Abstract
Equations are derived for potassium (K+) dynamics in simplified models of brain tissue. These describe K+ movement in extracellular space, transfer of K+ associated with current flow through cells (the so-called spatial buffer mechanism) and equilibration between extracellular space and cytoplasm. Numerical calculations show that the principal data on K+ dynamics from various laboratories can be accounted for with simple assumptions about spatial buffer action and uptake. Much of the data is inconsistent with extracellular diffusion being the main mechanism for K+ flux through brain tissue, including some that has earlier been cited in support of this hypothesis. The buffering actions of spatial buffer transfer of K+ and of cytoplasmic equilibration, in which these mechanisms reduce rises of [K+]o that would otherwise occur, are analysed quantitatively for specific K+ source distributions and for spatial and temporal frequency components of general disturbances. Spatial buffer action has most effect in reducing [K+]o rises with net release over extensive zones of tissue (greater than ca. 200 micron in diameter) for periods of the order of minutes. Reductions greater than 75% may be achieved. With localized but prolonged release, the maximum [K+]o rise is little affected but the volume of tissue affected by more moderate rises is substantially reduced. Cytoplasmic K+ uptake also has most effect with widespread release, but its effect diminishes with prolonged periods of release. The effects of the buffering mechanisms and of K+ re-uptake into active neurones in determining the decline of [K+]o after a period of stimulation are considered. Re-uptake is unlikely to be the major factor