Abstract
Intracellular recordings were made from turtle cochlear hair cells in order to examine the properties of the post-synaptic potentials evoked by electrical stimulation of the efferent axons. Single shocks to the efferents generated a hair cell membrane hyperpolarization with an average amplitude generally less than 1 mV and lasting for about 100 ms. With short trains of shocks, the size of the post-synaptic potential grew markedly to a maximum of 20-30 mV. The interaction between pairs of shocks separated by a varying interval was studied. For an interval of 4 ms, the response to the second shock was increased on average by a factor of 3 and the conditioning effect of the first shock decayed with a time constant of about 100 ms. We suggest the augmentation in response to trains of shocks may be partly due to facilitation of efferent transmitter release. The efferent post-synaptic potentials could be reversibly abolished by perfusion with perilymphs containing 3 microM-curare or atropine, and infusion of acetylcholine gave a transient membrane hyperpolarization. These observations are consistent with efferent action being mediated via a cholinergic synapse onto the hair cells. The post-synaptic potentials could be reversed in polarity by injection of hyperpolarizing currents through the recording electrode. The reversal potential was estimated as about -80 mV, 30 mV negative to the resting potential. Near reversal, a small brief depolarization was evident and may constitute a minor component of the synaptic response. The value of the reversal potential was unaffected by substitution of the perilymphatic chloride, but was altered in a predictable manner by changes in extracellular potassium concentration indicating that the post-synaptic potentials arise mainly by an increase in the permeability of the hair cell membrane to potassium ions. Throughout the post-synaptic hyperpolarization there was a reduction in the sensitivity of the hair cell to tones at its characteristic frequency. The desensitization, maximal for low sound pressures, varied in different cells from a factor of 1.6 to 28. At the peak of the largest synaptic potentials, the receptor potential remained negative to the resting potential with all but the loudest characteristic frequency tone s. We suggest that there are two factors in efferent inhibition; one a r duction in the receptor potential at the hair cell's characteristic frequency and the other a hyperpolarization of its membrane potential which should reduce the release of excitatory transmitter onto the afferent terminals.
