Abstract
Intracellular pH (pHi) of Purkinje fibres from sheep heart was recorded with pH-sensitive glass micro-electrodes. The cells were acidified by one of three methods: (1) exposure to and subsequent removal of NH4Cl, (2) exposure to solutions containing 5% CO2 or (3) exposure to an acidic Tyrode solution. The pHi recovery from these acidifications was studied. The time constant of recovery from an acidification induced by NH4Cl was almost twice as long as that from one induced by CO2 or acid extracellular pH. Following an acidification induced by exposure to CO2 the time constant of pHi recovery was not changed when the cell was depolarized to -40 mV (by replacement of some Na+ by K+). An intracellular acidification was produced when extracellular Na+ was removed and replaced by quaternary ammonium ions or K+. Such Na+-free solutions also inhibited pHi recovery from an acidification. A 50% inhibition of the rate of recovery was produced by lowering the [Na+]o to 8 mM. When used as a Na+ substitute, Li+ could permit recovery. Tris (22 mM) changed pHi in the alkaline direction. Amiloride (1 mM) or a decrease in temperature slowed the recovery from an acidification (Q10 = 2.65). There was no effect of SITS (4-acetamido-4'-isothiocyanatostilbene-2,2'-disulphonic acid disodium salt; 100 microM) on the recovery. Na+-sensitive glass micro-electrodes were used to measure the intracellular Na+ activity when [Na+]o was lowered to levels used in our pHi recovery