Abstract
The effects of ion substitution, inhibitors and variations in transmural p.d. on the movements of sodium and chloride across an in vitro preparation of rat gastric mucosa have been studied. The tissue maintained net steady-state transport of sodium in the mucosal-to-serosal direction in the absence of transmural gradients of electrochemical potential. Sodium transport was independent of the presence of chloride, and was abolished by 1 X 10(-5) M-amiloride. The inhibitor produced a decrease in short-circuit current equivalent to the depression of sodium transport, indicating that the sodium transport process was electrogenic. Variations in transmural p.d. showed that the sodium transport process included two components: one that varied with p.d. and one that was independent of it. These findings have been interpreted in terms of a system for sodium transport composed of three components: two rate-limiting entry mechanisms at the apical membrane, one of which can be represented as a conductive channel for sodium diffusion and the other as a neutral process possibly a sodium-hydrogen exchanger, and a voltage-independent pump at the basolateral membrane analogous to the constant-current pump models described in some other epithelia. The tissue maintained a net secretory movement of chloride in the short-circuited condition. The process responsible for net transport of chloride could be resolved into two components: one that was sodium dependent, electrogenic, and abolished by 8 X 10(-3) M-acetazolamide, and one that was independent of the presence of sodium, electrically silent and abolished by 5 X 10(-4) M-SITS (4-acetamido-4'-isothiocyano-2,2'-disulphonic acid stilbene). Both components of the chloride transport process varied with p.d. These findings were interpreted in terms of a system of three components: two entry