Abstract
Post-ganglionic neurones of the isolated rat superior cervical ganglion were voltage clamped at 37 degrees C using separate intracellular voltage and current micro-electrodes. Control experiments in current clamp suggested that the neurone is electrotonically compact, the soma and the proximal dendritic membranes being under good spatial voltage uniformity. Depolarizing voltage steps from membrane potentials near -50 mV evoked: (i) a voltage-dependent inward Na+ current, (ii) an inward Ca2+ current, (iii) a voltage-dependent outward K+ current, (iv) a Ca2+-activated K+ outward current. Depolarizations from holding potentials more negative than -60 mV elicited, besides the currents mentioned above, a fast transient outward current IA which peaked in 1-2.5 ms and then decayed to zero following an exponential time course. The IA current was shown to be primarily, if not exclusively, carried by K+. It was unaffected by removal of external Ca2+ or addition of Cd2+ and was weakly blocked by tetraethylammonium ions and partially by 4-aminopyridine. The IA current showed a linear instantaneous current-voltage relationship. Its activation ranged from -60 to 0 mV with a mid-point at -30 mV. The A conductance could be described in terms of a simple Boltzmann distribution for a single gating particle with a valency of +3. Both the development and removal of inactivation followed a single exponential time course with a voltage-dependent time constant which was large near the resting potential (42 ms at -70 mV) and small (11 ms) near -100 and -40 mV. Steady-state inactivation h infinity ranged from -100 to -50 mV, with a mid-point at -78 mV, suggesting that approximately 50% of the IA channels are available at the physiological resting potential. Action