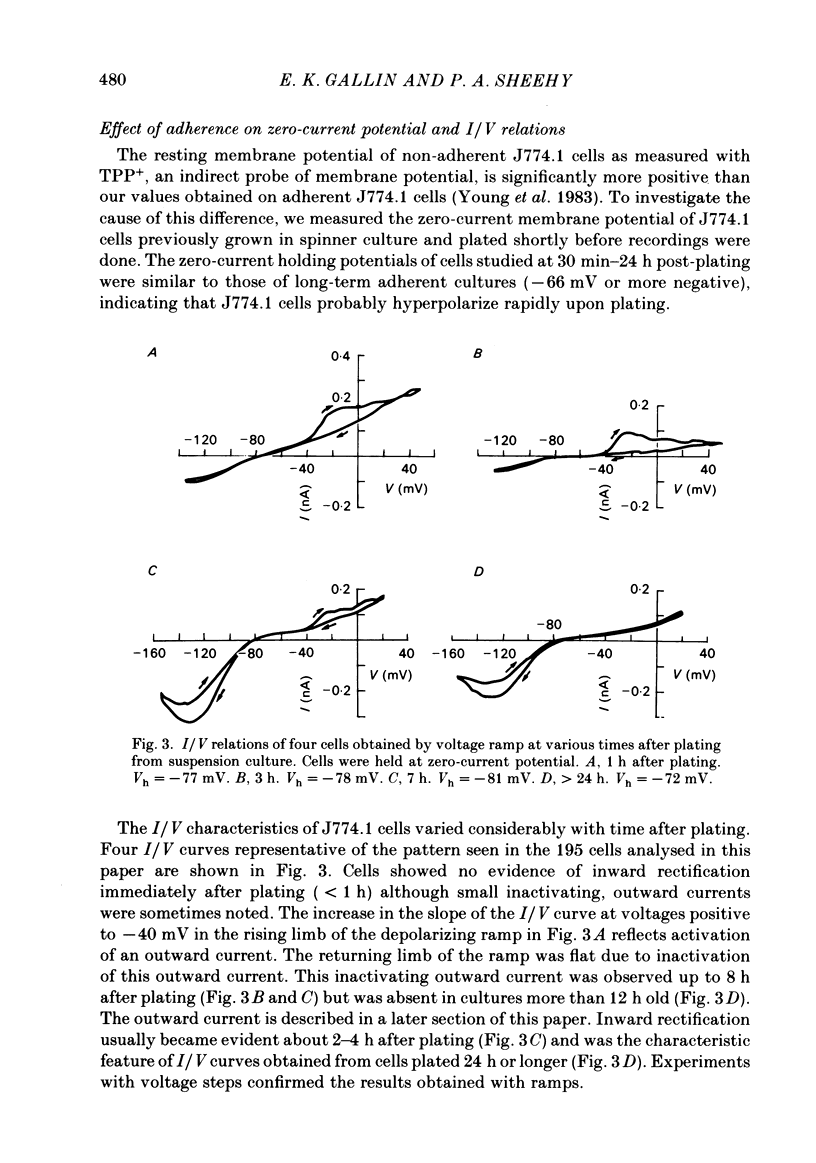
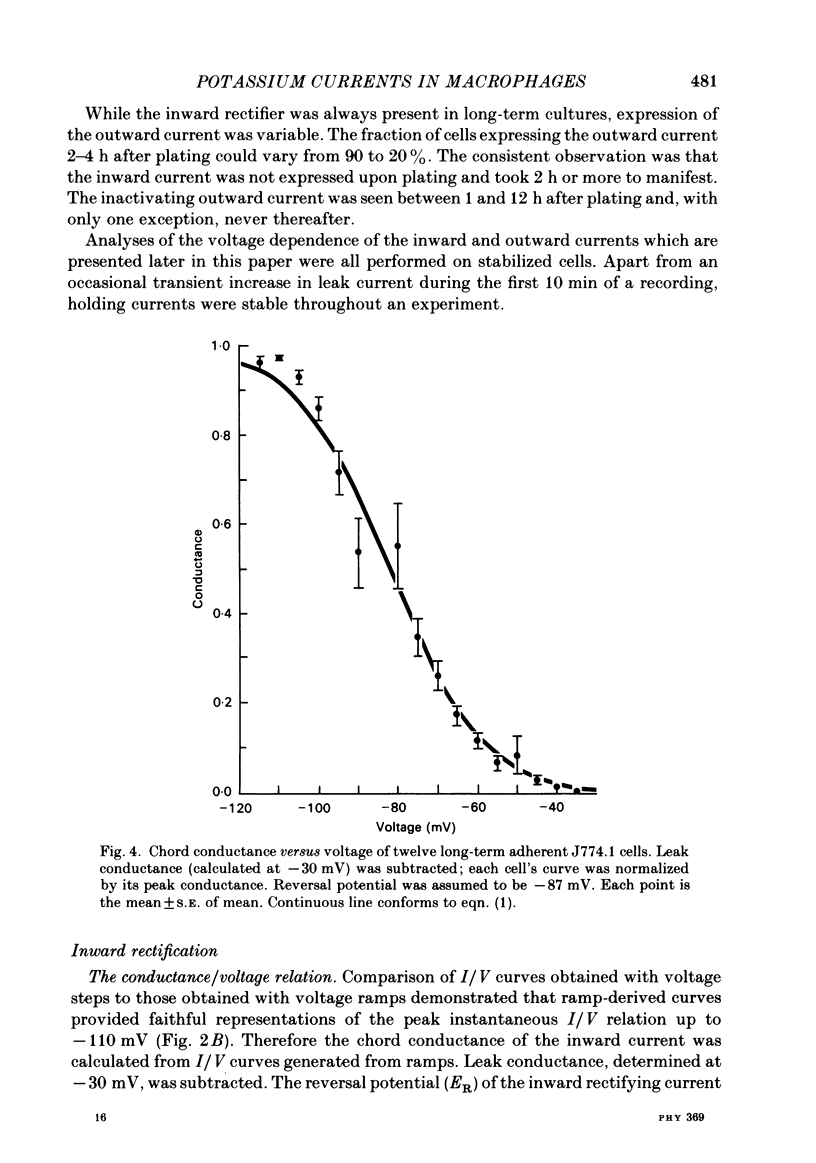
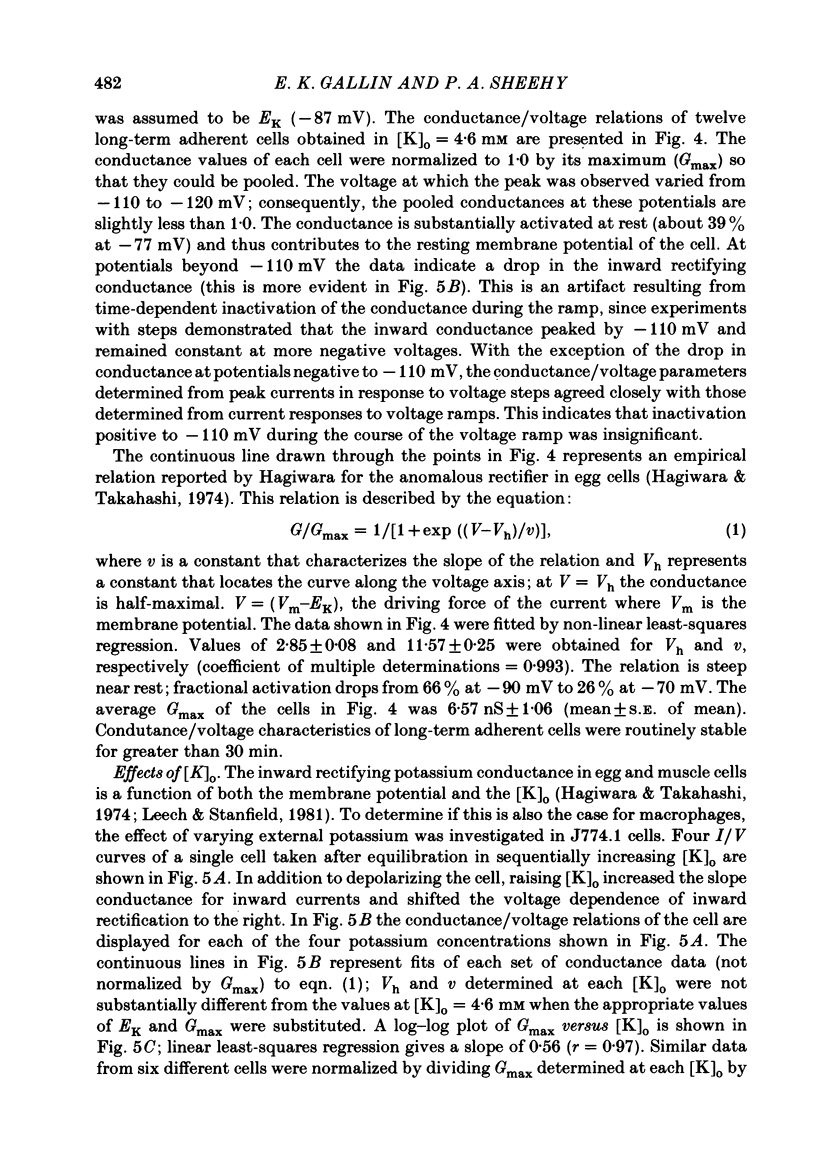