Full text
PDF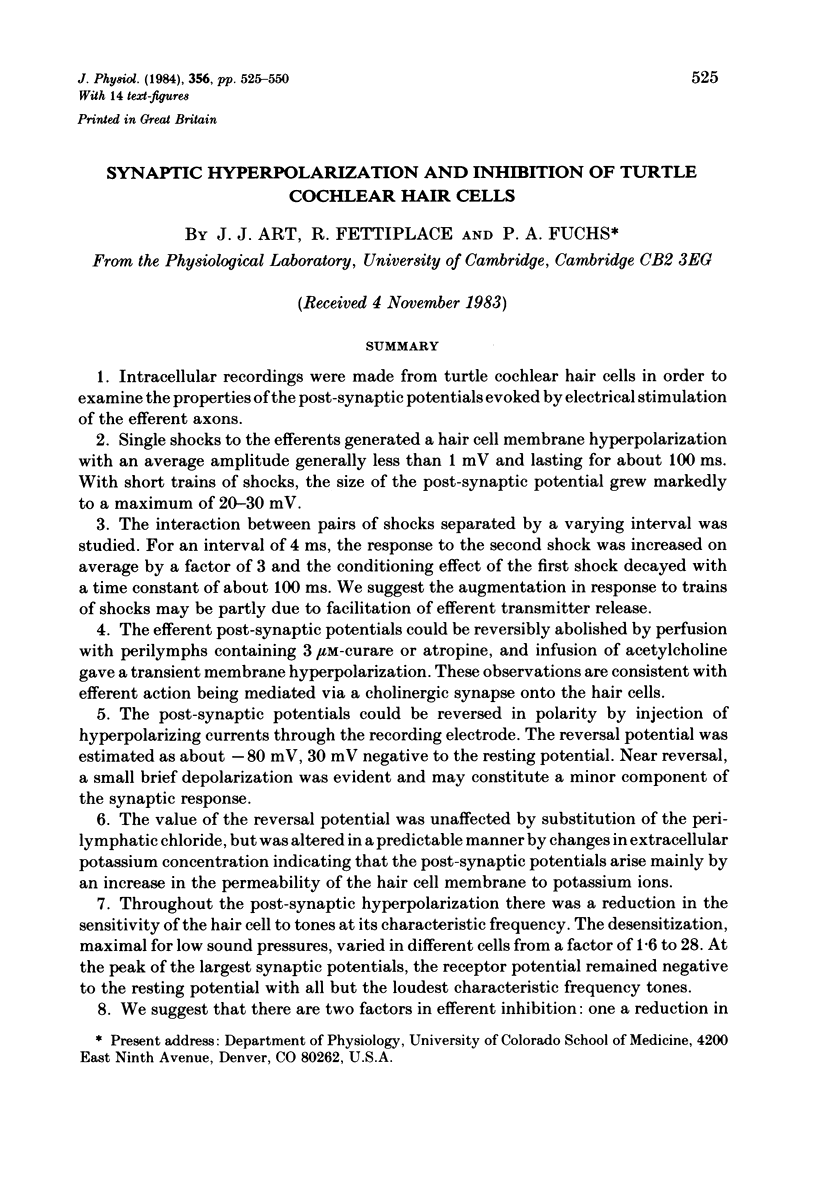
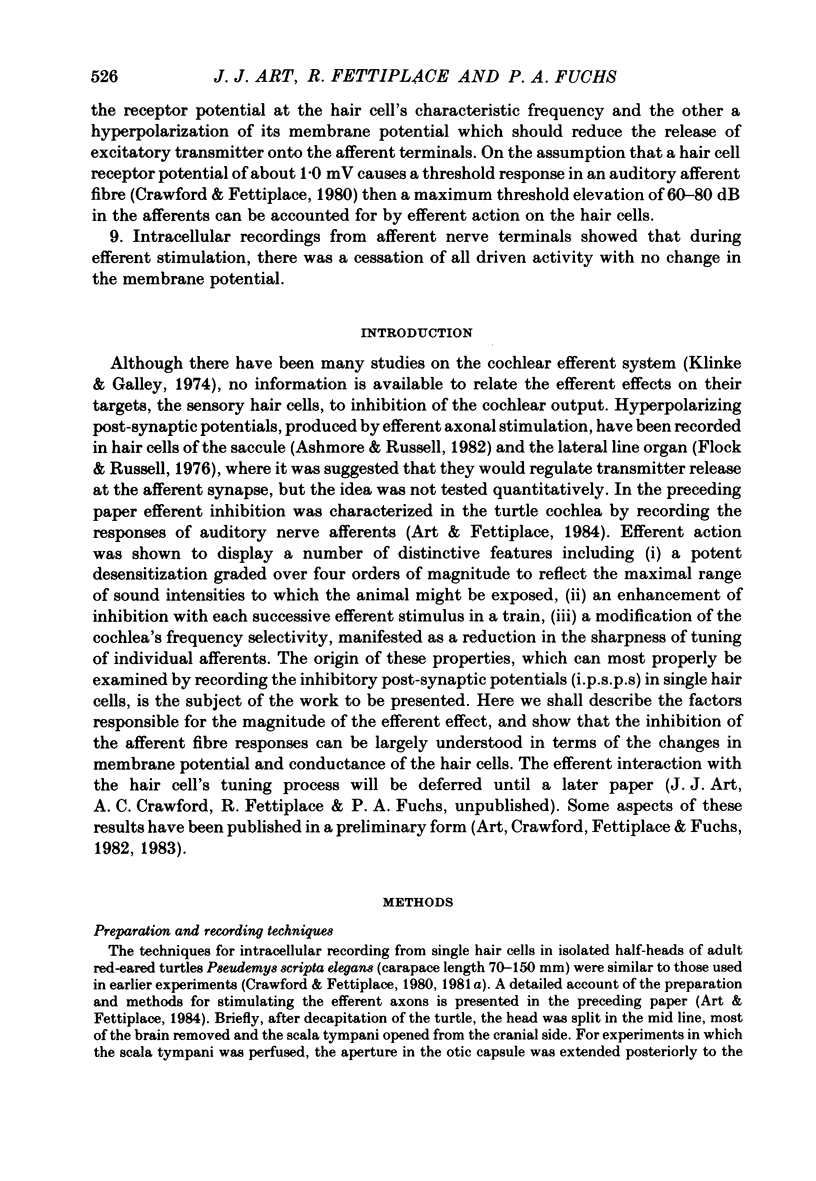
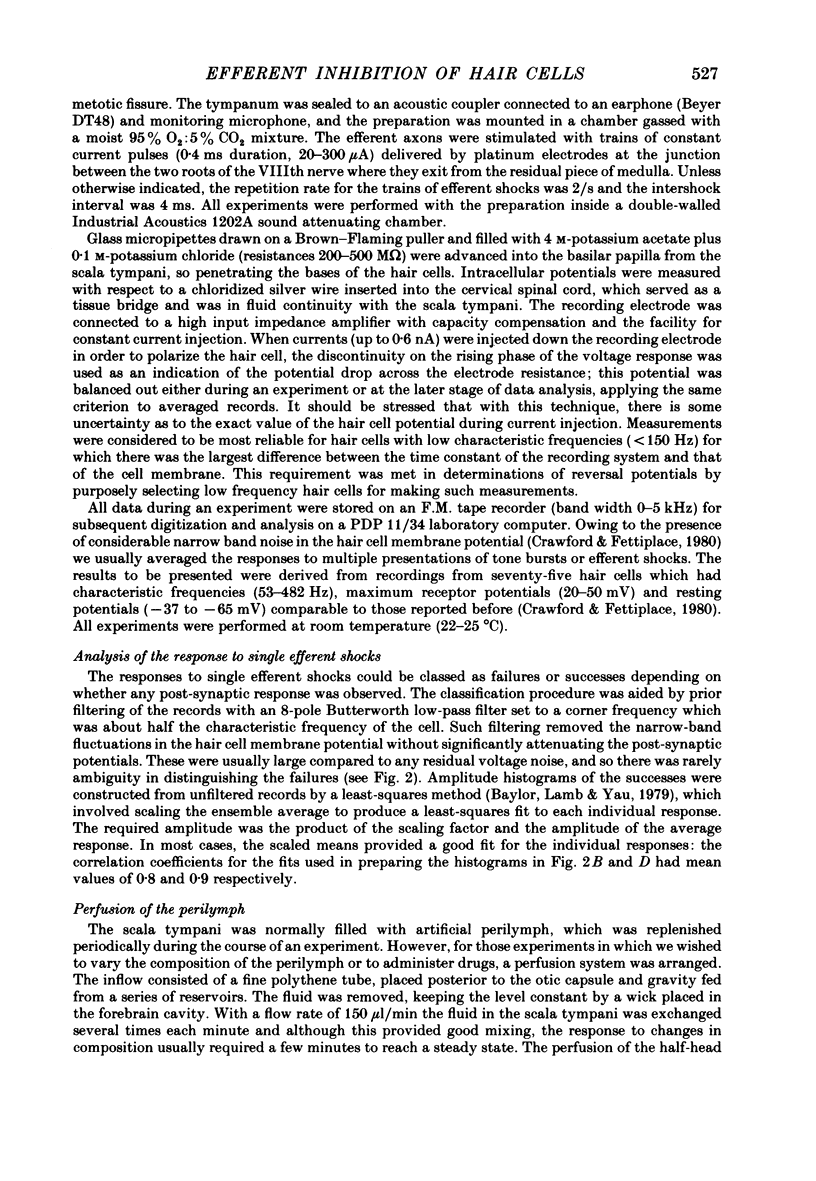
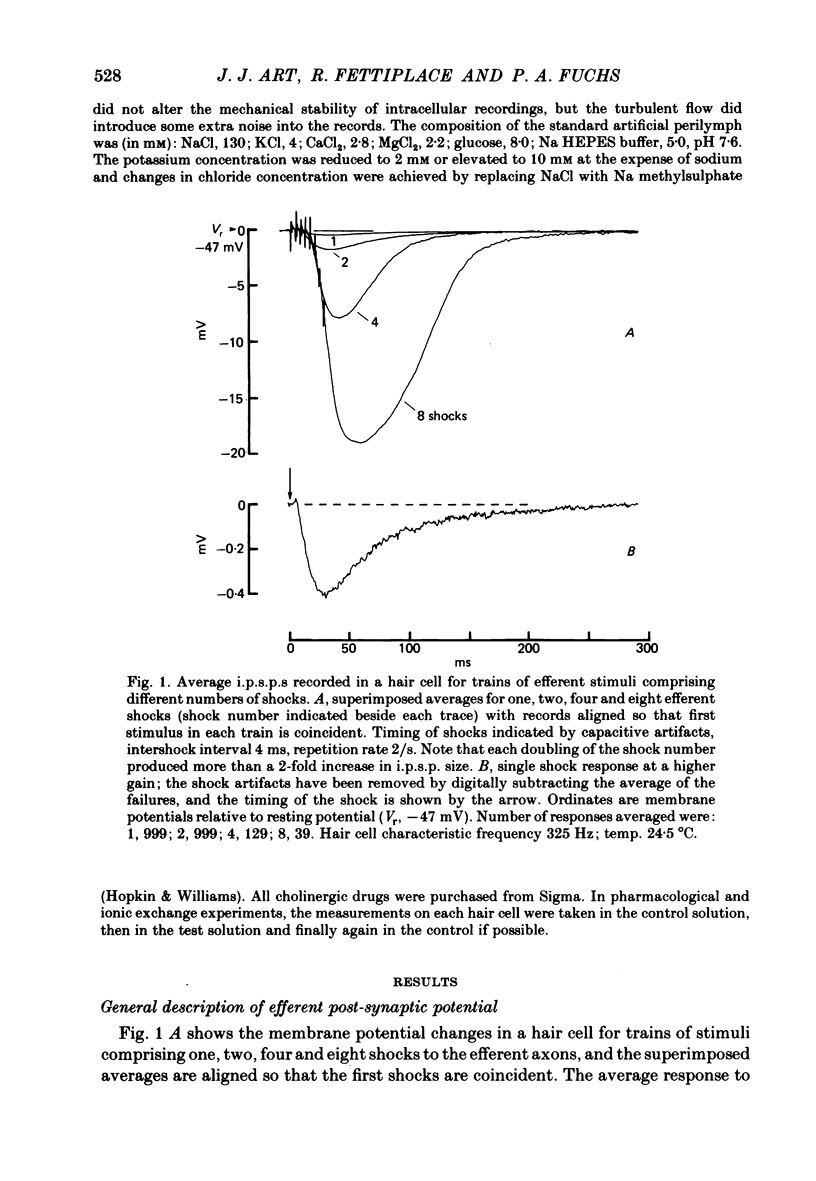
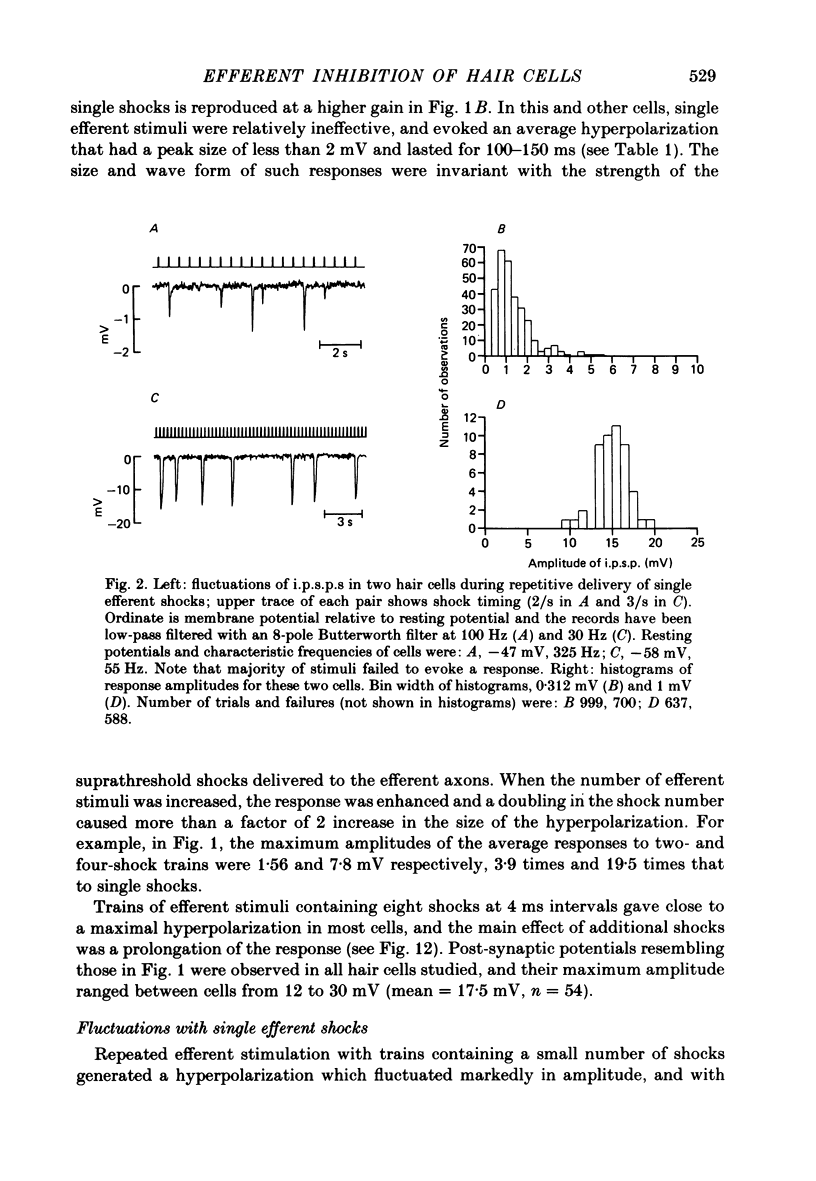
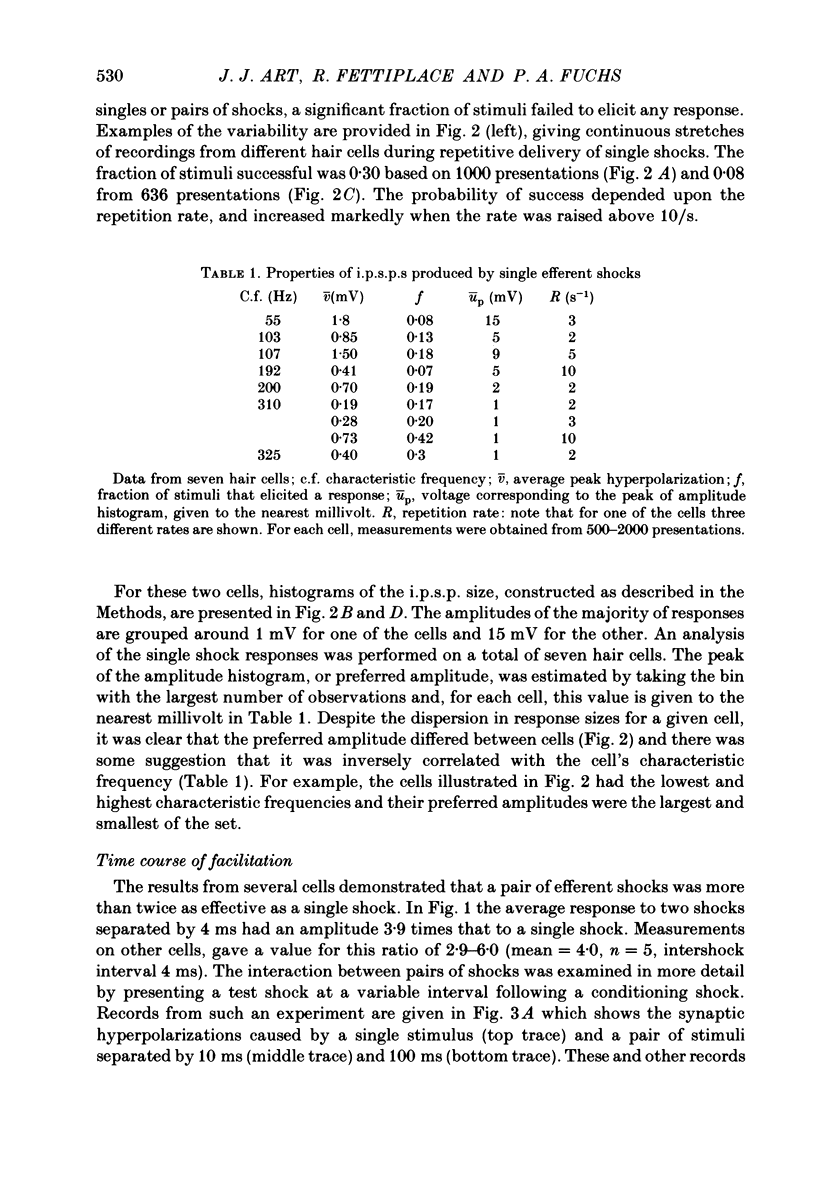
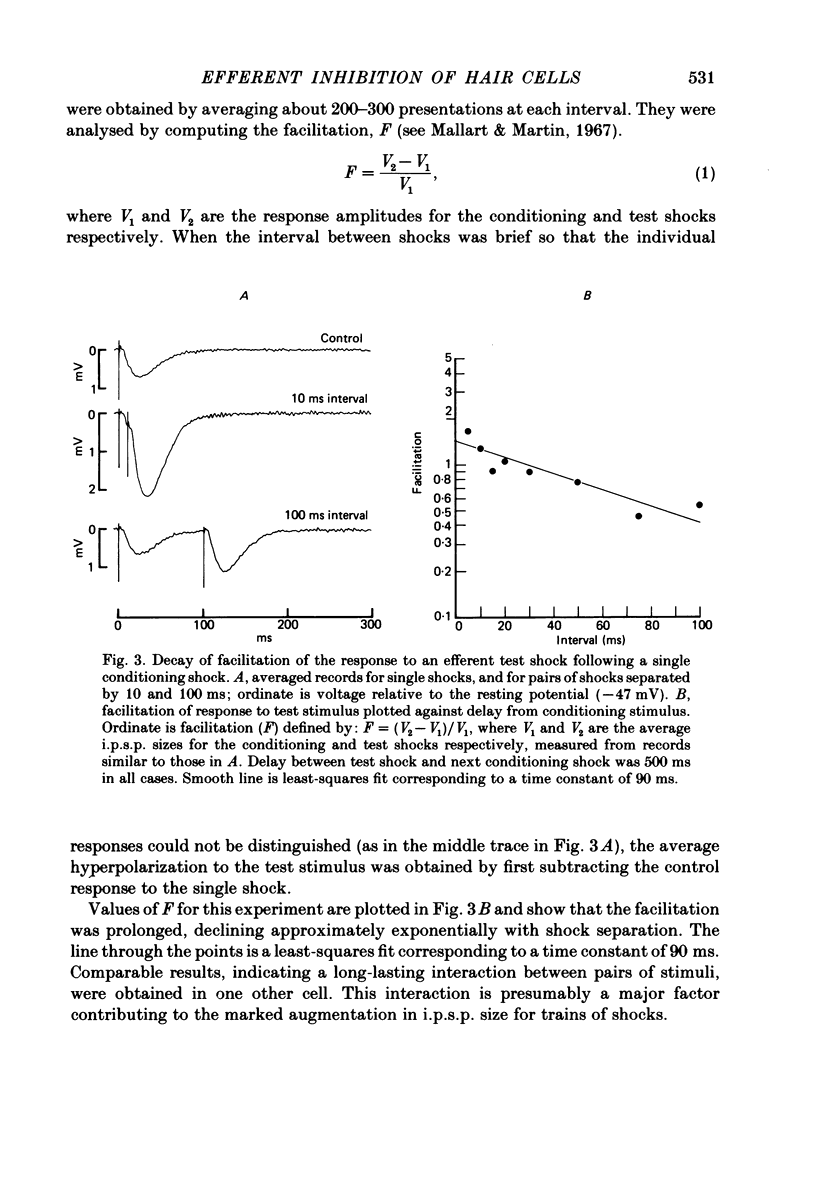
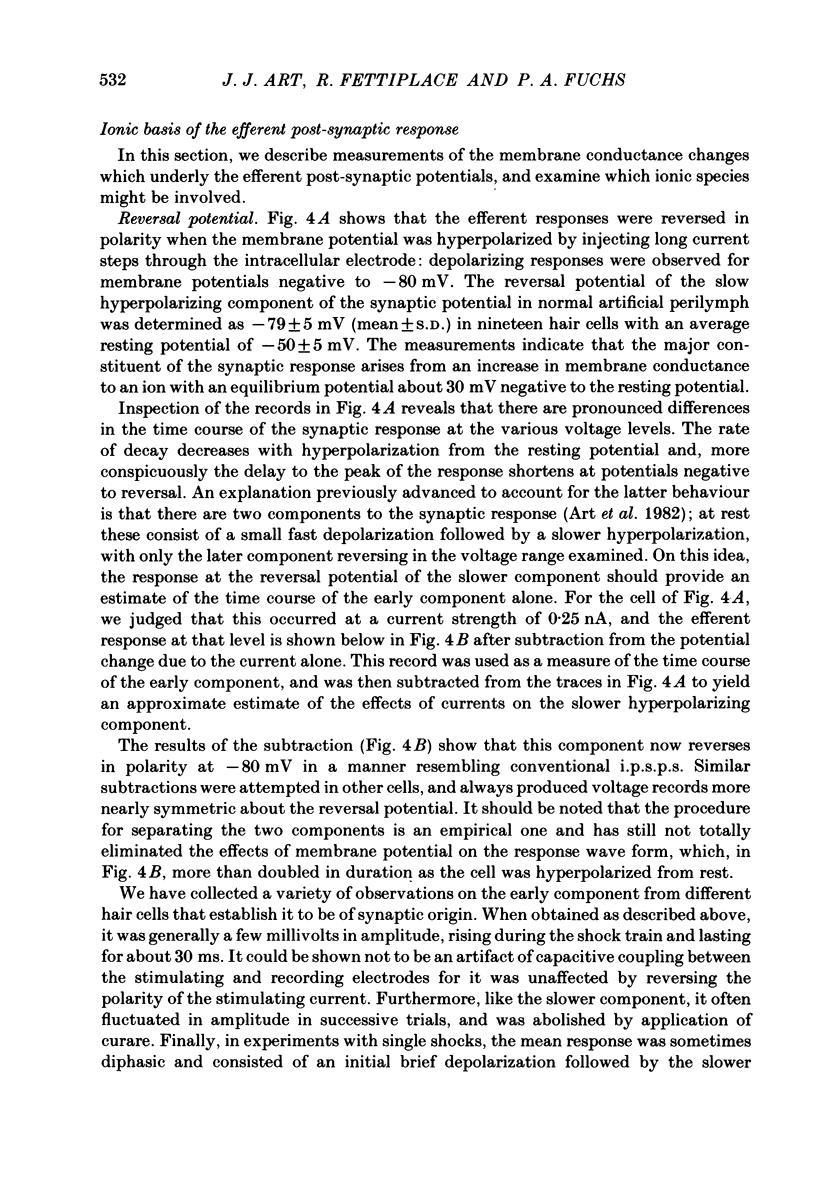
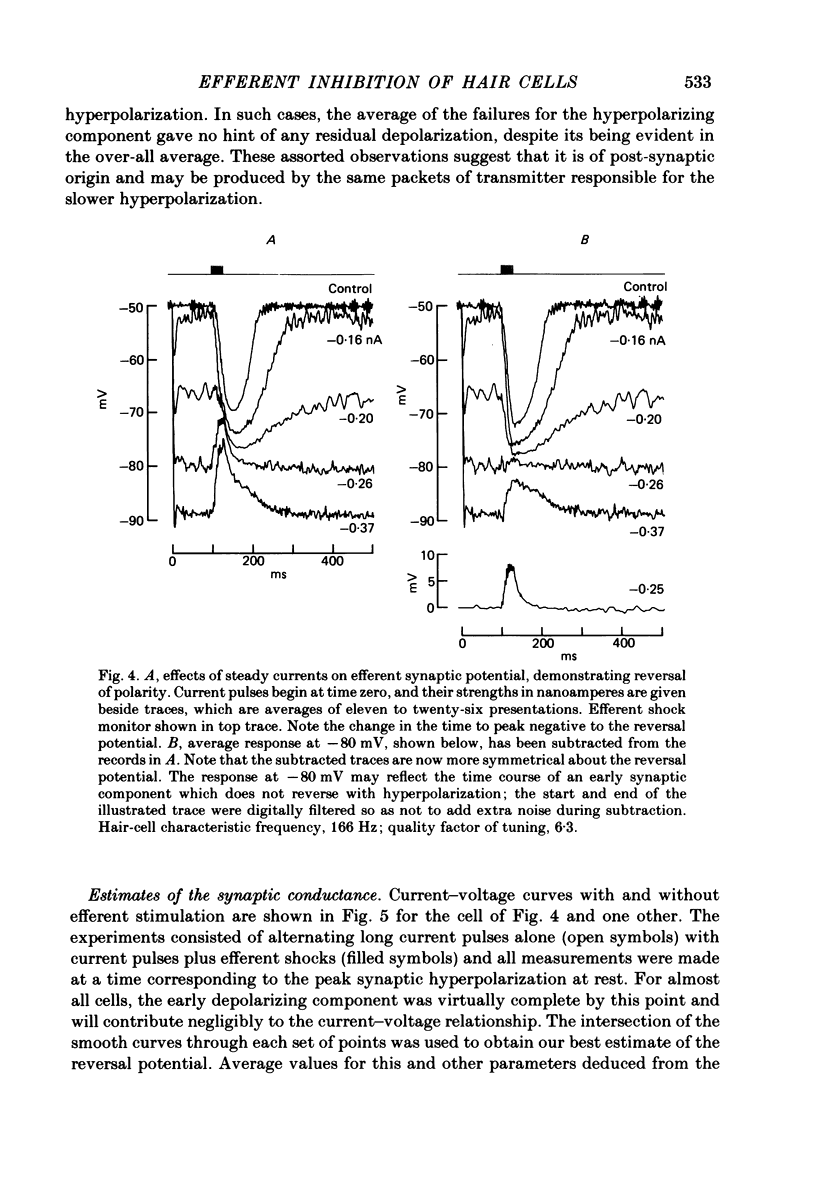
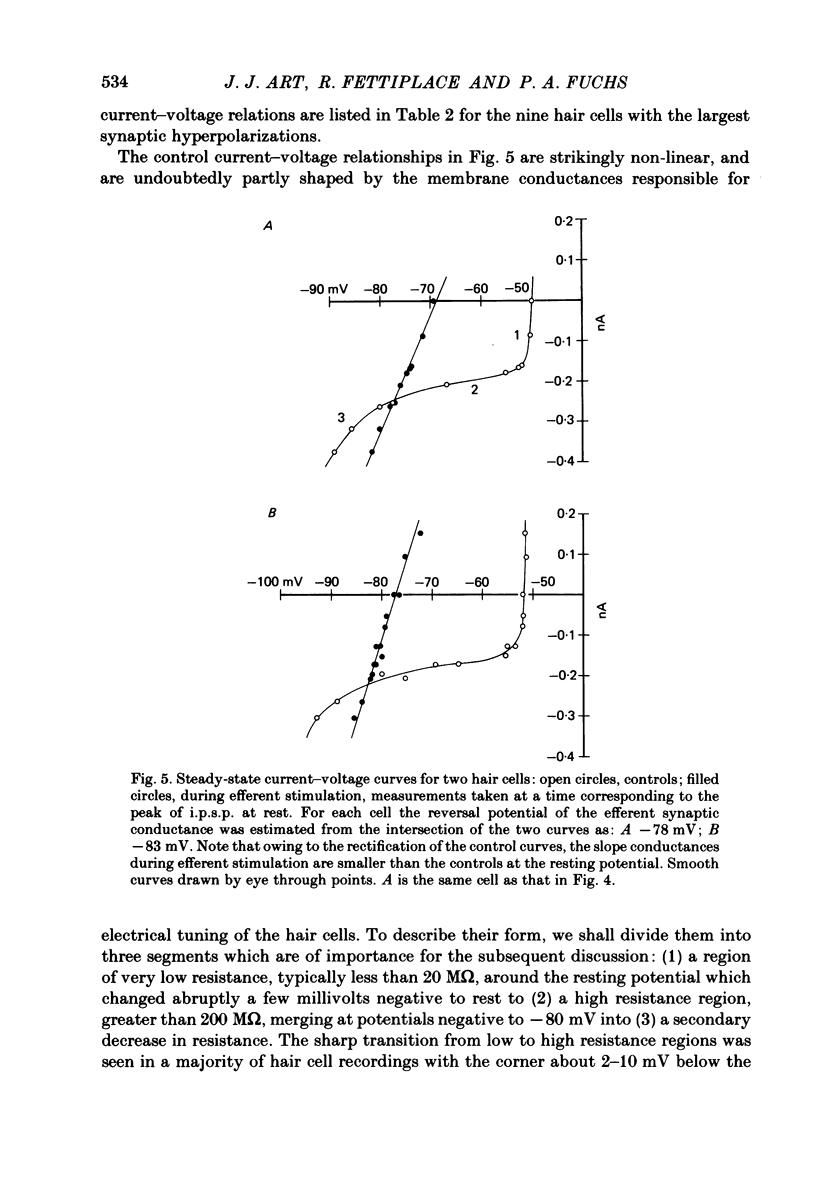
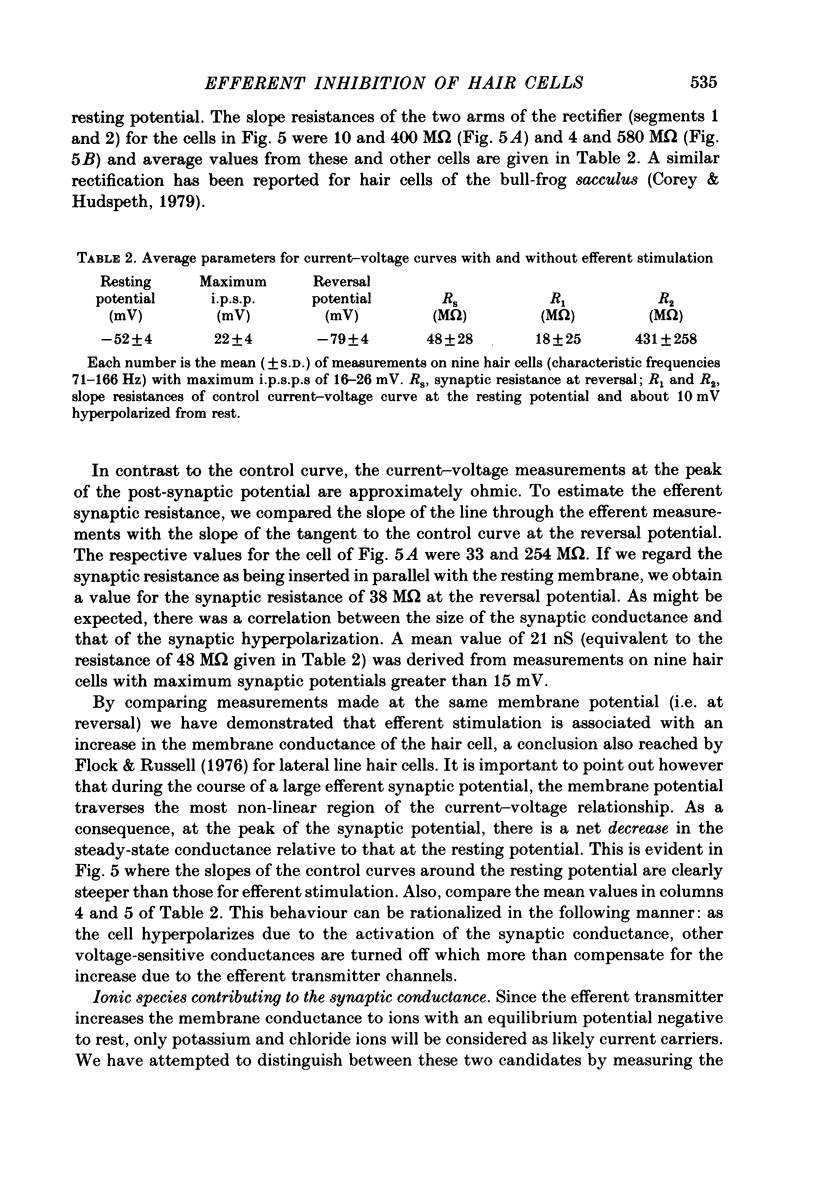
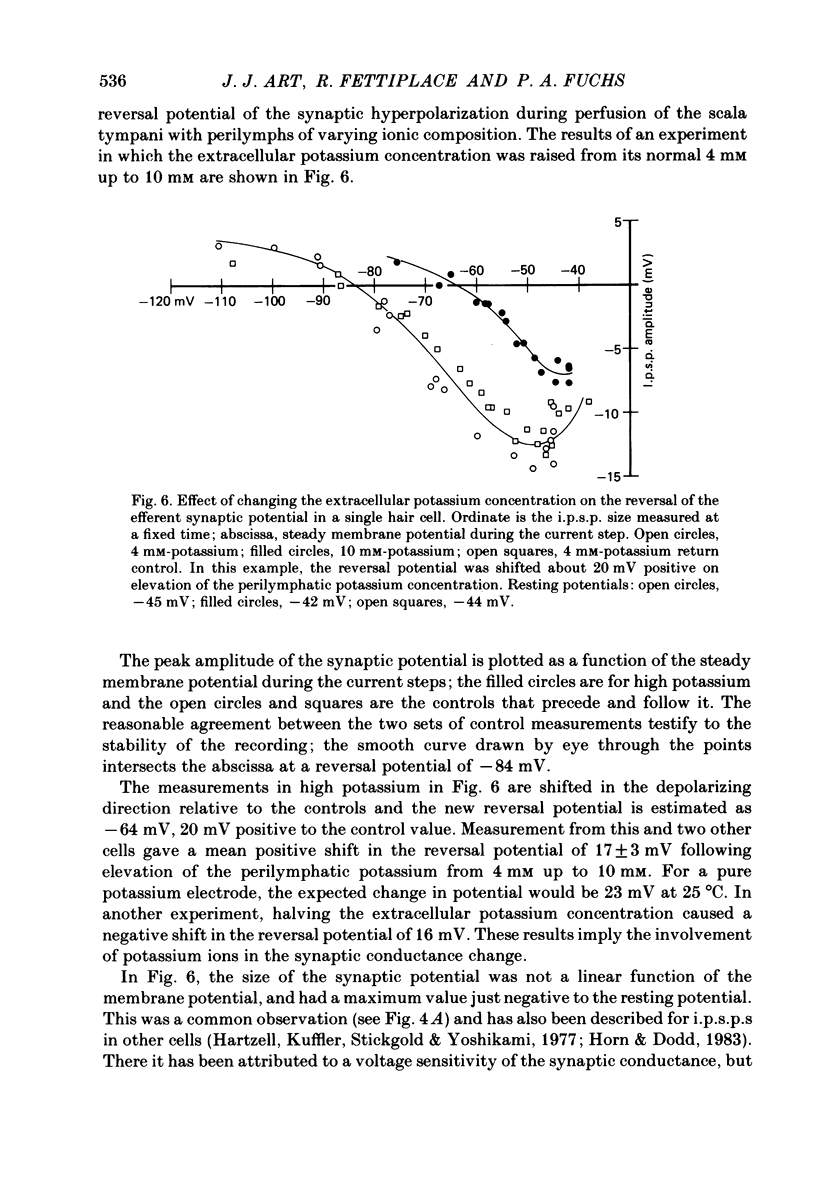
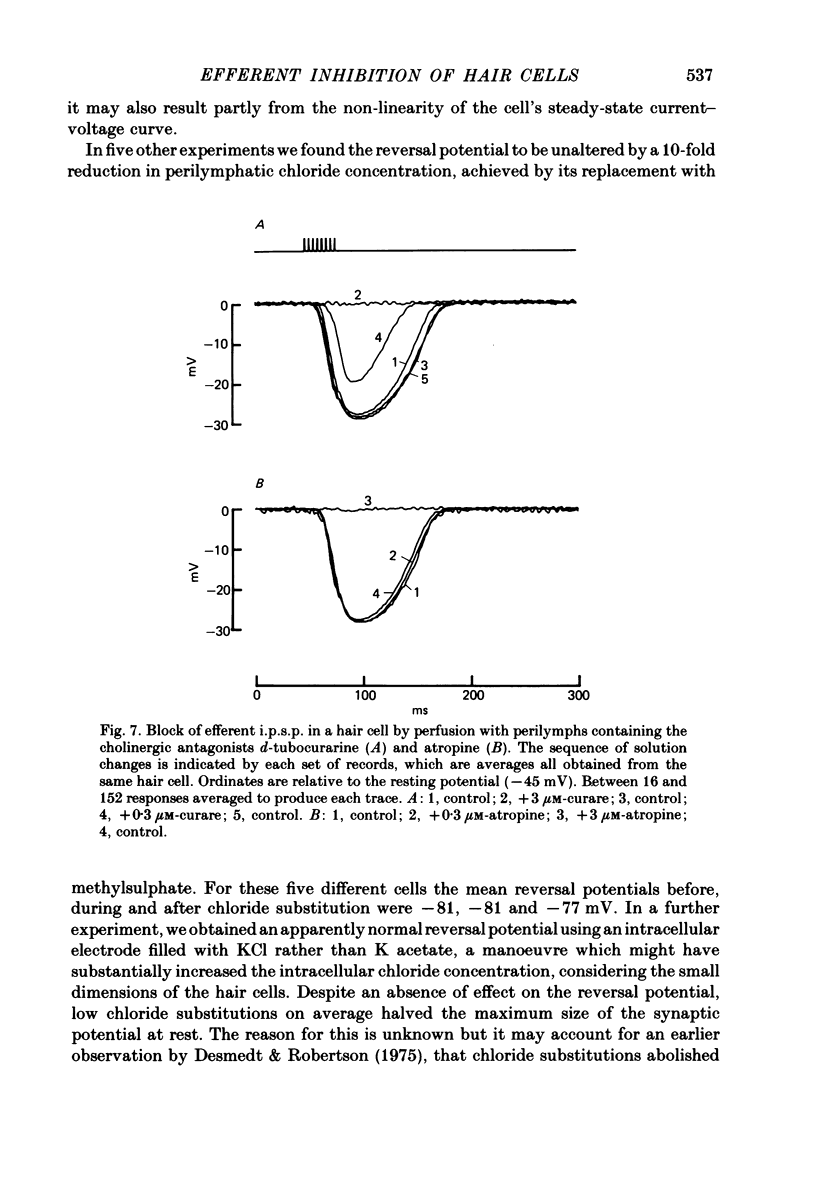
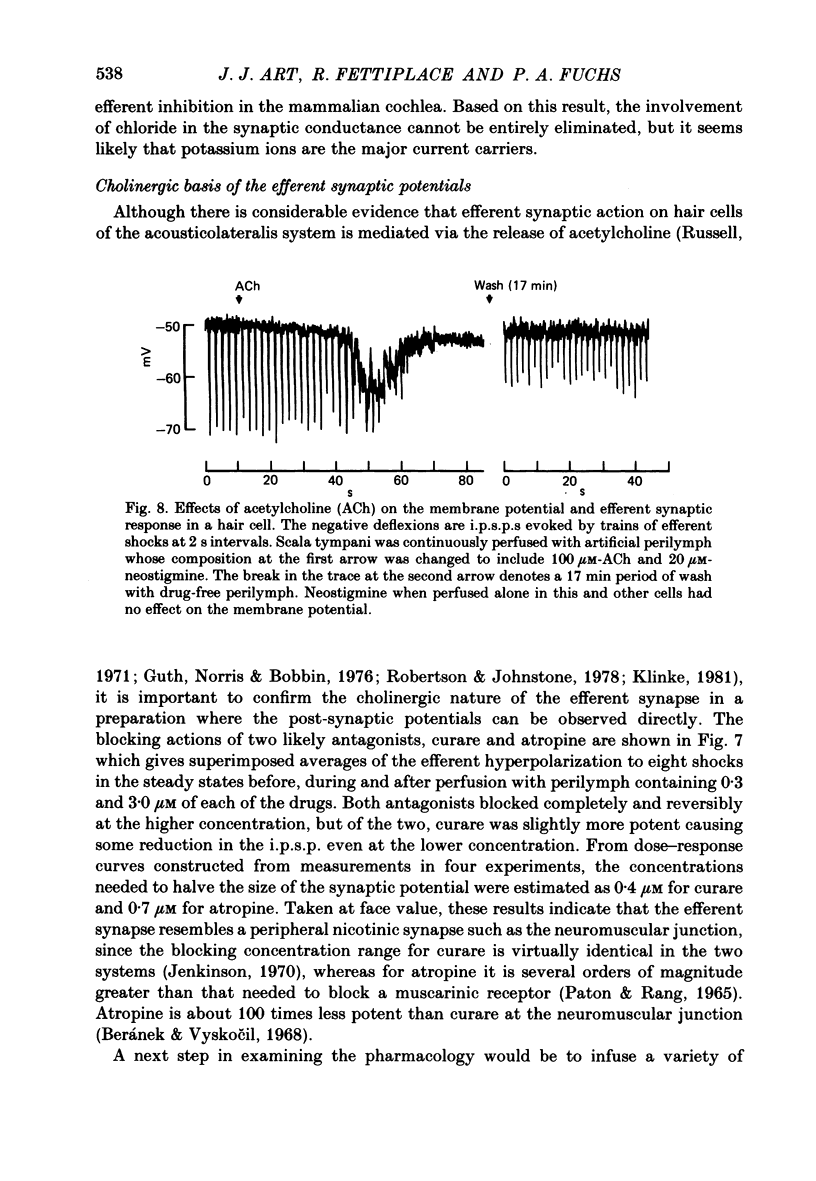
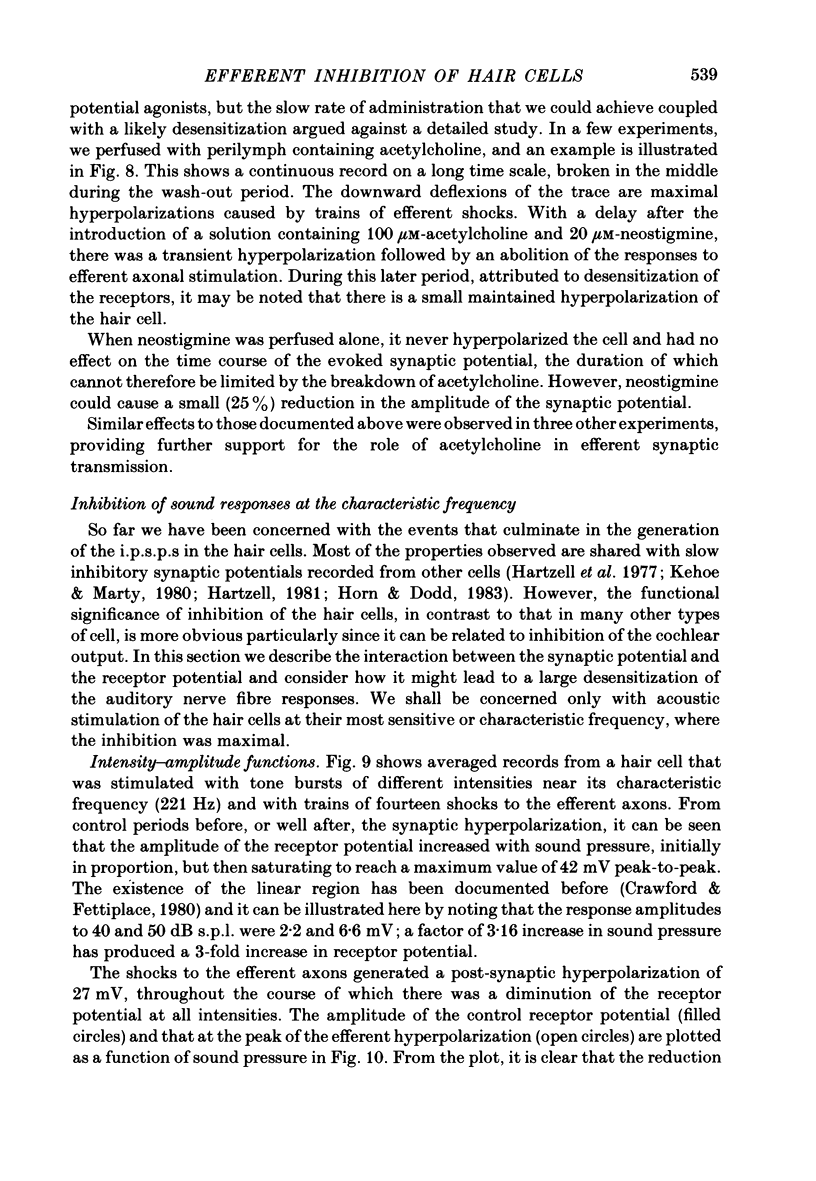
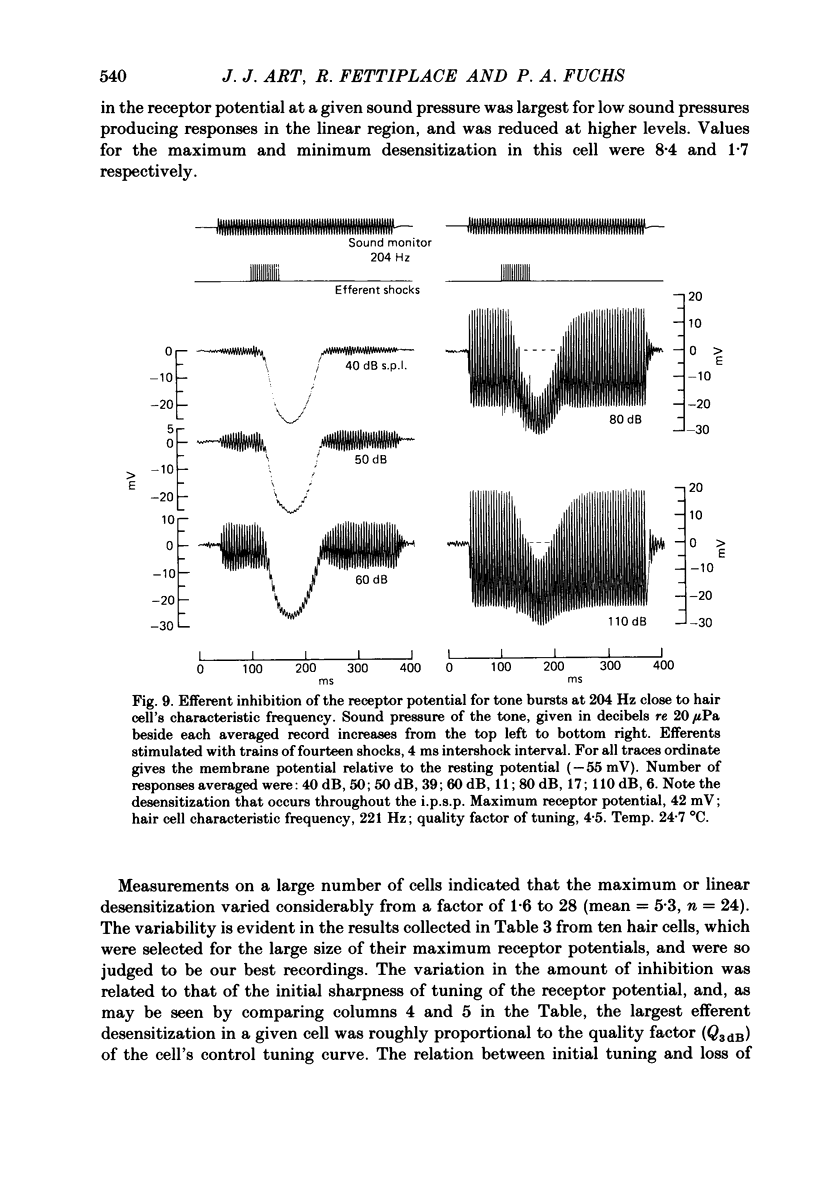
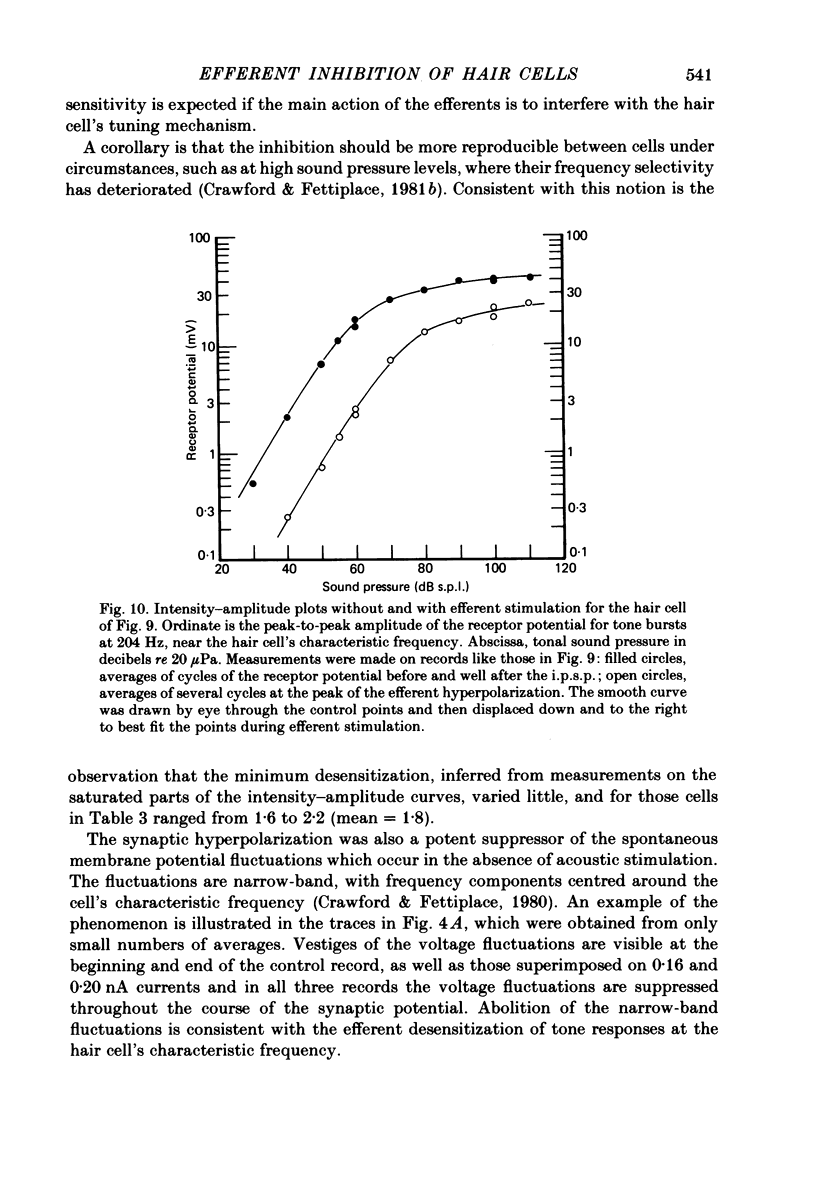
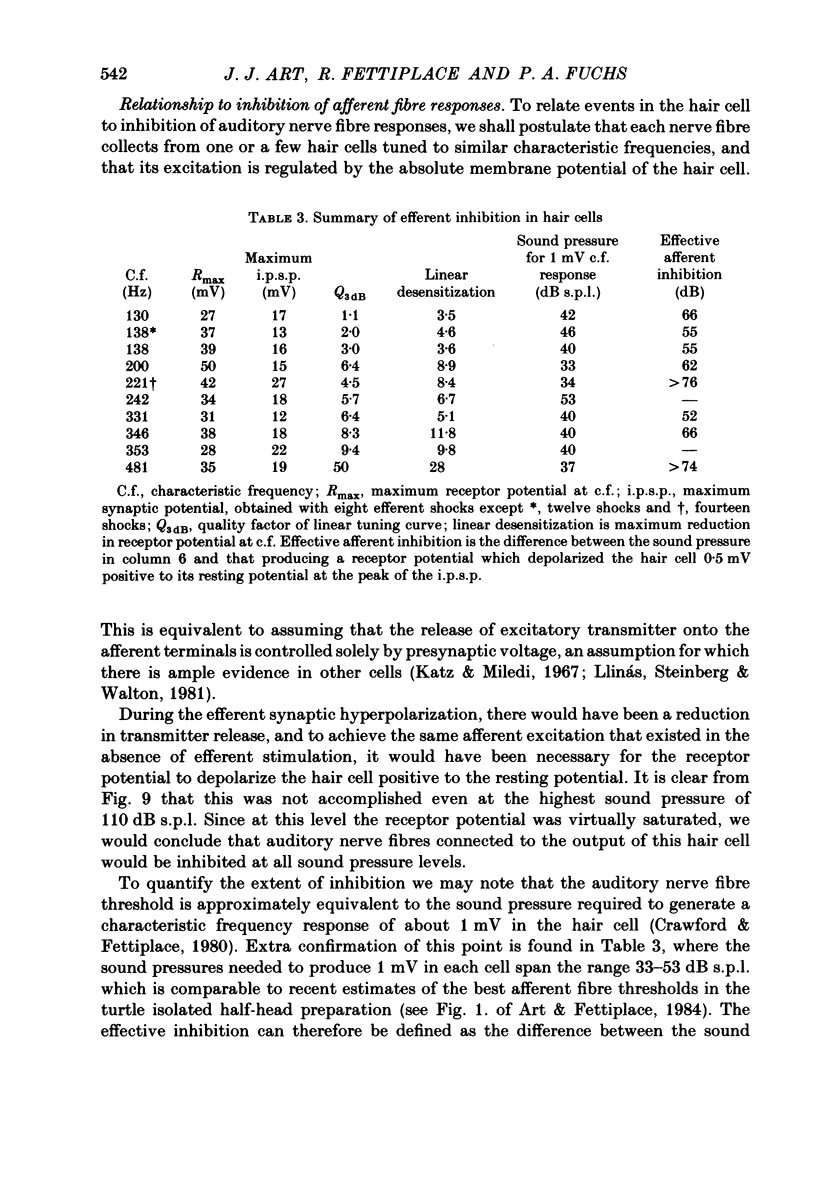
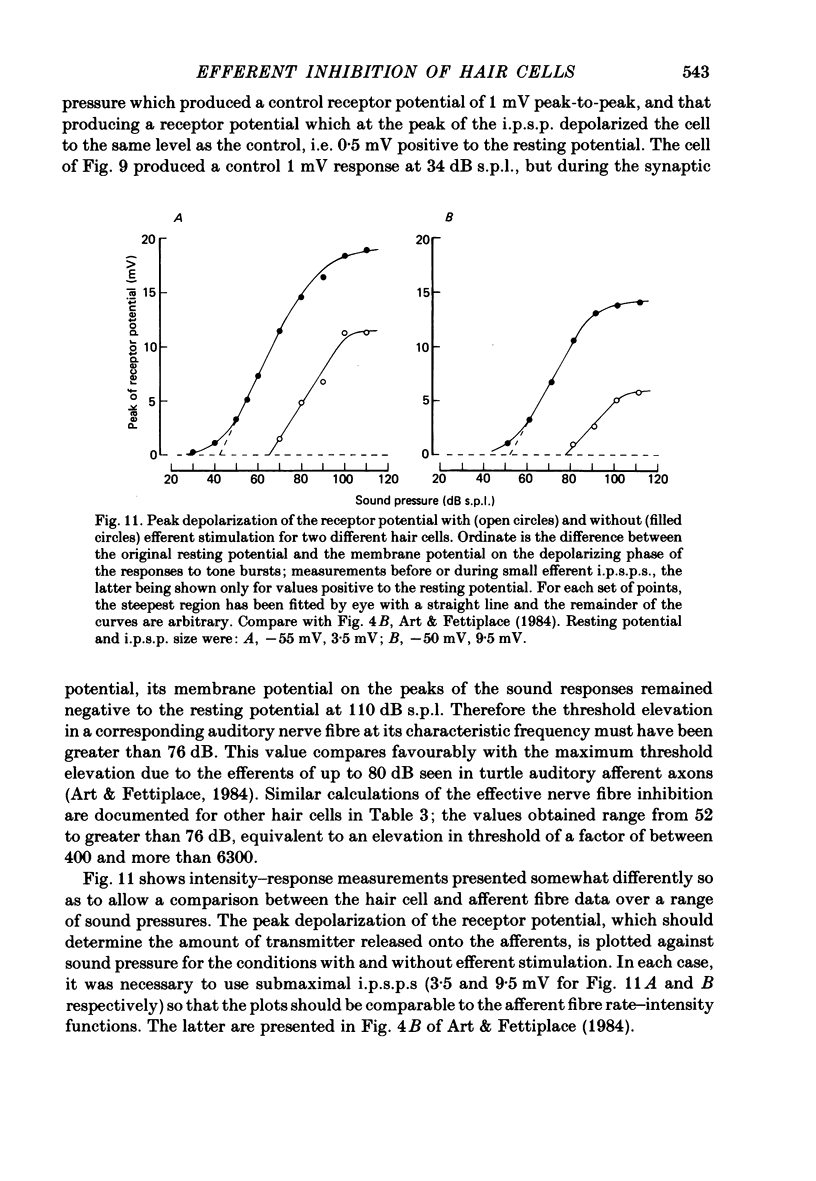
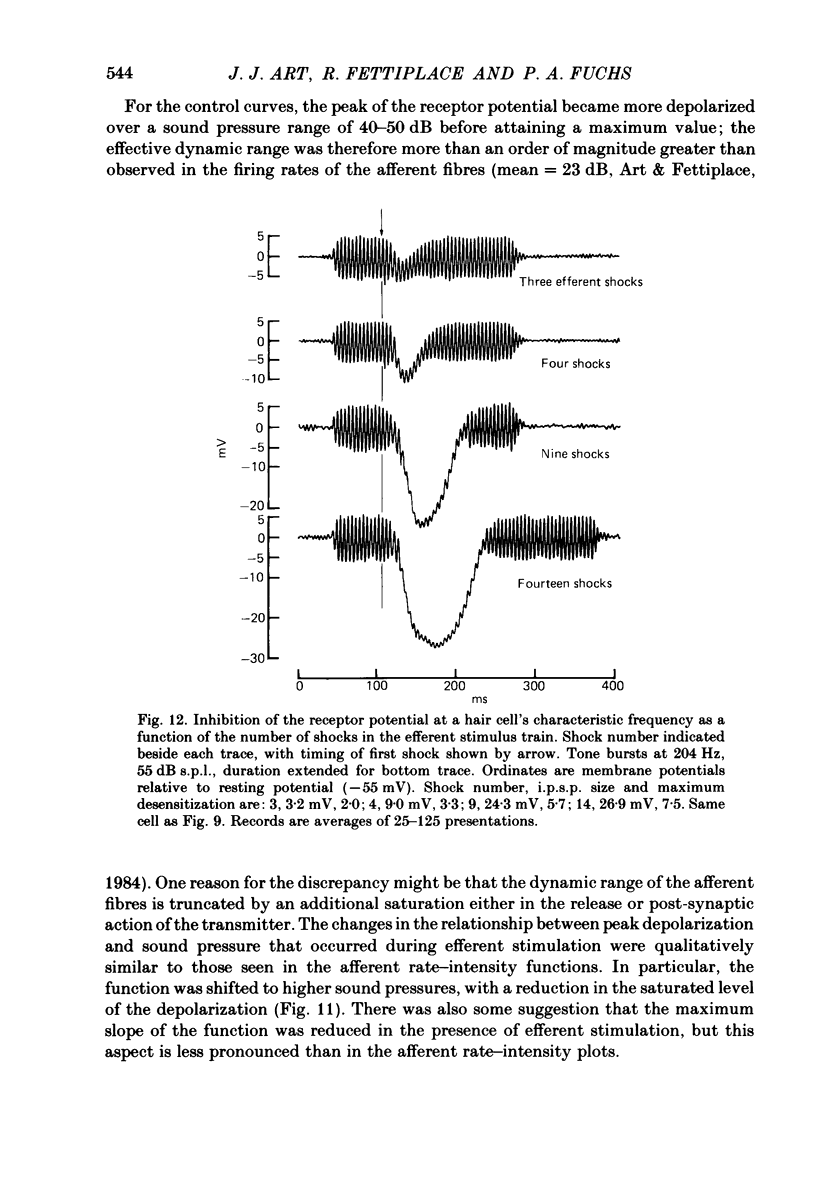
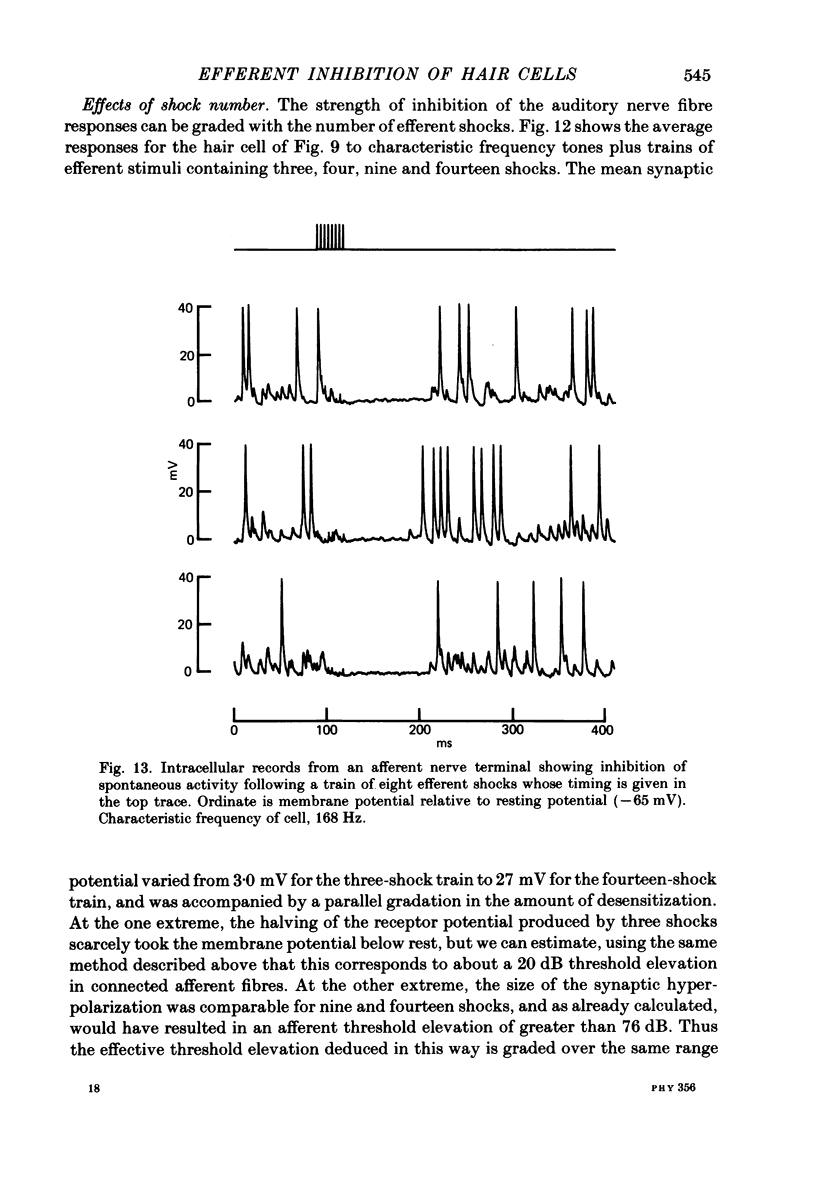
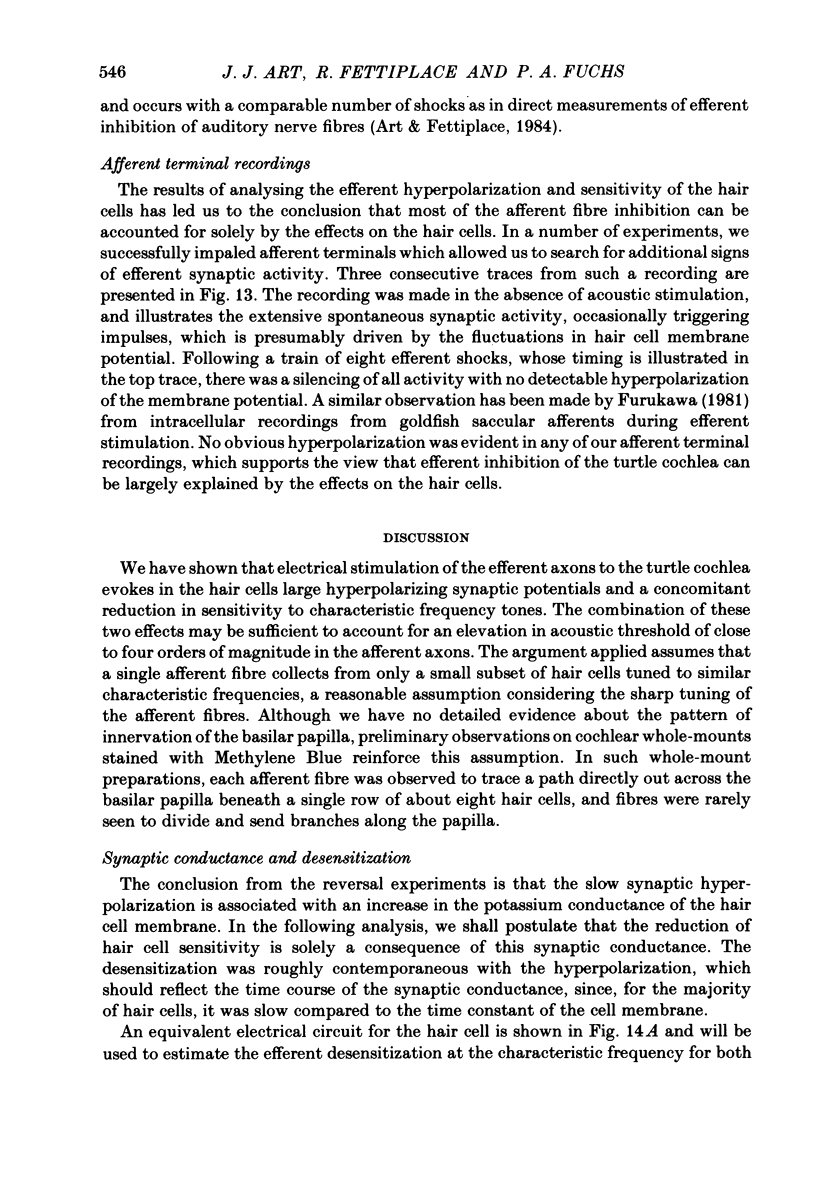
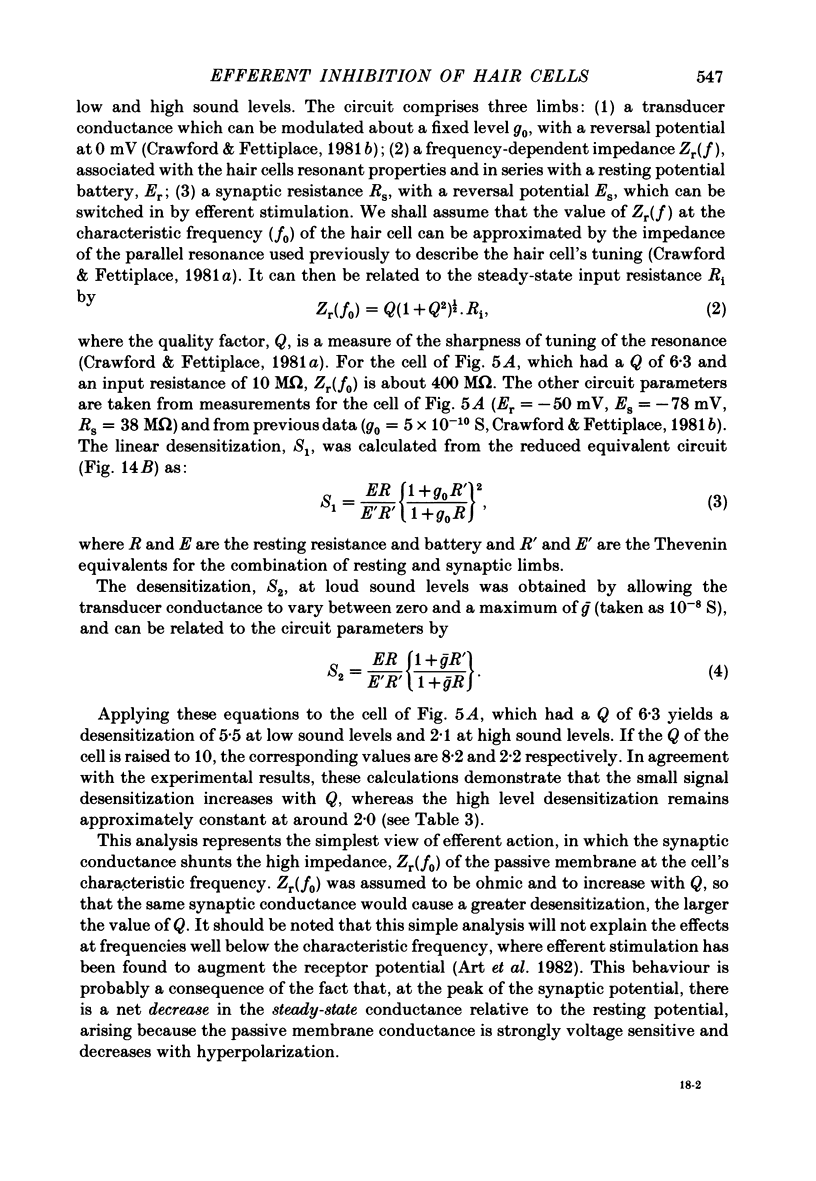
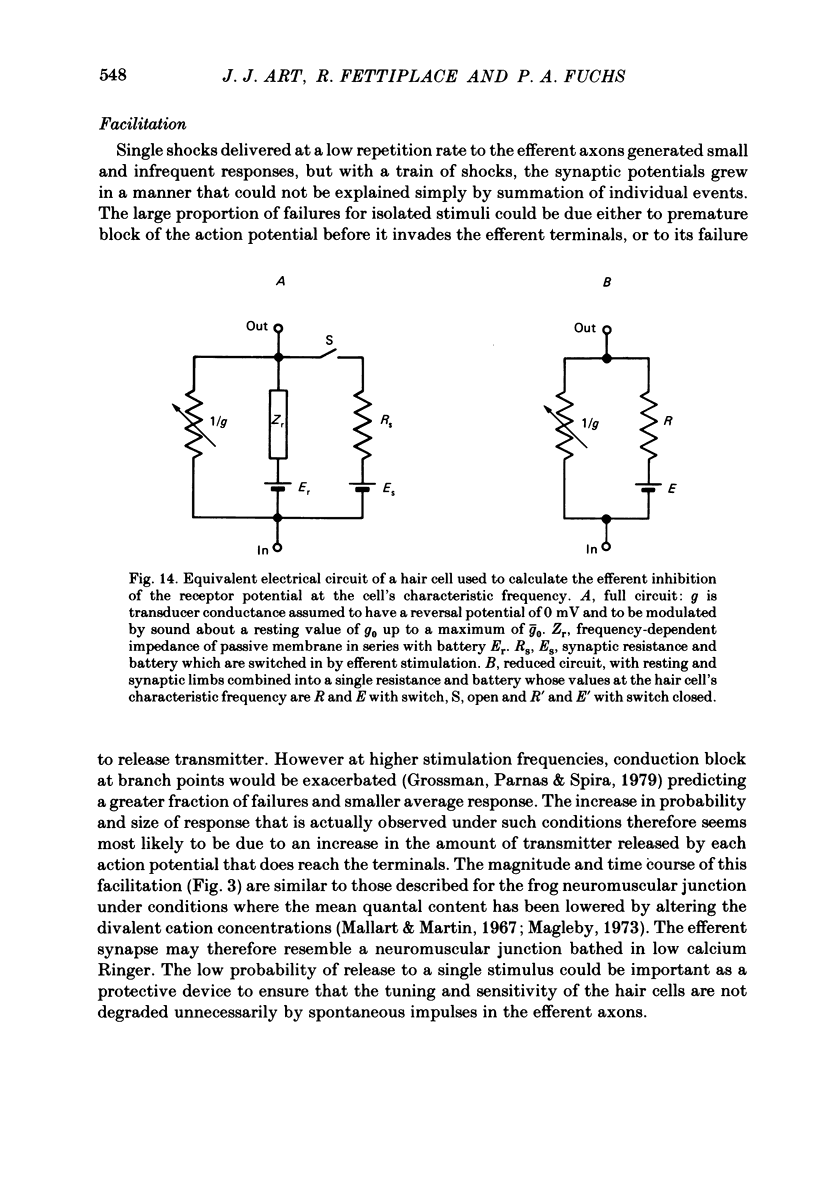
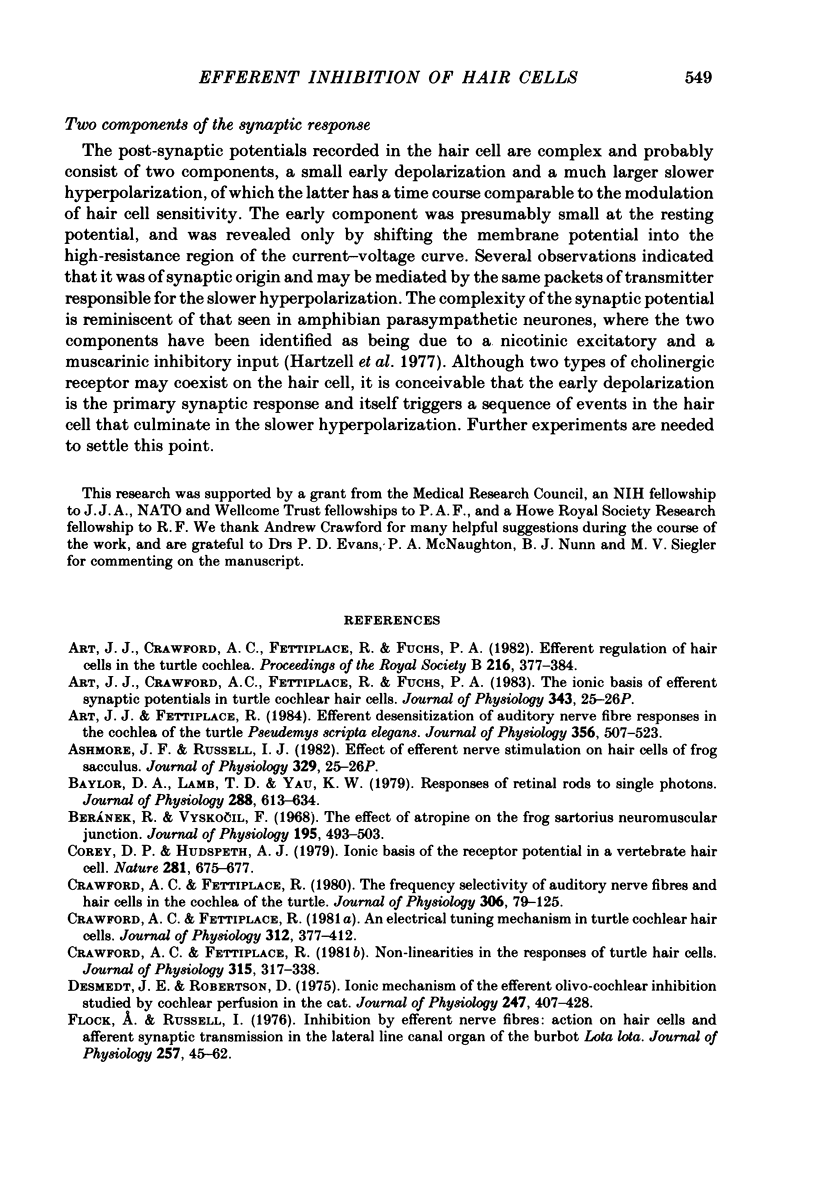