responsible for [K+]o decline when this has a time course of only a few seconds. The properties necessary for the cells mediating the spatial buffer mechanisms, possibly glial cells, are assessed.
Full text
PDF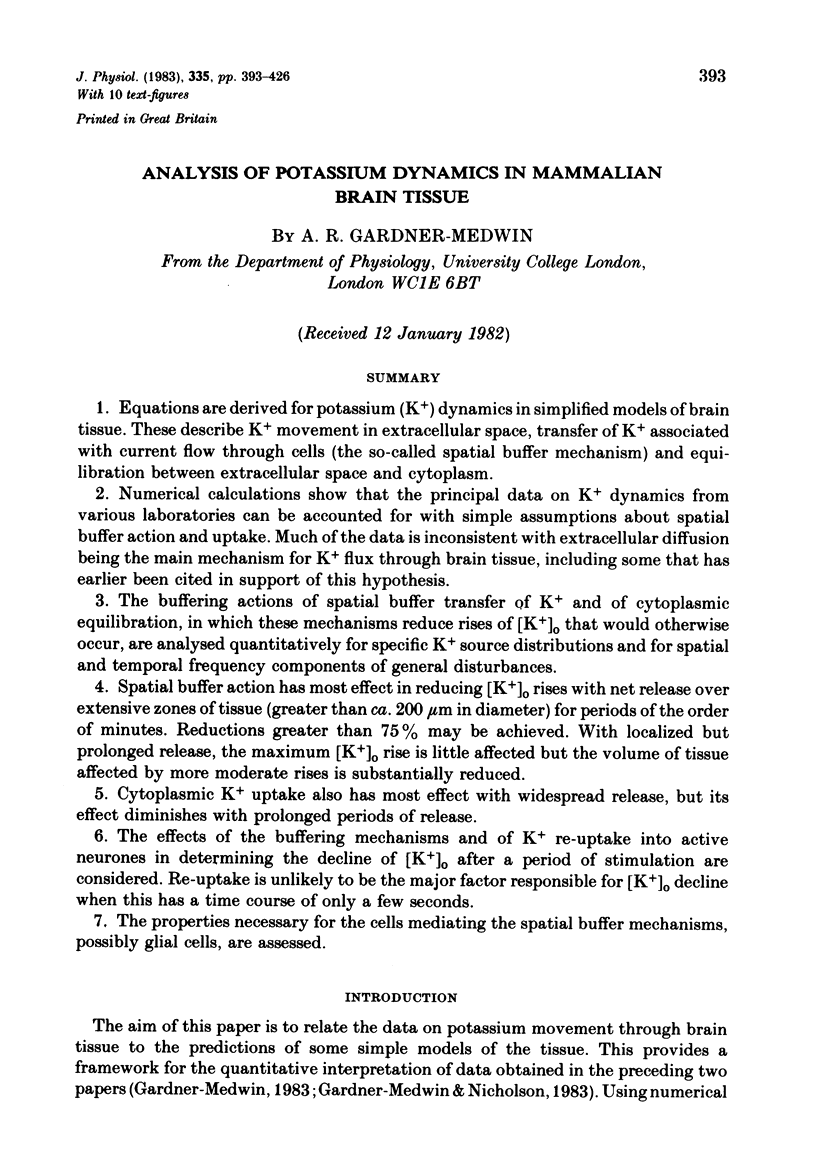
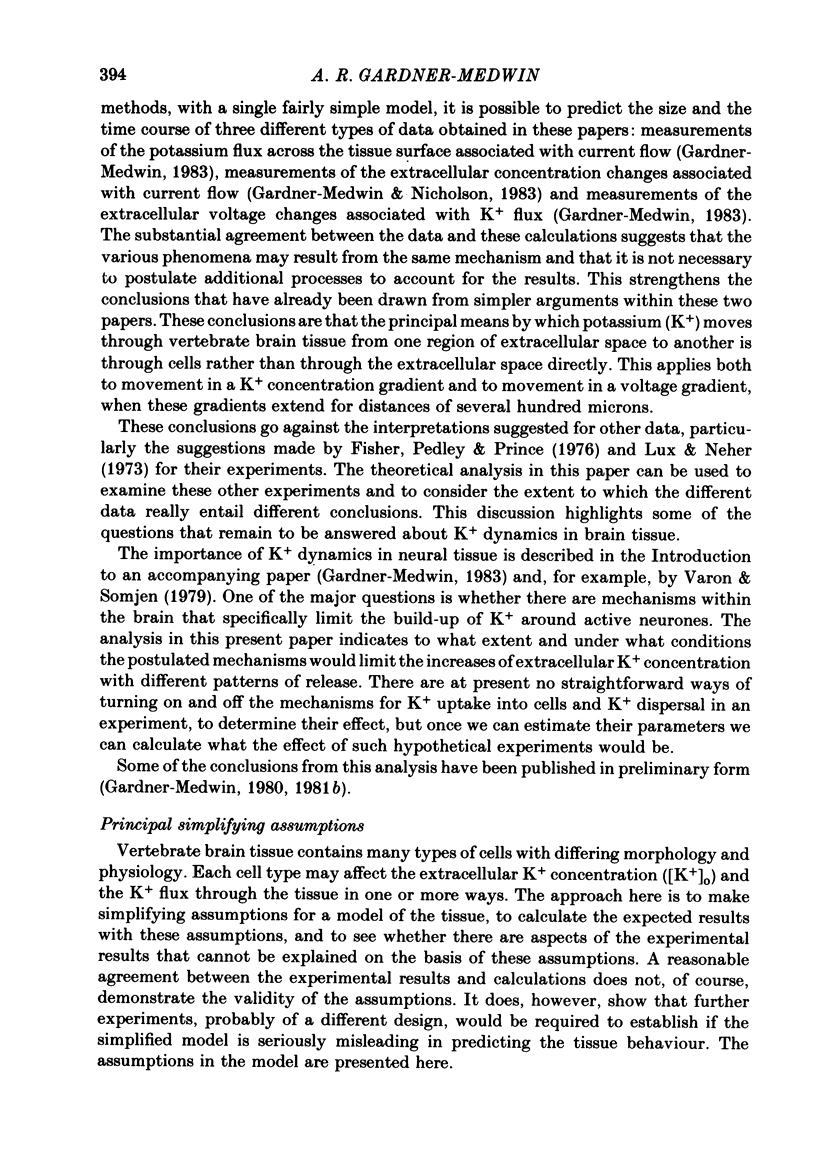
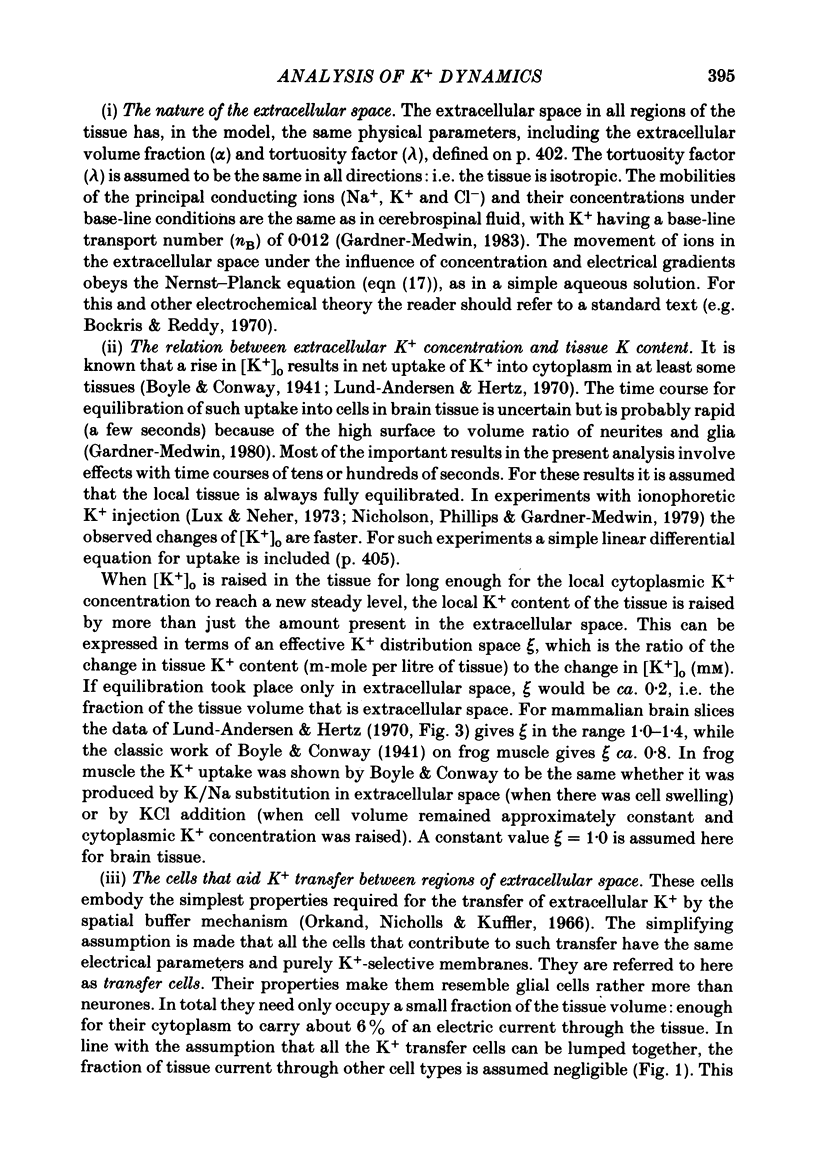