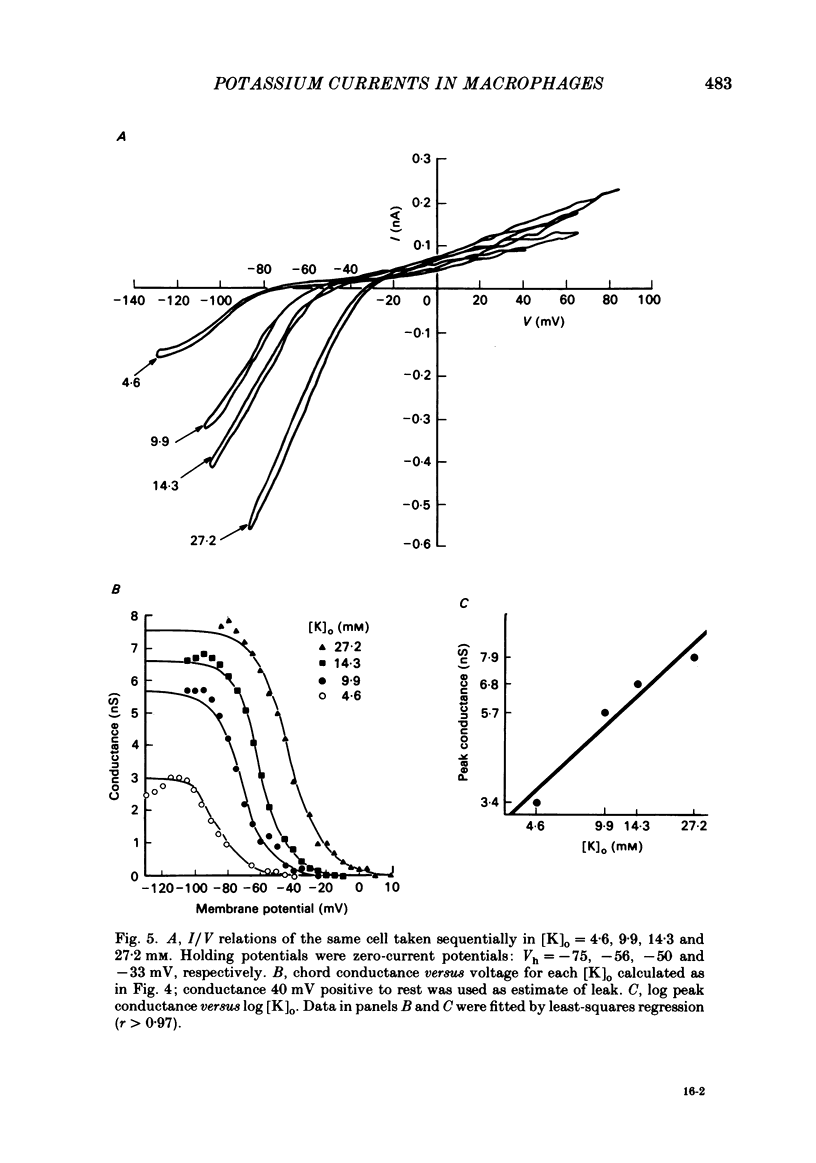
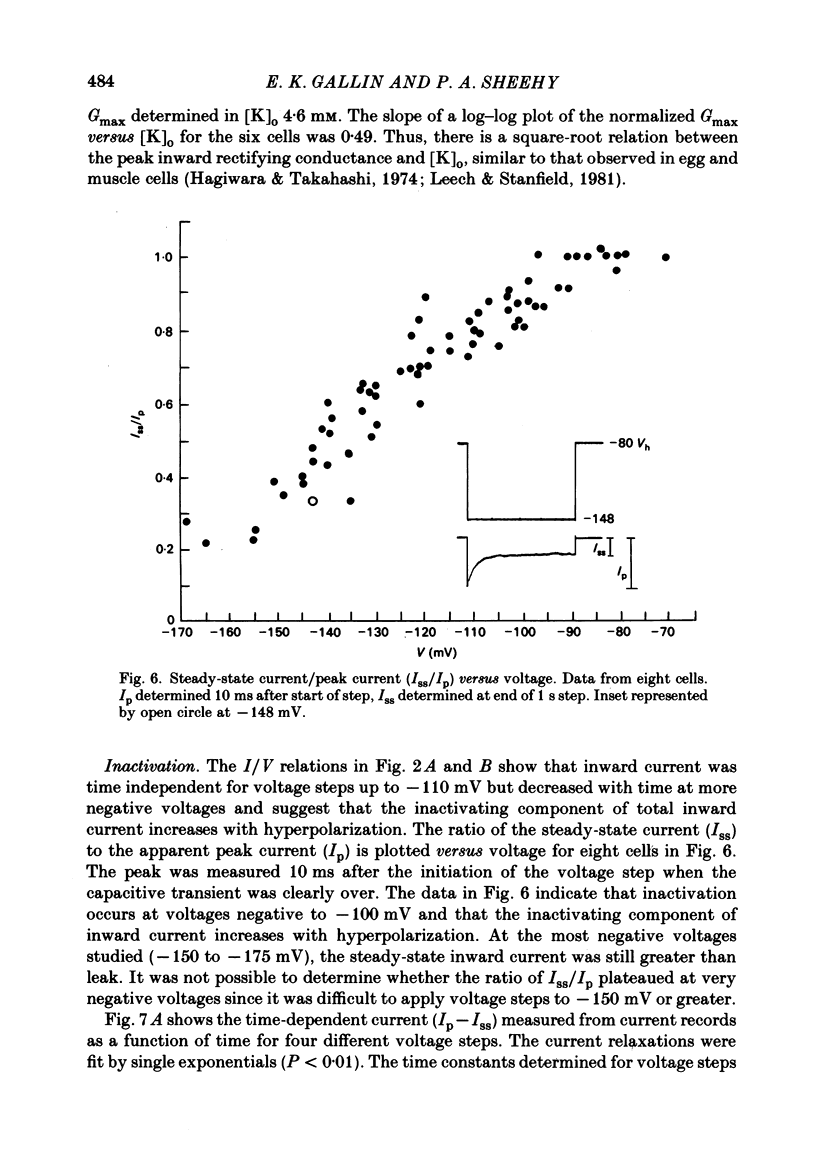
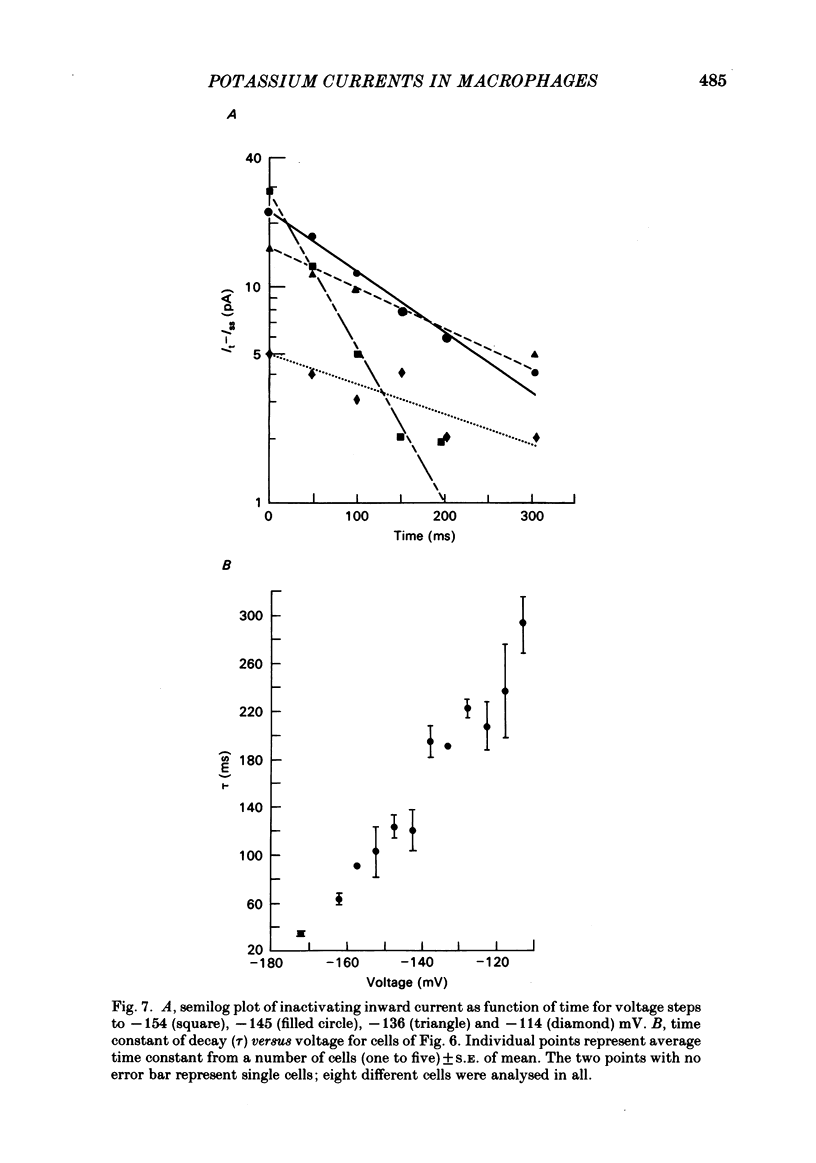
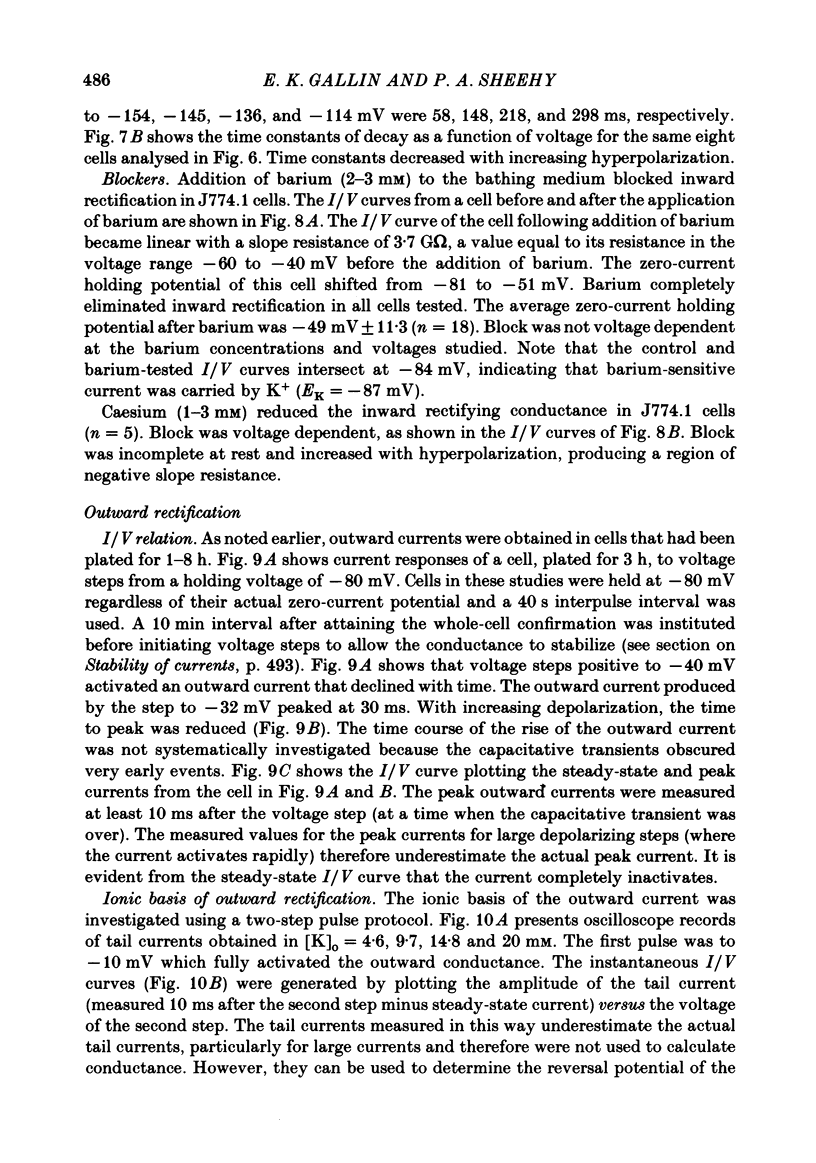