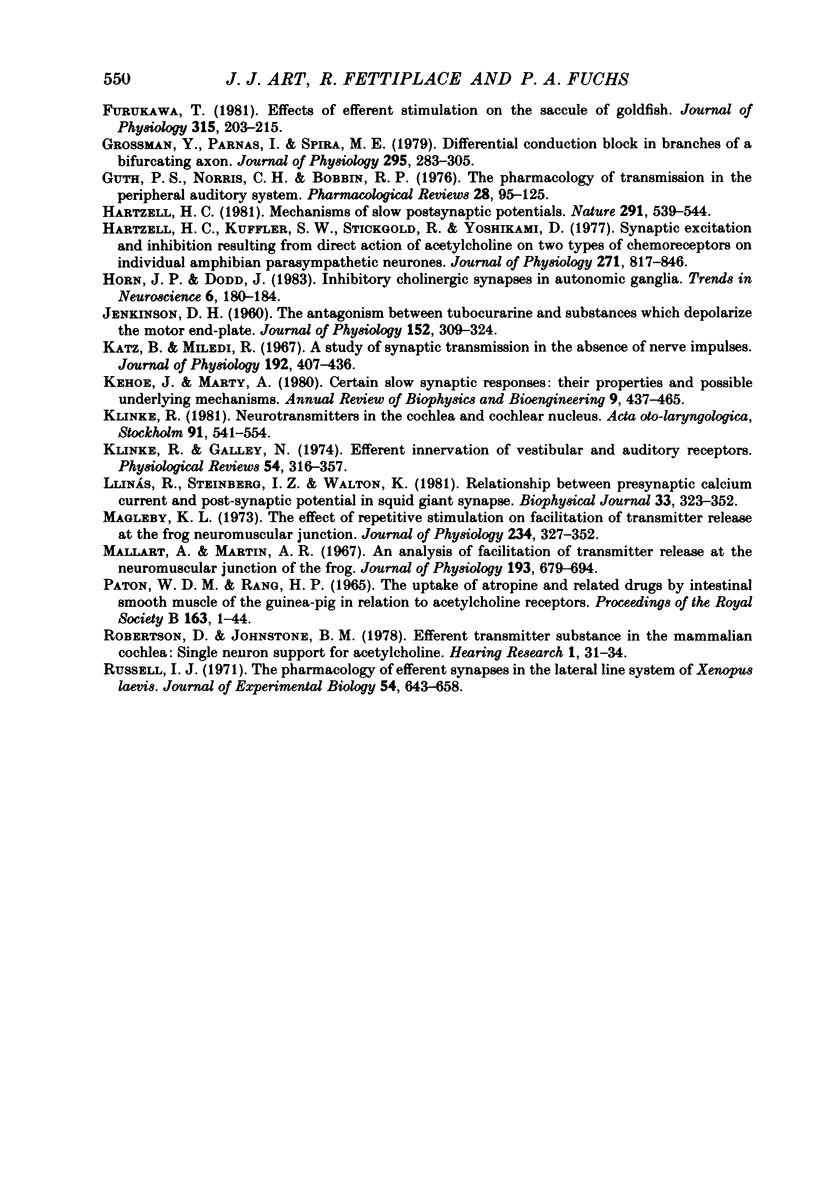
Images in this article
Selected References
These references are in PubMed. This may not be the complete list of references from this article.
- Art J. J., Crawford A. C., Fettiplace R., Fuchs P. A. Efferent regulation of hair cells in the turtle cochlea. Proc R Soc Lond B Biol Sci. 1982 Oct 22;216(1204):377–384. doi: 10.1098/rspb.1982.0081. [DOI] [PubMed] [Google Scholar]
- Art J. J., Fettiplace R. Efferent desensitization of auditory nerve fibre responses in the cochlea of the turtle Pseudemys scripta elegans. J Physiol. 1984 Nov;356:507–523. doi: 10.1113/jphysiol.1984.sp015480. [DOI] [PMC free article] [PubMed] [Google Scholar]
- Baylor D. A., Lamb T. D., Yau K. W. Responses of retinal rods to single photons. J Physiol. 1979 Mar;288:613–634. [PMC free article] [PubMed] [Google Scholar]
- Beránek R., Vyskocil F. The effect of atropine on the frog sartorius neuromuscular junction. J Physiol. 1968 Mar;195(2):493–503. doi: 10.1113/jphysiol.1968.sp008470. [DOI] [PMC free article] [PubMed] [Google Scholar]
- Corey D. P., Hudspeth A. J. Ionic basis of the receptor potential in a vertebrate hair cell. Nature. 1979 Oct 25;281(5733):675–677. doi: 10.1038/281675a0. [DOI] [PubMed] [Google Scholar]