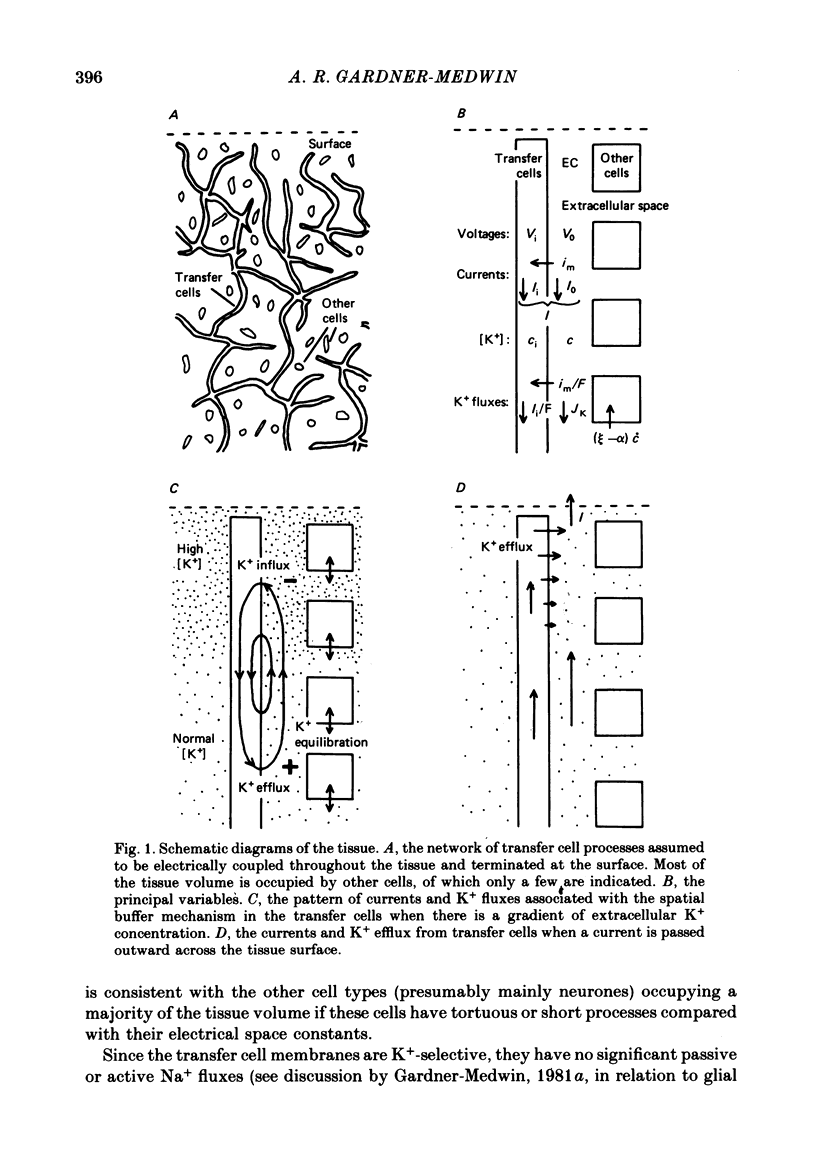
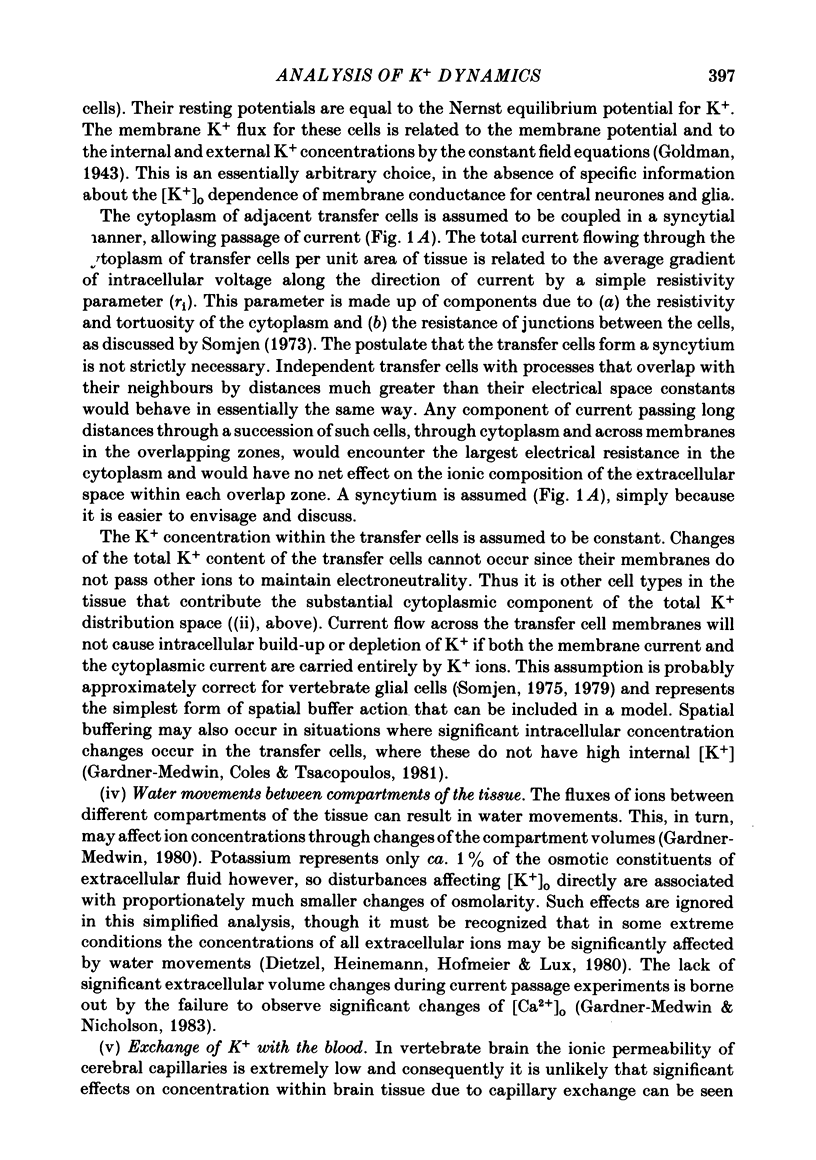
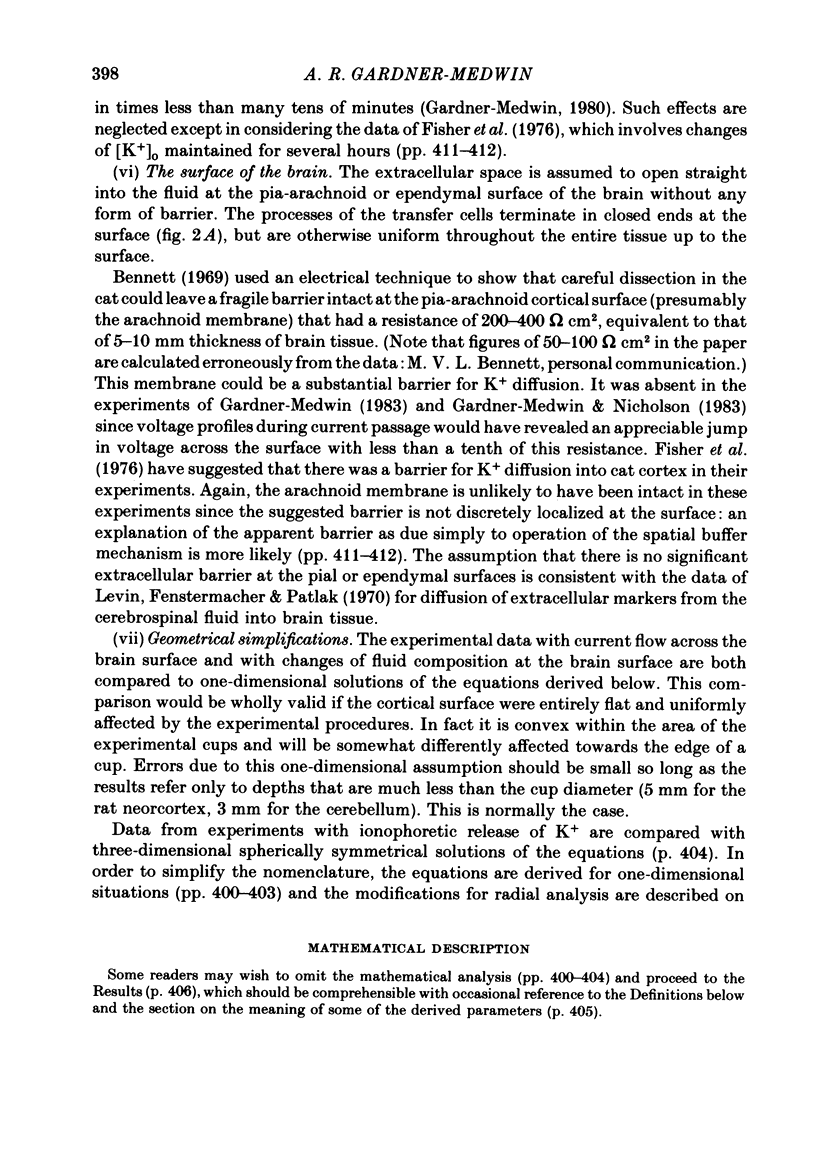
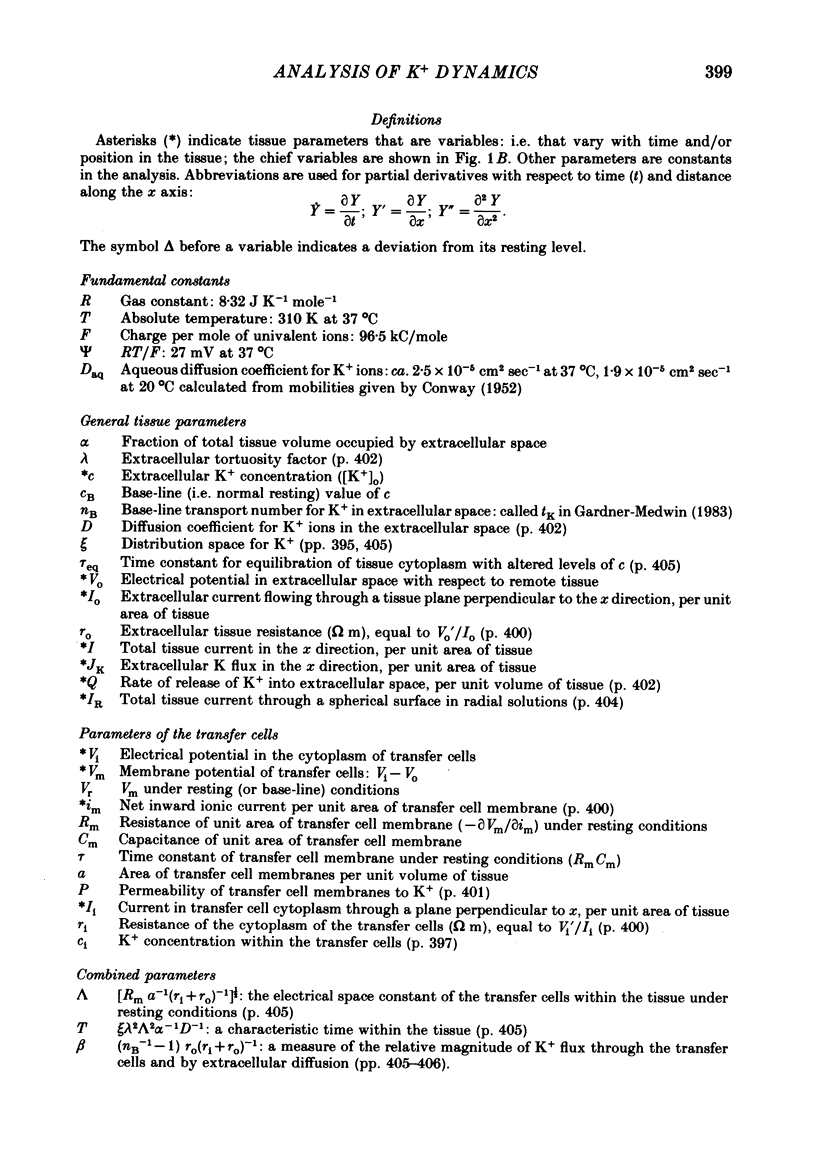
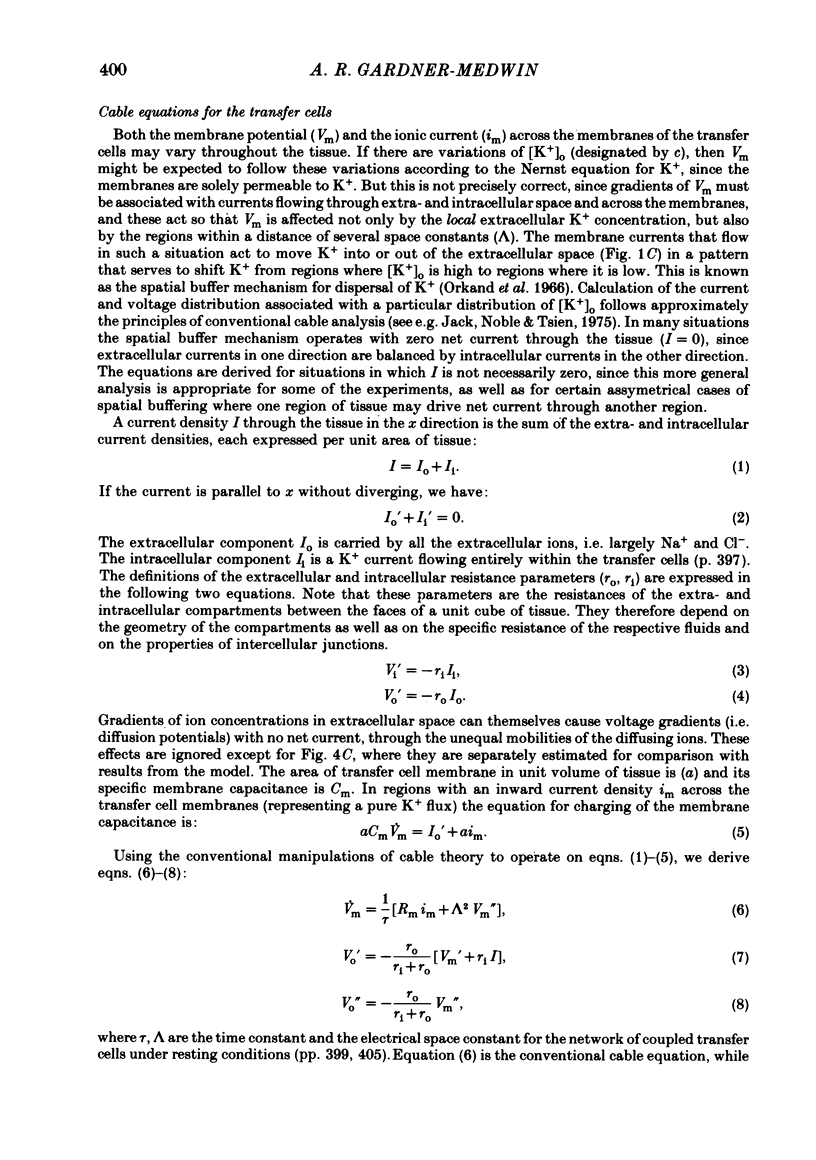
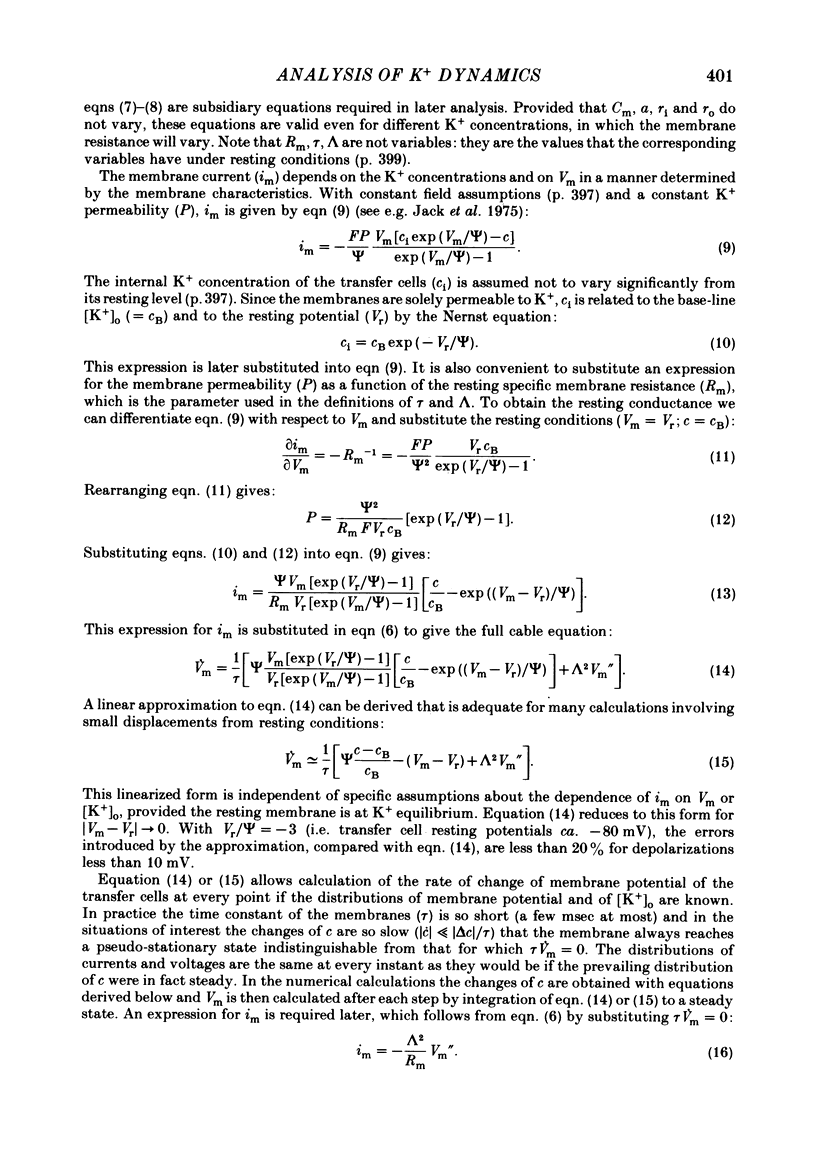
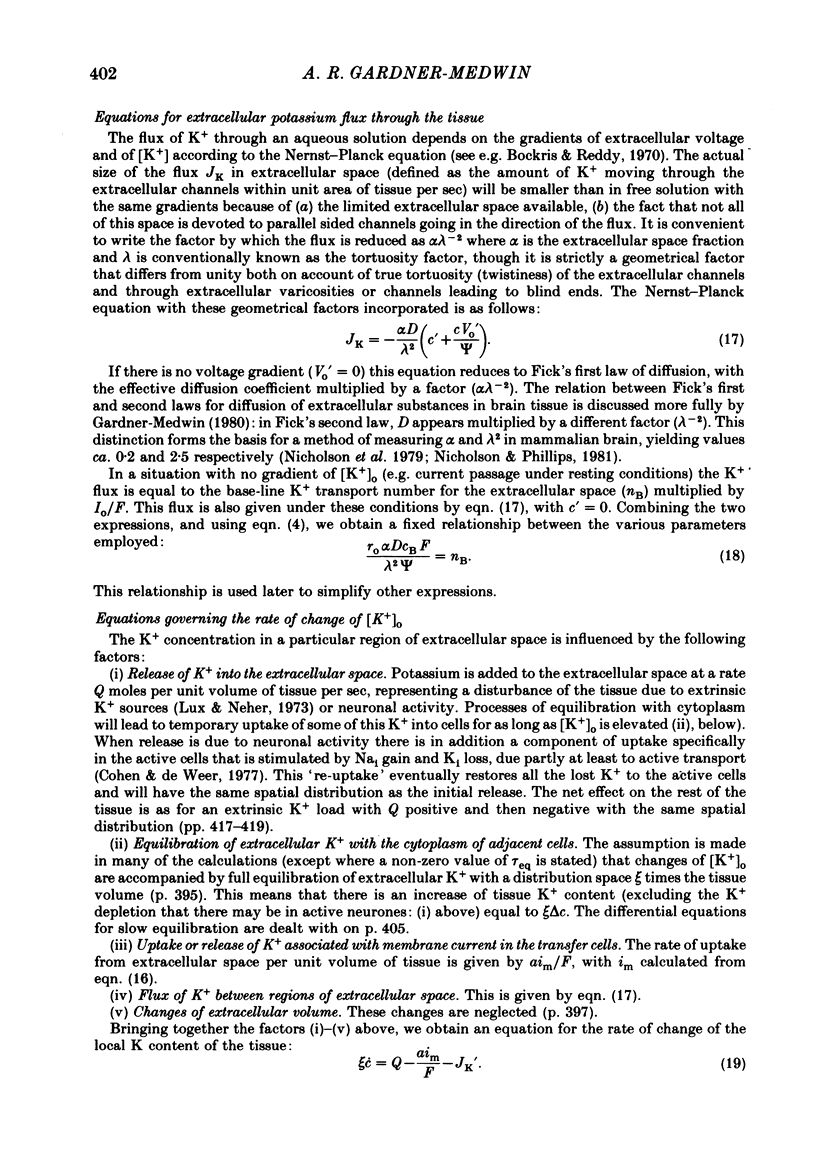
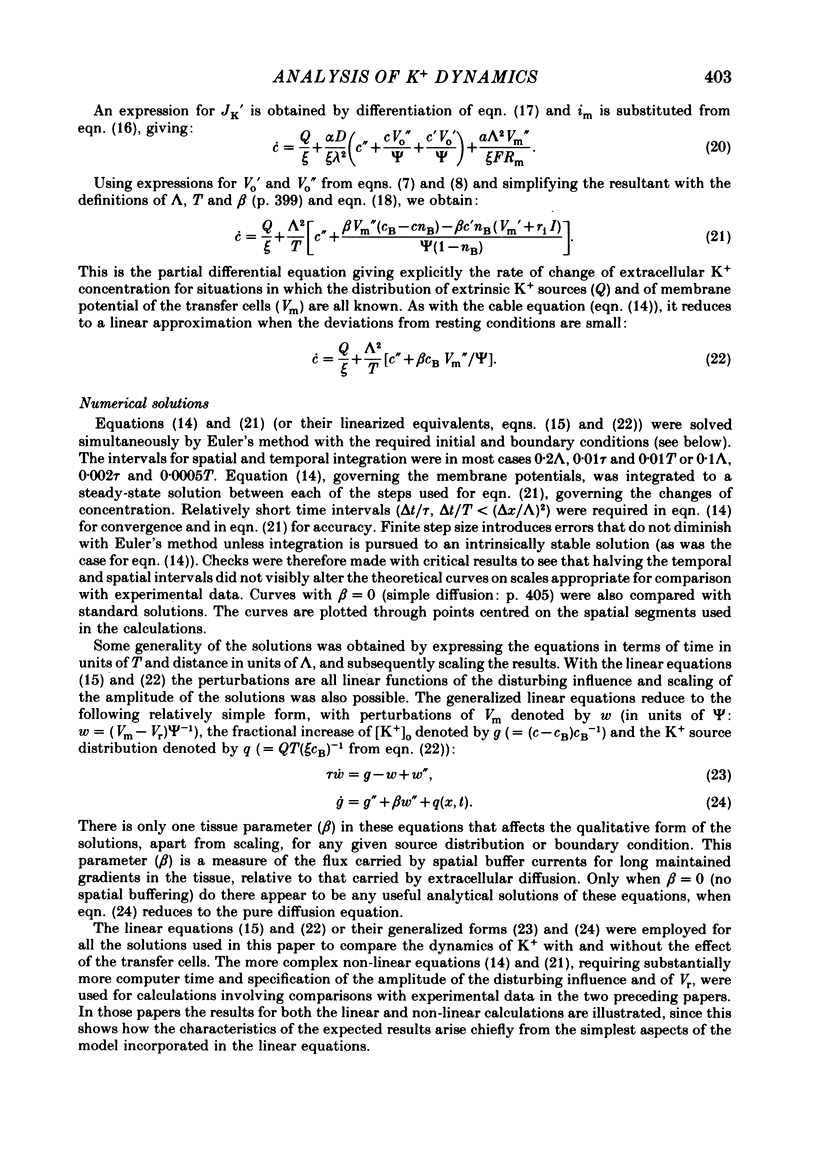
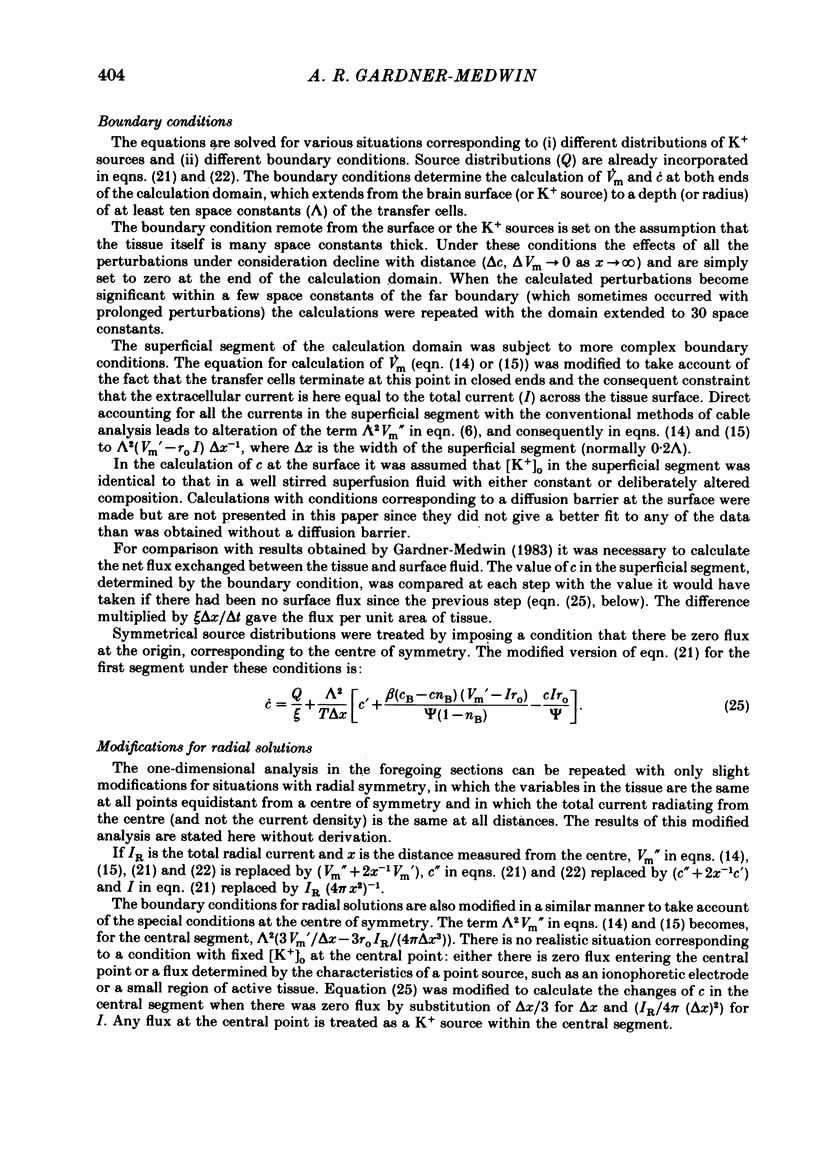