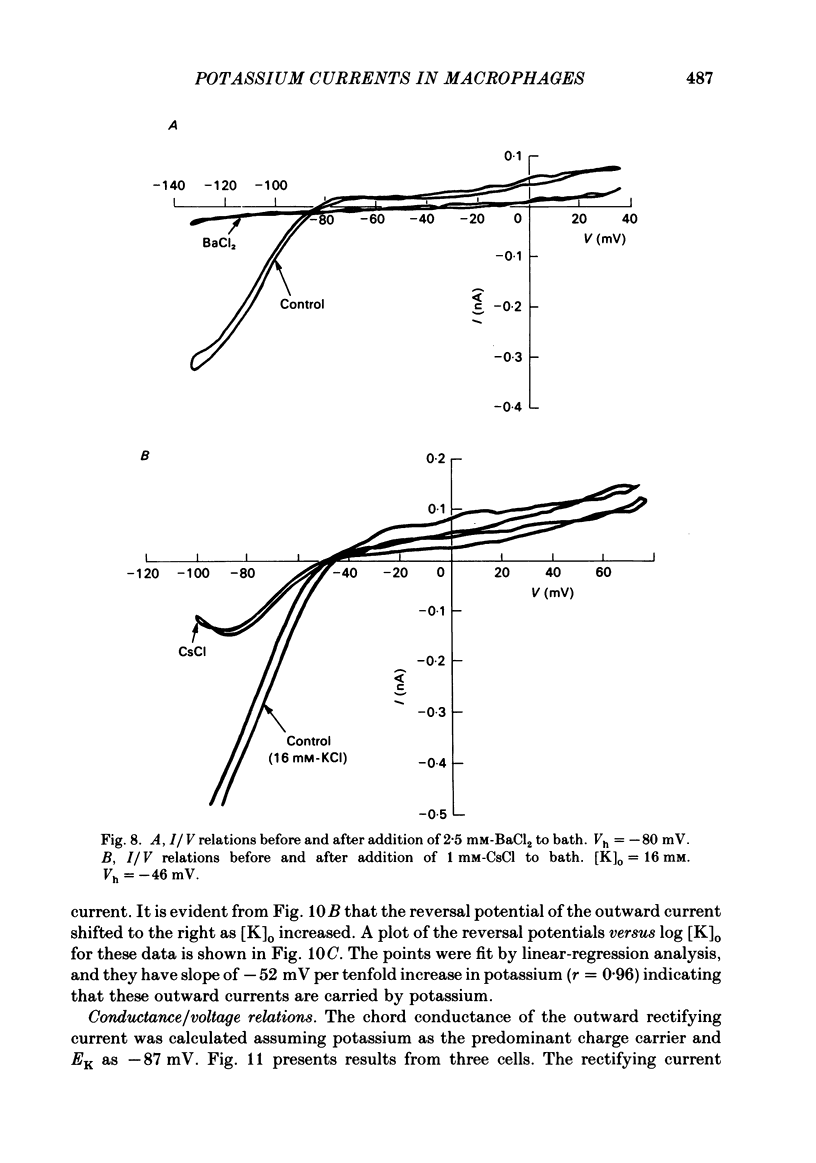
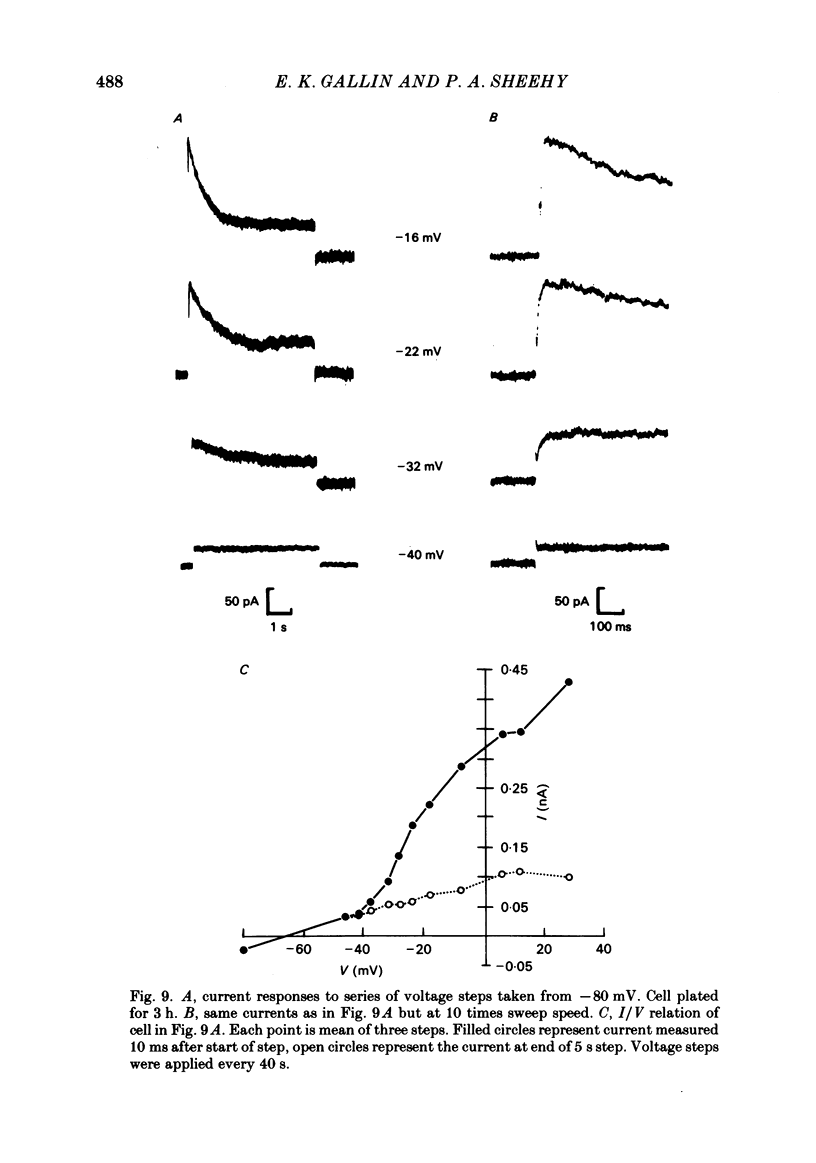
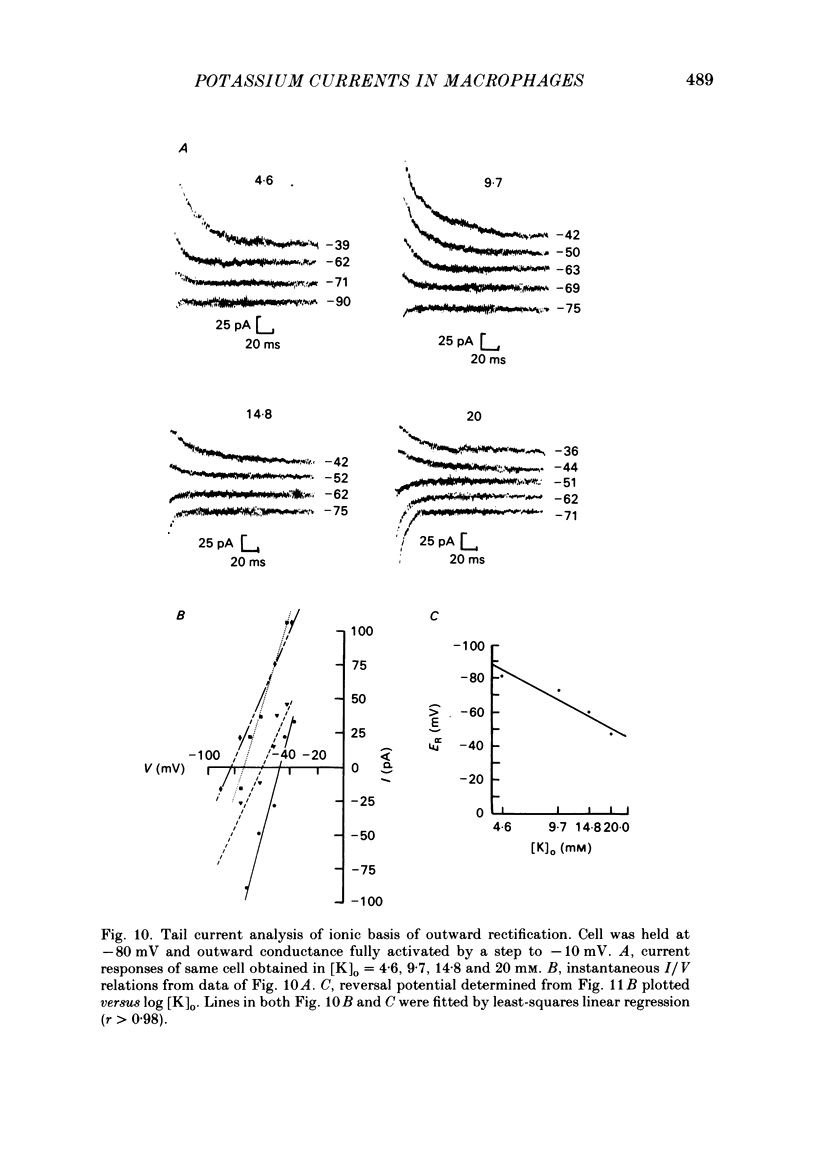