potentials elicited from various holding potentials showed maximal repolarization rates dependent on the holding potential itself. This voltage dependence was found to be in reasonably good agreement with that of h infinity curve. These data are consistent with the view that in the rat sympathetic neurone, under physiological conditions, it is the IA current rather than the delayed outward current that is responsible for the fast action potential repolarization.
Full text
PDF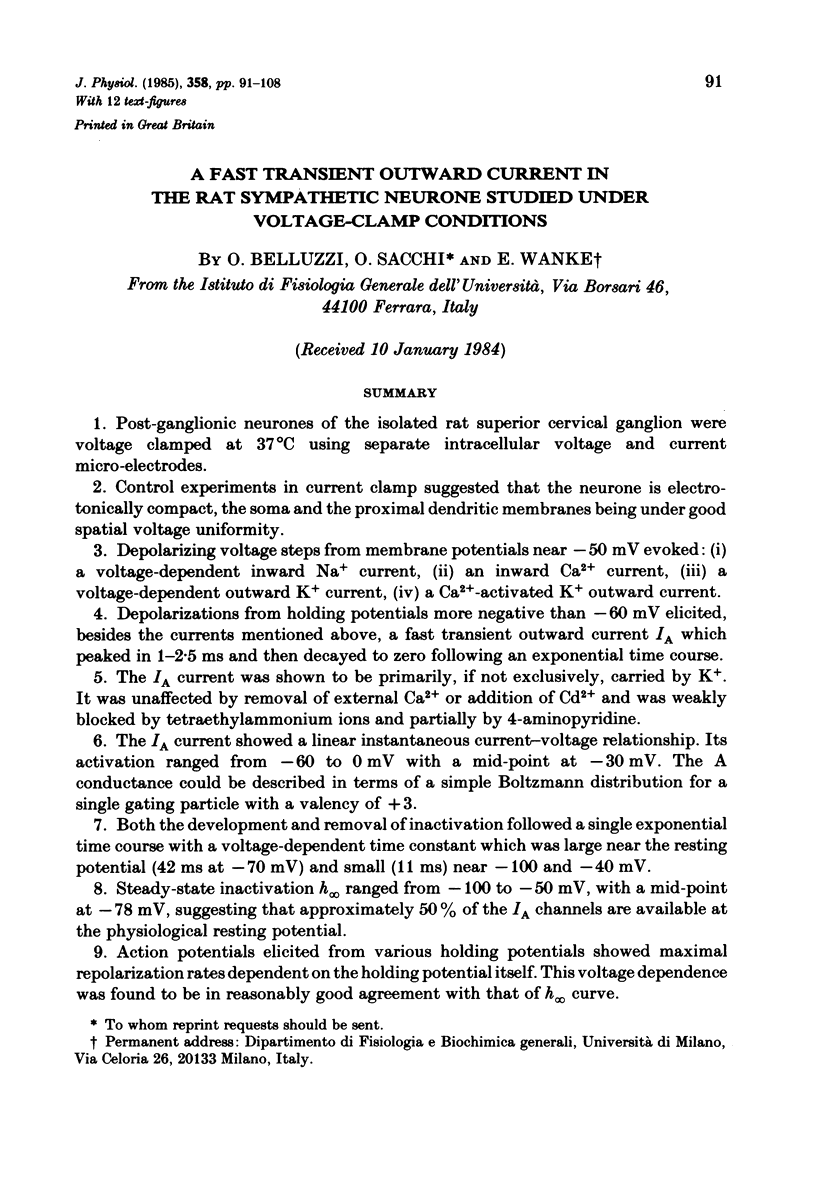
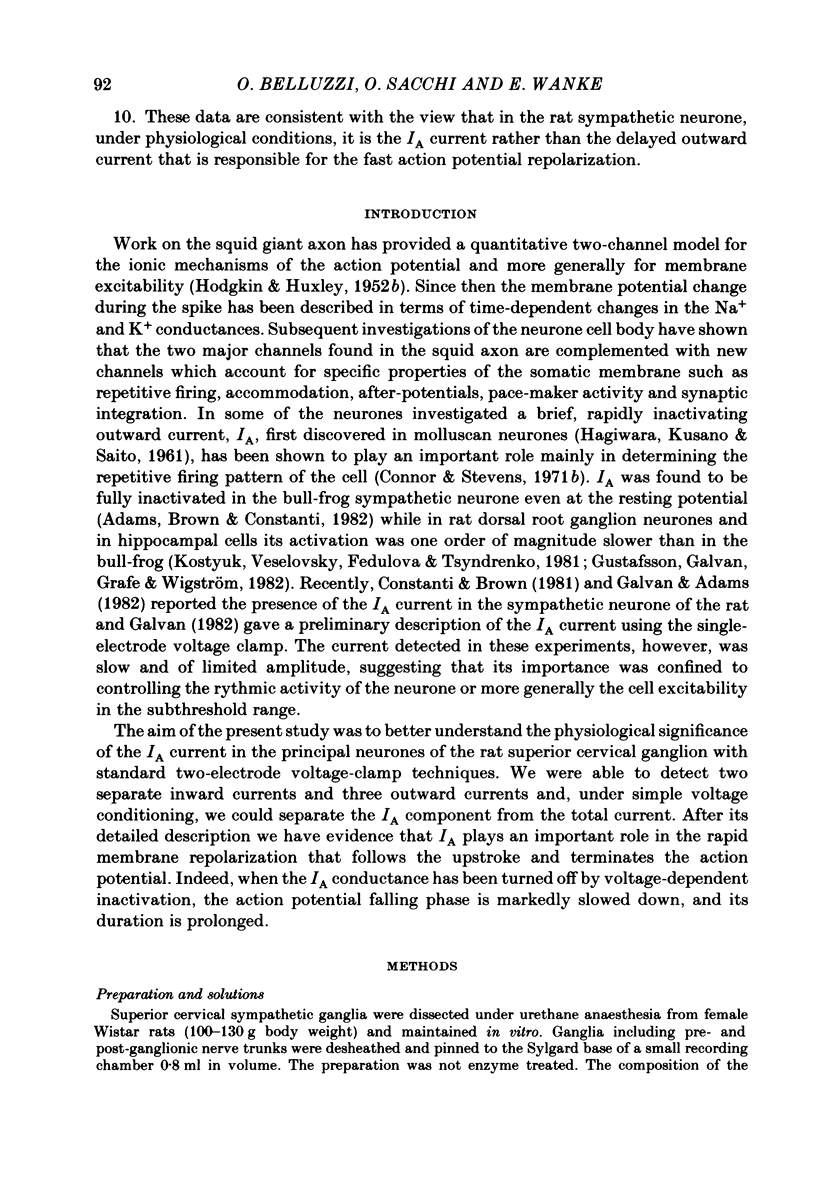
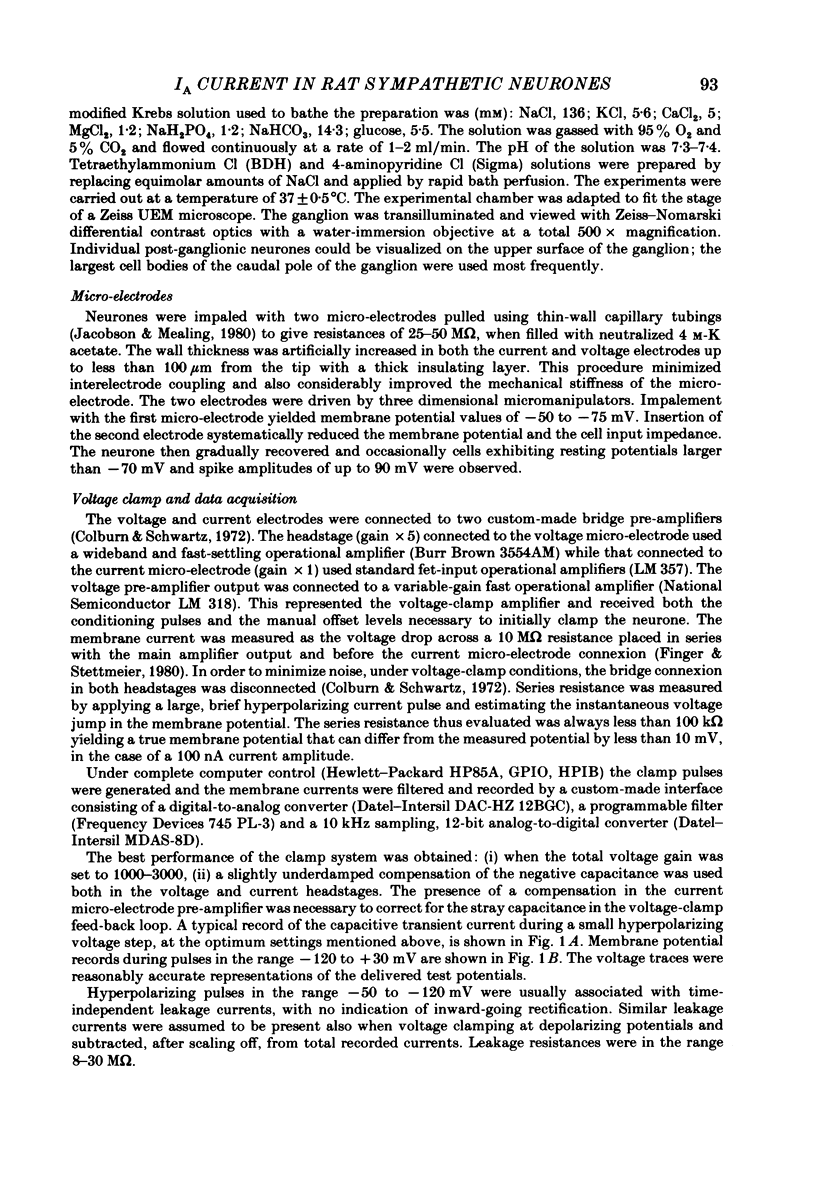
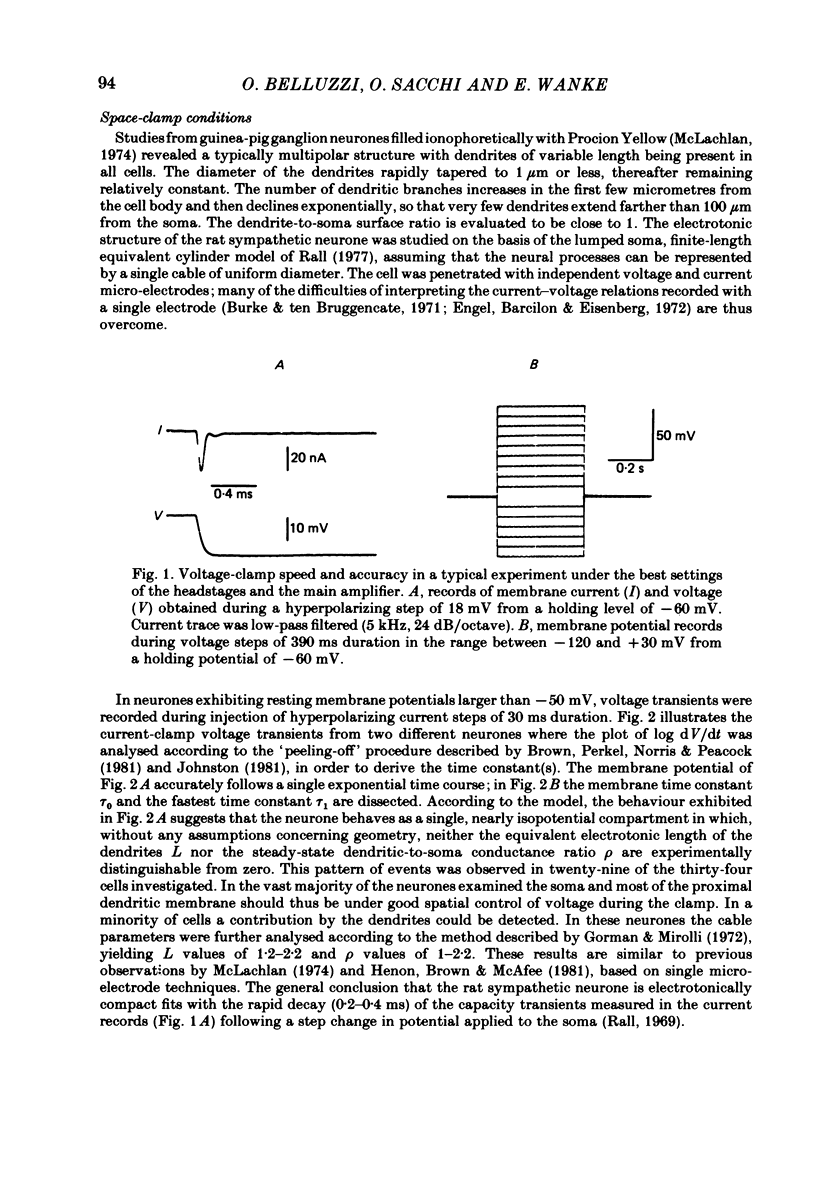
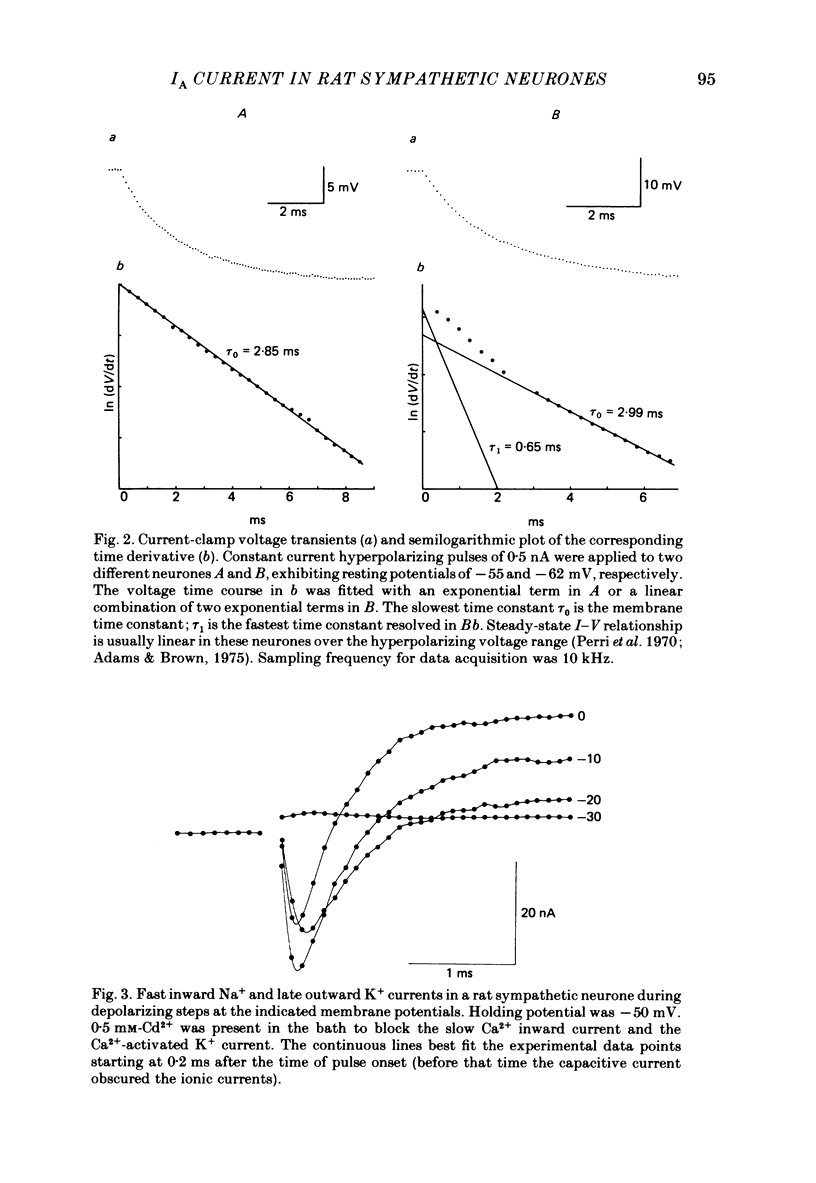
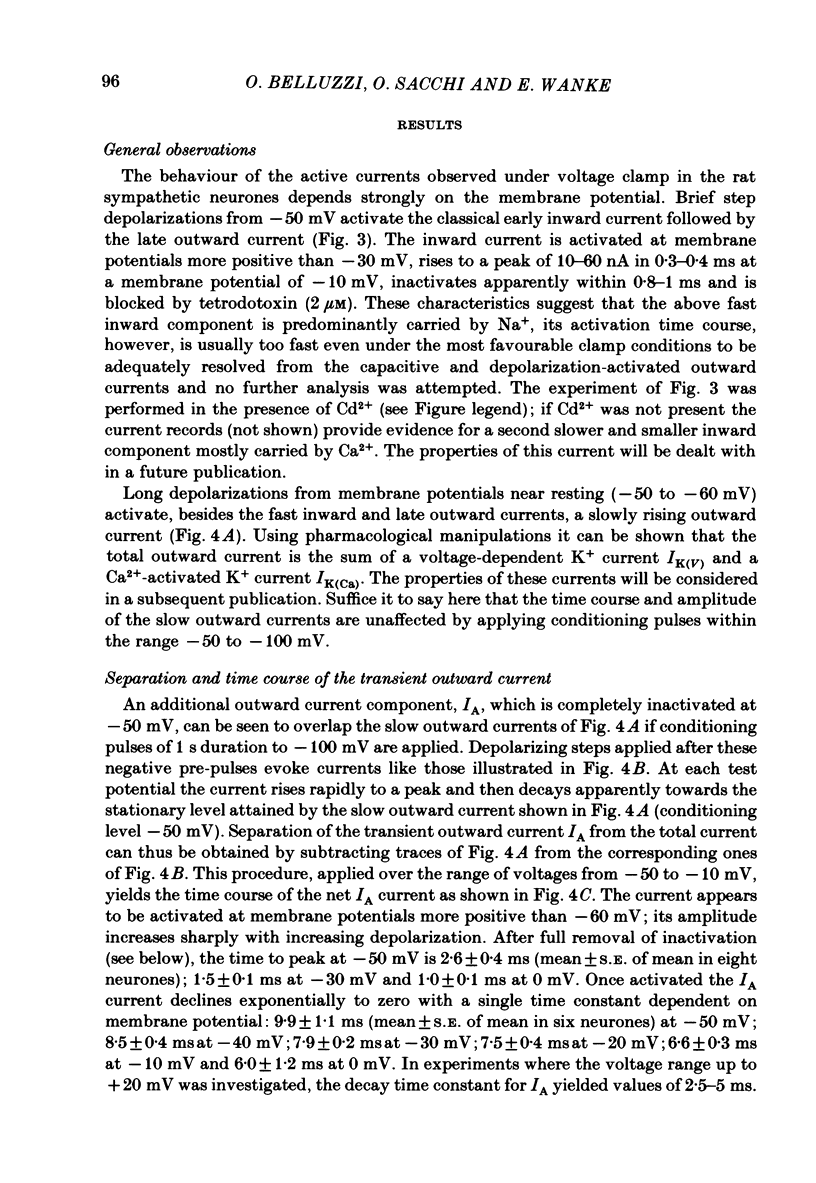
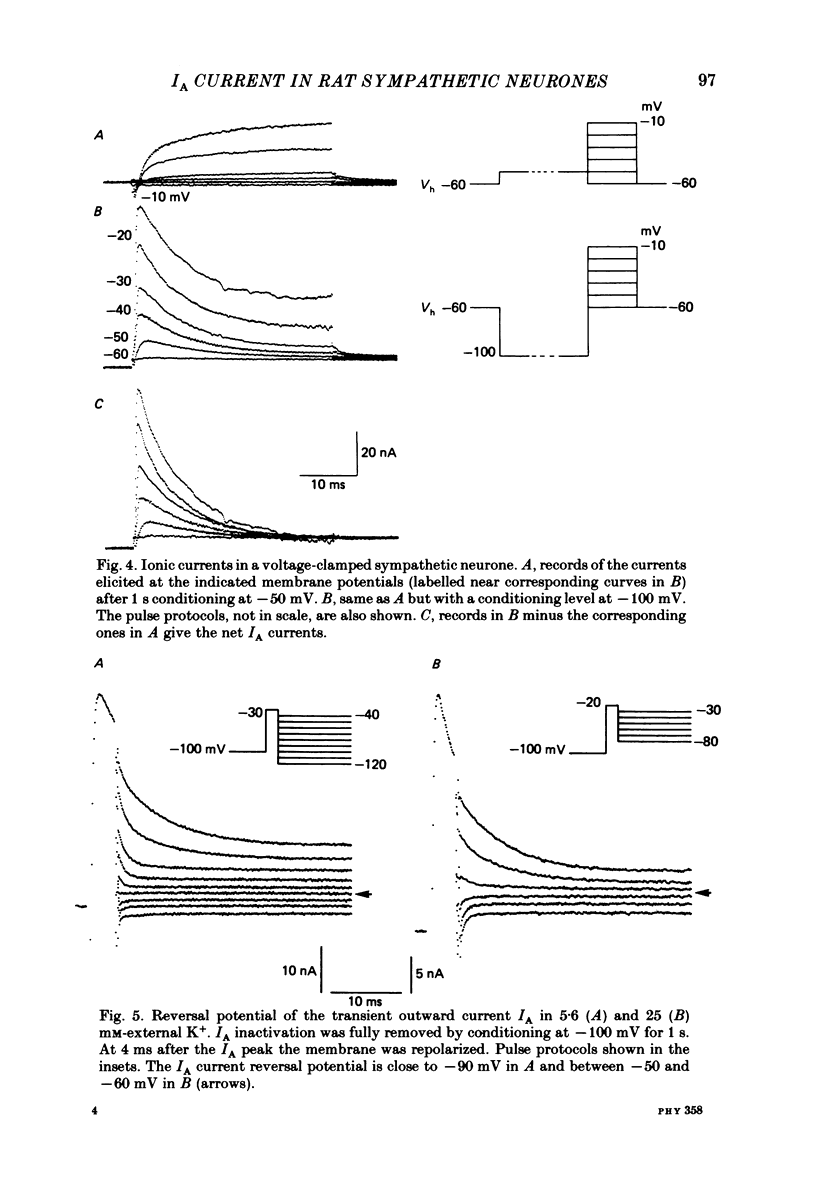
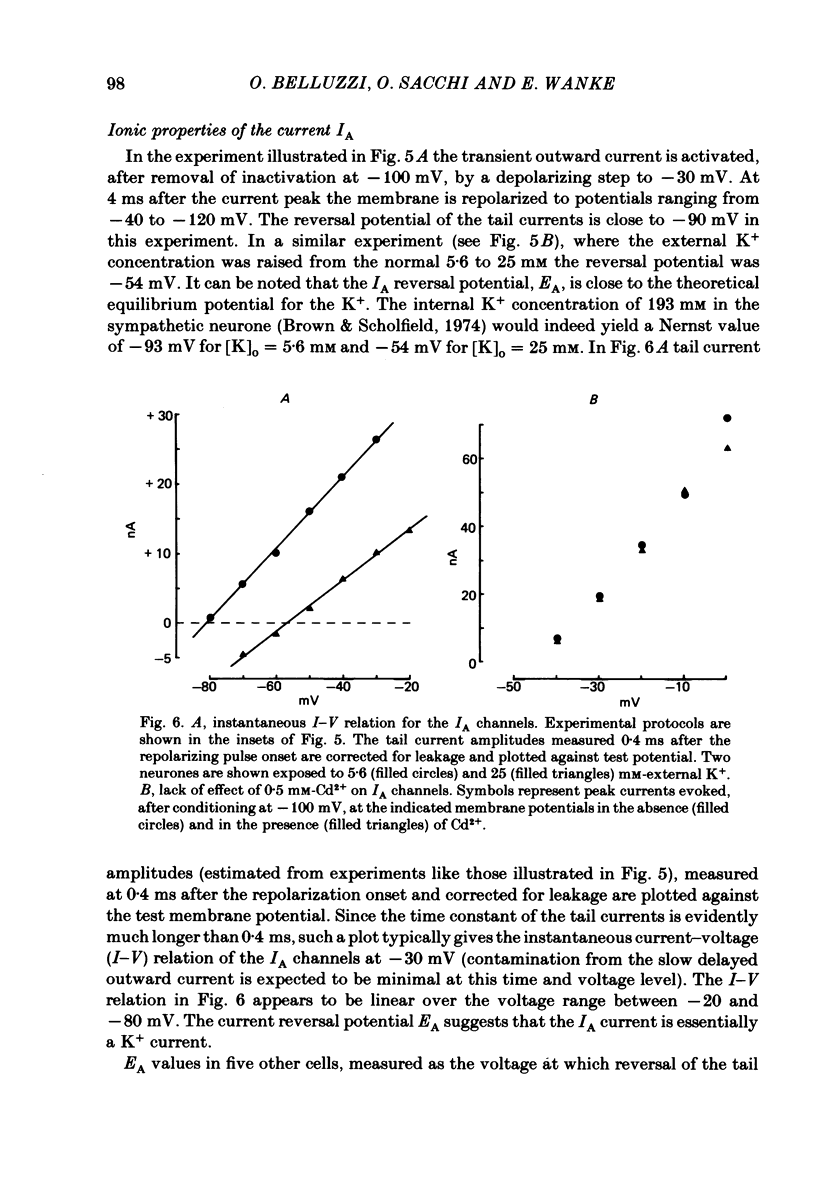
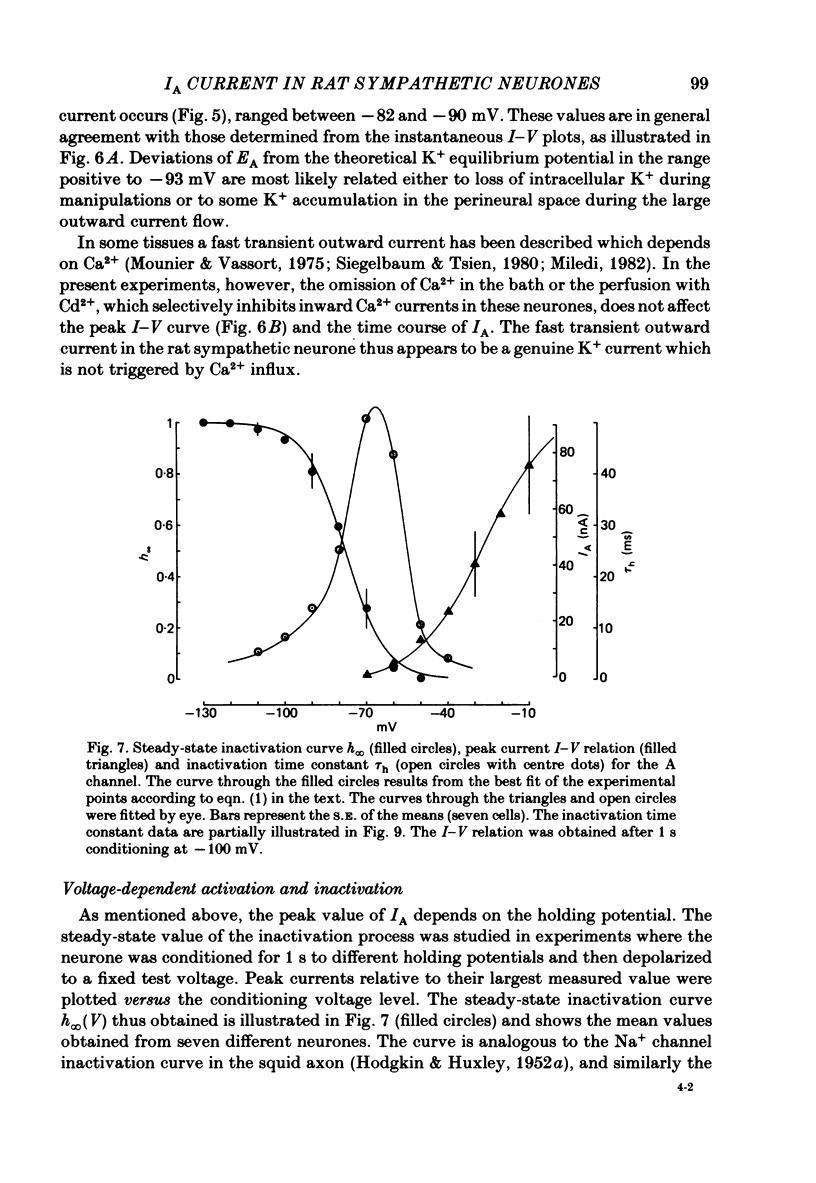
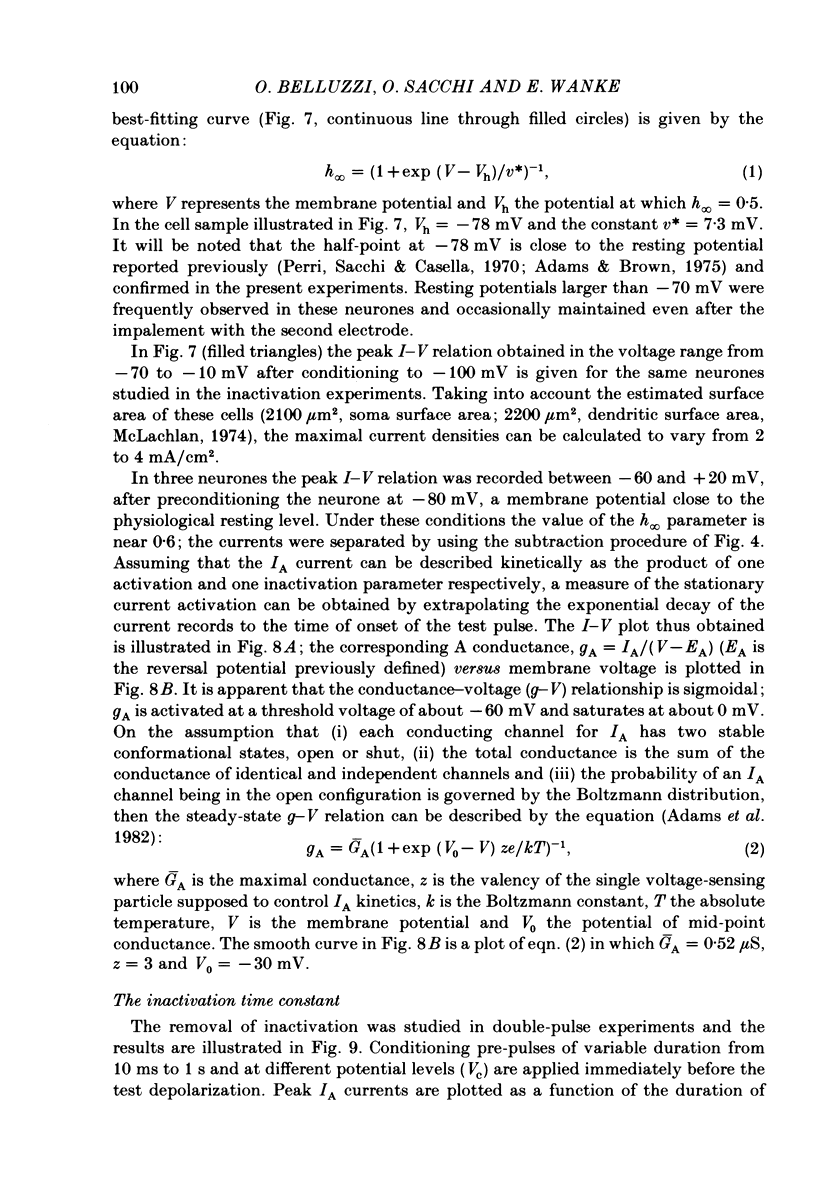