experiments. From these data we have calculated the apparent Na+ electrochemical gradient at different values of [Na+]o. If this gradient is responsible for H+ efflux from the cell then, by applying thermodynamic considerations, it can be shown that only low concentrations (1-2mM) of extracellular Na+ are required. Solutions containing a very low [Ca2+]o (less than 10(-8) M, buffered with EGTA) were used to prevent large rises of [Ca2+]i which may occur on removal of external Na+. Under these conditions pHi recovery is still dependent upon [Na+]o, and the apparent inhibition of pHi recovery by removal of Na+ is not simply due to rises in [Ca2+]i. The intracellular acidification which occurs on removal of Na+ does not occur when [Ca2+]o is very low (less than 10(-8) M).(ABSTRACT TRUNCATED AT 400 WORDS)
Full text
PDF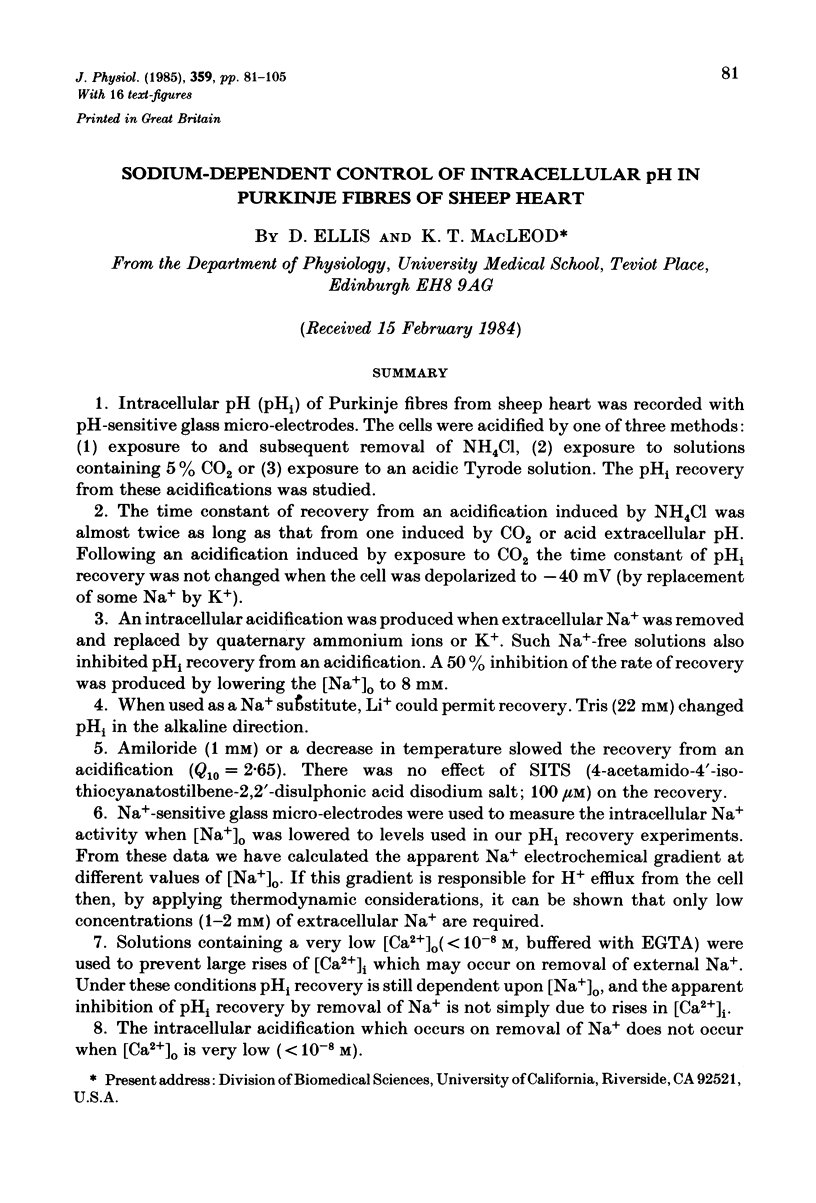
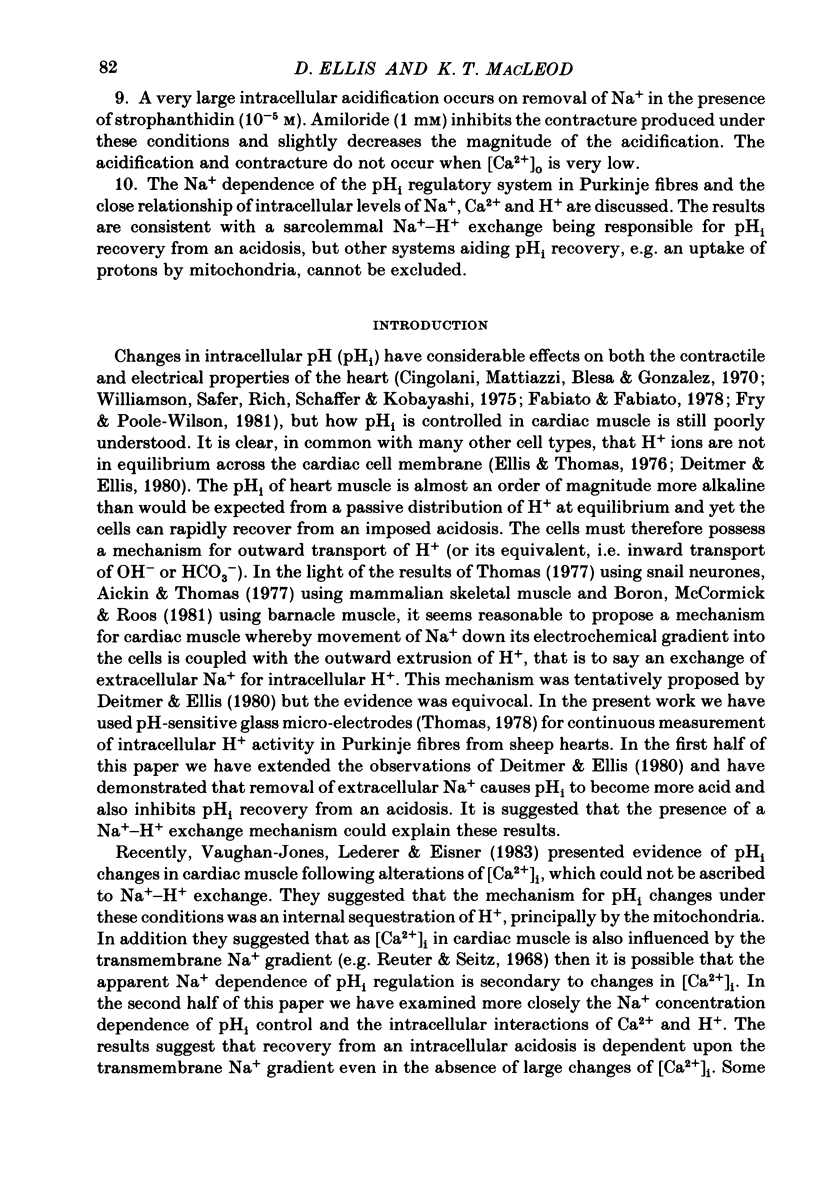
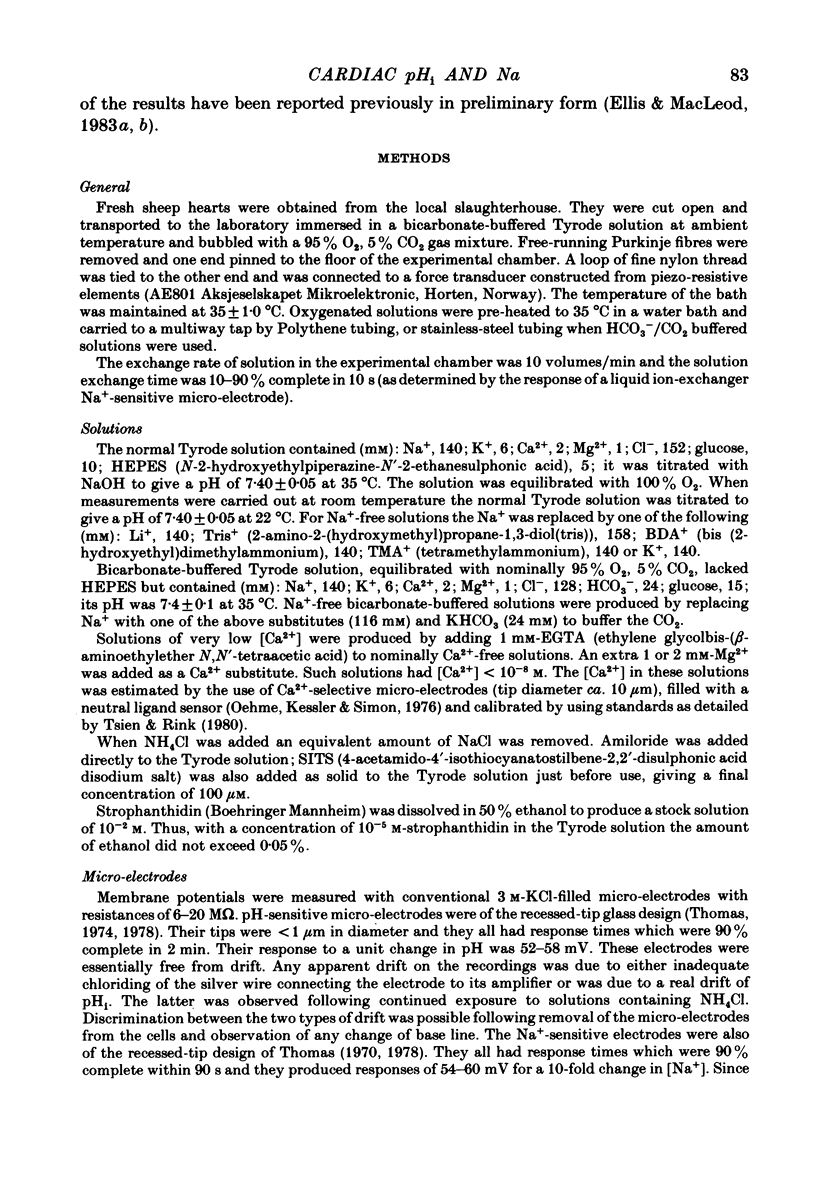
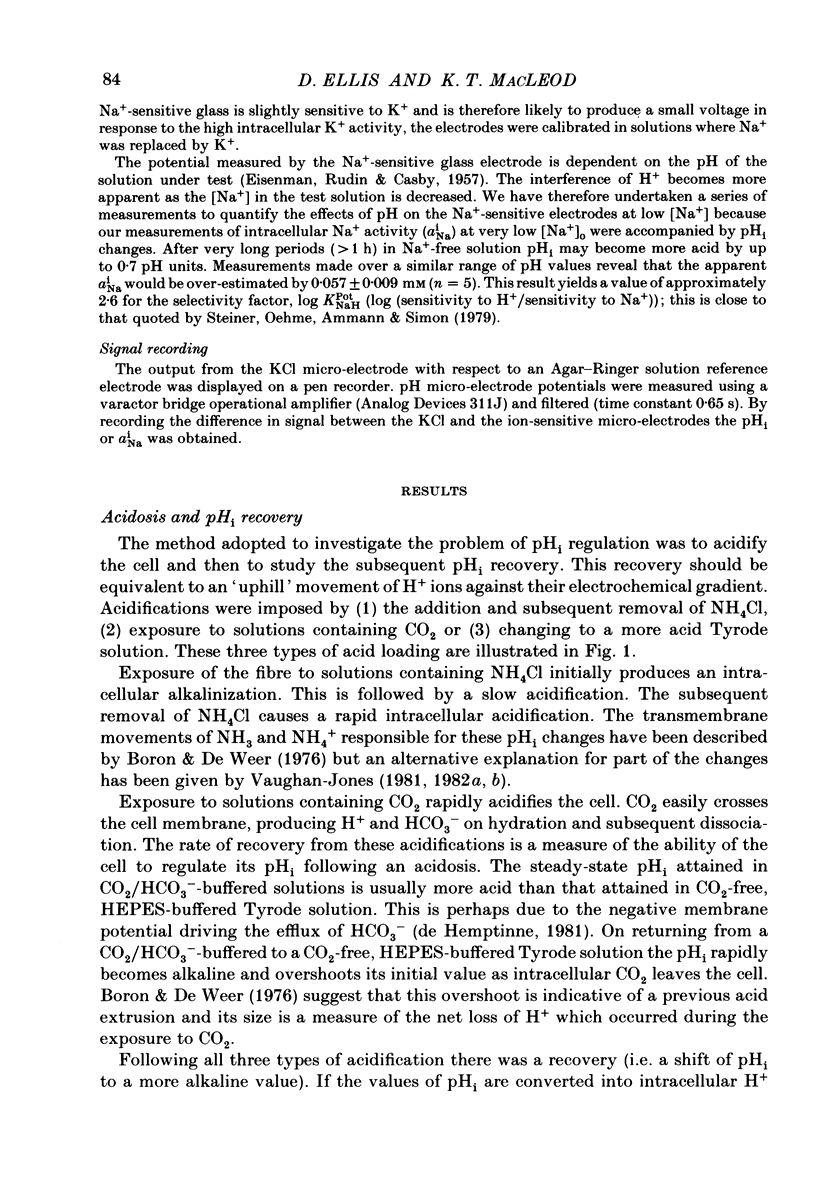