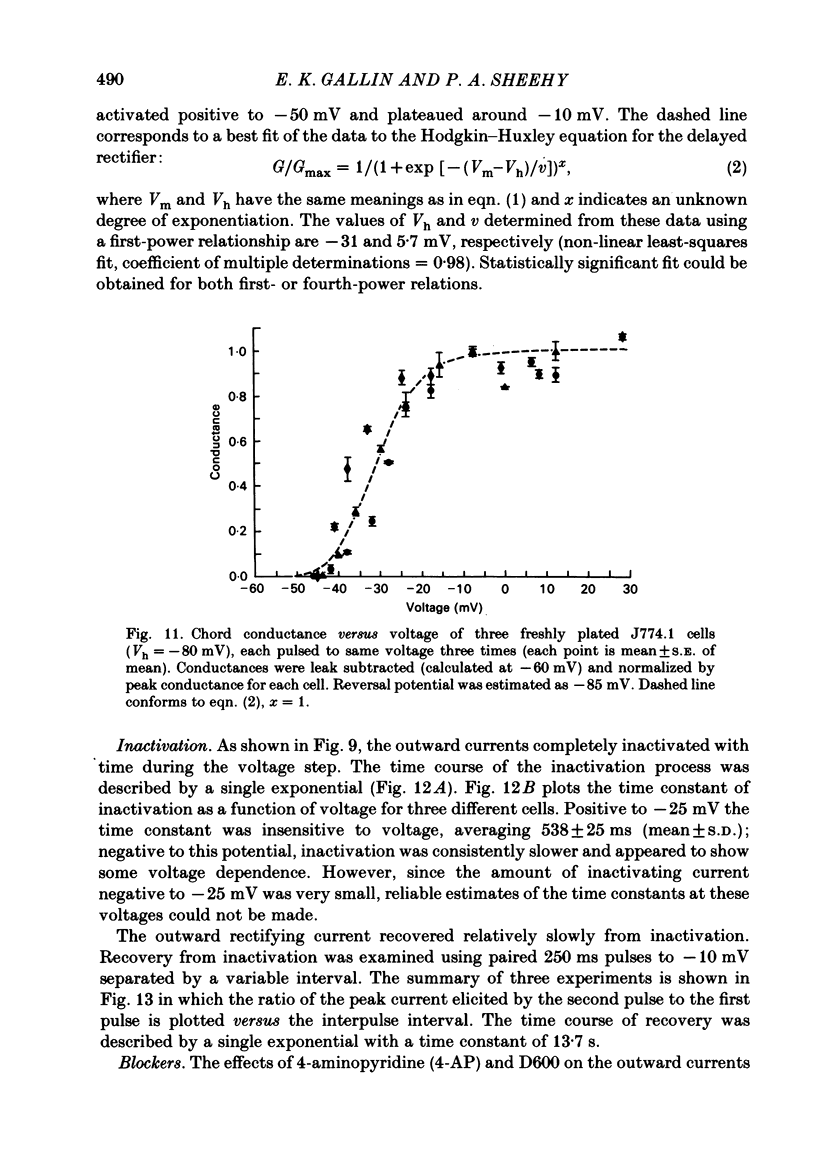
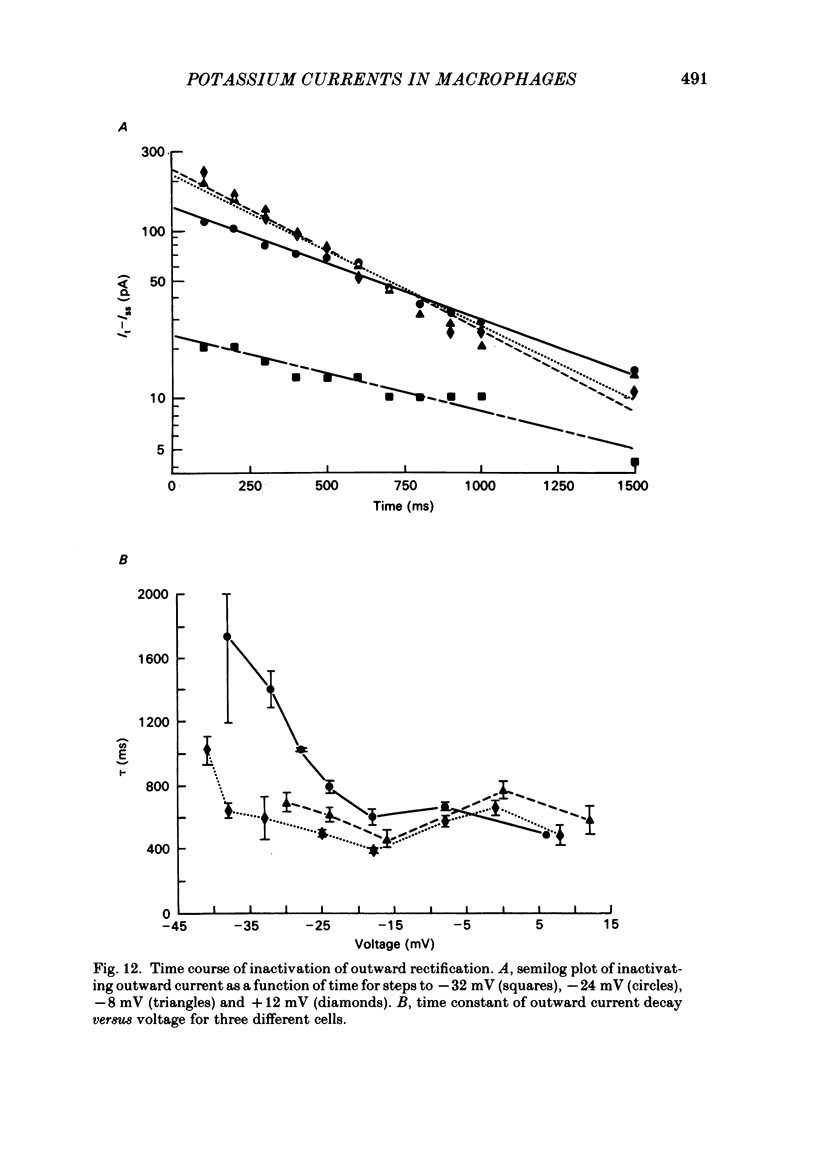
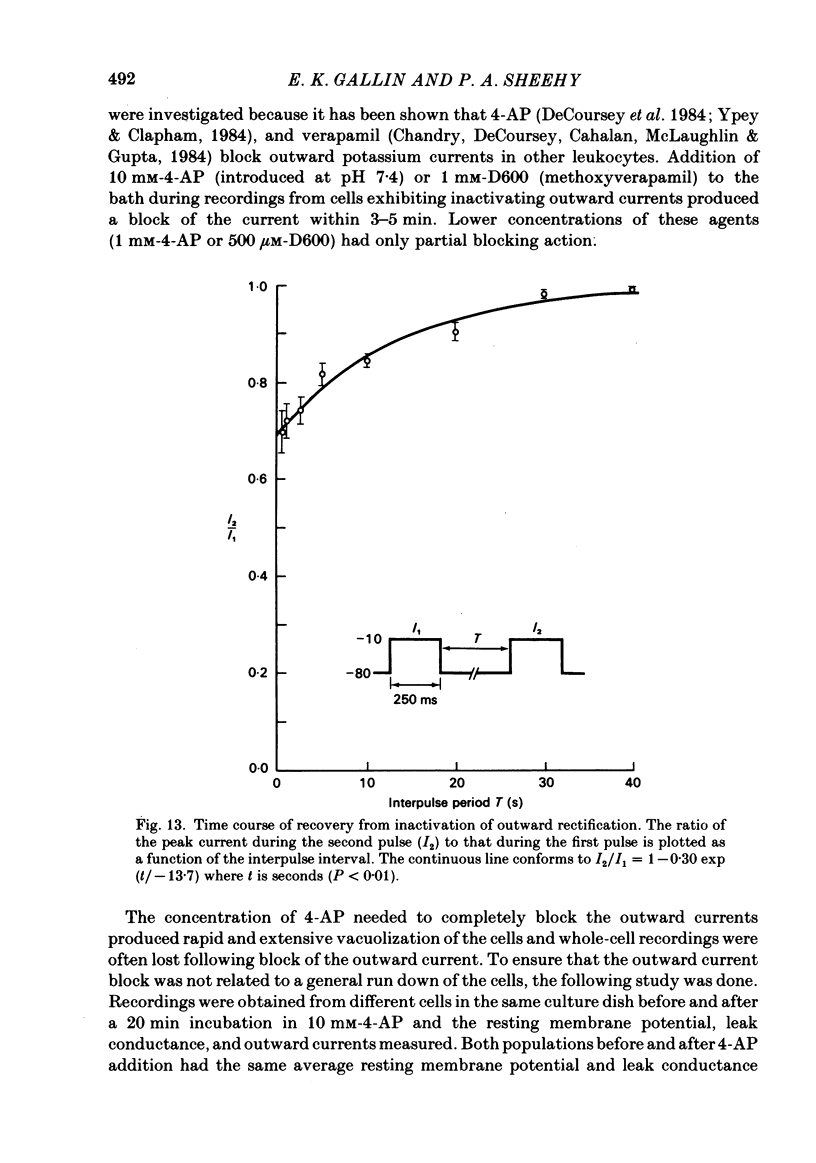