- Crawford A. C., Fettiplace R. An electrical tuning mechanism in turtle cochlear hair cells. J Physiol. 1981 Mar;312:377–412. doi: 10.1113/jphysiol.1981.sp013634. [DOI] [PMC free article] [PubMed] [Google Scholar]
- Crawford A. C., Fettiplace R. Non-linearities in the responses of turtle hair cells. J Physiol. 1981 Jun;315:317–338. doi: 10.1113/jphysiol.1981.sp013750. [DOI] [PMC free article] [PubMed] [Google Scholar]
- Crawford A. C., Fettiplace R. The frequency selectivity of auditory nerve fibres and hair cells in the cochlea of the turtle. J Physiol. 1980 Sep;306:79–125. doi: 10.1113/jphysiol.1980.sp013387. [DOI] [PMC free article] [PubMed] [Google Scholar]
- Desmedt J. E., Robertson D. Ionic mechanism of the efferent olivo-cochlear inhibition studied by cochlear perfusion in the cat. J Physiol. 1975 May;247(2):407–428. doi: 10.1113/jphysiol.1975.sp010938. [DOI] [PMC free article] [PubMed] [Google Scholar]
- Flock A., Russell I. Inhibition by efferent nerve fibres: action on hair cells and afferent synaptic transmission in the lateral line canal organ of the burbot Lota lota. J Physiol. 1976 May;257(1):45–62. doi: 10.1113/jphysiol.1976.sp011355. [DOI] [PMC free article] [PubMed] [Google Scholar]