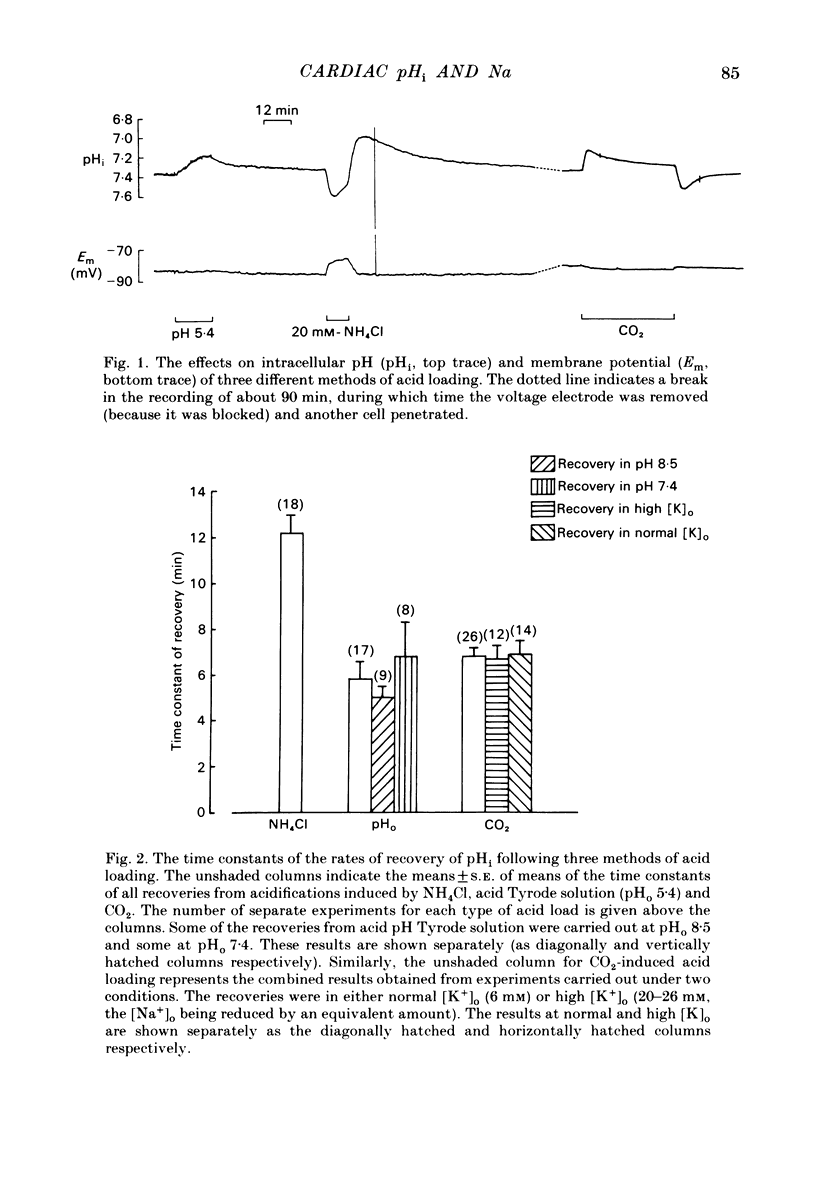
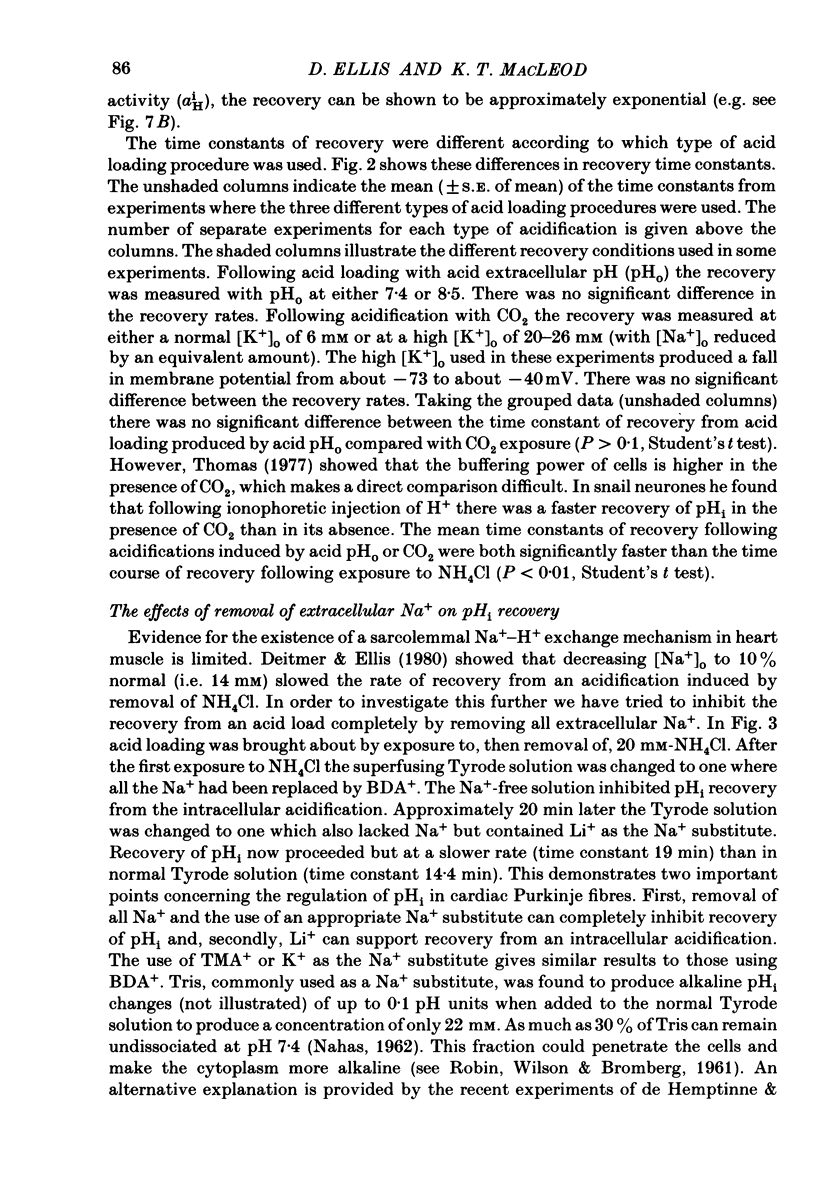
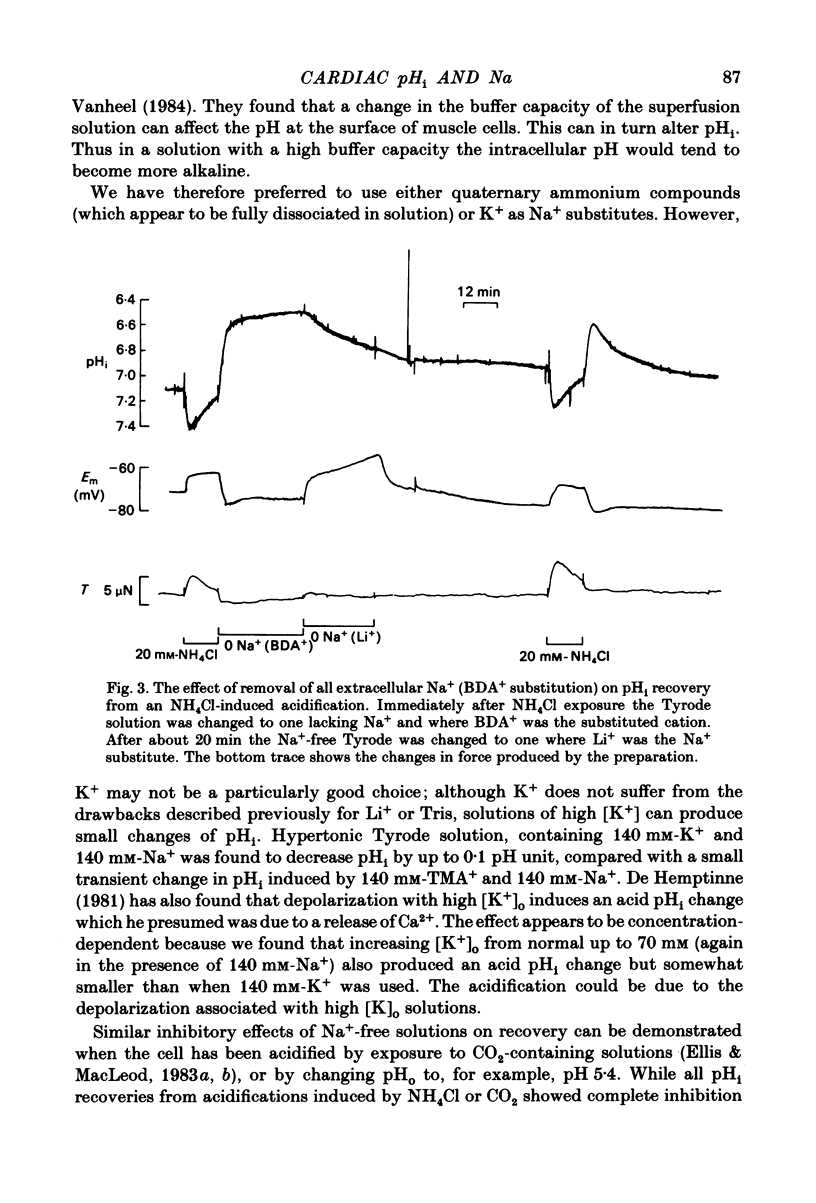
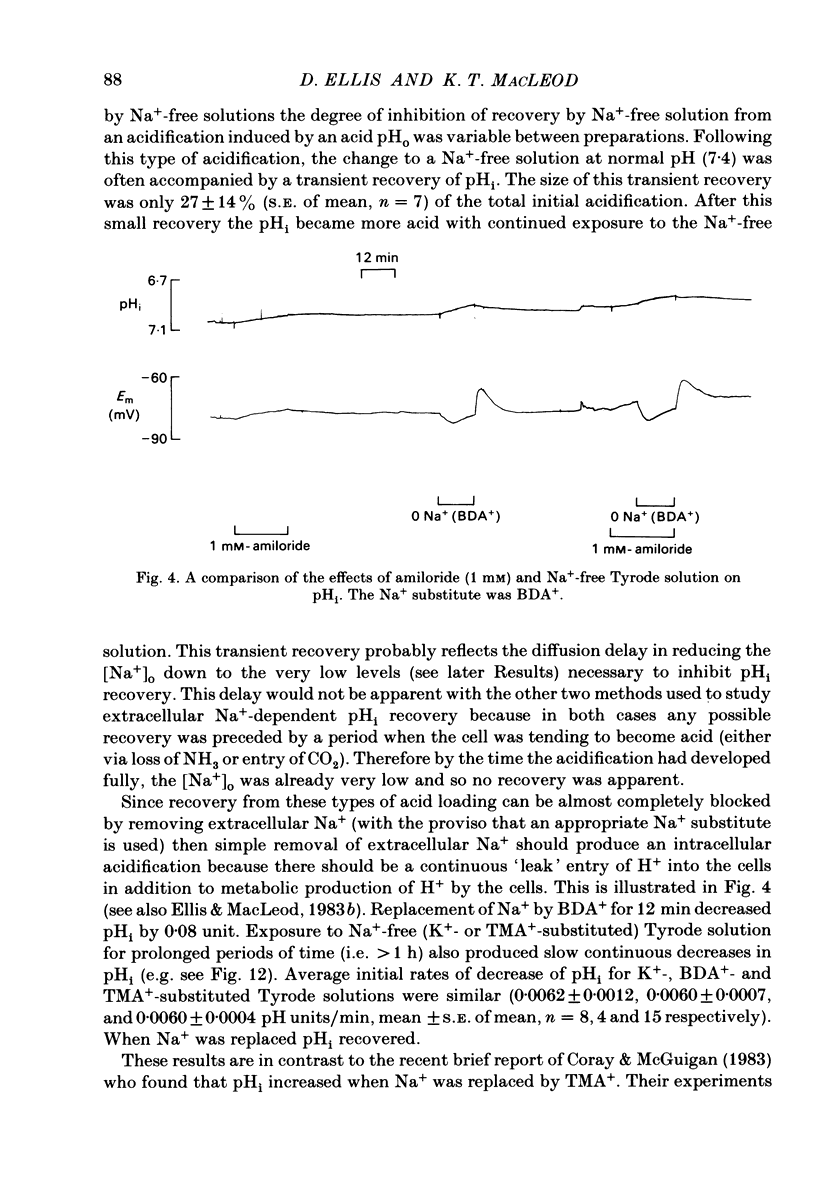
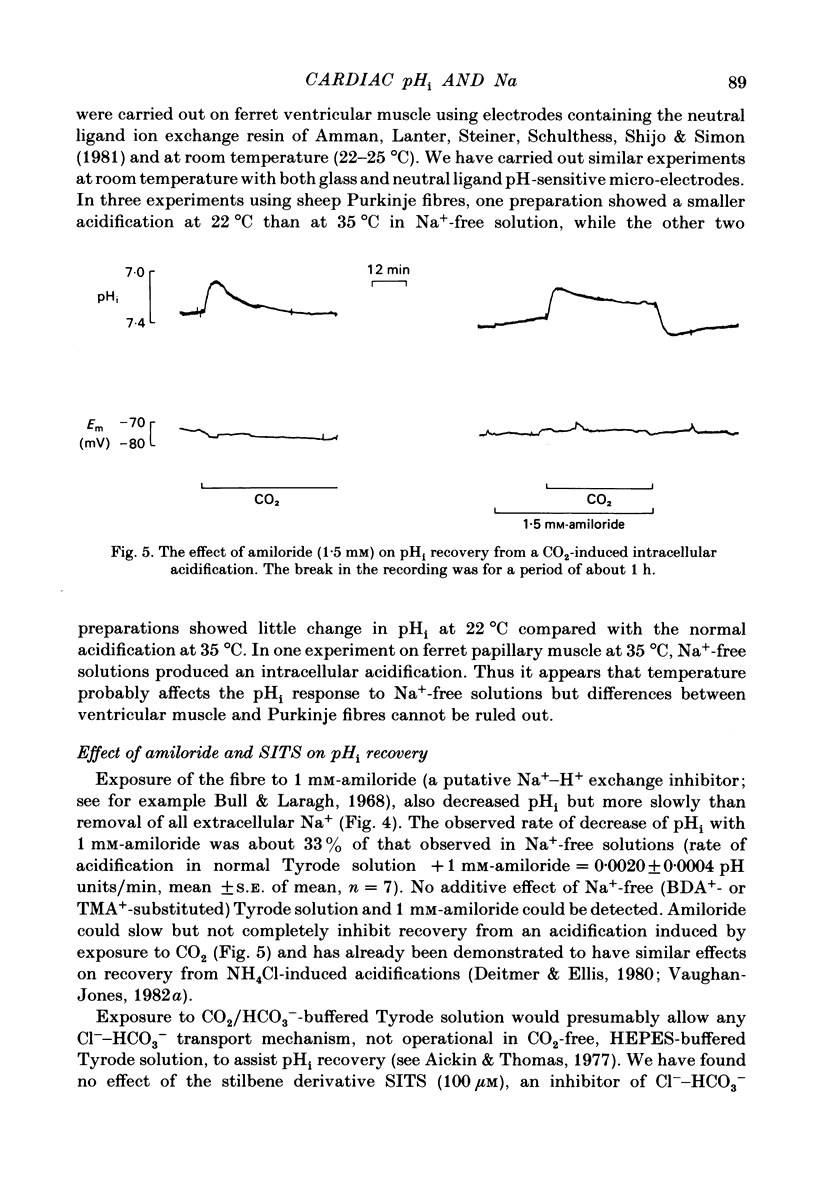
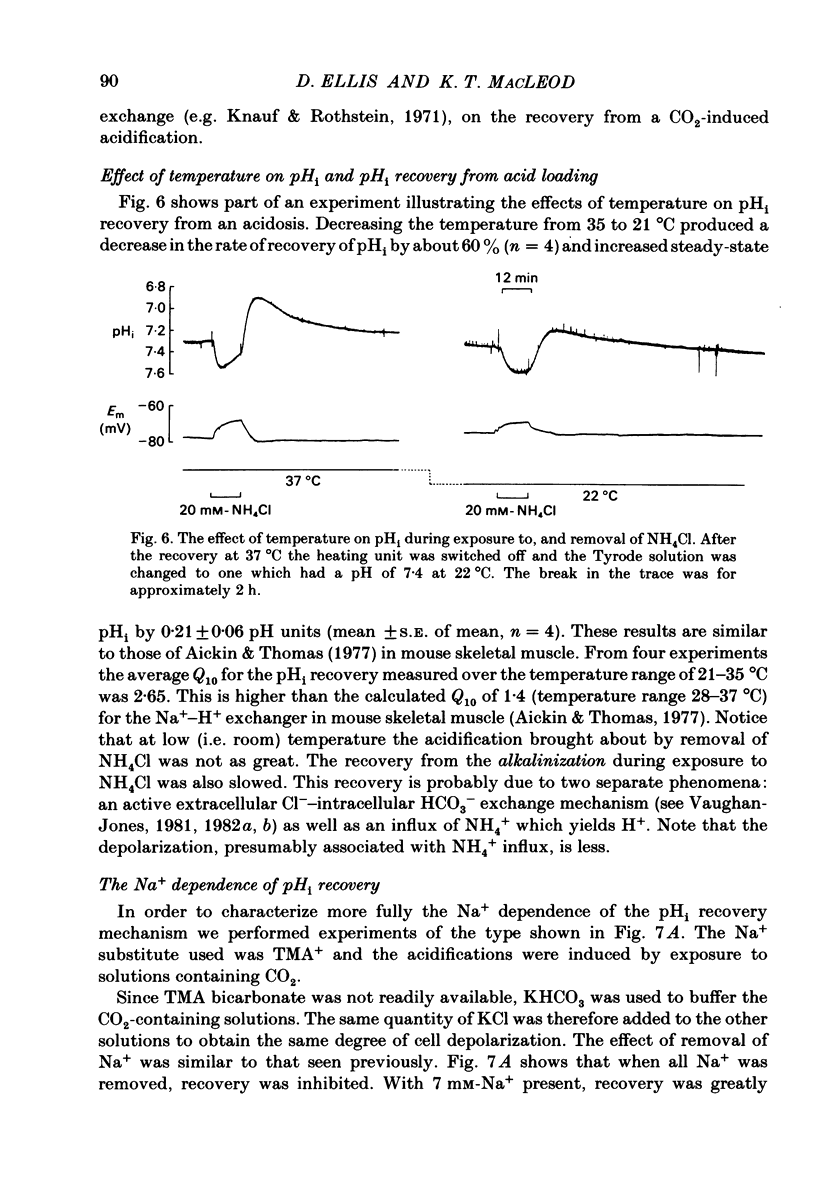
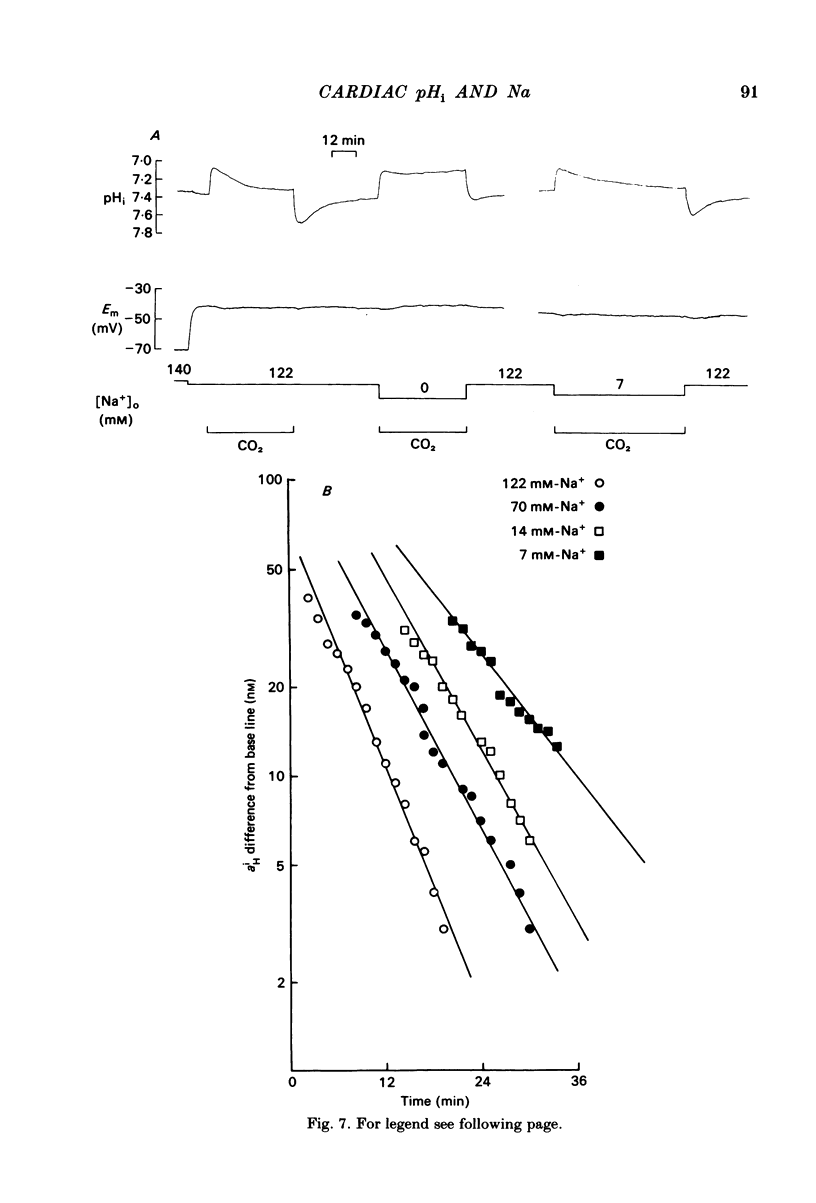
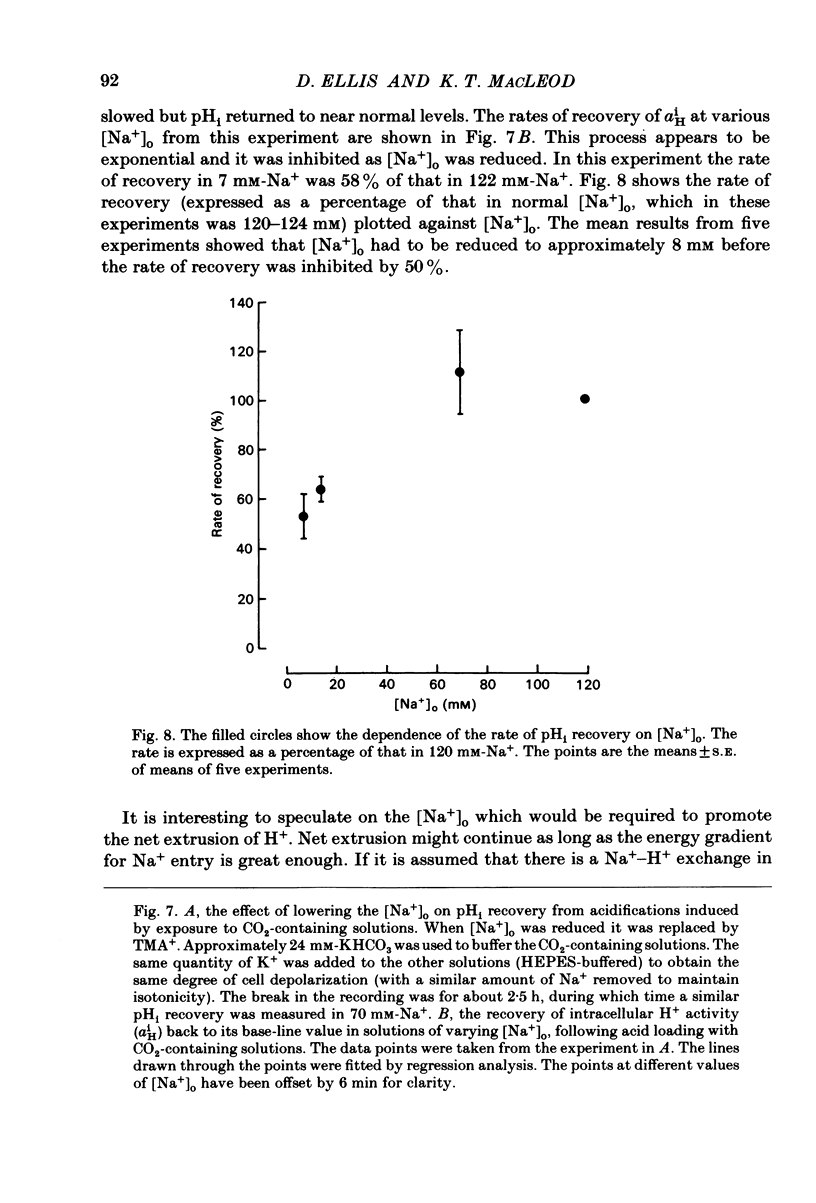
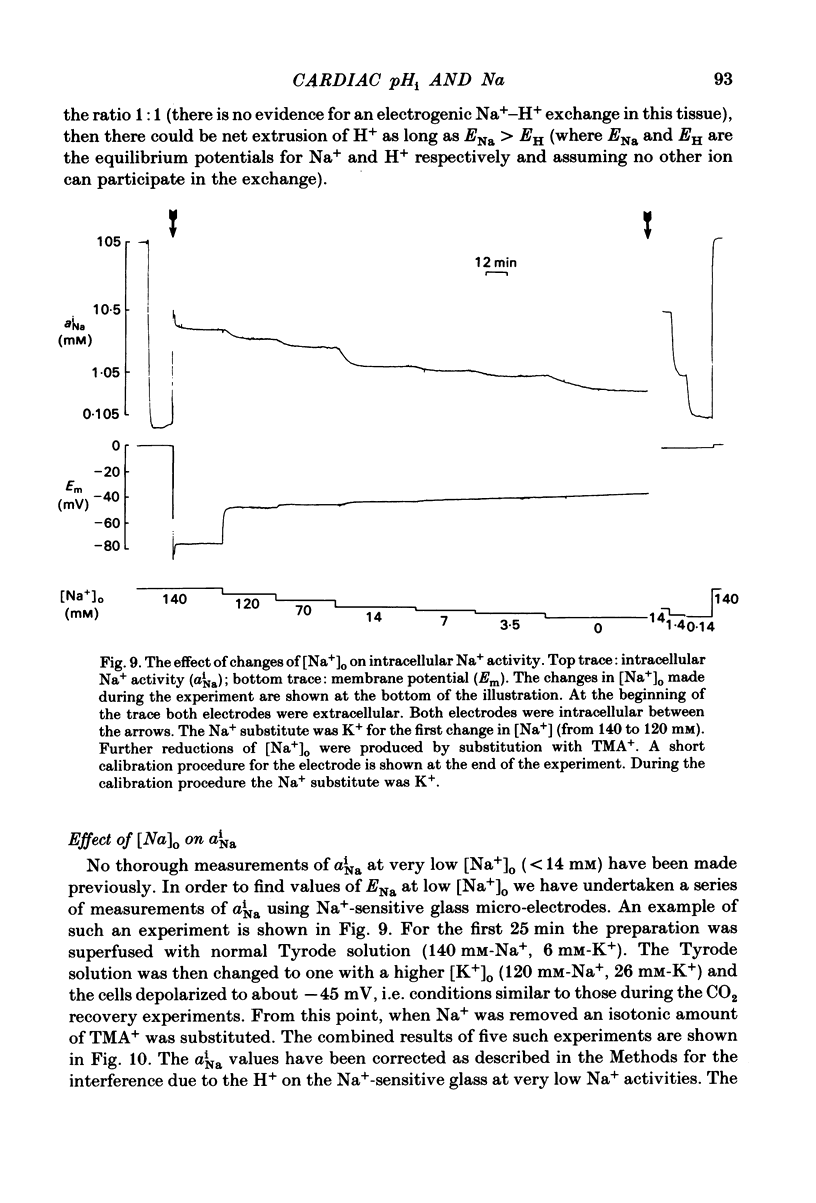
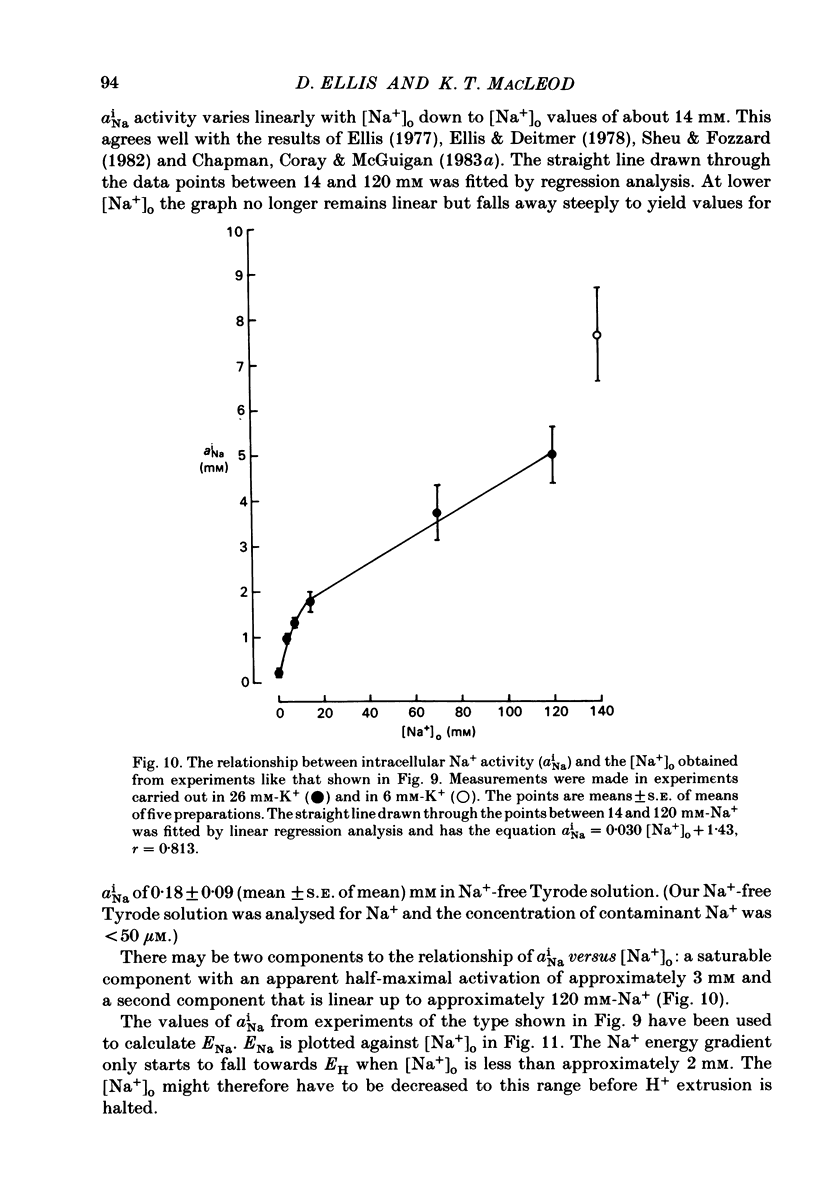
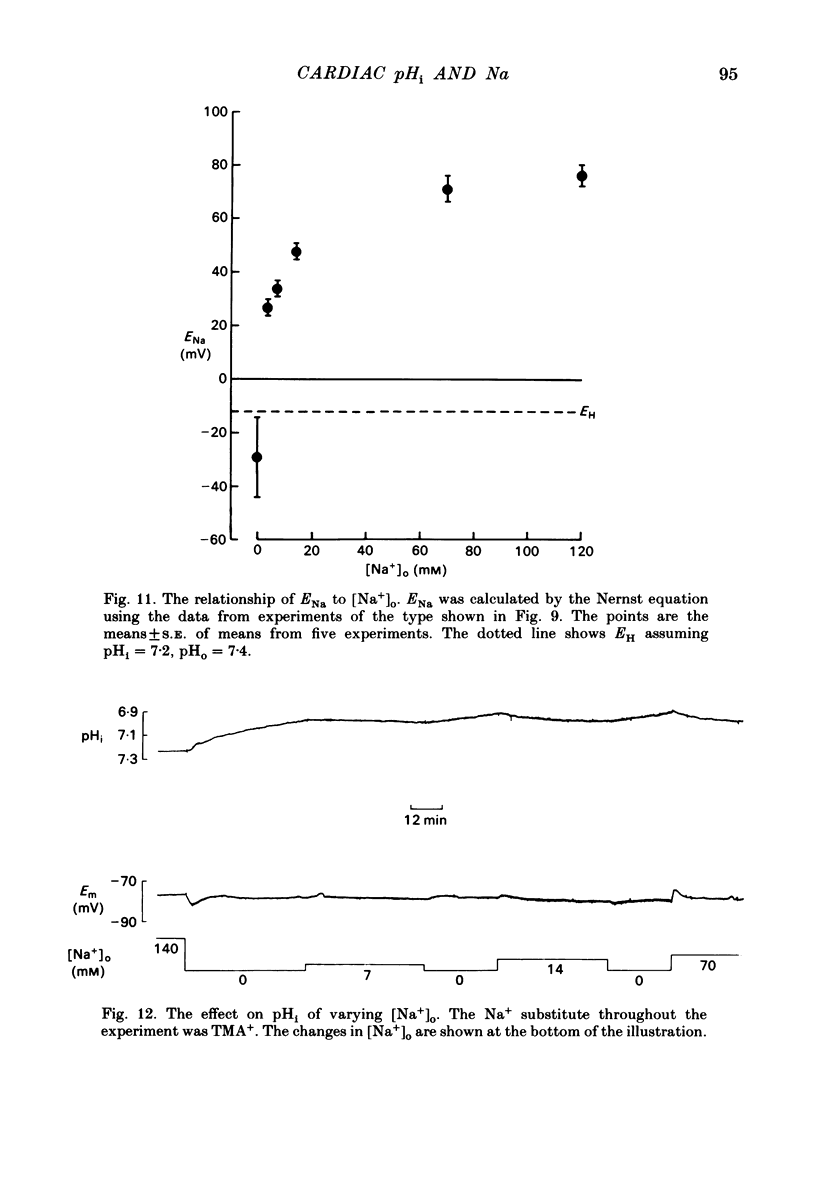
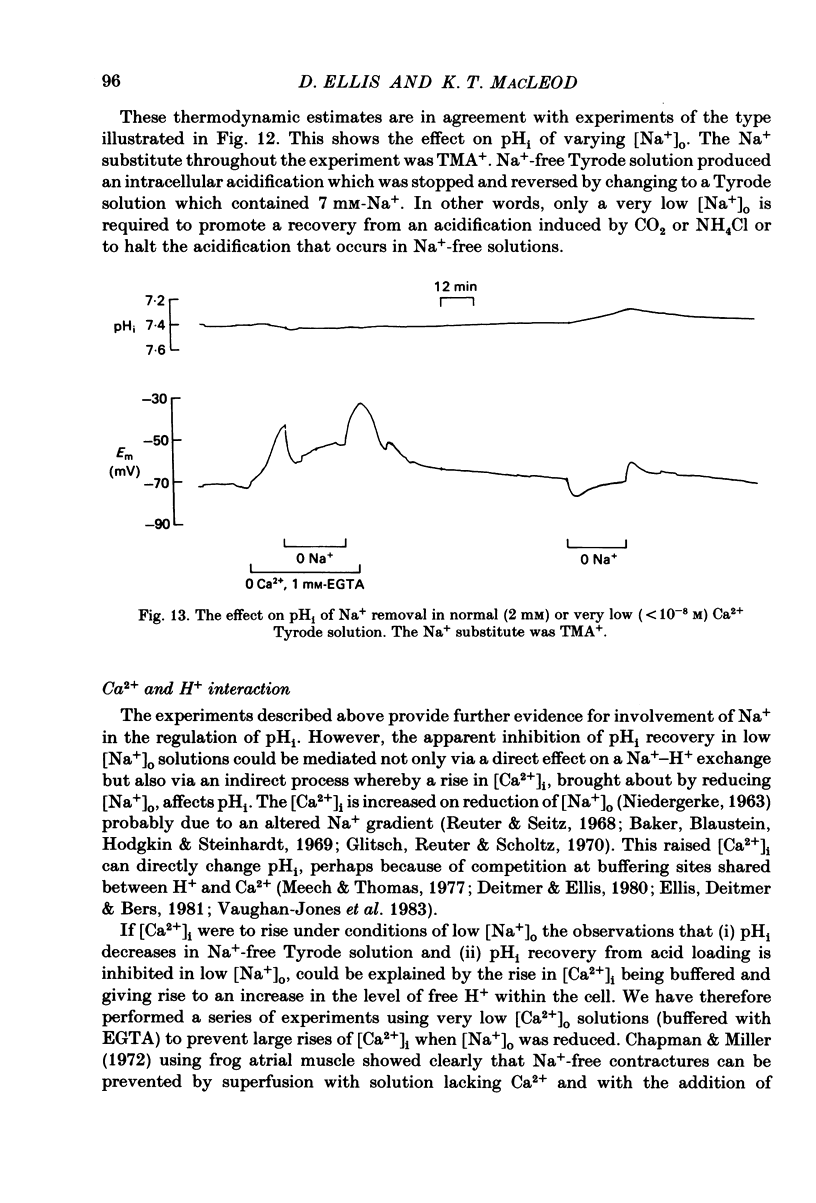