mechanisms at the basolateral membrane including a coupled sodium-chloride influx process and a chloride-bicarbonate exchanger in parallel, and a rate-limiting conductive channel at the apical membrane. In addition, the studies on the effects of variations in transmural p.d. on chloride fluxes revealed a symmetrical voltage-independent component, dependent on the presence of chloride in the trans compartment, and it was suggested that this component may reflect the presence of a chloride-chloride exchange mechanism.
Full text
PDF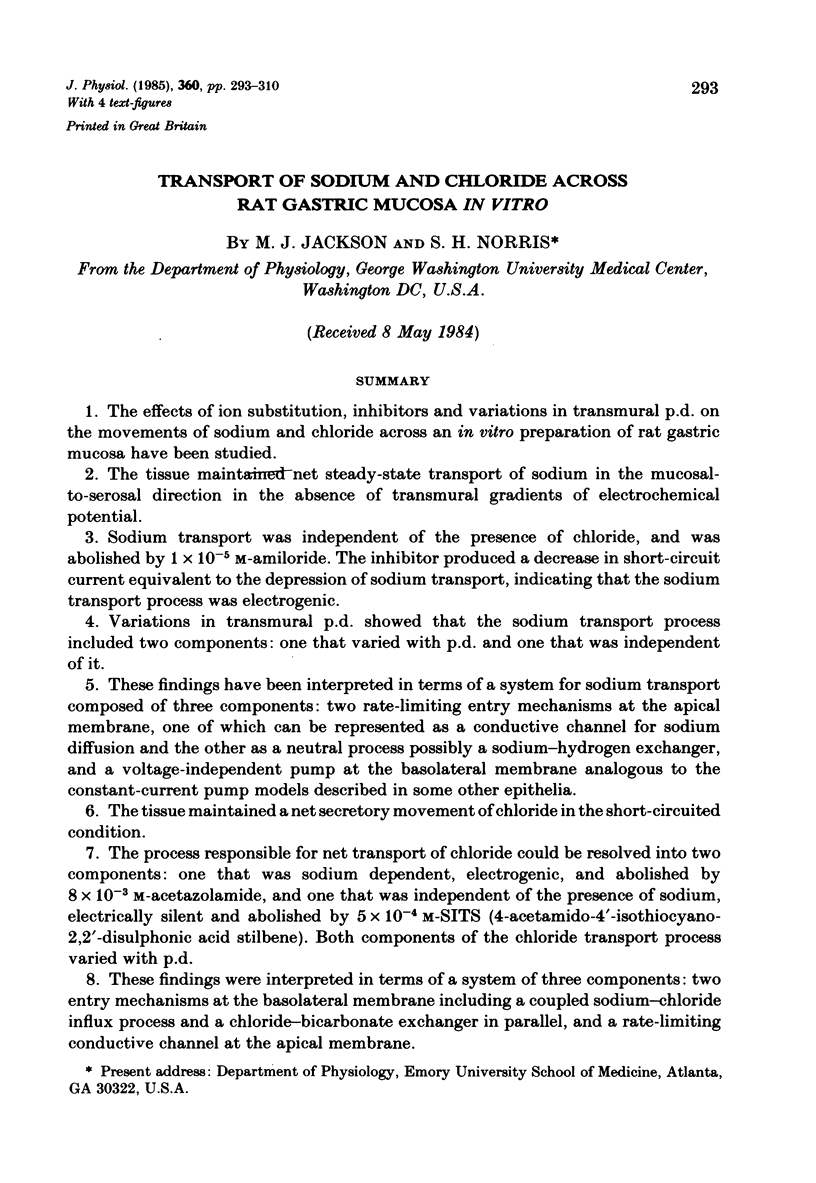
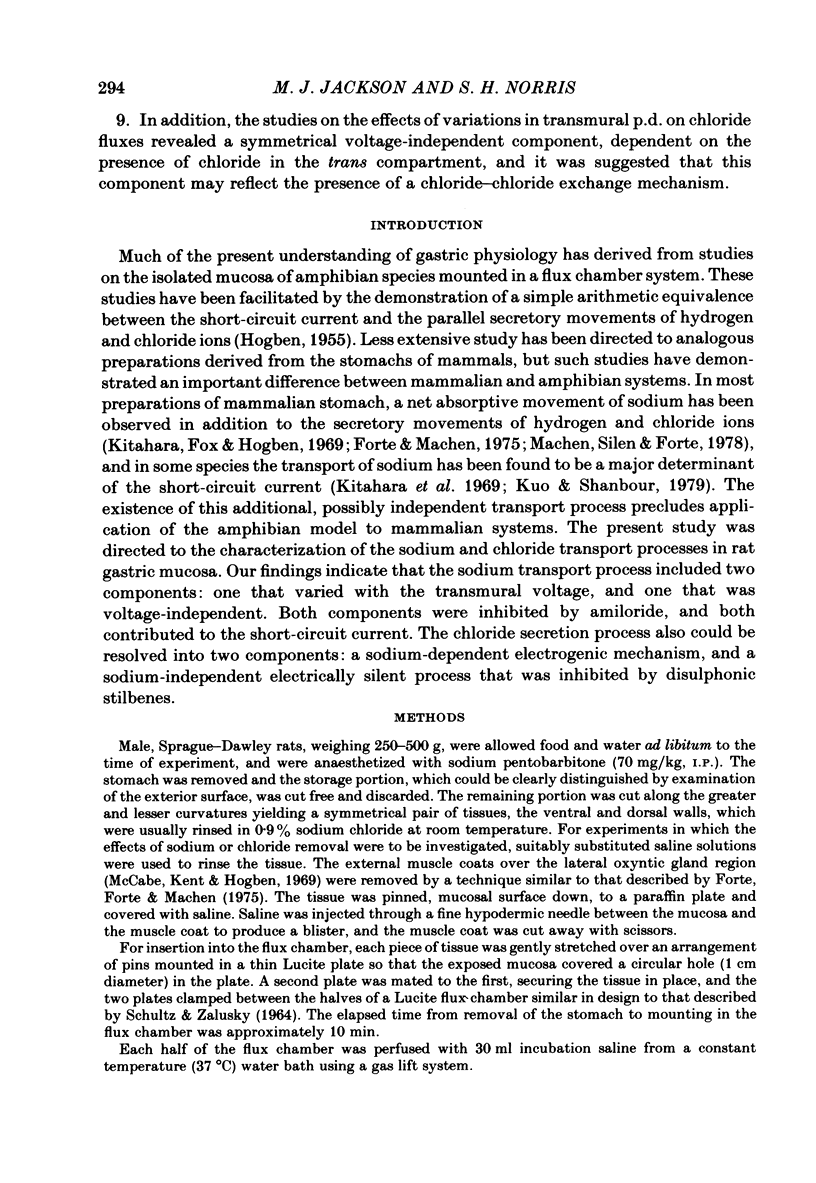
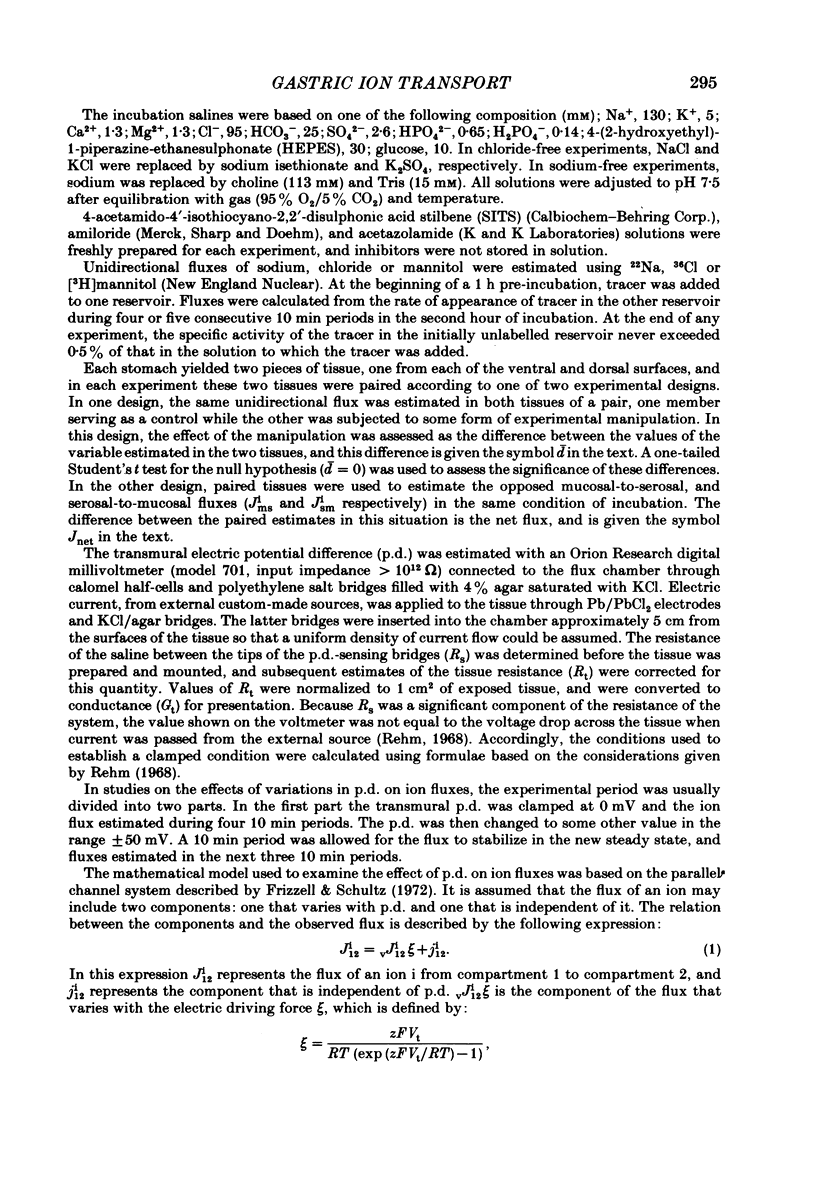
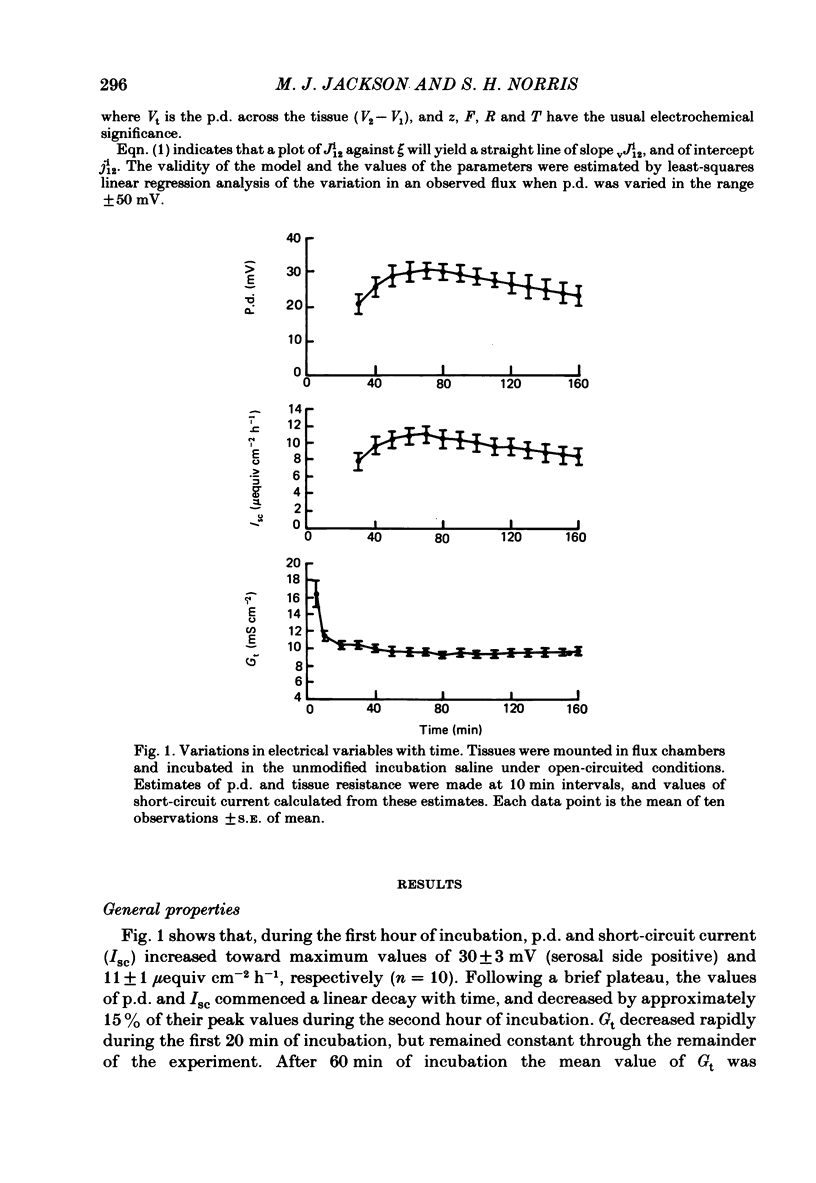
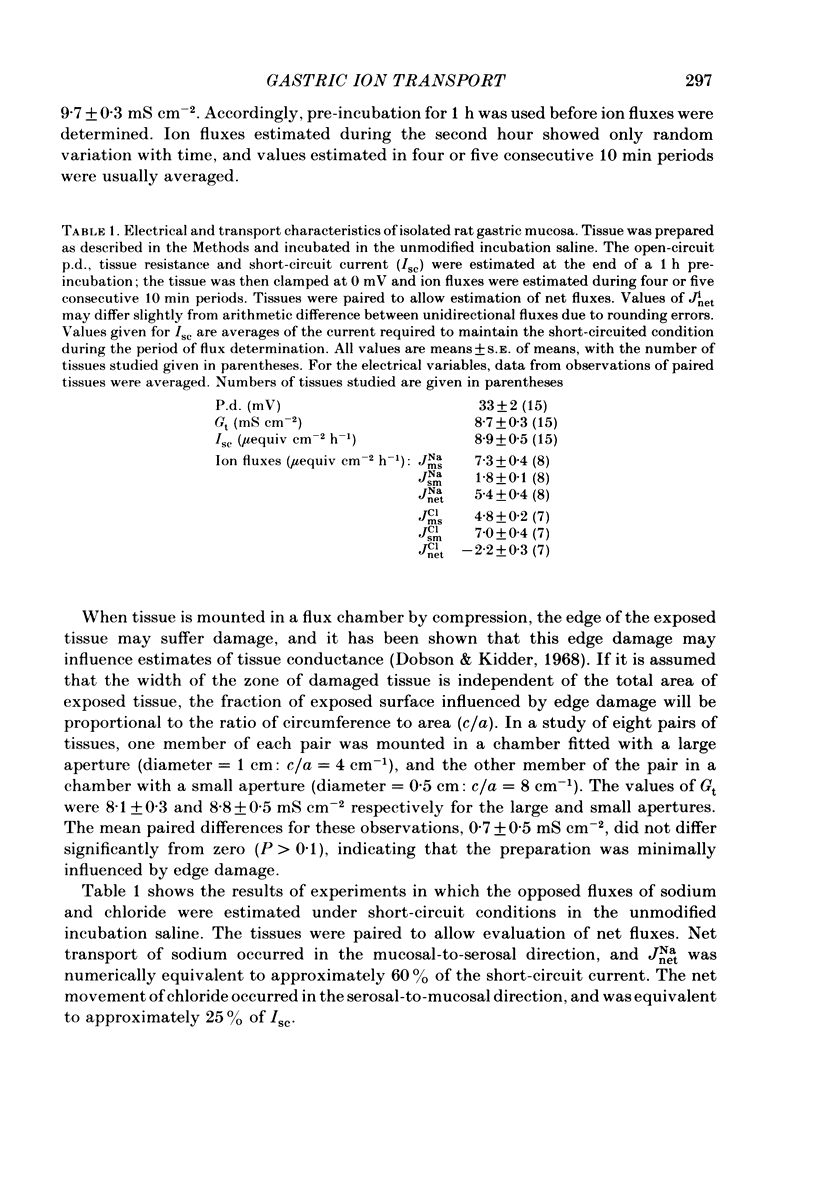
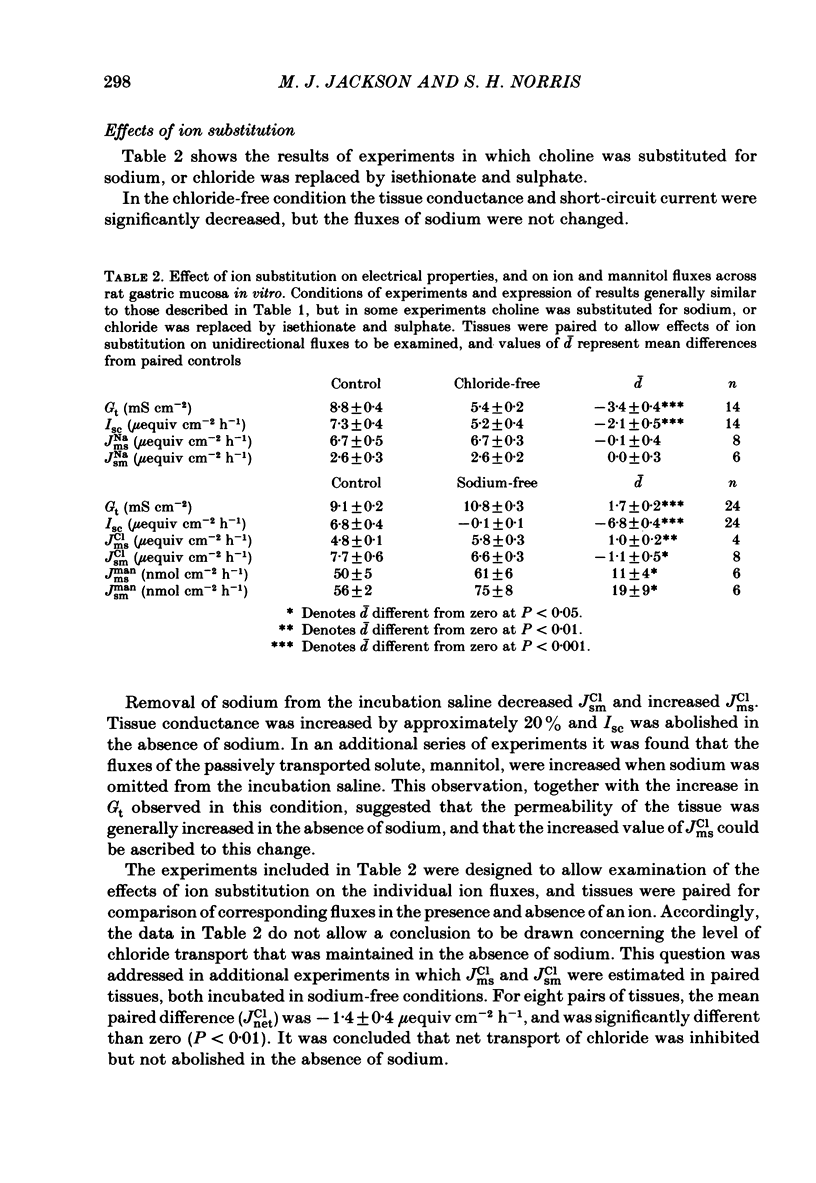
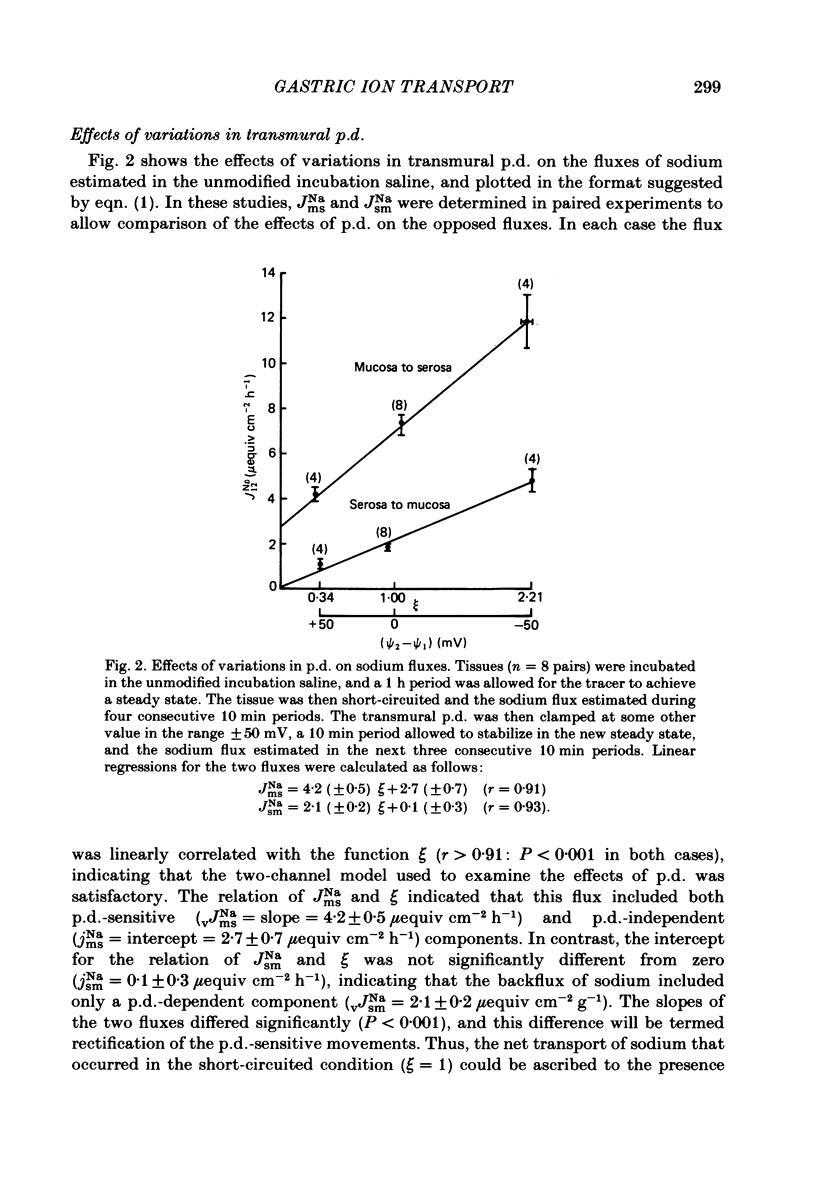
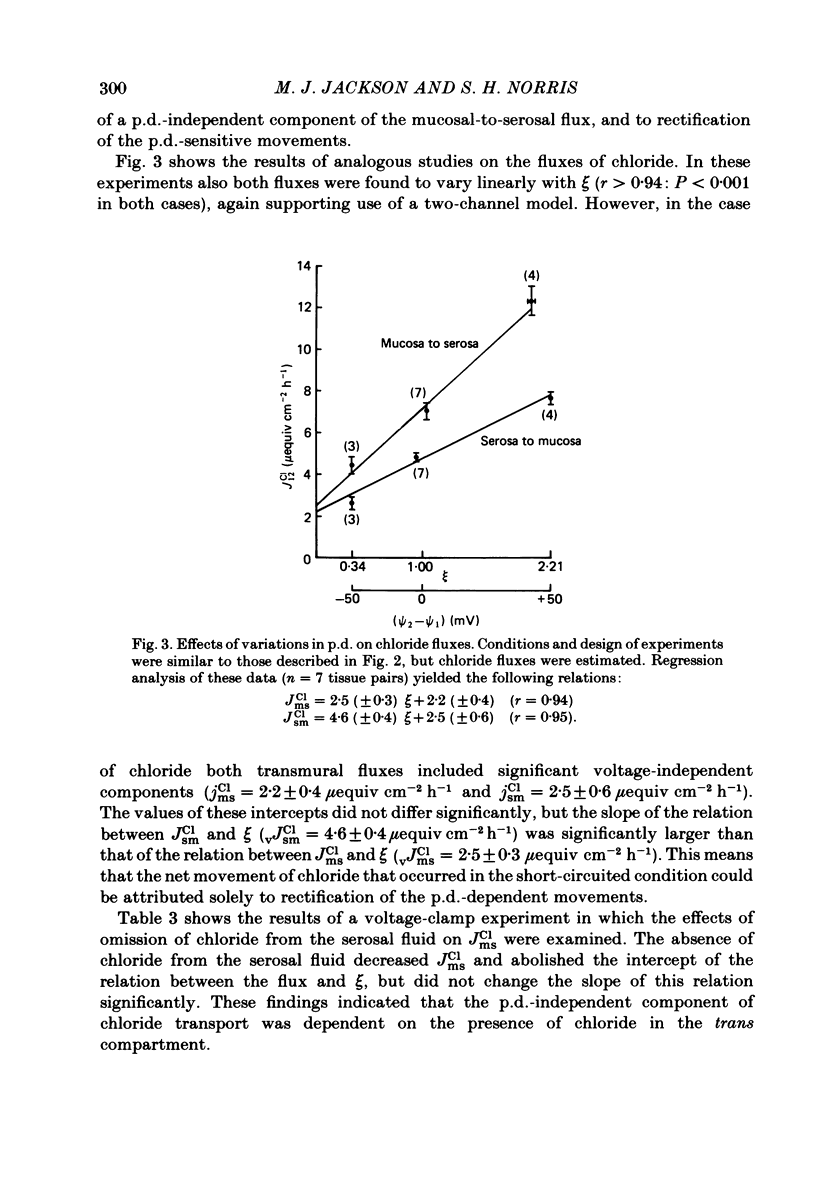
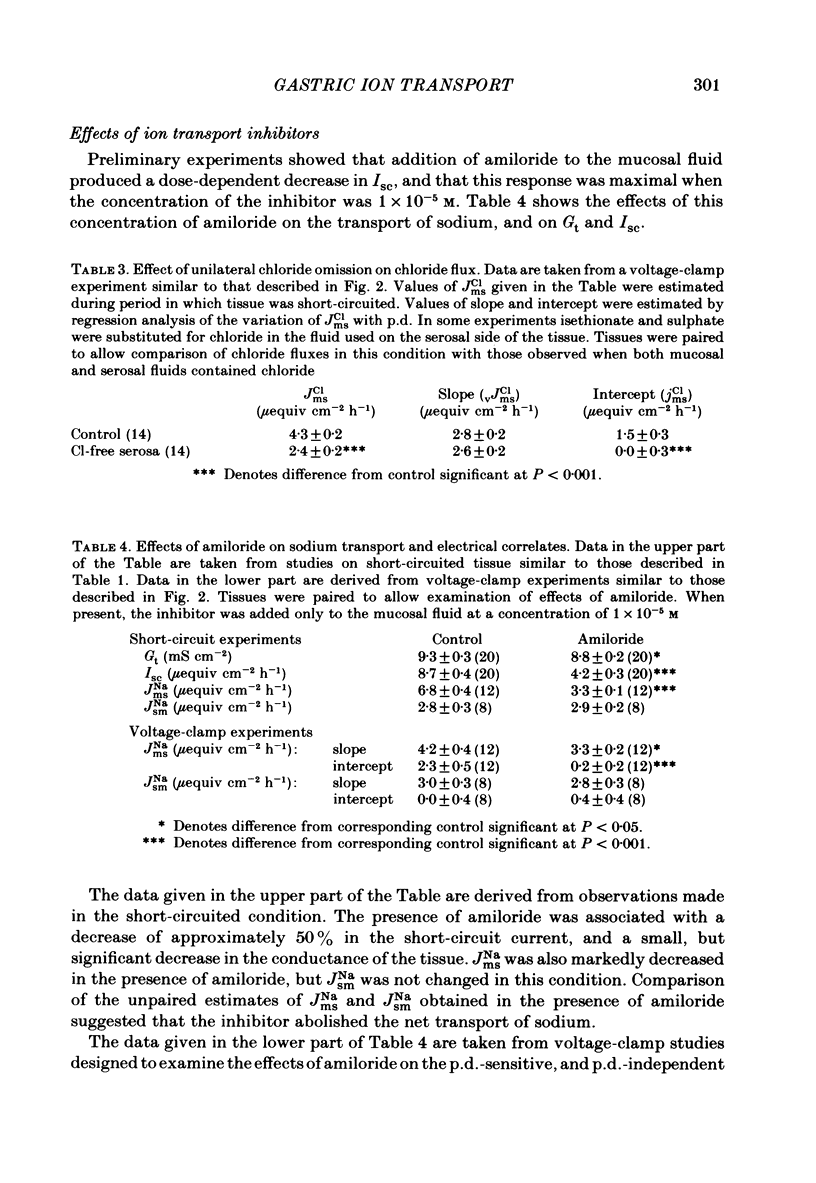
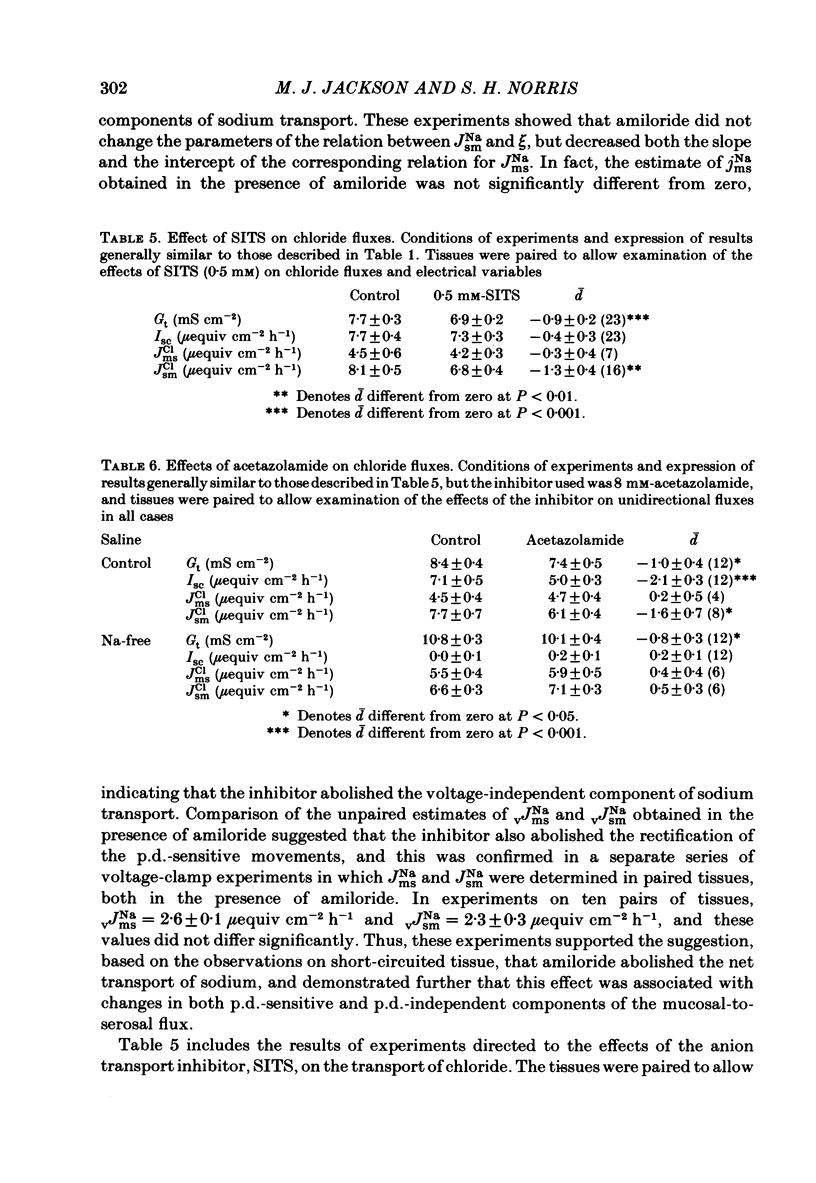