- Furukawa T. Effects of efferent stimulation on the saccule of goldfish. J Physiol. 1981 Jun;315:203–215. doi: 10.1113/jphysiol.1981.sp013742. [DOI] [PMC free article] [PubMed] [Google Scholar]
- Grossman Y., Parnas I., Spira M. E. Differential conduction block in branches of a bifurcating axon. J Physiol. 1979 Oct;295:283–305. doi: 10.1113/jphysiol.1979.sp012969. [DOI] [PMC free article] [PubMed] [Google Scholar]
- Guth P. S., Norris C. H., Bobbin R. P. The pharmacology of transmission in the peripheral auditory system. Pharmacol Rev. 1976 Jun;28(2):95–125. [PubMed] [Google Scholar]
- Hartzell H. C., Kuffler S. W., Stickgold R., Yoshikami D. Synaptic excitation and inhibition resulting from direct action of acetylcholine on two types of chemoreceptors on individual amphibian parasympathetic neurones. J Physiol. 1977 Oct;271(3):817–846. doi: 10.1113/jphysiol.1977.sp012027. [DOI] [PMC free article] [PubMed] [Google Scholar]
- Hartzell H. C. Mechanisms of slow postsynaptic potentials. Nature. 1981 Jun 18;291(5816):539–544. doi: 10.1038/291539a0. [DOI] [PubMed] [Google Scholar]