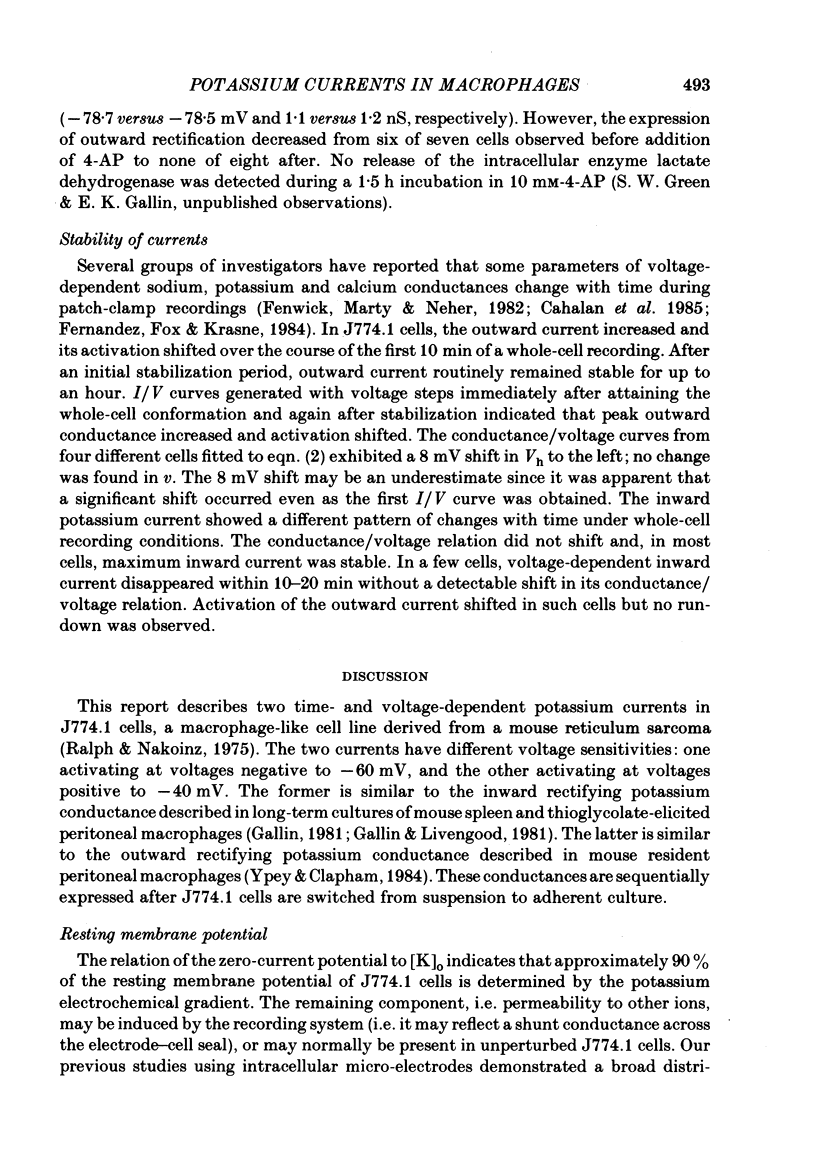
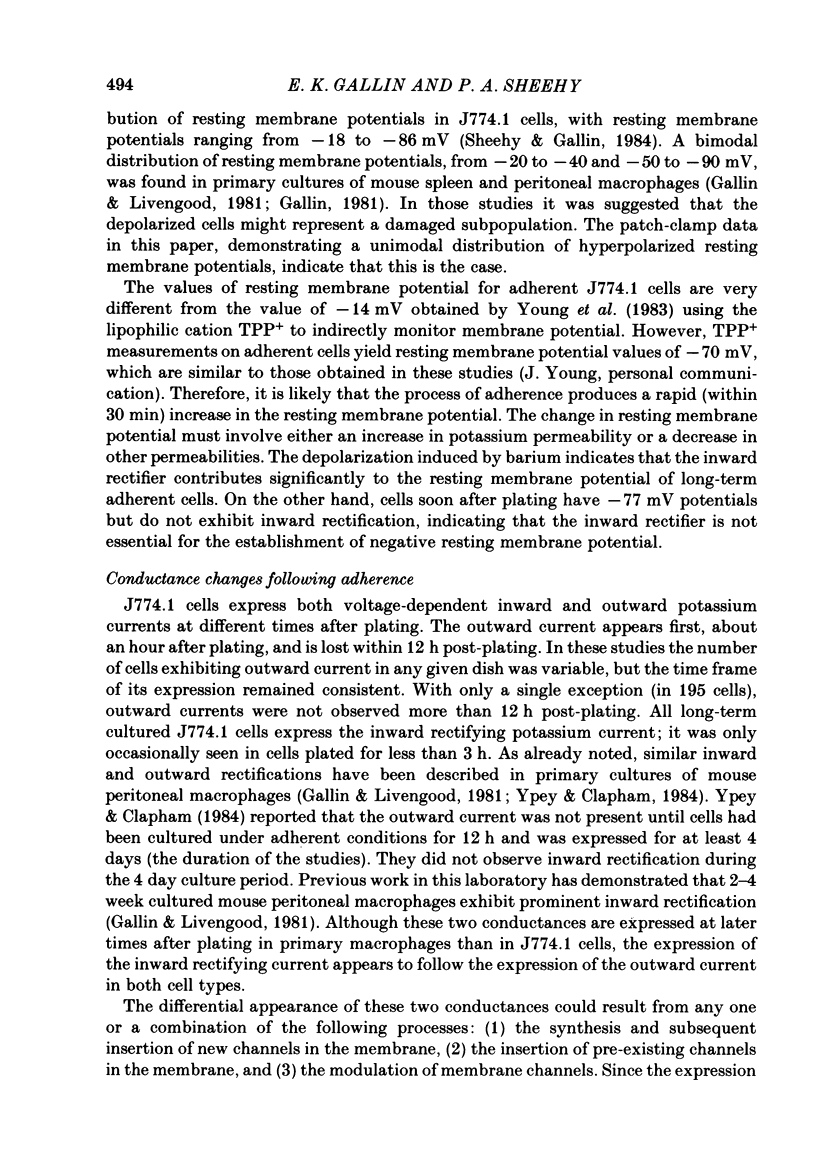
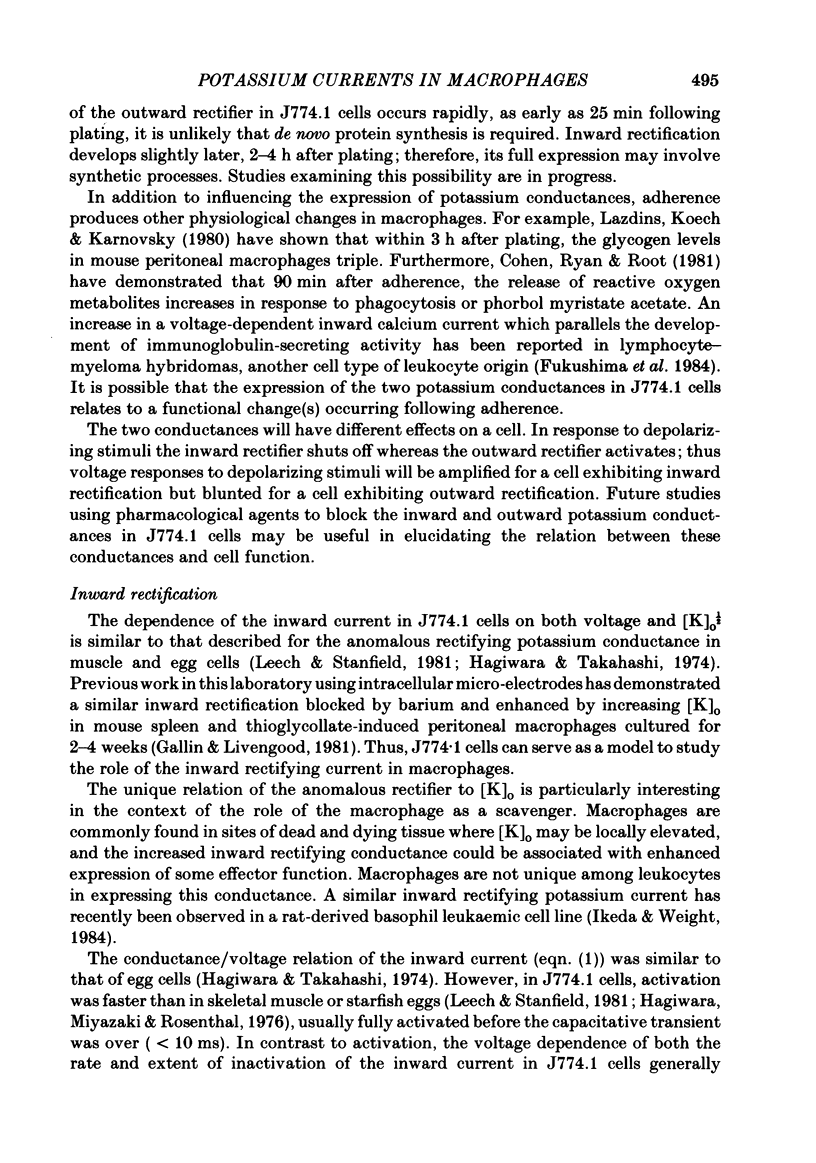