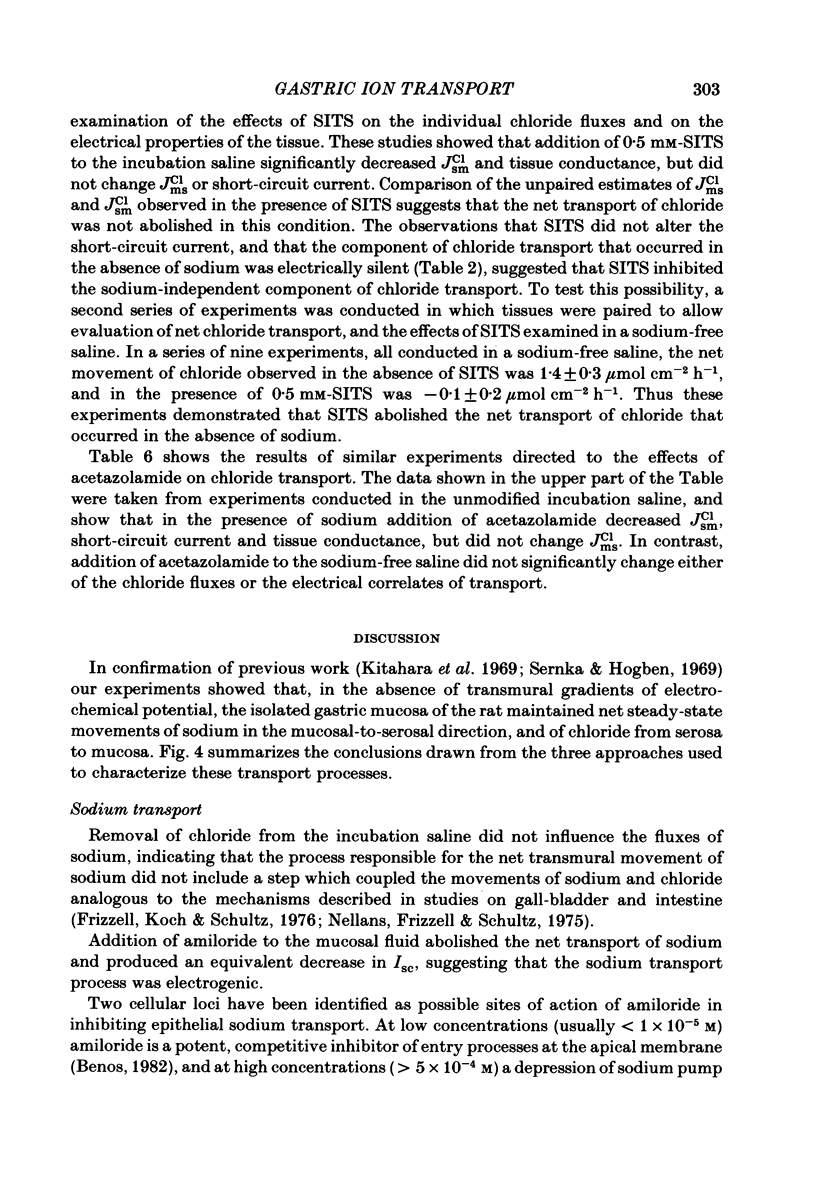
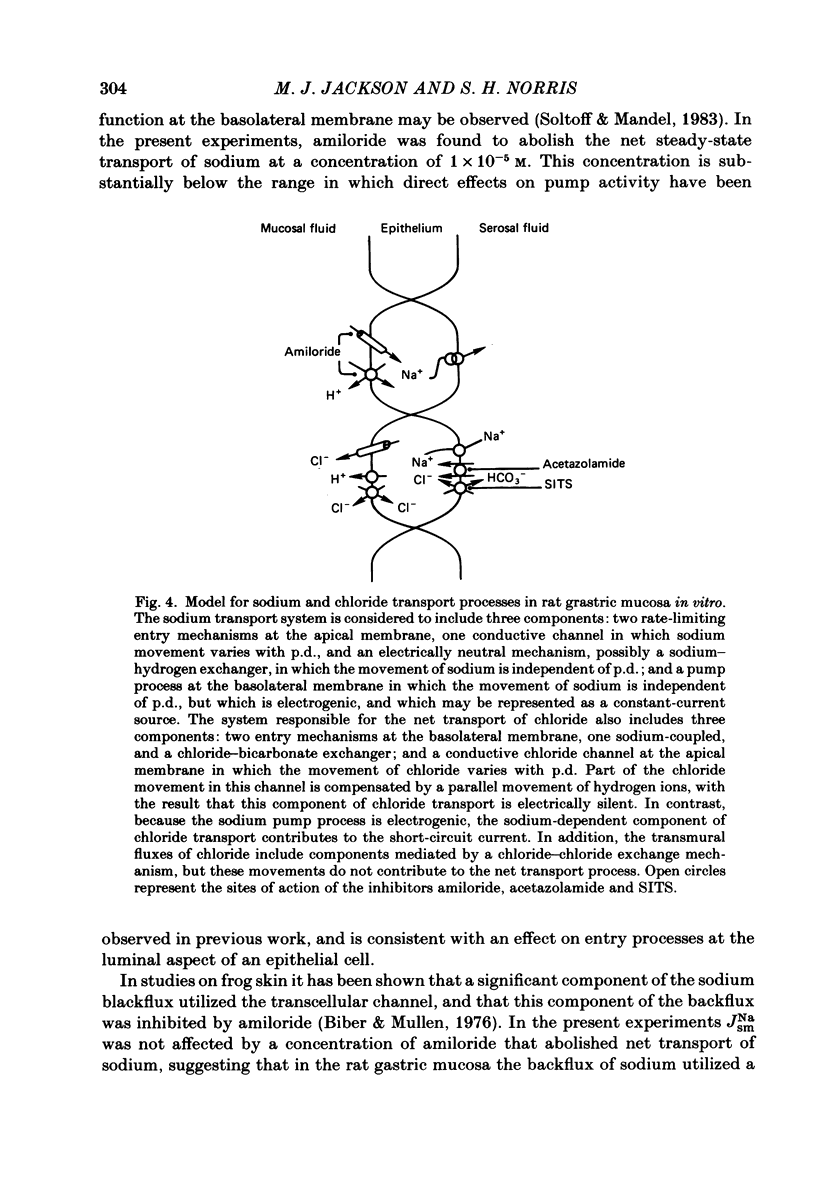
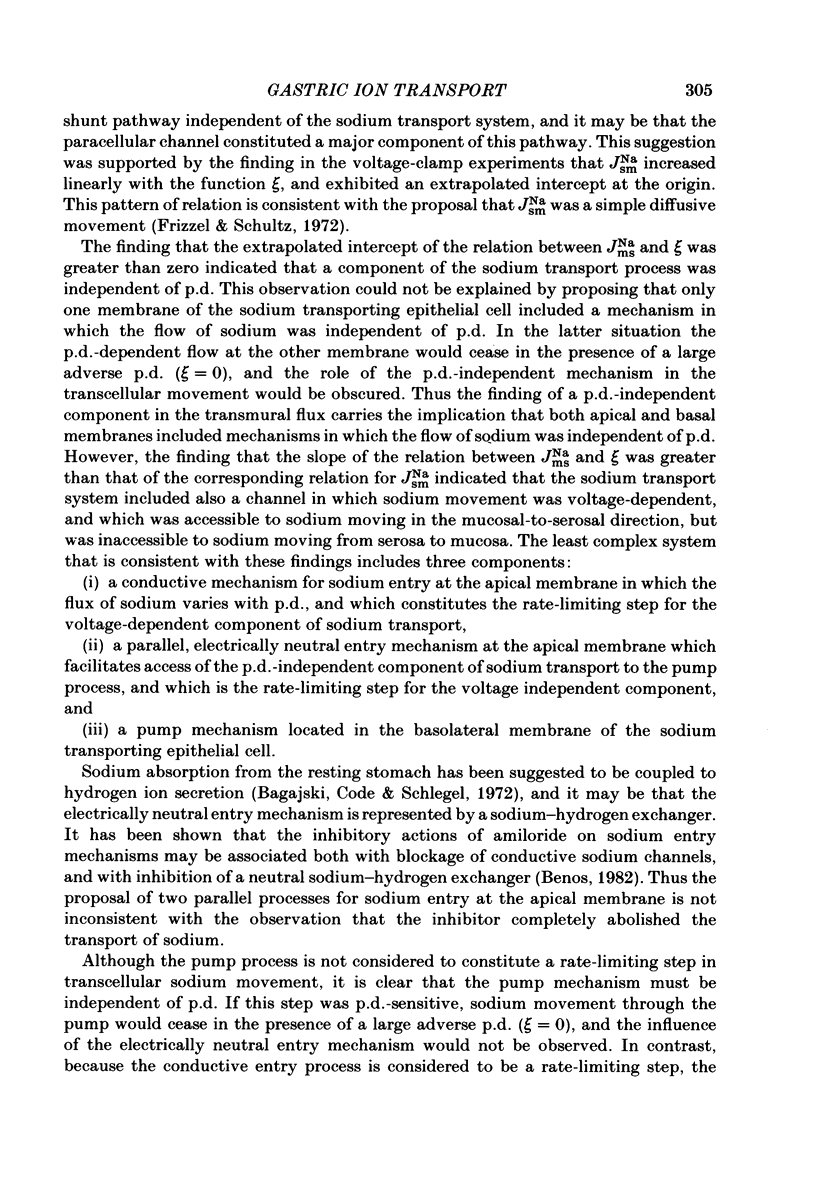
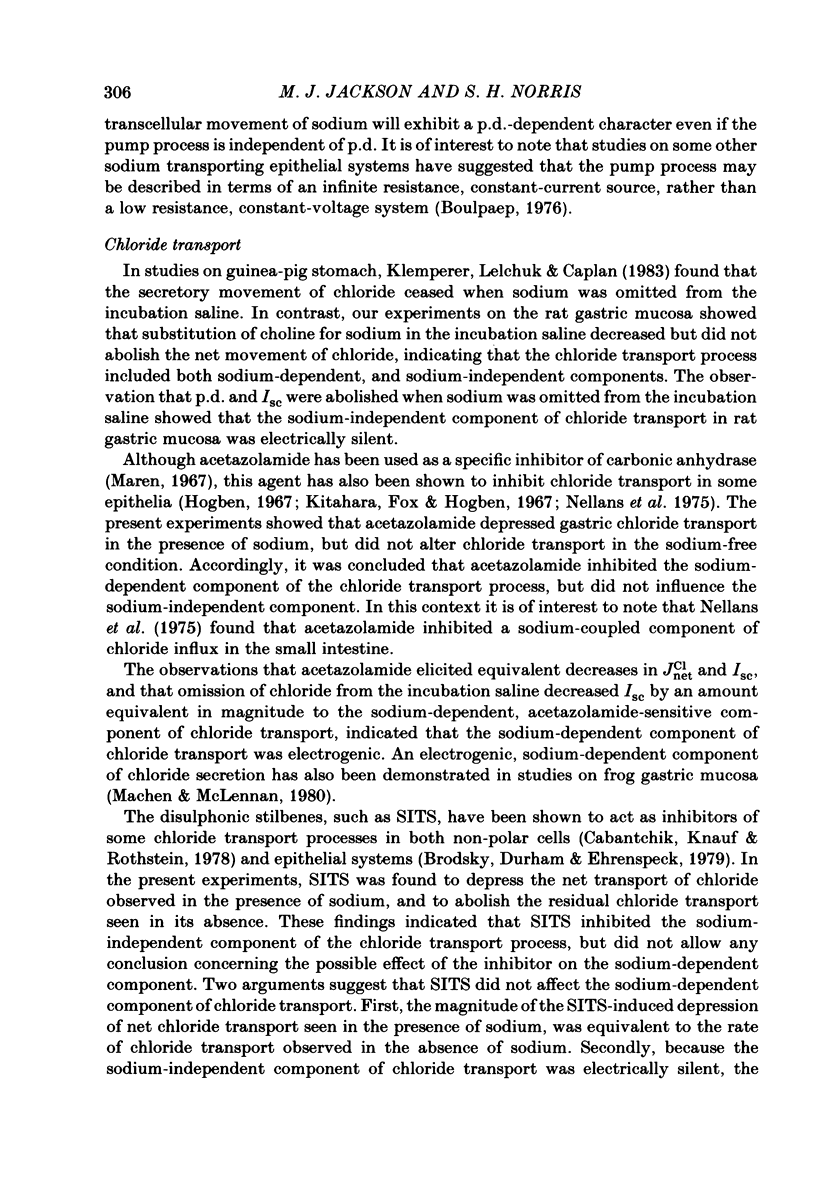
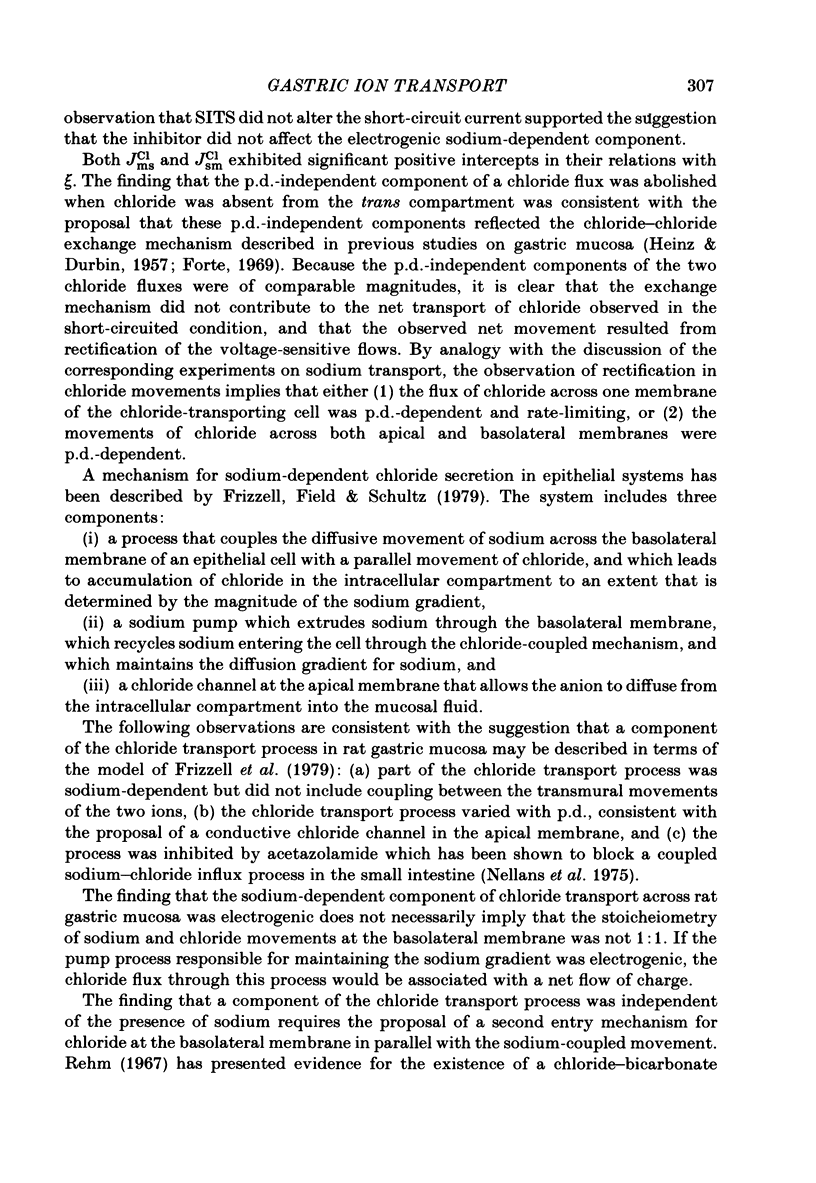
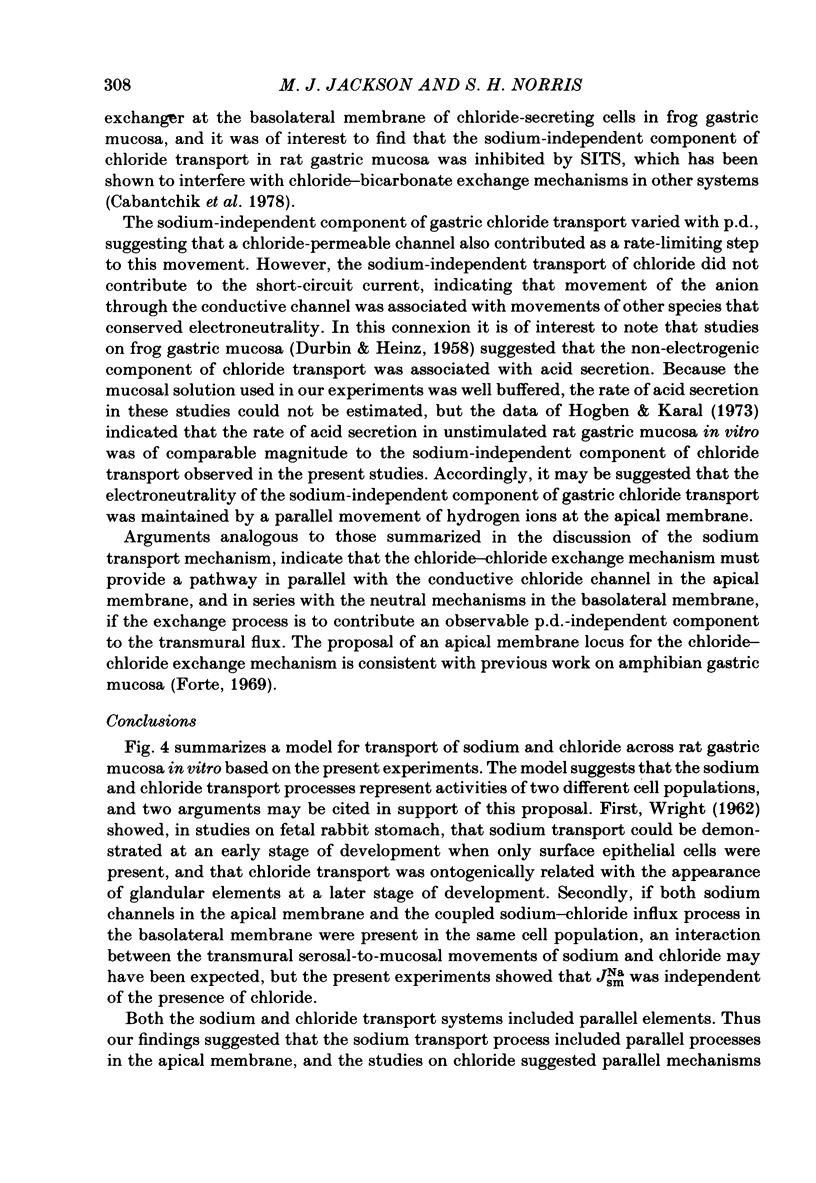
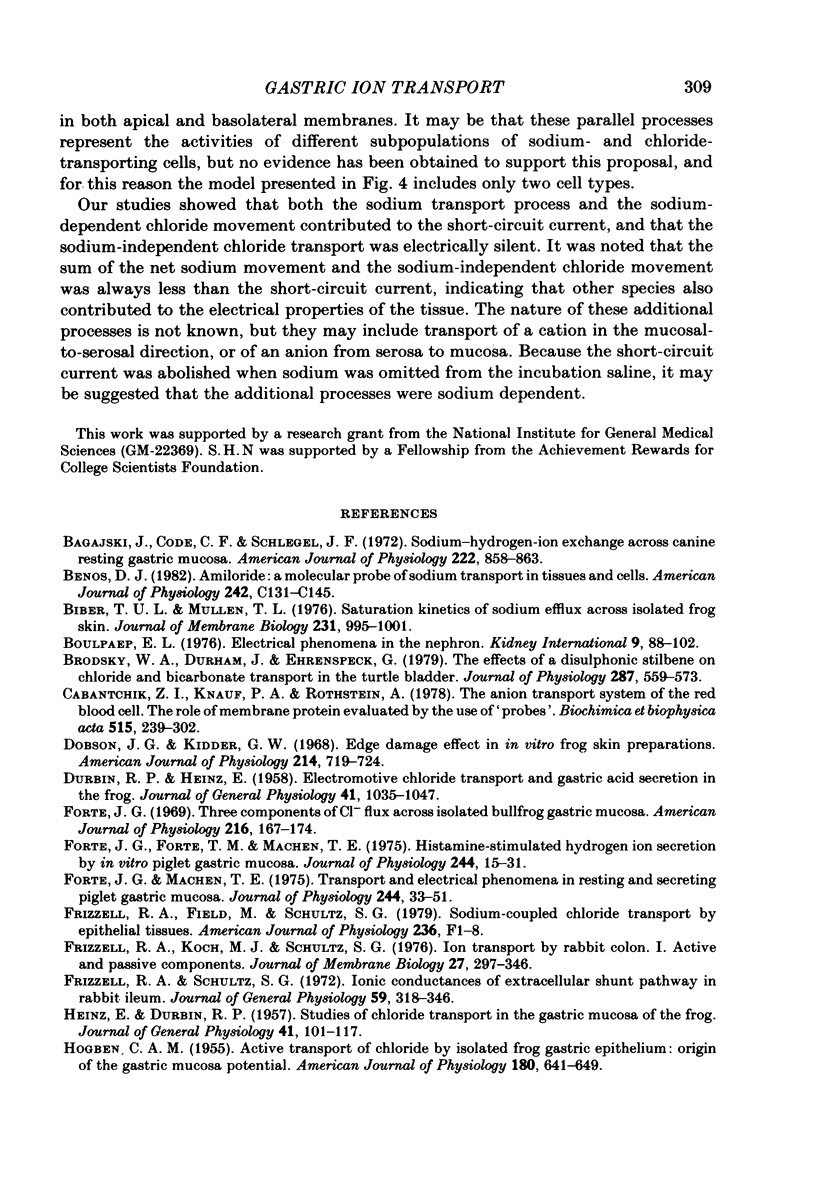
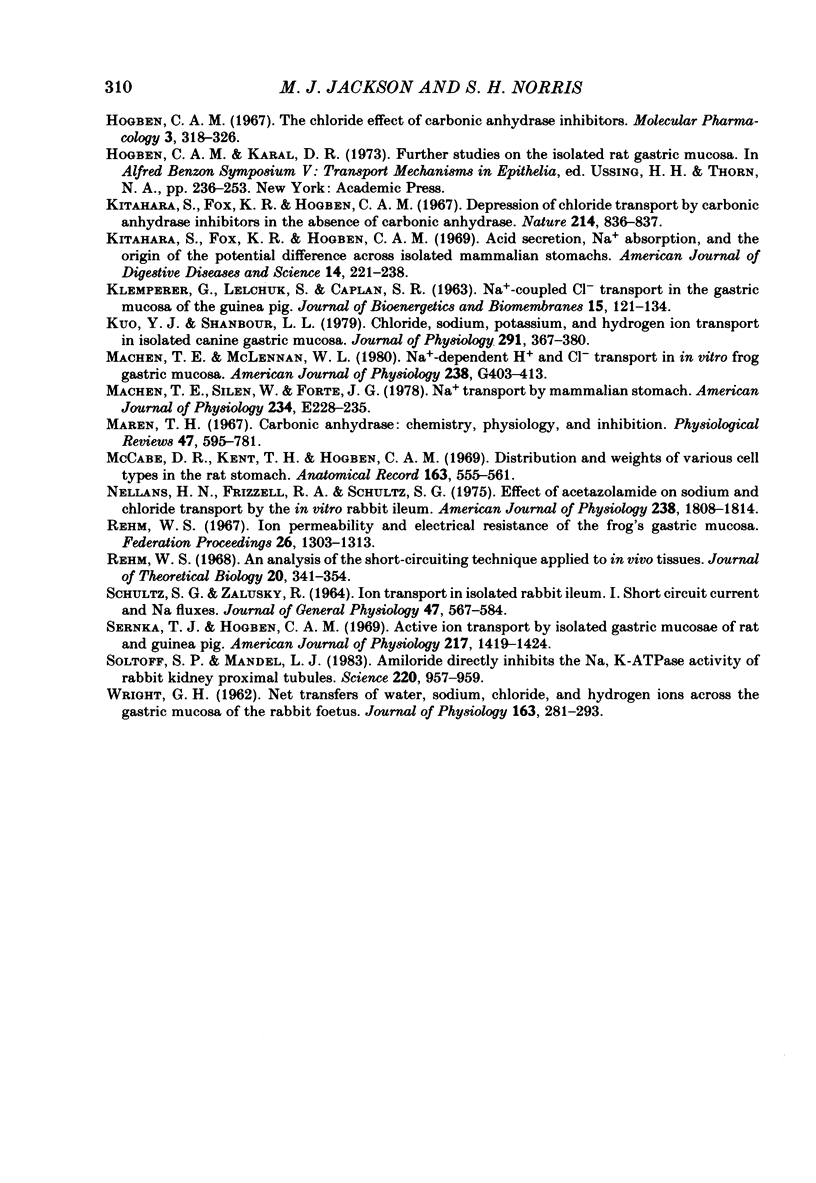
Selected References
These references are in PubMed. This may not be the complete list of references from this article.