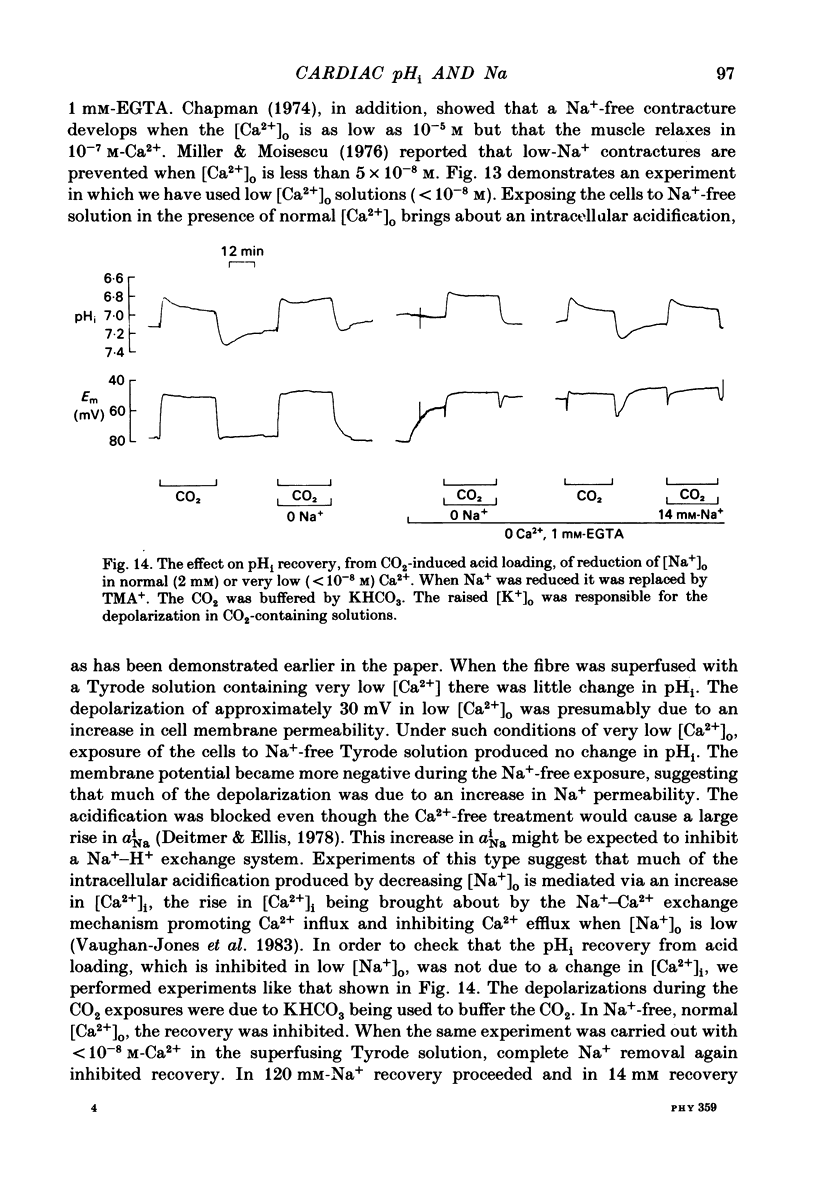
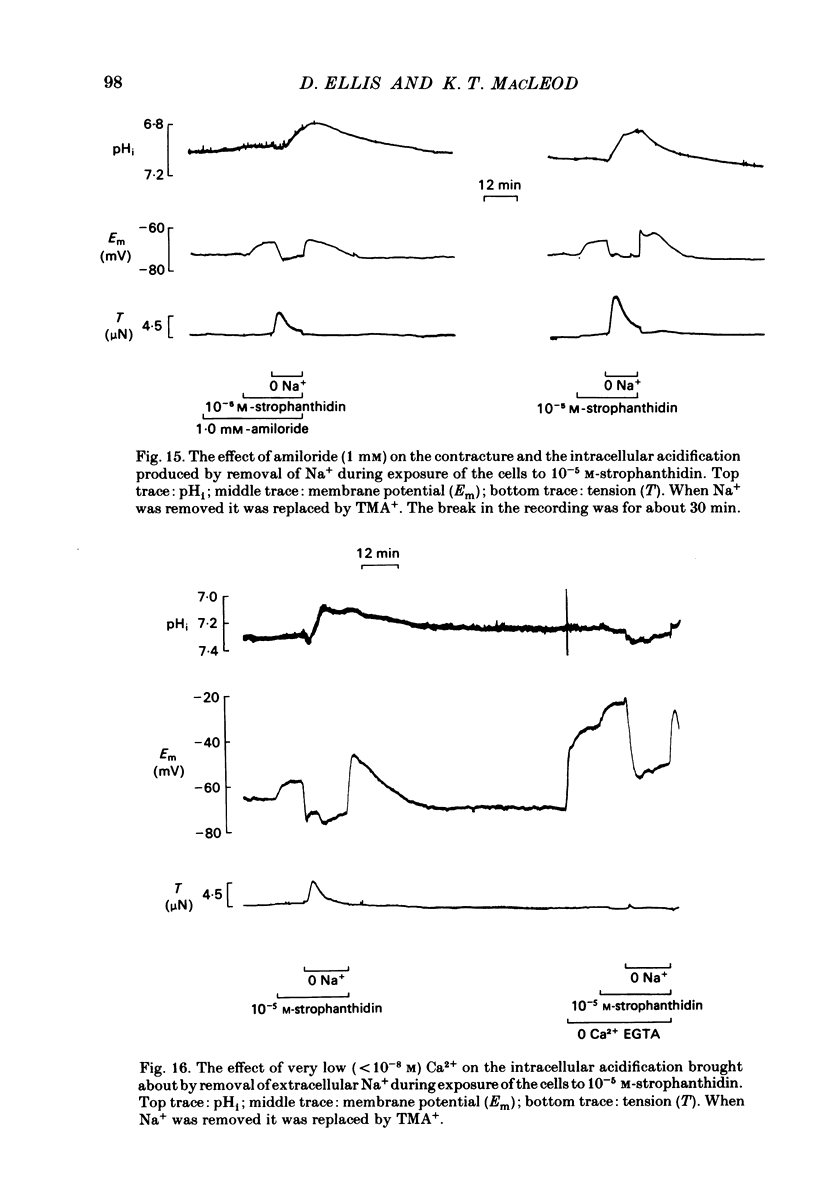
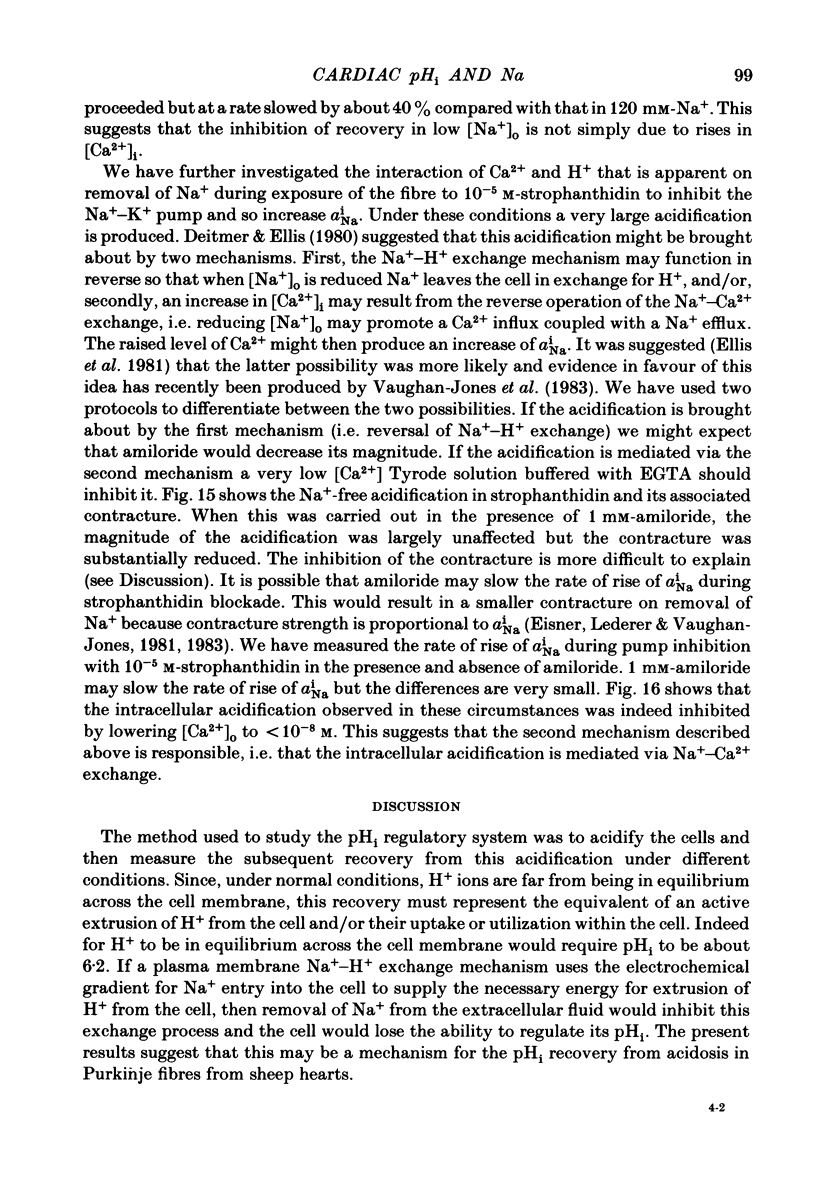
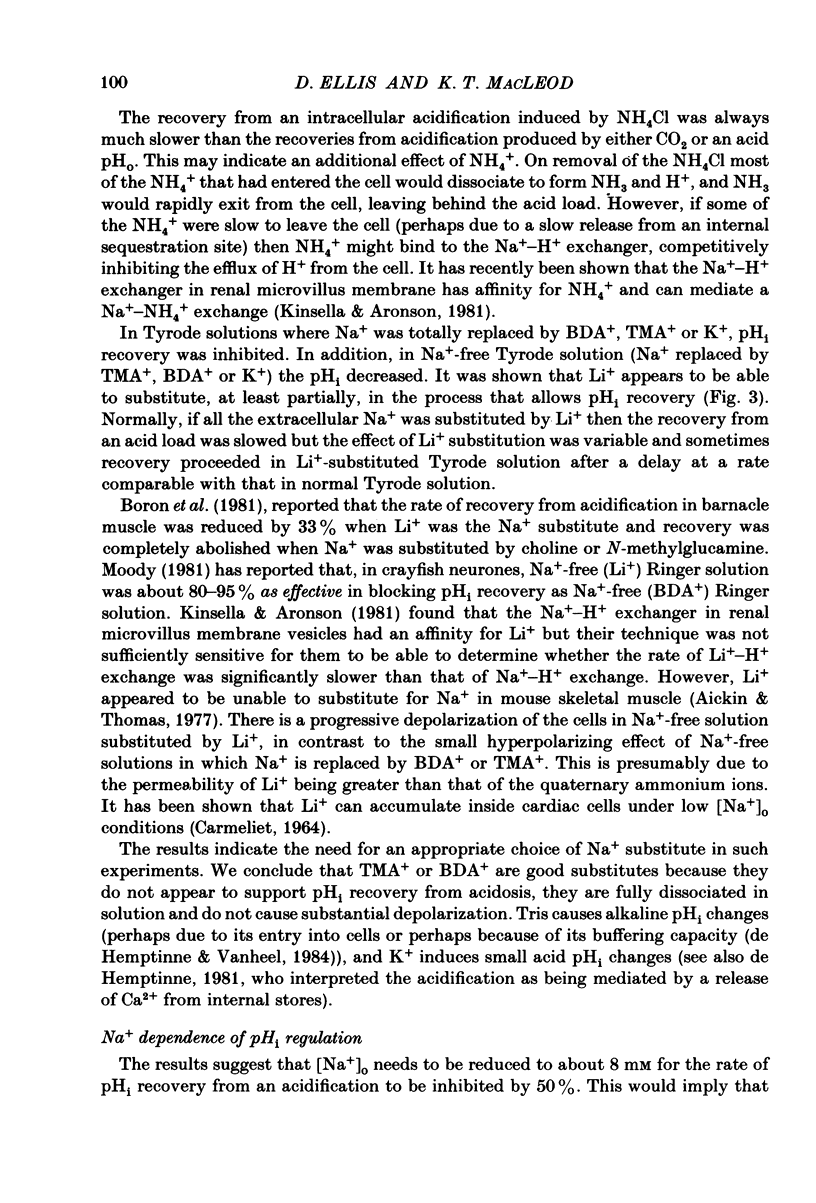
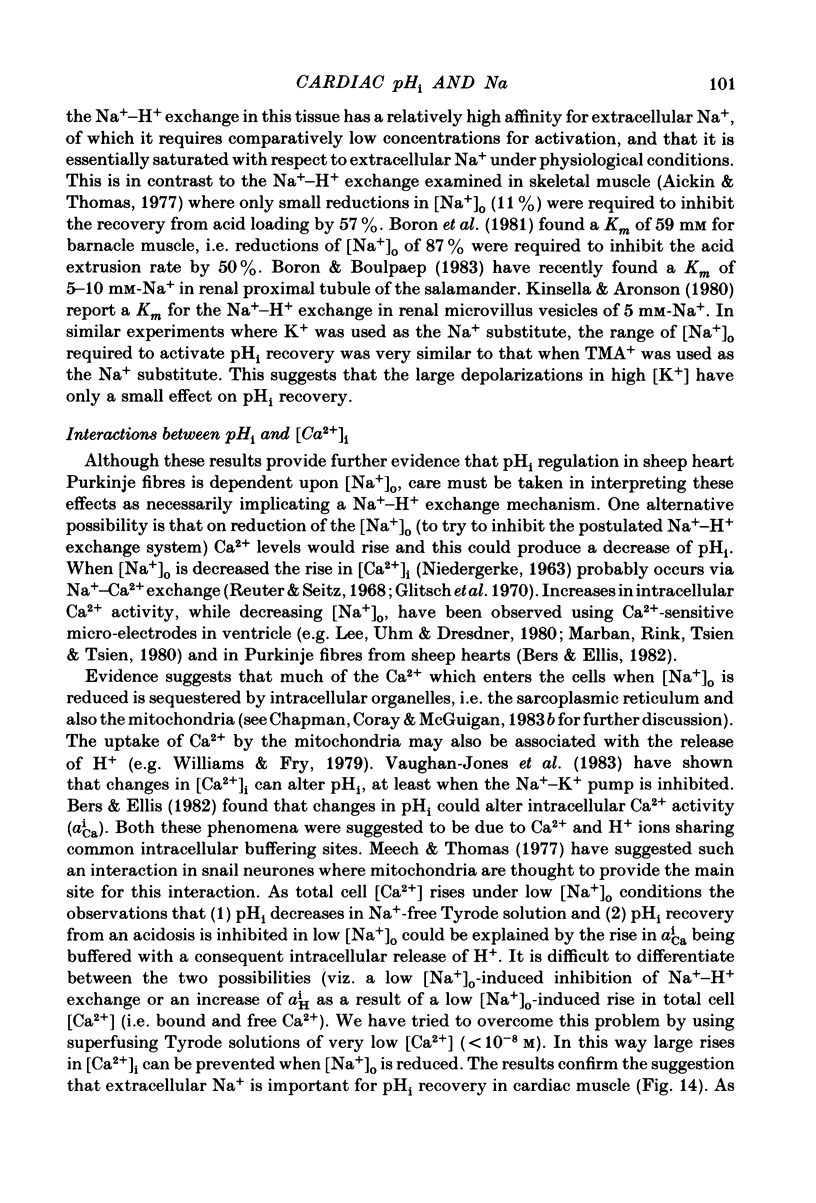
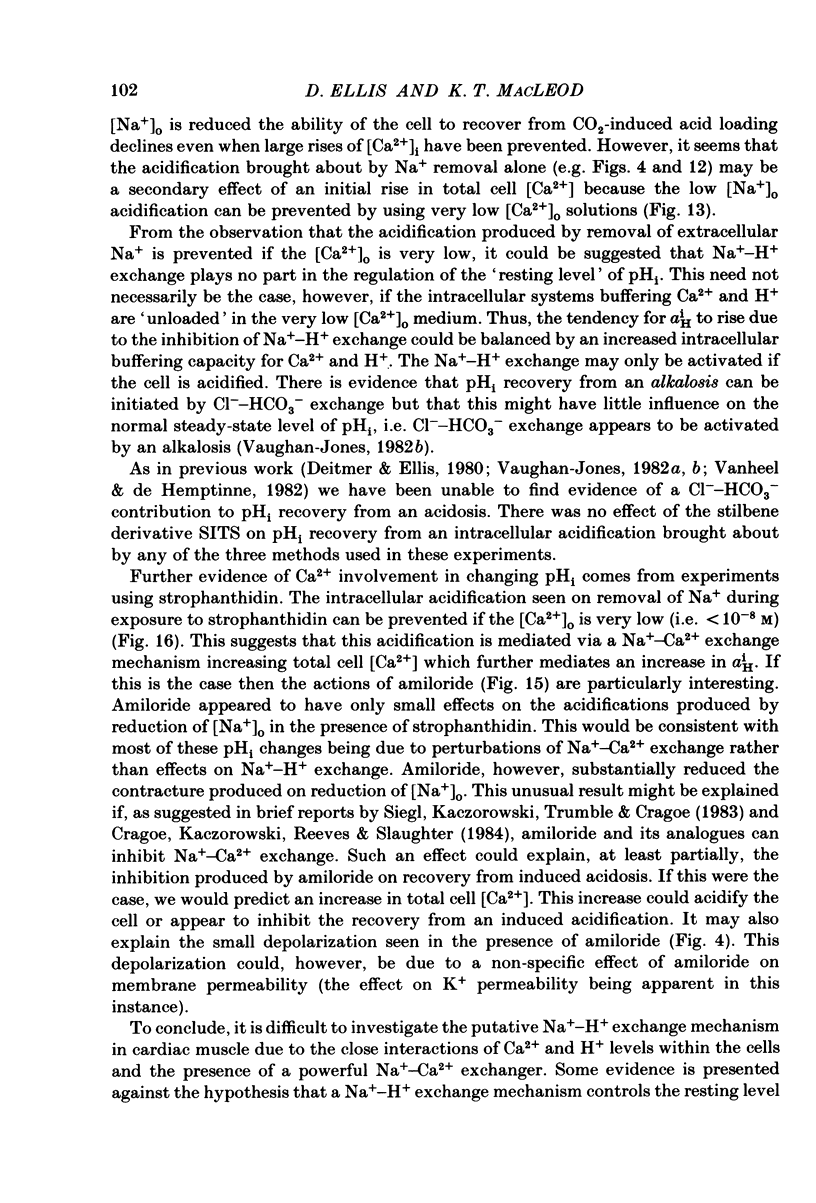
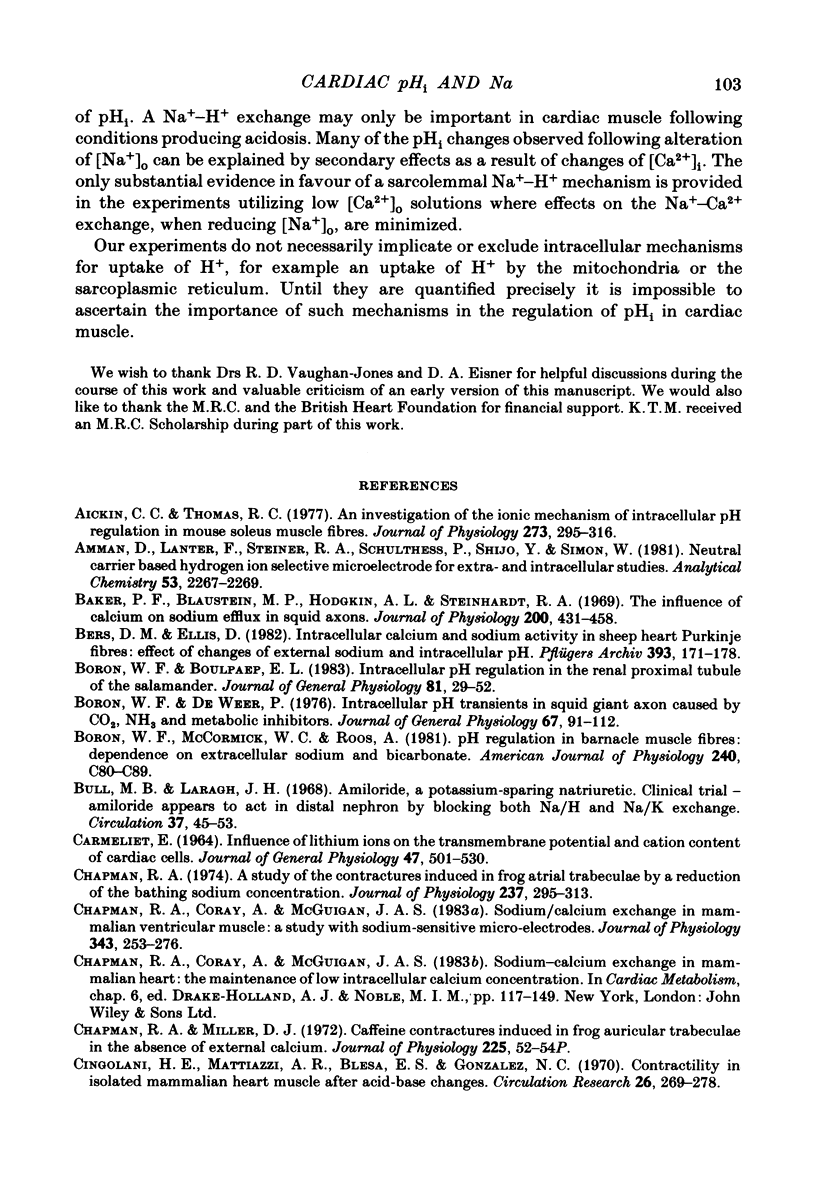
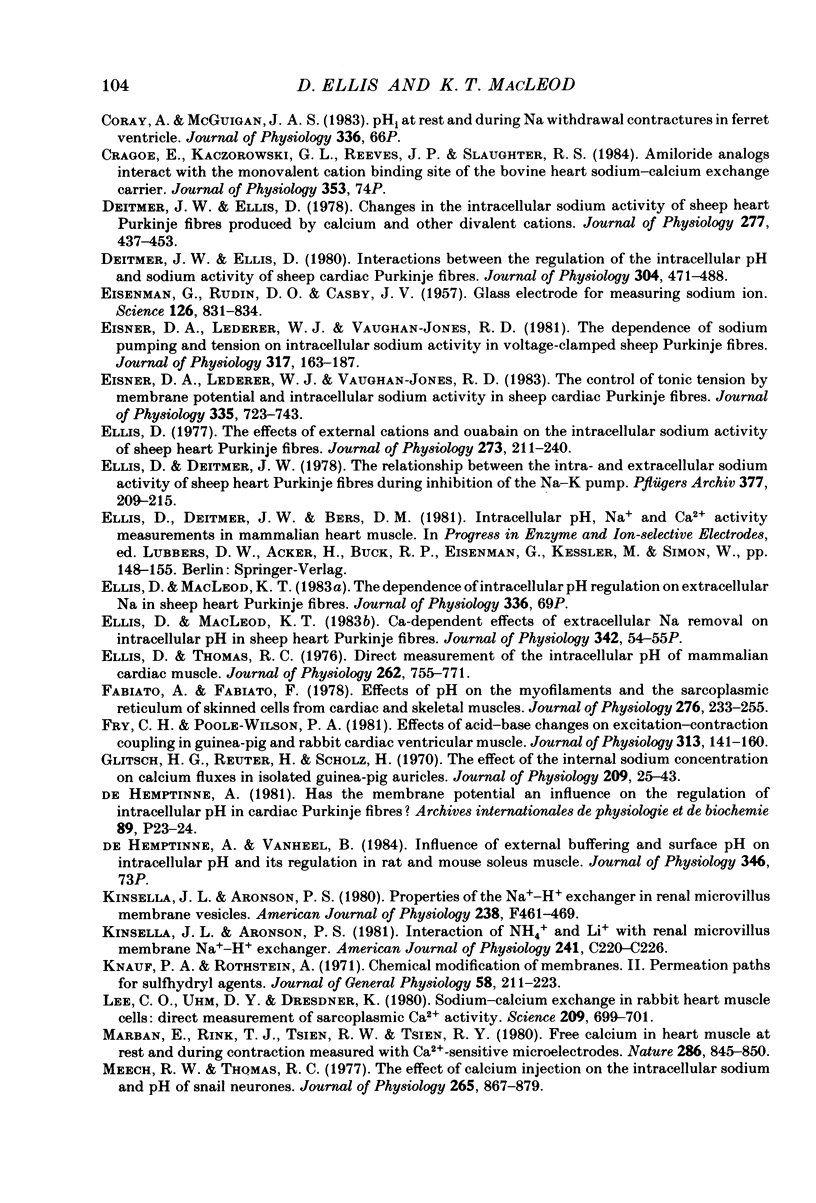
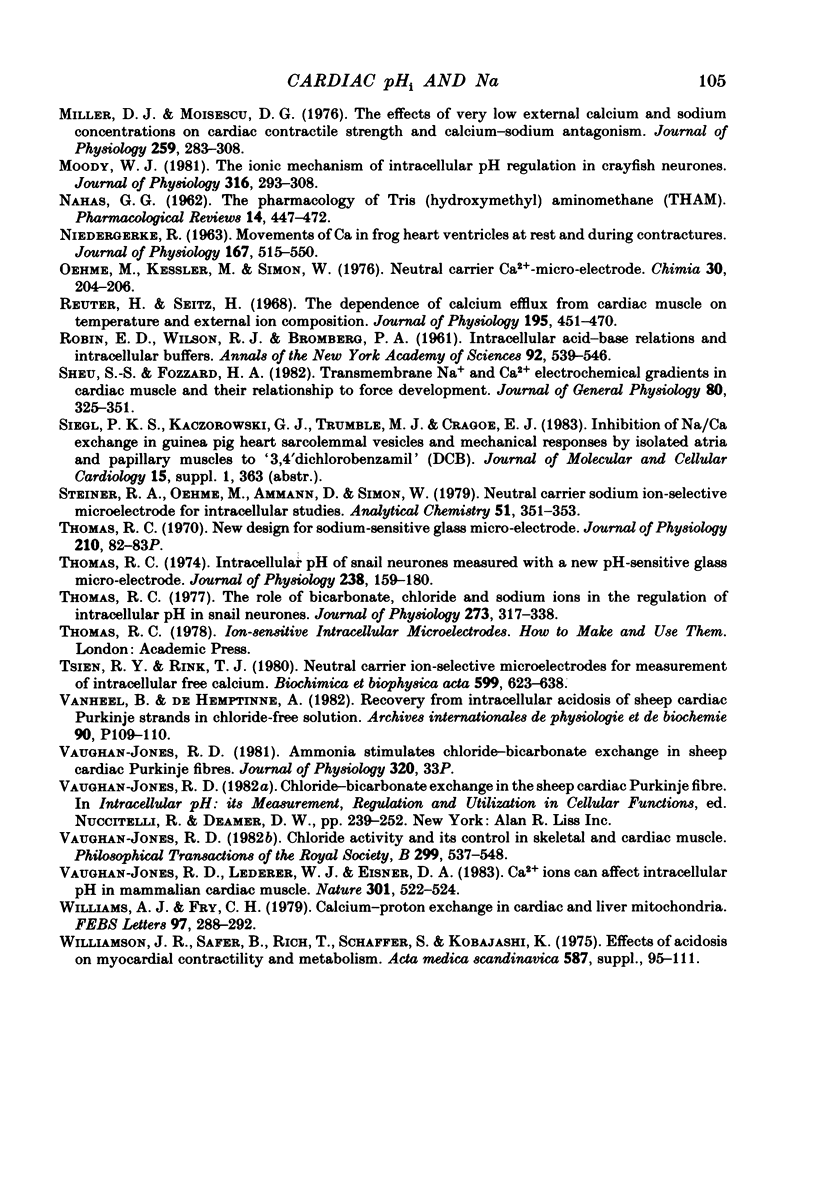
Selected References
These references are in PubMed. This may not be the complete list of references from this article.