- JENKINSON D. H. The antagonism between tubocurarine and substances which depolarize the motor end-plate. J Physiol. 1960 Jul;152:309–324. doi: 10.1113/jphysiol.1960.sp006489. [DOI] [PMC free article] [PubMed] [Google Scholar]
- Katz B., Miledi R. A study of synaptic transmission in the absence of nerve impulses. J Physiol. 1967 Sep;192(2):407–436. doi: 10.1113/jphysiol.1967.sp008307. [DOI] [PMC free article] [PubMed] [Google Scholar]
- Kehoe J. S., Marty A. Certain slow synaptic responses: their properties and possible underlying mechanisms. Annu Rev Biophys Bioeng. 1980;9:437–465. doi: 10.1146/annurev.bb.09.060180.002253. [DOI] [PubMed] [Google Scholar]
- Klinke R., Galley N. Efferent innervation of vestibular and auditory receptors. Physiol Rev. 1974 Apr;54(2):316–357. doi: 10.1152/physrev.1974.54.2.316. [DOI] [PubMed] [Google Scholar]
- Klinke R. Neurotransmitters in the cochlea and the cochlear nucleus. Acta Otolaryngol. 1981 May-Jun;91(5-6):541–554. doi: 10.3109/00016488109138540. [DOI] [PubMed] [Google Scholar]
- Llinás R., Steinberg I. Z., Walton K. Relationship between presynaptic calcium current and postsynaptic potential in squid giant synapse. Biophys J. 1981 Mar;33(3):323–351. doi: 10.1016/S0006-3495(81)84899-0. [DOI] [PMC free article] [PubMed] [Google Scholar]