- Adrian C., Hogben M. The chloride effect of carbonic anhydrase inhibitors. Mol Pharmacol. 1967 Jul;3(4):318–326. [PubMed] [Google Scholar]
- Benos D. J. Amiloride: a molecular probe of sodium transport in tissues and cells. Am J Physiol. 1982 Mar;242(3):C131–C145. doi: 10.1152/ajpcell.1982.242.3.C131. [DOI] [PubMed] [Google Scholar]
- Biber T. U., Mullen T. L. Saturation kinetics of sodium efflux across isolated frog skin. Am J Physiol. 1976 Oct;231(4):995–1001. doi: 10.1152/ajplegacy.1976.231.4.995. [DOI] [PubMed] [Google Scholar]
- Boulpaep E. L. Electrical phenomena in the nephron. Kidney Int. 1976 Feb;9(2):88–102. doi: 10.1038/ki.1976.14. [DOI] [PubMed] [Google Scholar]
- Brodsky W. A., Durham J., Ehrenspeck G. The effects of a disulphonic stilbene on chloride and bicarbonate transport in the turtle bladder. J Physiol. 1979 Feb;287:559–573. doi: 10.1113/jphysiol.1979.sp012677. [DOI] [PMC free article] [PubMed] [Google Scholar]
- Bugajski J., Code C. F., Schlegel J. F. Sodium-hydrogen ion exchange across canine resting gastric mucosa. Am J Physiol. 1972 Apr;222(4):858–863. doi: 10.1152/ajplegacy.1972.222.4.858. [DOI] [PubMed] [Google Scholar]
- Cabantchik Z. I., Knauf P. A., Rothstein A. The anion transport system of the red blood cell. The role of membrane protein evaluated by the use of 'probes'. Biochim Biophys Acta. 1978 Sep 29;515(3):239–302. doi: 10.1016/0304-4157(78)90016-3. [DOI] [PubMed] [Google Scholar]
- DURBIN R. P., HEINZ E. Electromotive chloride transport and gastric acid secretion in the frog. J Gen Physiol. 1958 May 20;41(5):1035–1047. doi: 10.1085/jgp.41.5.1035. [DOI] [PMC free article] [PubMed] [Google Scholar]
- Dobson J. G., Jr, Kidder G. W., 3rd Edge damage effect in in vitro frog skin preparations. Am J Physiol. 1968 Apr;214(4):719–724. doi: 10.1152/ajplegacy.1968.214.4.719. [DOI] [PubMed] [Google Scholar]
- Forte J. G., Forte T. M., Machen T. E. Histamine-stimulated hydrogen ion secretion by in vitro piglet gastric mucosa. J Physiol. 1975 Jan;244(1):15–31. doi: 10.1113/jphysiol.1975.sp010782. [DOI] [PMC free article] [PubMed] [Google Scholar]
- Forte J. G., Machen T. E. Transport and electrical phenomena in resting and secreting piglet gastric mucosa. J Physiol. 1975 Jan;244(1):33–51. doi: 10.1113/jphysiol.1975.sp010783. [DOI] [PMC free article] [PubMed] [Google Scholar]
- Forte J. G. Three components of Cl flux across isolated bullfrog gastric mucosa. Am J Physiol. 1969 Jan;216(1):167–174. doi: 10.1152/ajplegacy.1969.216.1.167. [DOI] [PubMed] [Google Scholar]
- Frizzell R. A., Field M., Schultz S. G. Sodium-coupled chloride transport by epithelial tissues. Am J Physiol. 1979 Jan;236(1):F1–F8. doi: 10.1152/ajprenal.1979.236.1.F1. [DOI] [PubMed] [Google Scholar]
- Frizzell R. A., Koch M. J., Schultz S. G. Ion transport by rabbit colon. I. Active and passive components. J Membr Biol. 1976;27(3):297–316. doi: 10.1007/BF01869142. [DOI] [PubMed] [Google Scholar]
- Frizzell R. A., Schultz S. G. Ionic conductances of extracellular shunt pathway in rabbit ileum. Influence of shunt on transmural sodium transport and electrical potential differences. J Gen Physiol. 1972 Mar;59(3):318–346. doi: 10.1085/jgp.59.3.318. [DOI] [PMC free article] [PubMed] [Google Scholar]
- HEINZ E., DURBIN R. P. Studies of the chloride transport in the gastric mucosa of the frog. J Gen Physiol. 1957 Sep 20;41(1):101–117. doi: 10.1085/jgp.41.1.101. [DOI] [PMC free article] [PubMed] [Google Scholar]
- HOGBEN C. A. Active transport of chloride by isolated frog gastric epithelium; origin of the gastric mucosal potential. Am J Physiol. 1955 Mar;180(3):641–649. [PubMed] [Google Scholar]
- Kitahara S., Fox K. R., Hogben C. A. Acid secretion, Na+ absorption, and the origin of the potential difference across isolated mammalian stomachs. Am J Dig Dis. 1969 Apr;14(4):221–238. doi: 10.1007/BF02235951. [DOI] [PubMed] [Google Scholar]
- Kitahara S., Fox K. R., Hogben C. A. Depression of chloride transport by carbonic anhydrase inhibitors in the absence of carbonic anhydrase. Nature. 1967 May 20;214(5090):836–837. doi: 10.1038/214836a0. [DOI] [PubMed] [Google Scholar]
- Kuo Y. J., Shanbour L. L. Chloride, sodium, potassium and hydrogen ion transport in isolated canine gastric mucosa. J Physiol. 1979 Jun;291:367–380. doi: 10.1113/jphysiol.1979.sp012819. [DOI] [PMC free article] [PubMed] [Google Scholar]
- Machen T. E., McLennan W. L. Na+-dependent H+ and Cl- transport in in vitro frog gastric mucosa. Am J Physiol. 1980 May;238(5):G403–G413. doi: 10.1152/ajpgi.1980.238.5.G403. [DOI] [PubMed] [Google Scholar]
- Machen T. E., Silen W., Forte J. G. Na+ transport by mammalian stomach. Am J Physiol. 1978 Mar;234(3):E228–E235. doi: 10.1152/ajpendo.1978.234.3.E228. [DOI] [PubMed] [Google Scholar]
- Maren T. H. Carbonic anhydrase: chemistry, physiology, and inhibition. Physiol Rev. 1967 Oct;47(4):595–781. doi: 10.1152/physrev.1967.47.4.595. [DOI] [PubMed] [Google Scholar]
- McCabe D. R., Kent T. H., Hogben C. A. Distribution and weights of various cell types in the rat stomach. Anat Rec. 1969 Apr;163(4):555–561. doi: 10.1002/ar.1091630407. [DOI] [PubMed] [Google Scholar]
- Nellans H. N., Frizzell R. A., Schultz S. G. Effect of acetazolamide on sodium and chloride transport by in vitro rabbit ileum. Am J Physiol. 1975 Jun;228(6):1808–1814. doi: 10.1152/ajplegacy.1975.228.6.1808. [DOI] [PubMed] [Google Scholar]
- Rehm W. S. An analysis of the short-circuiting technique applied to in vivo tissues. J Theor Biol. 1968 Sep;20(3):341–354. doi: 10.1016/0022-5193(68)90134-3. [DOI] [PubMed] [Google Scholar]
- Rehm W. S. Ion permeability and electrical resistance of the frog's gastric mucosa. Fed Proc. 1967 Sep;26(5):1303–1313. [PubMed] [Google Scholar]
- Sernka T. J., Hogben C. A. Active ion transport by isolated gastric mucosae of rat and guinea pig. Am J Physiol. 1969 Nov;217(5):1419–1424. doi: 10.1152/ajplegacy.1969.217.5.1419. [DOI] [PubMed] [Google Scholar]
- Soltoff S. P., Mandel L. J. Amiloride directly inhibits the Na,K-ATPase activity of rabbit kidney proximal tubules. Science. 1983 May 27;220(4600):957–958. doi: 10.1126/science.6302840. [DOI] [PubMed] [Google Scholar]
- WRIGHT G. H. Net transfers of water, sodium, chloride and hydrogen ions across the gastric mucosa of the rabbit foetus. J Physiol. 1962 Sep;163:281–293. doi: 10.1113/jphysiol.1962.sp006974. [DOI] [PMC free article] [PubMed] [Google Scholar]