- Aickin C. C., Thomas R. C. An investigation of the ionic mechanism of intracellular pH regulation in mouse soleus muscle fibres. J Physiol. 1977 Dec;273(1):295–316. doi: 10.1113/jphysiol.1977.sp012095. [DOI] [PMC free article] [PubMed] [Google Scholar]
- Ammann D., Lanter F., Steiner R. A., Schulthess P., Shijo Y., Simon W. Neutral carrier based hydrogen ion selective microelectrode for extra- and intracellular studies. Anal Chem. 1981 Dec;53(14):2267–2269. doi: 10.1021/ac00237a031. [DOI] [PubMed] [Google Scholar]
- Baker P. F., Blaustein M. P., Hodgkin A. L., Steinhardt R. A. The influence of calcium on sodium efflux in squid axons. J Physiol. 1969 Feb;200(2):431–458. doi: 10.1113/jphysiol.1969.sp008702. [DOI] [PMC free article] [PubMed] [Google Scholar]
- Bers D. M., Ellis D. Intracellular calcium and sodium activity in sheep heart Purkinje fibres. Effect of changes of external sodium and intracellular pH. Pflugers Arch. 1982 Apr;393(2):171–178. doi: 10.1007/BF00582941. [DOI] [PubMed] [Google Scholar]
- Bessou P., Joffroy M., Montoya R., Pagès B. Effects of triceps stretch by ankle flexion on intact afferents and efferents of gastrocnemius in the decerebrate cat. J Physiol. 1984 Jan;346:73–91. doi: 10.1113/jphysiol.1984.sp015008. [DOI] [PMC free article] [PubMed] [Google Scholar]
- Boron W. F., Boulpaep E. L. Intracellular pH regulation in the renal proximal tubule of the salamander. Na-H exchange. J Gen Physiol. 1983 Jan;81(1):29–52. doi: 10.1085/jgp.81.1.29. [DOI] [PMC free article] [PubMed] [Google Scholar]
- Boron W. F., De Weer P. Intracellular pH transients in squid giant axons caused by CO2, NH3, and metabolic inhibitors. J Gen Physiol. 1976 Jan;67(1):91–112. doi: 10.1085/jgp.67.1.91. [DOI] [PMC free article] [PubMed] [Google Scholar]
- Boron W. F., McCormick W. C., Roos A. pH regulation in barnacle muscle fibers: dependence on extracellular sodium and bicarbonate. Am J Physiol. 1981 Jan;240(1):C80–C89. doi: 10.1152/ajpcell.1981.240.1.C80. [DOI] [PubMed] [Google Scholar]
- Bull M. B., Laragh J. H. Amiloride. A potassium-sparing natriuretic agent. Circulation. 1968 Jan;37(1):45–53. doi: 10.1161/01.cir.37.1.45. [DOI] [PubMed] [Google Scholar]
- CARMELIET E. E. INFLUENCE OF LITHIUM IONS ON THE TRANSMEMBRANE POTENTIAL AND CATION CONTENT OF CARDIAC CELLS. J Gen Physiol. 1964 Jan;47:501–530. doi: 10.1085/jgp.47.3.501. [DOI] [PMC free article] [PubMed] [Google Scholar]
- Chapman R. A. A study of the contractures induced in frog atrial trabeculae by a reduction of the bathing sodium concentration. J Physiol. 1974 Mar;237(2):295–313. doi: 10.1113/jphysiol.1974.sp010483. [DOI] [PMC free article] [PubMed] [Google Scholar]
- Chapman R. A., Coray A., McGuigan J. A. Sodium/calcium exchange in mammalian ventricular muscle: a study with sodium-sensitive micro-electrodes. J Physiol. 1983 Oct;343:253–276. doi: 10.1113/jphysiol.1983.sp014891. [DOI] [PMC free article] [PubMed] [Google Scholar]
- Chapman R. A., Miller D. J. Caffeine contractures induced in frog auricular trabeculae in the absence of external calcium. J Physiol. 1972 Sep;225(2):52P–54P. [PubMed] [Google Scholar]
- Cingolani H. E., Mattiazzi A. R., Blesa E. S., Gonzalez N. C. Contractility in isolated mammalian heart muscle after acid-base changes. Circ Res. 1970 Mar;26(3):269–278. doi: 10.1161/01.res.26.3.269. [DOI] [PubMed] [Google Scholar]
- Deitmer J. W., Ellis D. Changes in the intracellular sodium activity of sheep heart Purkinje fibres produced by calcium and other divalent cations. J Physiol. 1978 Apr;277:437–453. doi: 10.1113/jphysiol.1978.sp012283. [DOI] [PMC free article] [PubMed] [Google Scholar]
- Deitmer J. W., Ellis D. Interactions between the regulation of the intracellular pH and sodium activity of sheep cardiac Purkinje fibres. J Physiol. 1980 Jul;304:471–488. doi: 10.1113/jphysiol.1980.sp013337. [DOI] [PMC free article] [PubMed] [Google Scholar]
- EISENMAN G., RUDIN D. O., CASBY J. U. Glass electrode for measuring sodium ion. Science. 1957 Oct 25;126(3278):831–834. doi: 10.1126/science.126.3278.831. [DOI] [PubMed] [Google Scholar]
- Eisner D. A., Lederer W. J., Vaughan-Jones R. D. The control of tonic tension by membrane potential and intracellular sodium activity in the sheep cardiac Purkinje fibre. J Physiol. 1983 Feb;335:723–743. doi: 10.1113/jphysiol.1983.sp014560. [DOI] [PMC free article] [PubMed] [Google Scholar]
- Eisner D. A., Lederer W. J., Vaughan-Jones R. D. The dependence of sodium pumping and tension on intracellular sodium activity in voltage-clamped sheep Purkinje fibres. J Physiol. 1981 Aug;317:163–187. doi: 10.1113/jphysiol.1981.sp013819. [DOI] [PMC free article] [PubMed] [Google Scholar]
- Ellis D., Deitmer J. W. The relationship between the intra- and extracellular sodium activity of sheep heart Purkinje fibres during inhibition of the Na-K pump. Pflugers Arch. 1978 Nov 30;377(3):209–215. doi: 10.1007/BF00584274. [DOI] [PubMed] [Google Scholar]
- Ellis D. The effects of external cations and ouabain on the intracellular sodium activity of sheep heart Purkinje fibres. J Physiol. 1977 Dec;273(1):211–240. doi: 10.1113/jphysiol.1977.sp012090. [DOI] [PMC free article] [PubMed] [Google Scholar]
- Ellis D., Thomas R. C. Direct measurement of the intracellular pH of mammalian cardiac muscle. J Physiol. 1976 Nov;262(3):755–771. doi: 10.1113/jphysiol.1976.sp011619. [DOI] [PMC free article] [PubMed] [Google Scholar]
- Fabiato A., Fabiato F. Effects of pH on the myofilaments and the sarcoplasmic reticulum of skinned cells from cardiace and skeletal muscles. J Physiol. 1978 Mar;276:233–255. doi: 10.1113/jphysiol.1978.sp012231. [DOI] [PMC free article] [PubMed] [Google Scholar]
- Fry C. H., Poole-Wilson P. A. Effects of acid-base changes on excitation--contraction coupling in guinea-pig and rabbit cardiac ventricular muscle. J Physiol. 1981;313:141–160. doi: 10.1113/jphysiol.1981.sp013655. [DOI] [PMC free article] [PubMed] [Google Scholar]
- Glitsch H. G., Reuter H., Scholz H. The effect of the internal sodium concentration on calcium fluxes in isolated guinea-pig auricles. J Physiol. 1970 Jul;209(1):25–43. doi: 10.1113/jphysiol.1970.sp009153. [DOI] [PMC free article] [PubMed] [Google Scholar]
- Kinsella J. L., Aronson P. S. Interaction of NH4+ and Li+ with the renal microvillus membrane Na+-H+ exchanger. Am J Physiol. 1981 Nov;241(5):C220–C226. doi: 10.1152/ajpcell.1981.241.5.C220. [DOI] [PubMed] [Google Scholar]
- Kinsella J. L., Aronson P. S. Properties of the Na+-H+ exchanger in renal microvillus membrane vesicles. Am J Physiol. 1980 Jun;238(6):F461–F469. doi: 10.1152/ajprenal.1980.238.6.F461. [DOI] [PubMed] [Google Scholar]
- Knauf P. A., Rothstein A. Chemical modification of membranes. II. Permeation paths for sulfhydryl agents. J Gen Physiol. 1971 Aug;58(2):211–223. doi: 10.1085/jgp.58.2.211. [DOI] [PMC free article] [PubMed] [Google Scholar]
- Lee C. O., Uhm D. Y., Dresdner K. Sodium-calcium exchange in rabbit heart muscle cells: direct measurement of sarcoplasmic Ca2+ activity. Science. 1980 Aug 8;209(4457):699–701. doi: 10.1126/science.7394527. [DOI] [PubMed] [Google Scholar]
- Marban E., Rink T. J., Tsien R. W., Tsien R. Y. Free calcium in heart muscle at rest and during contraction measured with Ca2+ -sensitive microelectrodes. Nature. 1980 Aug 28;286(5776):845–850. doi: 10.1038/286845a0. [DOI] [PubMed] [Google Scholar]
- Meech R. W., Thomas R. C. The effect of calcium injection on the intracellular sodium and pH of snail neurones. J Physiol. 1977 Mar;265(3):867–879. doi: 10.1113/jphysiol.1977.sp011749. [DOI] [PMC free article] [PubMed] [Google Scholar]
- Miller D. J., Moisescu D. G. The effects of very low external calcium and sodium concentrations on cardiac contractile strength and calcium-sodium antagonism. J Physiol. 1976 Jul;259(2):283–308. doi: 10.1113/jphysiol.1976.sp011466. [DOI] [PMC free article] [PubMed] [Google Scholar]
- Moody W. J., Jr The ionic mechanism of intracellular pH regulation in crayfish neurones. J Physiol. 1981 Jul;316:293–308. doi: 10.1113/jphysiol.1981.sp013788. [DOI] [PMC free article] [PubMed] [Google Scholar]
- NAHAS G. G. The pharmacology of tris(hydroxymethyl) aminomethane (THAM). Pharmacol Rev. 1962 Sep;14:447–472. [PubMed] [Google Scholar]
- NIEDERGERKE R. MOVEMENTS OF CA IN FROG HEART VENTRICLES AT REST AND DURING CONTRACTURES. J Physiol. 1963 Jul;167:515–550. doi: 10.1113/jphysiol.1963.sp007166. [DOI] [PMC free article] [PubMed] [Google Scholar]
- ROBIN E. D., WILSON R. J., BROMBERG P. A. Intracellular acidbase relations and intracellular buffers. Ann N Y Acad Sci. 1961 Jun 17;92:539–546. doi: 10.1111/j.1749-6632.1961.tb45004.x. [DOI] [PubMed] [Google Scholar]
- Reuter H., Seitz N. The dependence of calcium efflux from cardiac muscle on temperature and external ion composition. J Physiol. 1968 Mar;195(2):451–470. doi: 10.1113/jphysiol.1968.sp008467. [DOI] [PMC free article] [PubMed] [Google Scholar]
- Sheu S. S., Fozzard H. A. Transmembrane Na+ and Ca2+ electrochemical gradients in cardiac muscle and their relationship to force development. J Gen Physiol. 1982 Sep;80(3):325–351. doi: 10.1085/jgp.80.3.325. [DOI] [PMC free article] [PubMed] [Google Scholar]
- Thomas R. C. Intracellular pH of snail neurones measured with a new pH-sensitive glass mirco-electrode. J Physiol. 1974 Apr;238(1):159–180. doi: 10.1113/jphysiol.1974.sp010516. [DOI] [PMC free article] [PubMed] [Google Scholar]
- Thomas R. C. New design for sodium-sensitive glass micro-electrode. J Physiol. 1970 Sep;210(2):82P–83P. [PubMed] [Google Scholar]
- Thomas R. C. The role of bicarbonate, chloride and sodium ions in the regulation of intracellular pH in snail neurones. J Physiol. 1977 Dec;273(1):317–338. doi: 10.1113/jphysiol.1977.sp012096. [DOI] [PMC free article] [PubMed] [Google Scholar]
- Tsien R. Y., Rink T. J. Neutral carrier ion-selective microelectrodes for measurement of intracellular free calcium. Biochim Biophys Acta. 1980 Jul;599(2):623–638. doi: 10.1016/0005-2736(80)90205-9. [DOI] [PubMed] [Google Scholar]
- Vaughan-Jones R. D. Chloride activity and its control in skeletal and cardiac muscle. Philos Trans R Soc Lond B Biol Sci. 1982 Dec 1;299(1097):537–548. doi: 10.1098/rstb.1982.0150. [DOI] [PubMed] [Google Scholar]
- Vaughan-Jones R. D., Lederer W. J., Eisner D. A. Ca2+ ions can affect intracellular pH in mammalian cardiac muscle. Nature. 1983 Feb 10;301(5900):522–524. doi: 10.1038/301522a0. [DOI] [PubMed] [Google Scholar]
- Williams A. J., Fry C. H. Calcium-proton exchange in cardiac and liver mitochondria. FEBS Lett. 1979 Jan 15;97(2):288–292. doi: 10.1016/0014-5793(79)80104-0. [DOI] [PubMed] [Google Scholar]