- Magleby K. L. The effect of repetitive stimulation on facilitation of transmitter release at the frog neuromuscular junction. J Physiol. 1973 Oct;234(2):327–352. doi: 10.1113/jphysiol.1973.sp010348. [DOI] [PMC free article] [PubMed] [Google Scholar]
- Mallart A., Martin A. R. An analysis of facilitation of transmitter release at the neuromuscular junction of the frog. J Physiol. 1967 Dec;193(3):679–694. doi: 10.1113/jphysiol.1967.sp008388. [DOI] [PMC free article] [PubMed] [Google Scholar]
- PATON W. D., RANG H. P. THE UPTAKE OF ATROPINE AND RELATED DRUGS BY INTESTINAL SMOOTH MUSCLE OF THE GUINEA-PIG IN RELATION TO ACETYLCHOLINE RECEPTORS. Proc R Soc Lond B Biol Sci. 1965 Aug 24;163:1–44. doi: 10.1098/rspb.1965.0058. [DOI] [PubMed] [Google Scholar]
- Robertson D., Johnstone B. M. Efferent transmitter substance in the mammalian cochlea: single neuron support for acetylcholine. Hear Res. 1978 Oct;1(1):31–34. doi: 10.1016/0378-5955(78)90006-0. [DOI] [PubMed] [Google Scholar]
- Russell I. J. The pharmacology of efferent synapses in the lateral-line system of Xenopus laevis. J Exp Biol. 1971 Jun;54(3):643–658. doi: 10.1242/jeb.54.3.643. [DOI] [PubMed] [Google Scholar]