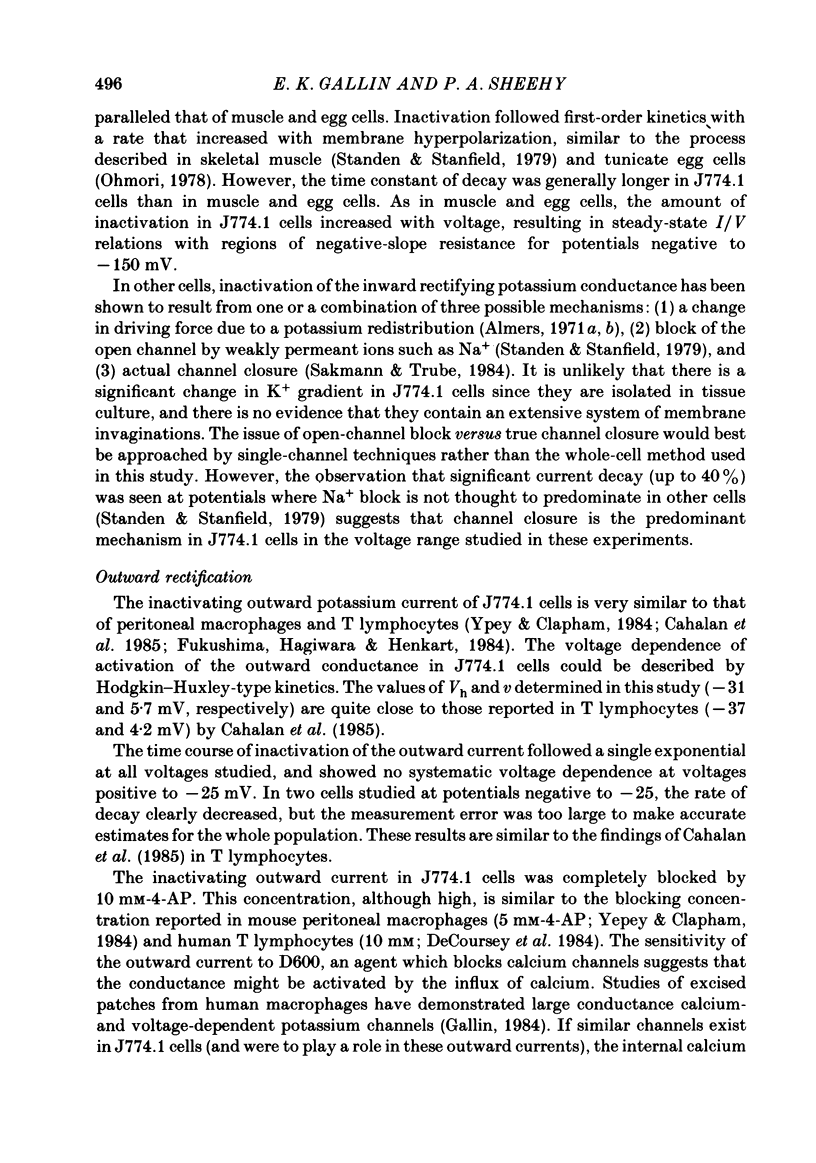
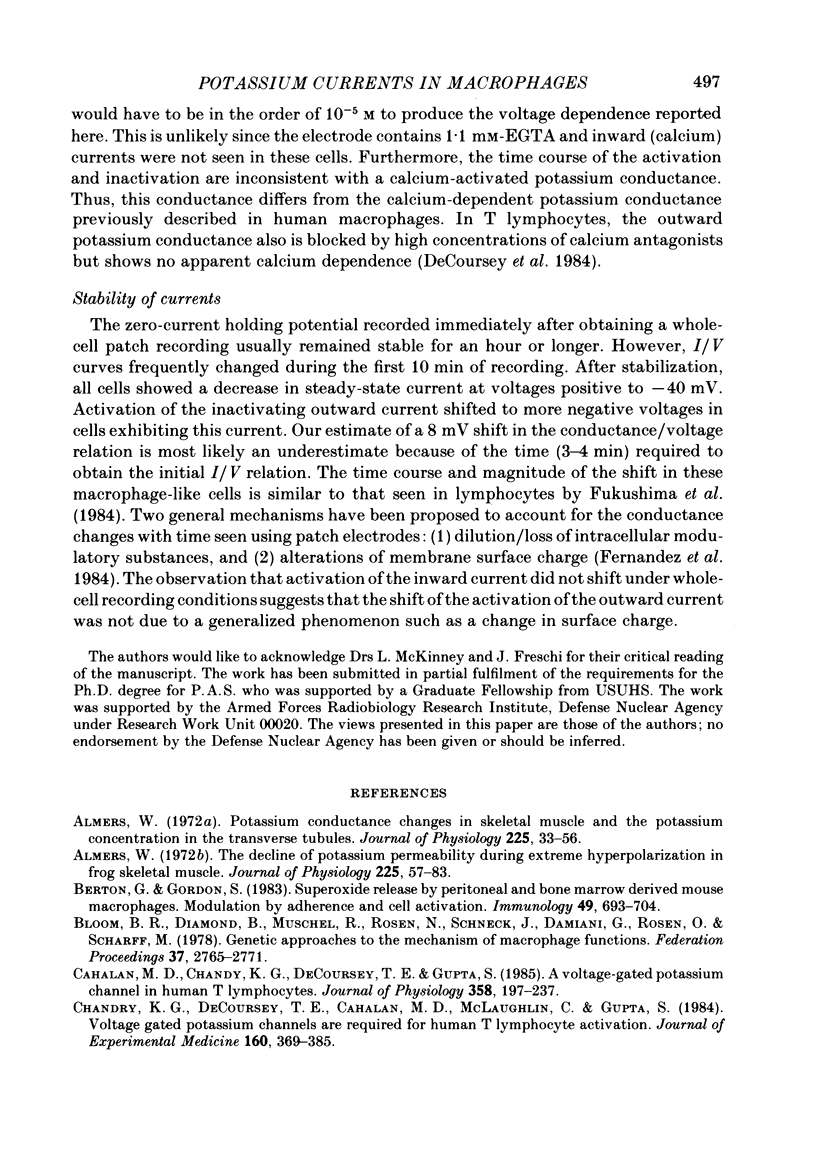
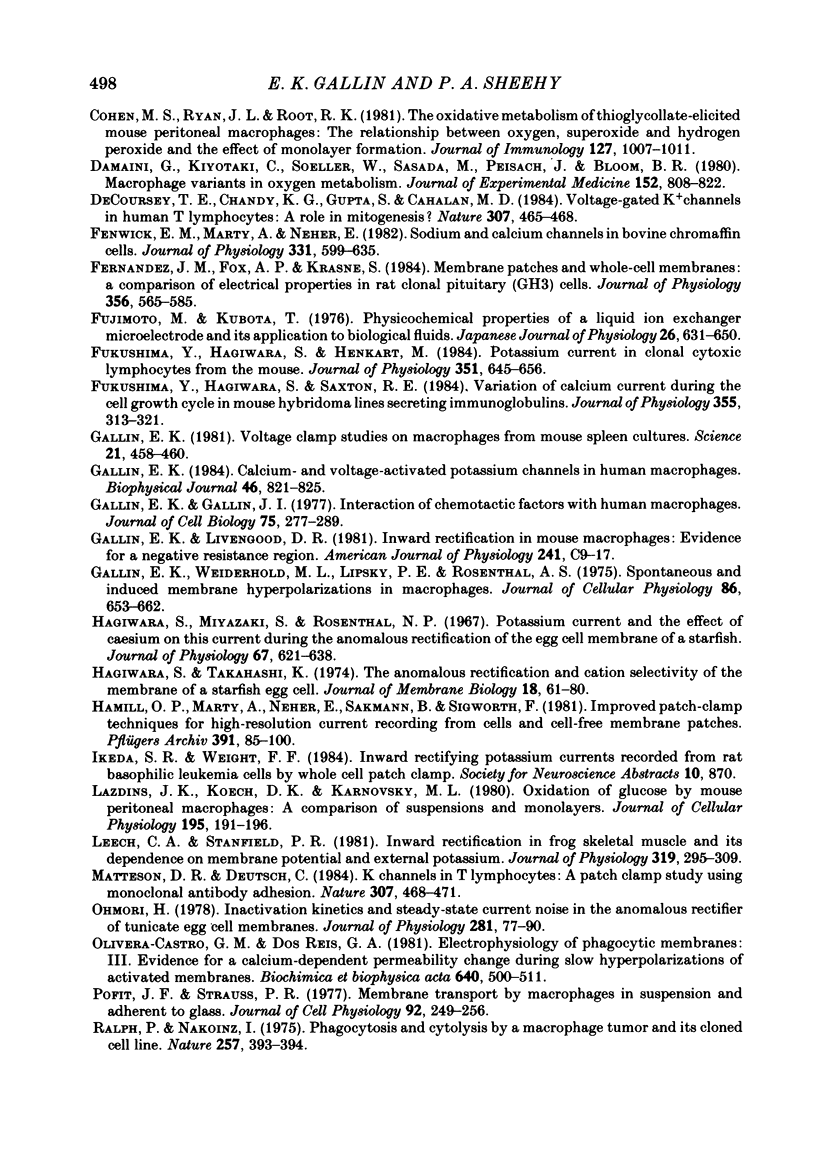
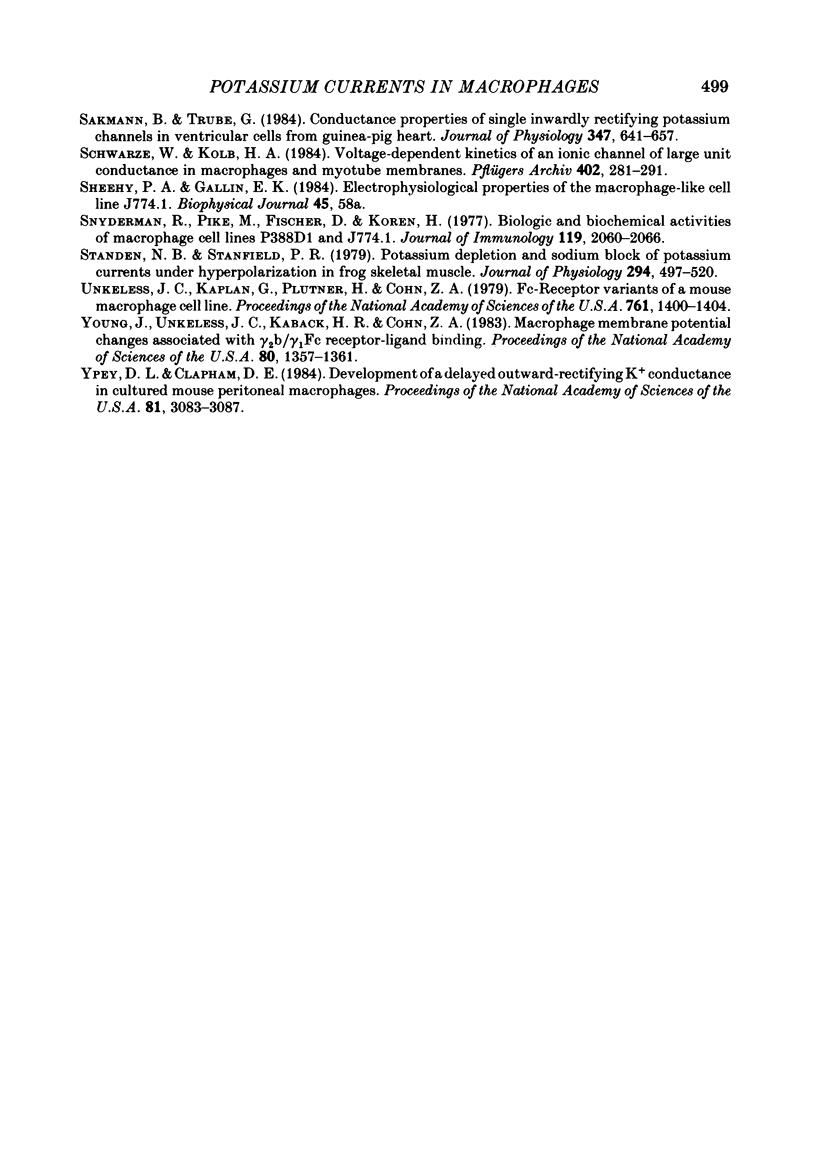
Selected References
These references are in PubMed. This may not be the complete list of references from this article.