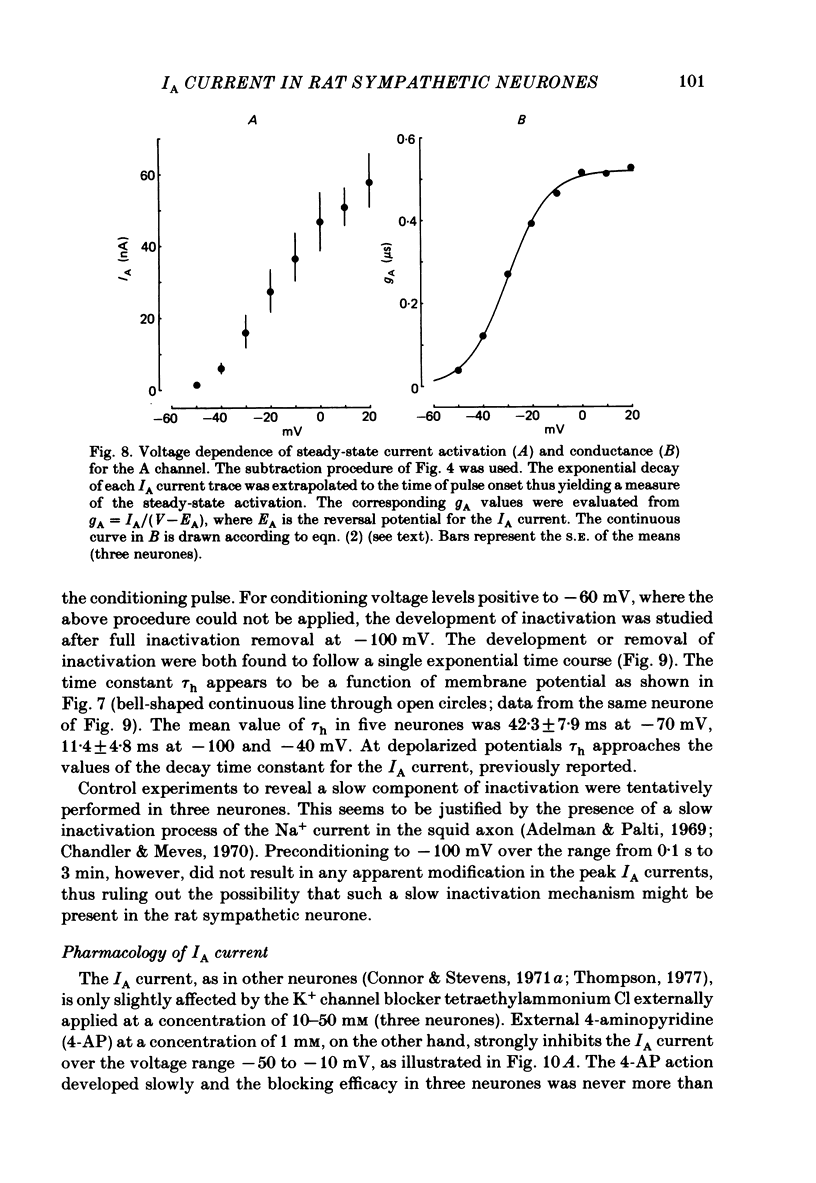
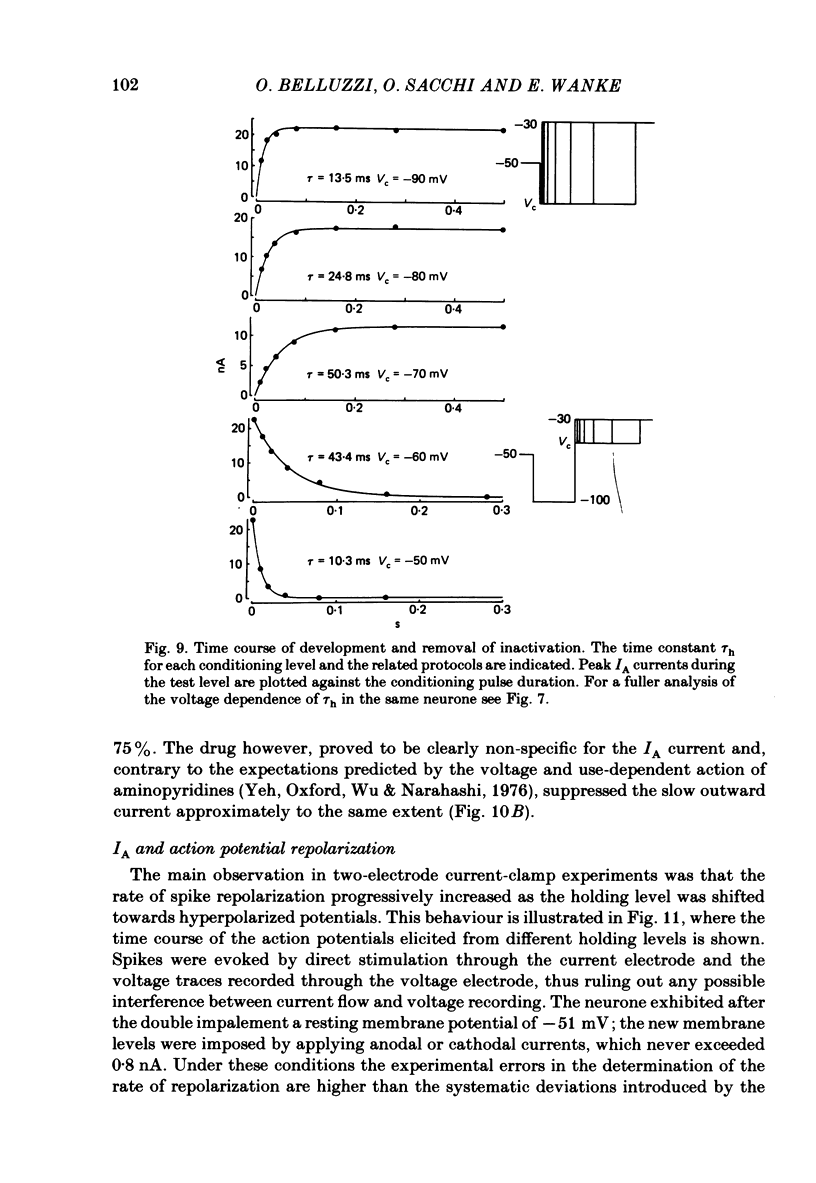
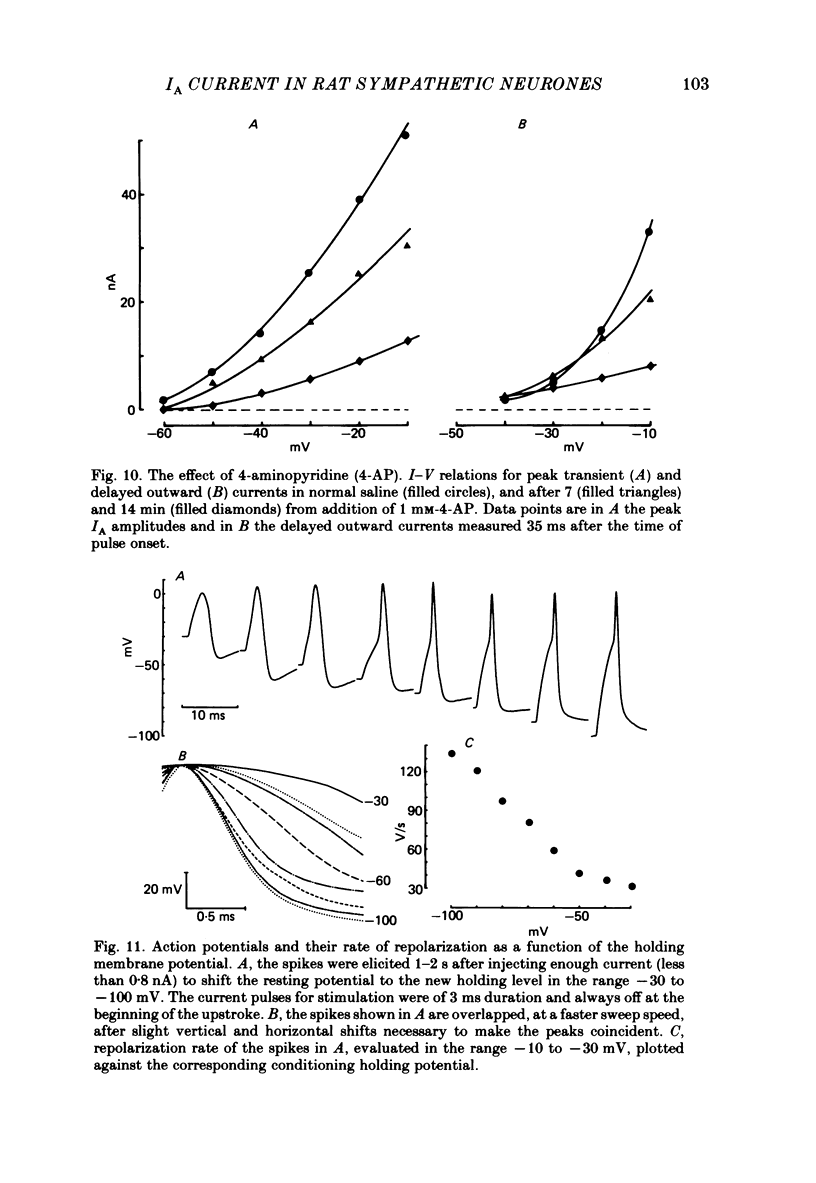
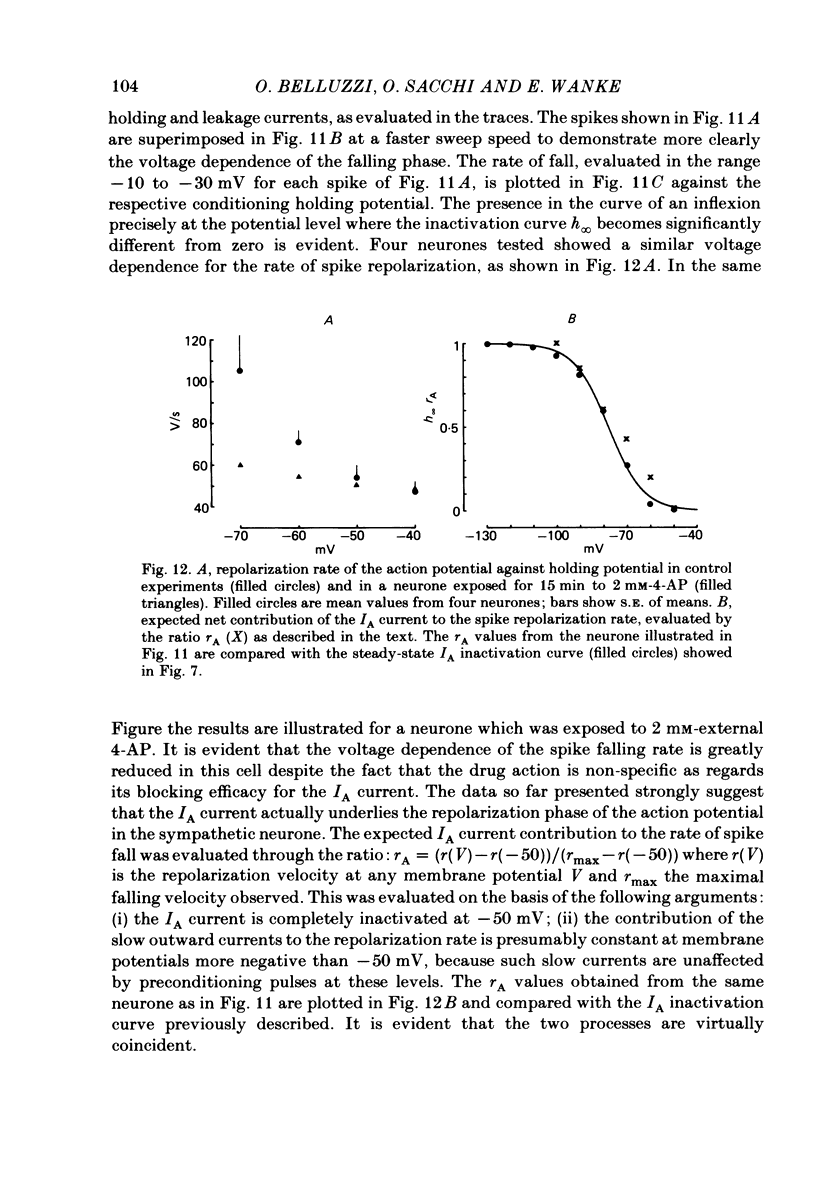
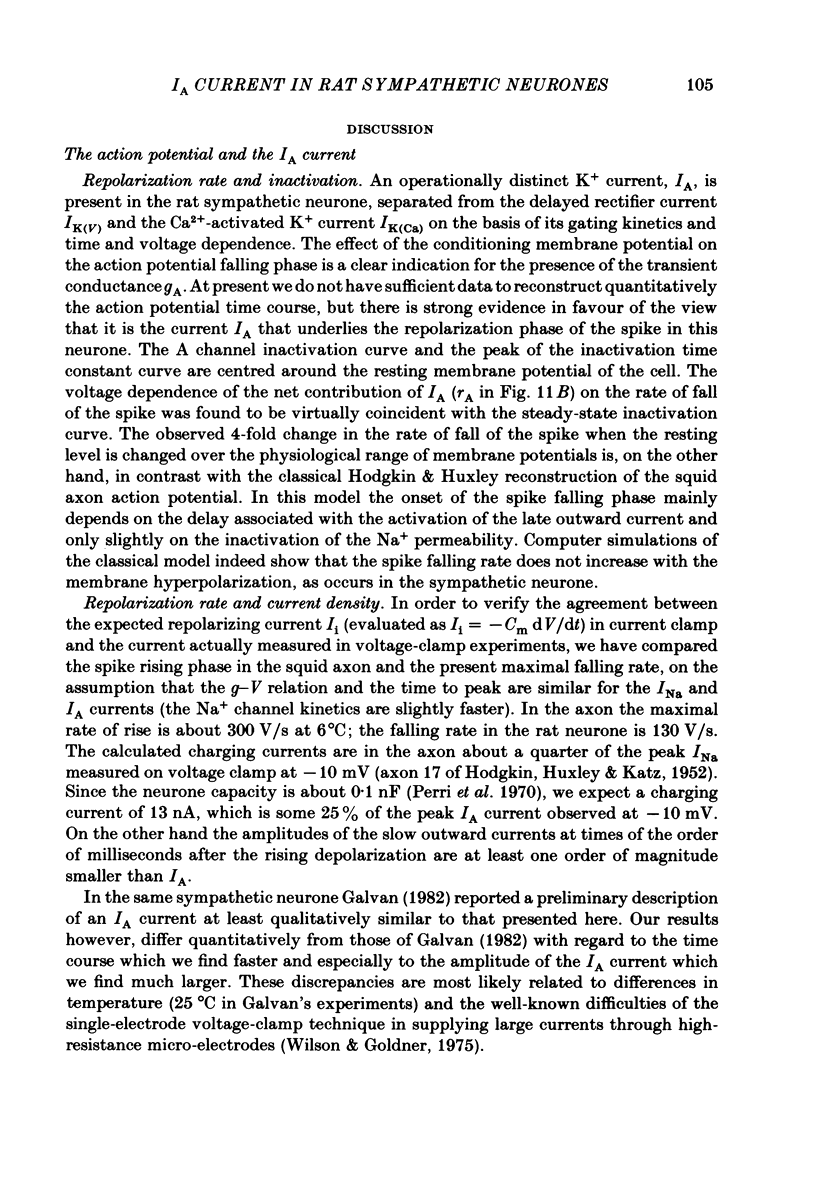
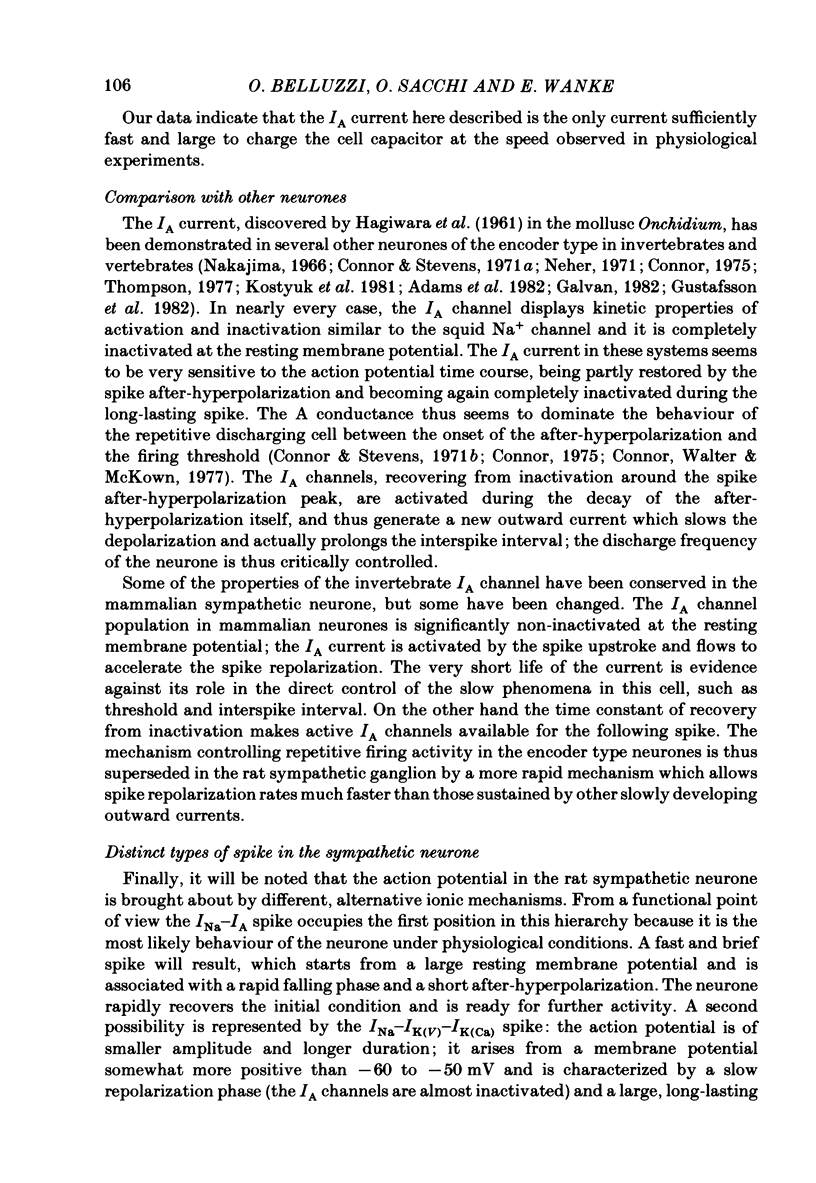
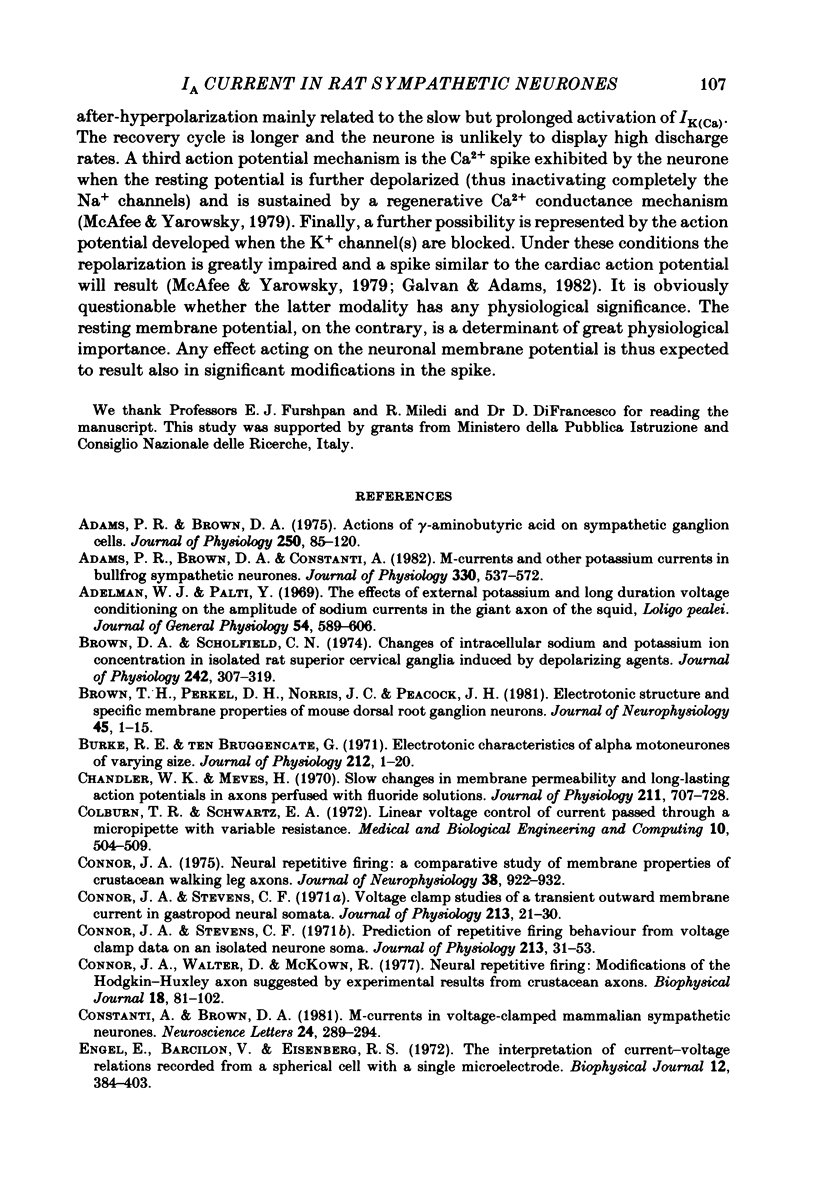
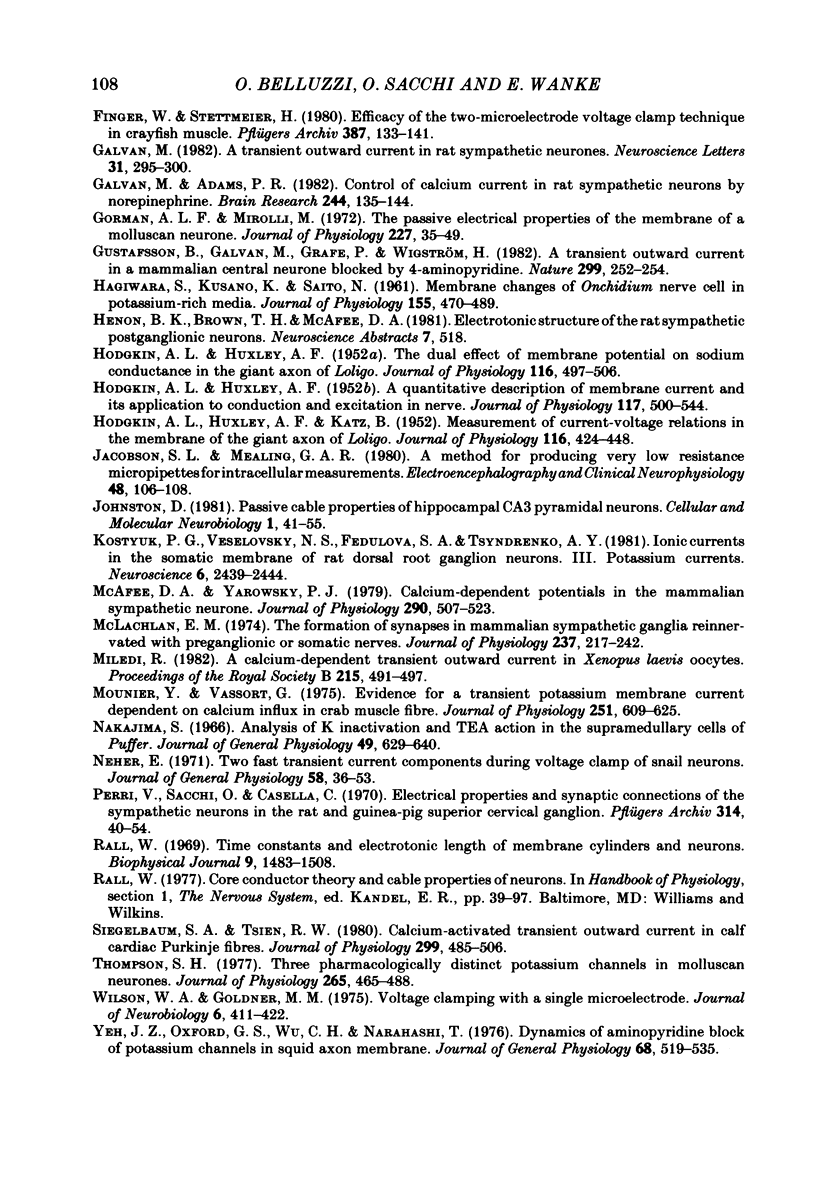
Selected References
These references are in PubMed. This may not be the complete list of references from this article.