- Almers W. Potassium conductance changes in skeletal muscle and the potassium concentration in the transverse tubules. J Physiol. 1972 Aug;225(1):33–56. doi: 10.1113/jphysiol.1972.sp009928. [DOI] [PMC free article] [PubMed] [Google Scholar]
- Almers W. The decline of potassium permeability during extreme hyperpolarization in frog skeletal muscle. J Physiol. 1972 Aug;225(1):57–83. doi: 10.1113/jphysiol.1972.sp009929. [DOI] [PMC free article] [PubMed] [Google Scholar]
- Berton G., Gordon S. Superoxide release by peritoneal and bone marrow-derived mouse macrophages. Modulation by adherence and cell activation. Immunology. 1983 Aug;49(4):693–704. [PMC free article] [PubMed] [Google Scholar]
- Bloom B. R., Diamond B., Muschel R., Rosen N., Schneck J., Damiani G., Rosen O., Scharff M. Genetic approaches to the mechanism of macrophage functions. Fed Proc. 1978 Nov;37(13):2765–2771. [PubMed] [Google Scholar]
- Cahalan M. D., Chandy K. G., DeCoursey T. E., Gupta S. A voltage-gated potassium channel in human T lymphocytes. J Physiol. 1985 Jan;358:197–237. doi: 10.1113/jphysiol.1985.sp015548. [DOI] [PMC free article] [PubMed] [Google Scholar]
- Chandy K. G., DeCoursey T. E., Cahalan M. D., McLaughlin C., Gupta S. Voltage-gated potassium channels are required for human T lymphocyte activation. J Exp Med. 1984 Aug 1;160(2):369–385. doi: 10.1084/jem.160.2.369. [DOI] [PMC free article] [PubMed] [Google Scholar]
- Cohen M. S., Ryan J. L., Root R. K. The oxidative metabolism of thioglycollate-elicited mouse peritoneal macrophages: the relationship between oxygen, superoxide and hydrogen peroxide and the effect of monolayer formation. J Immunol. 1981 Sep;127(3):1007–1011. [PubMed] [Google Scholar]
- Damiani G., Kiyotaki C., Soeller W., Sasada M., Peisach J., Bloom B. R. Macrophage variants in oxygen metabolism. J Exp Med. 1980 Oct 1;152(4):808–822. doi: 10.1084/jem.152.4.808. [DOI] [PMC free article] [PubMed] [Google Scholar]
- DeCoursey T. E., Chandy K. G., Gupta S., Cahalan M. D. Voltage-gated K+ channels in human T lymphocytes: a role in mitogenesis? Nature. 1984 Feb 2;307(5950):465–468. doi: 10.1038/307465a0. [DOI] [PubMed] [Google Scholar]
- Fenwick E. M., Marty A., Neher E. Sodium and calcium channels in bovine chromaffin cells. J Physiol. 1982 Oct;331:599–635. doi: 10.1113/jphysiol.1982.sp014394. [DOI] [PMC free article] [PubMed] [Google Scholar]
- Fernandez J. M., Fox A. P., Krasne S. Membrane patches and whole-cell membranes: a comparison of electrical properties in rat clonal pituitary (GH3) cells. J Physiol. 1984 Nov;356:565–585. doi: 10.1113/jphysiol.1984.sp015483. [DOI] [PMC free article] [PubMed] [Google Scholar]
- Fujimoto M., Kubota T. Physicochemical properties of a liquid ion exchanger microelectrode and its application to biological fluids. Jpn J Physiol. 1976;26(6):631–650. doi: 10.2170/jjphysiol.26.631. [DOI] [PubMed] [Google Scholar]
- Fukushima Y., Hagiwara S., Henkart M. Potassium current in clonal cytotoxic T lymphocytes from the mouse. J Physiol. 1984 Jun;351:645–656. doi: 10.1113/jphysiol.1984.sp015268. [DOI] [PMC free article] [PubMed] [Google Scholar]
- Fukushima Y., Hagiwara S., Saxton R. E. Variation of calcium current during the cell growth cycle in mouse hybridoma lines secreting immunoglobulins. J Physiol. 1984 Oct;355:313–321. doi: 10.1113/jphysiol.1984.sp015421. [DOI] [PMC free article] [PubMed] [Google Scholar]
- Gallin E. K. Calcium- and voltage-activated potassium channels in human macrophages. Biophys J. 1984 Dec;46(6):821–825. doi: 10.1016/S0006-3495(84)84080-1. [DOI] [PMC free article] [PubMed] [Google Scholar]
- Gallin E. K., Gallin J. I. Interaction of chemotactic factors with human macrophages. Induction of transmembrane potential changes. J Cell Biol. 1977 Oct;75(1):277–289. doi: 10.1083/jcb.75.1.277. [DOI] [PMC free article] [PubMed] [Google Scholar]
- Gallin E. K., Livengood D. R. Inward rectification in mouse macrophages: evidence for a negative resistance region. Am J Physiol. 1981 Jul;241(1):C9–17. doi: 10.1152/ajpcell.1981.241.1.C9. [DOI] [PubMed] [Google Scholar]
- Gallin E. K. Voltage clamp studies in macrophages from mouse spleen cultures. Science. 1981 Oct 23;214(4519):458–460. doi: 10.1126/science.7291986. [DOI] [PubMed] [Google Scholar]
- Gallin E. K., Wiederhold M. L., Lipsky P. E., Rosenthal A. S. Spontaneous and induced membrane hyperpolarizations in macrophages. J Cell Physiol. 1975 Dec;86 (Suppl 2)(3 Pt 2):653–661. doi: 10.1002/jcp.1040860510. [DOI] [PubMed] [Google Scholar]
- Hagiwara S., Miyazaki S., Rosenthal N. P. Potassium current and the effect of cesium on this current during anomalous rectification of the egg cell membrane of a starfish. J Gen Physiol. 1976 Jun;67(6):621–638. doi: 10.1085/jgp.67.6.621. [DOI] [PMC free article] [PubMed] [Google Scholar]
- Hagiwara S., Takahashi K. The anomalous rectification and cation selectivity of the membrane of a starfish egg cell. J Membr Biol. 1974;18(1):61–80. doi: 10.1007/BF01870103. [DOI] [PubMed] [Google Scholar]
- Hamill O. P., Marty A., Neher E., Sakmann B., Sigworth F. J. Improved patch-clamp techniques for high-resolution current recording from cells and cell-free membrane patches. Pflugers Arch. 1981 Aug;391(2):85–100. doi: 10.1007/BF00656997. [DOI] [PubMed] [Google Scholar]
- Lazdins J. K., Koech D. K., Karnovsky M. L. Oxidation of glucose by mouse peritoneal macrophages: a comparison of suspensions and monolayers. J Cell Physiol. 1980 Nov;105(2):191–196. doi: 10.1002/jcp.1041050202. [DOI] [PubMed] [Google Scholar]
- Leech C. A., Stanfield P. R. Inward rectification in frog skeletal muscle fibres and its dependence on membrane potential and external potassium. J Physiol. 1981;319:295–309. doi: 10.1113/jphysiol.1981.sp013909. [DOI] [PMC free article] [PubMed] [Google Scholar]
- Matteson D. R., Deutsch C. K channels in T lymphocytes: a patch clamp study using monoclonal antibody adhesion. Nature. 1984 Feb 2;307(5950):468–471. doi: 10.1038/307468a0. [DOI] [PubMed] [Google Scholar]
- Ohmori H. Inactivation kinetics and steady-state current noise in the anomalous rectifier of tunicate egg cell membranes. J Physiol. 1978 Aug;281:77–99. doi: 10.1113/jphysiol.1978.sp012410. [DOI] [PMC free article] [PubMed] [Google Scholar]
- Oliveira-Castro G. M., Dos Reis G. A. Electrophysiology of phagocytic membranes. III. Evidence for a calcium-dependent potassium permeability change during slow hyperpolarizations of activated macrophages. Biochim Biophys Acta. 1981 Jan 22;640(2):500–511. doi: 10.1016/0005-2736(81)90474-0. [DOI] [PubMed] [Google Scholar]
- Pofit J. F., Strauss P. R. Membrane transport by macrophages in suspension and adherent to glass. J Cell Physiol. 1977 Aug;92(2):249–255. doi: 10.1002/jcp.1040920213. [DOI] [PubMed] [Google Scholar]
- Ralph P., Nakoinz I. Phagocytosis and cytolysis by a macrophage tumour and its cloned cell line. Nature. 1975 Oct 2;257(5525):393–394. doi: 10.1038/257393a0. [DOI] [PubMed] [Google Scholar]
- Sakmann B., Trube G. Conductance properties of single inwardly rectifying potassium channels in ventricular cells from guinea-pig heart. J Physiol. 1984 Feb;347:641–657. doi: 10.1113/jphysiol.1984.sp015088. [DOI] [PMC free article] [PubMed] [Google Scholar]
- Schwarze W., Kolb H. A. Voltage-dependent kinetics of an anionic channel of large unit conductance in macrophages and myotube membranes. Pflugers Arch. 1984 Nov;402(3):281–291. doi: 10.1007/BF00585511. [DOI] [PubMed] [Google Scholar]
- Snyderman R., Pike M. C., Fischer D. G., Koren H. S. Biologic and biochemical activities of continuous macrophage cell lines P388D1 and J774.1. J Immunol. 1977 Dec;119(6):2060–2066. [PubMed] [Google Scholar]
- Standen N. B., Stanfield P. R. Potassium depletion and sodium block of potassium currents under hyperpolarization in frog sartorius muscle. J Physiol. 1979 Sep;294:497–520. doi: 10.1113/jphysiol.1979.sp012943. [DOI] [PMC free article] [PubMed] [Google Scholar]
- Unkeless J. C., Kaplan G., Plutner H., Cohn Z. A. Fc-receptor variants of a mouse macrophage cell line. Proc Natl Acad Sci U S A. 1979 Mar;76(3):1400–1404. doi: 10.1073/pnas.76.3.1400. [DOI] [PMC free article] [PubMed] [Google Scholar]
- Ypey D. L., Clapham D. E. Development of a delayed outward-rectifying K+ conductance in cultured mouse peritoneal macrophages. Proc Natl Acad Sci U S A. 1984 May;81(10):3083–3087. doi: 10.1073/pnas.81.10.3083. [DOI] [PMC free article] [PubMed] [Google Scholar]