- Adams P. R., Brown D. A. Actions of gamma-aminobutyric acid on sympathetic ganglion cells. J Physiol. 1975 Aug;250(1):85–120. doi: 10.1113/jphysiol.1975.sp011044. [DOI] [PMC free article] [PubMed] [Google Scholar]
- Adams P. R., Brown D. A., Constanti A. M-currents and other potassium currents in bullfrog sympathetic neurones. J Physiol. 1982 Sep;330:537–572. doi: 10.1113/jphysiol.1982.sp014357. [DOI] [PMC free article] [PubMed] [Google Scholar]
- Adelman W. J., Jr, Palti Y. The effects of external potassium and long duration voltage conditioning on the amplitude of sodium currents in the giant axon of the squid, Loligo pealei. J Gen Physiol. 1969 Nov;54(5):589–606. doi: 10.1085/jgp.54.5.589. [DOI] [PMC free article] [PubMed] [Google Scholar]
- Brown D. A., Scholfield C. N. Changes of intracellular sodium and potassium ion concentrations in isolated rat superior cervical ganglia induced by depolarizing agents. J Physiol. 1974 Oct;242(2):307–319. doi: 10.1113/jphysiol.1974.sp010709. [DOI] [PMC free article] [PubMed] [Google Scholar]
- Brown T. H., Perkel D. H., Norris J. C., Peacock J. H. Electrotonic structure and specific membrane properties of mouse dorsal root ganglion neurons. J Neurophysiol. 1981 Jan;45(1):1–15. doi: 10.1152/jn.1981.45.1.1. [DOI] [PubMed] [Google Scholar]
- Chandler W. K., Meves H. Slow changes in membrane permeability and long-lasting action potentials in axons perfused with fluoride solutions. J Physiol. 1970 Dec;211(3):707–728. doi: 10.1113/jphysiol.1970.sp009300. [DOI] [PMC free article] [PubMed] [Google Scholar]
- Colburn T. R., Schwartz E. A. Linear voltage control of current passed through a micropipette with variable resistance. Med Biol Eng. 1972 Jul;10(4):504–509. doi: 10.1007/BF02474198. [DOI] [PubMed] [Google Scholar]
- Connor J. A. Neural repetitive firing: a comparative study of membrane properties of crustacean walking leg axons. J Neurophysiol. 1975 Jul;38(4):922–932. doi: 10.1152/jn.1975.38.4.922. [DOI] [PubMed] [Google Scholar]
- Connor J. A., Stevens C. F. Prediction of repetitive firing behaviour from voltage clamp data on an isolated neurone soma. J Physiol. 1971 Feb;213(1):31–53. doi: 10.1113/jphysiol.1971.sp009366. [DOI] [PMC free article] [PubMed] [Google Scholar]
- Connor J. A., Stevens C. F. Voltage clamp studies of a transient outward membrane current in gastropod neural somata. J Physiol. 1971 Feb;213(1):21–30. doi: 10.1113/jphysiol.1971.sp009365. [DOI] [PMC free article] [PubMed] [Google Scholar]
- Connor J. A., Walter D., McKown R. Neural repetitive firing: modifications of the Hodgkin-Huxley axon suggested by experimental results from crustacean axons. Biophys J. 1977 Apr;18(1):81–102. doi: 10.1016/S0006-3495(77)85598-7. [DOI] [PMC free article] [PubMed] [Google Scholar]
- Constanti A., Brown D. A. M-Currents in voltage-clamped mammalian sympathetic neurones. Neurosci Lett. 1981 Jul 17;24(3):289–294. doi: 10.1016/0304-3940(81)90173-7. [DOI] [PubMed] [Google Scholar]
- Engel E., Barcilon V., Eisenberg R. S. The interpretation of current-voltage relations recorded from a spherical cell with a single microelectrode. Biophys J. 1972 Apr;12(4):384–403. doi: 10.1016/S0006-3495(72)86091-0. [DOI] [PMC free article] [PubMed] [Google Scholar]
- Finger W., Stettmeier H. Efficacy of the two-microelectrode voltage clamp technique in crayfish muscle. Pflugers Arch. 1980 Sep;387(2):133–141. doi: 10.1007/BF00584264. [DOI] [PubMed] [Google Scholar]
- Galvan M. A transient outward current in rat sympathetic neurones. Neurosci Lett. 1982 Aug 31;31(3):295–300. doi: 10.1016/0304-3940(82)90036-2. [DOI] [PubMed] [Google Scholar]
- Galvan M., Adams P. R. Control of calcium current in rat sympathetic neurons by norepinephrine. Brain Res. 1982 Jul 22;244(1):135–144. doi: 10.1016/0006-8993(82)90911-8. [DOI] [PubMed] [Google Scholar]
- Gorman A. L., Mirolli M. The passive electrical properties of the membrane of a molluscan neurone. J Physiol. 1972 Dec;227(1):35–49. doi: 10.1113/jphysiol.1972.sp010018. [DOI] [PMC free article] [PubMed] [Google Scholar]
- Gustafsson B., Galvan M., Grafe P., Wigström H. A transient outward current in a mammalian central neurone blocked by 4-aminopyridine. Nature. 1982 Sep 16;299(5880):252–254. doi: 10.1038/299252a0. [DOI] [PubMed] [Google Scholar]
- HAGIWARA S., KUSANO K., SAITO N. Membrane changes of Onchidium nerve cell in potassium-rich media. J Physiol. 1961 Mar;155:470–489. doi: 10.1113/jphysiol.1961.sp006640. [DOI] [PMC free article] [PubMed] [Google Scholar]
- HODGKIN A. L., HUXLEY A. F. A quantitative description of membrane current and its application to conduction and excitation in nerve. J Physiol. 1952 Aug;117(4):500–544. doi: 10.1113/jphysiol.1952.sp004764. [DOI] [PMC free article] [PubMed] [Google Scholar]
- HODGKIN A. L., HUXLEY A. F., KATZ B. Measurement of current-voltage relations in the membrane of the giant axon of Loligo. J Physiol. 1952 Apr;116(4):424–448. doi: 10.1113/jphysiol.1952.sp004716. [DOI] [PMC free article] [PubMed] [Google Scholar]
- HODGKIN A. L., HUXLEY A. F. The dual effect of membrane potential on sodium conductance in the giant axon of Loligo. J Physiol. 1952 Apr;116(4):497–506. doi: 10.1113/jphysiol.1952.sp004719. [DOI] [PMC free article] [PubMed] [Google Scholar]
- Jacobson S. L., Mealing G. A. A method for producing very low resistance micropipettes for intracellular measurements. Electroencephalogr Clin Neurophysiol. 1980 Jan;48(1):106–108. doi: 10.1016/0013-4694(80)90050-4. [DOI] [PubMed] [Google Scholar]
- Johnston D. Passive cable properties of hippocampal CA3 pyramidal neurons. Cell Mol Neurobiol. 1981 Mar;1(1):41–55. doi: 10.1007/BF00736038. [DOI] [PMC free article] [PubMed] [Google Scholar]
- Kostyuk P. G., Veselovsky N. S., Fedulova S. A., Tsyndrenko A. Y. Ionic currents in the somatic membrane of rat dorsal root ganglion neurons-III. Potassium currents. Neuroscience. 1981;6(12):2439–2444. doi: 10.1016/0306-4522(81)90090-7. [DOI] [PubMed] [Google Scholar]
- McAfee D. A., Yarowsky P. J. Calcium-dependent potentials in the mammalian sympathetic neurone. J Physiol. 1979 May;290(2):507–523. doi: 10.1113/jphysiol.1979.sp012787. [DOI] [PMC free article] [PubMed] [Google Scholar]
- McLachlan E. M. The formation of synapses in mammalian sympathetic ganglia reinnervated with preganglionic or somatic nerves. J Physiol. 1974 Feb;237(1):217–242. doi: 10.1113/jphysiol.1974.sp010479. [DOI] [PMC free article] [PubMed] [Google Scholar]
- Miledi R. A calcium-dependent transient outward current in Xenopus laevis oocytes. Proc R Soc Lond B Biol Sci. 1982 Jul 22;215(1201):491–497. doi: 10.1098/rspb.1982.0056. [DOI] [PubMed] [Google Scholar]
- Mounier Y., Vassort G. Evidence for a transient potassium membrane current dependent on calcium influx in crab muscle fibre. J Physiol. 1975 Oct;251(3):609–625. doi: 10.1113/jphysiol.1975.sp011111. [DOI] [PMC free article] [PubMed] [Google Scholar]
- Nakajima S. Analysis of K inactivation and TEA action in the supramedullary cells of puffer. J Gen Physiol. 1966 Mar;49(4):629–640. doi: 10.1085/jgp.49.4.629. [DOI] [PMC free article] [PubMed] [Google Scholar]
- Neher E. Two fast transient current components during voltage clamp on snail neurons. J Gen Physiol. 1971 Jul;58(1):36–53. doi: 10.1085/jgp.58.1.36. [DOI] [PMC free article] [PubMed] [Google Scholar]
- Perri V., Sacchi O., Caella C. Electrical properties and synaptic connections of the sympathetic neurons in the rat and guinea-pig superior cervical ganglion. Pflugers Arch. 1970;314(1):40–54. doi: 10.1007/BF00587045. [DOI] [PubMed] [Google Scholar]
- Rall W. Time constants and electrotonic length of membrane cylinders and neurons. Biophys J. 1969 Dec;9(12):1483–1508. doi: 10.1016/S0006-3495(69)86467-2. [DOI] [PMC free article] [PubMed] [Google Scholar]
- Siegelbaum S. A., Tsien R. W. Calcium-activated transient outward current in calf cardiac Purkinje fibres. J Physiol. 1980 Feb;299:485–506. doi: 10.1113/jphysiol.1980.sp013138. [DOI] [PMC free article] [PubMed] [Google Scholar]
- Thompson S. H. Three pharmacologically distinct potassium channels in molluscan neurones. J Physiol. 1977 Feb;265(2):465–488. doi: 10.1113/jphysiol.1977.sp011725. [DOI] [PMC free article] [PubMed] [Google Scholar]
- Wilson W. A., Goldner M. M. Voltage clamping with a single microelectrode. J Neurobiol. 1975 Jul;6(4):411–422. doi: 10.1002/neu.480060406. [DOI] [PubMed] [Google Scholar]
- Yeh J. Z., Oxford G. S., Wu C. H., Narahashi T. Dynamics of aminopyridine block of potassium channels in squid axon membrane. J Gen Physiol. 1976 Nov;68(5):519–535. doi: 10.1085/jgp.68.5.519. [DOI] [PMC free article] [PubMed] [Google Scholar]