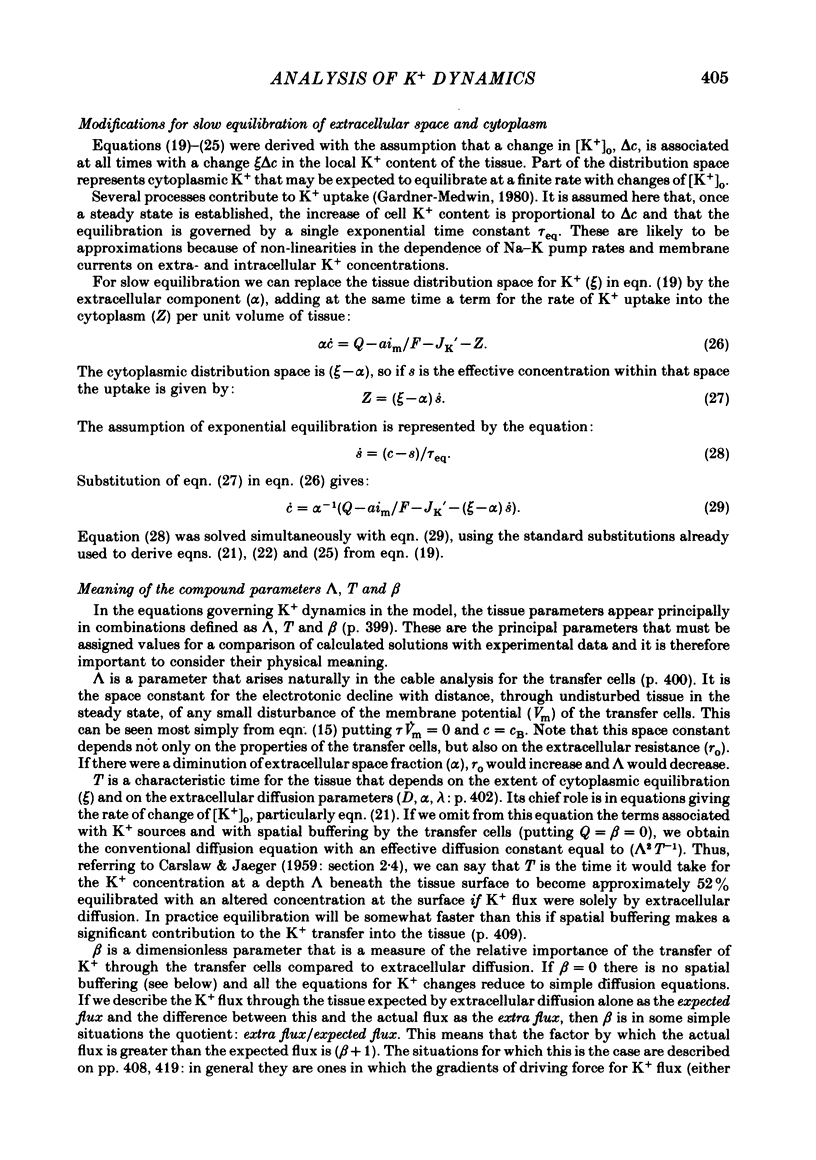
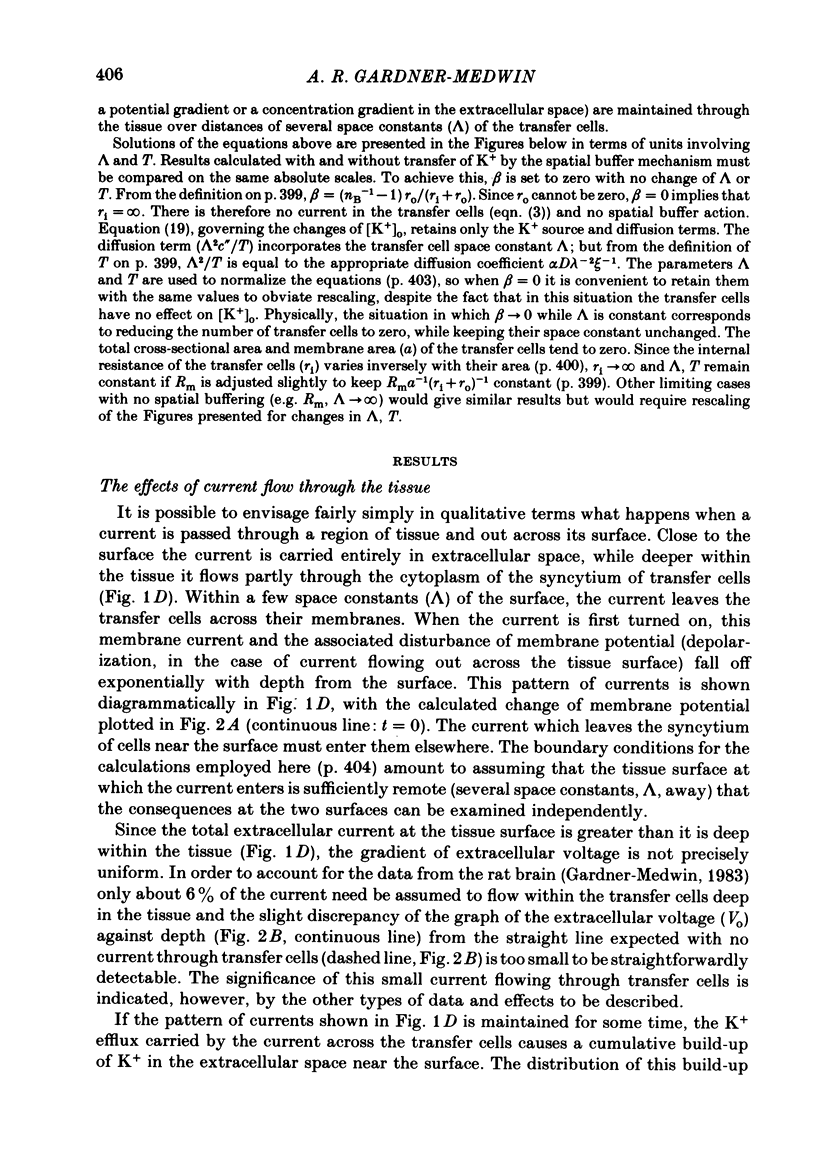
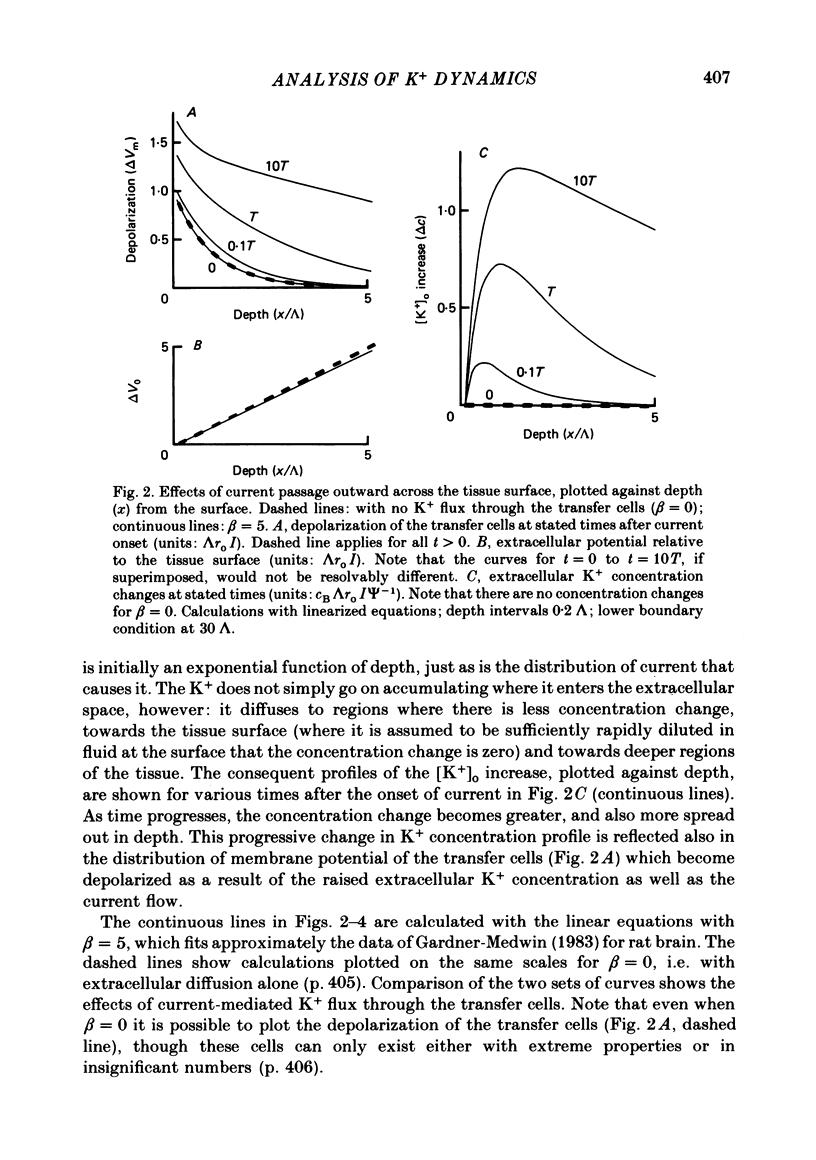
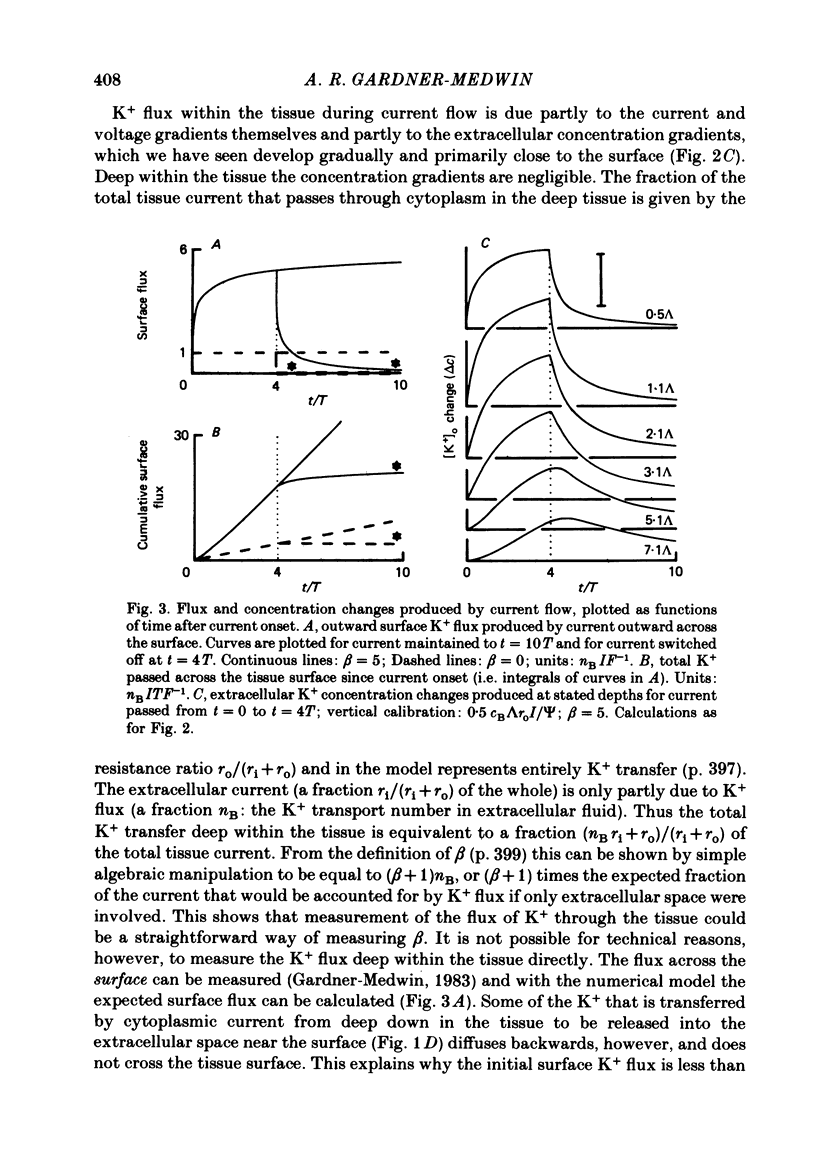
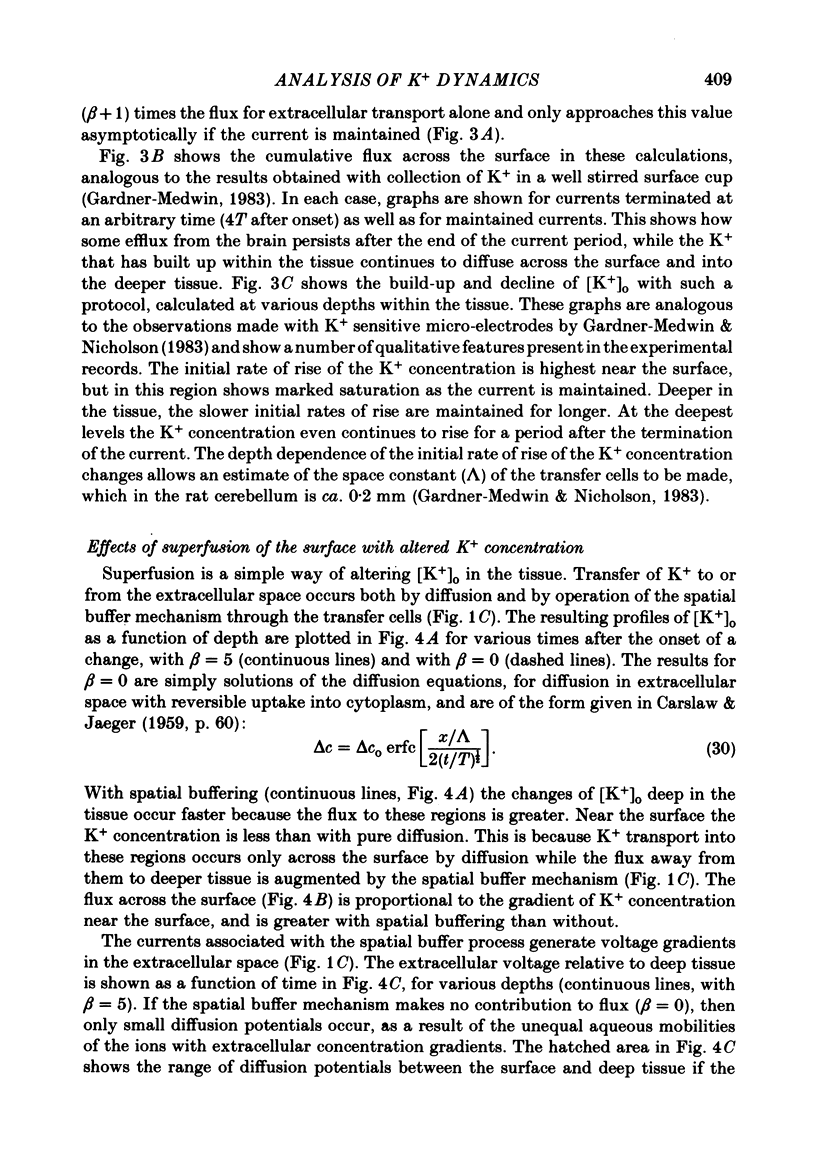
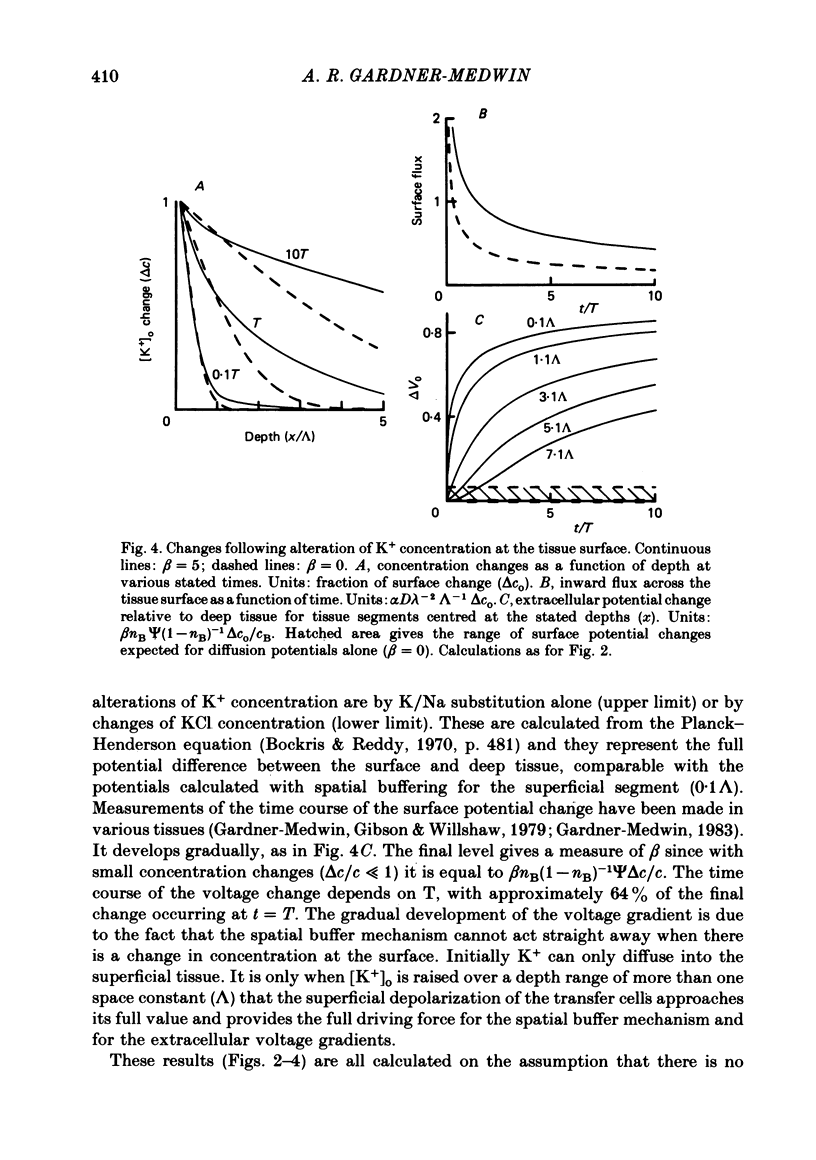
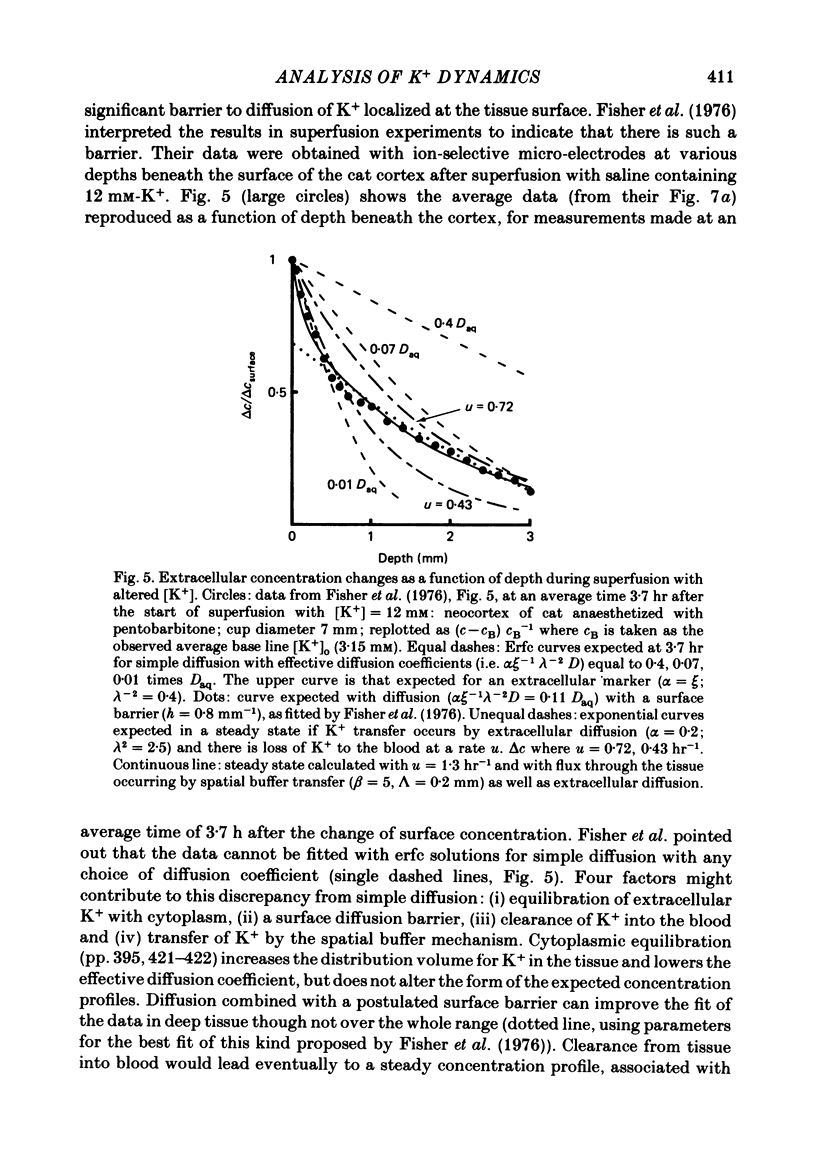
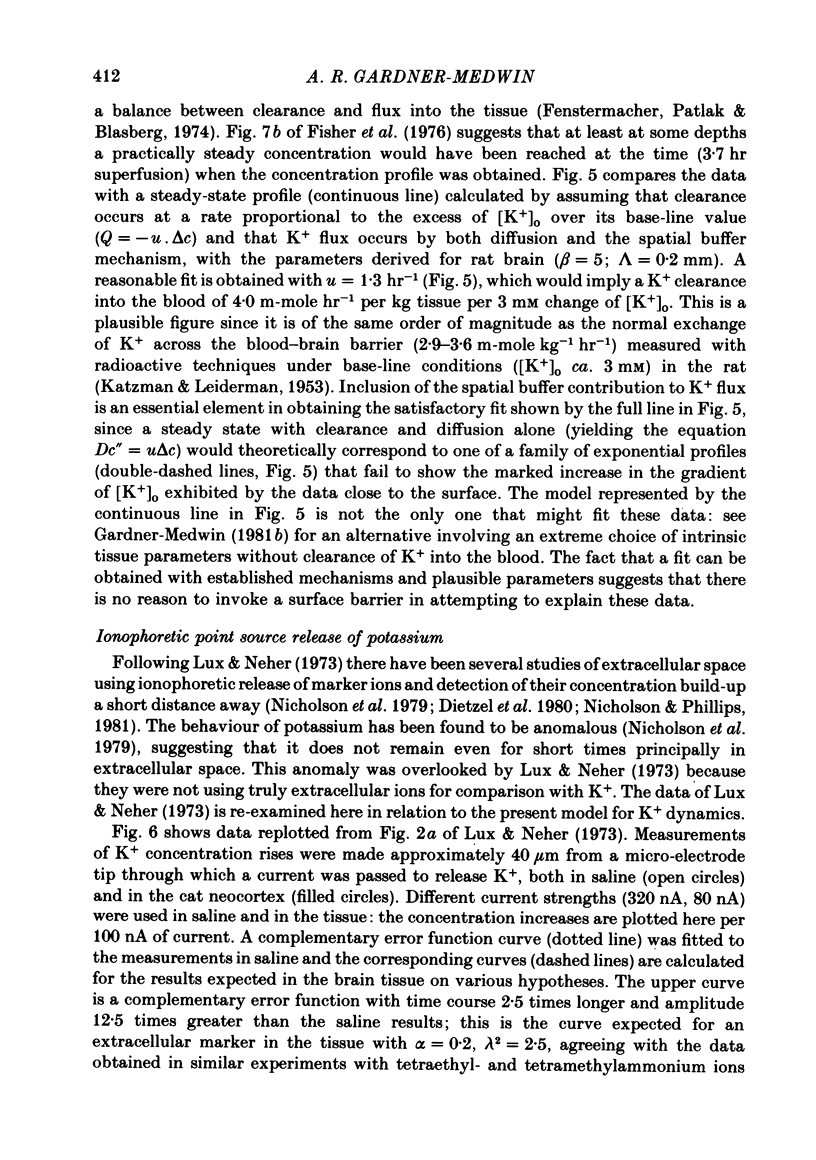
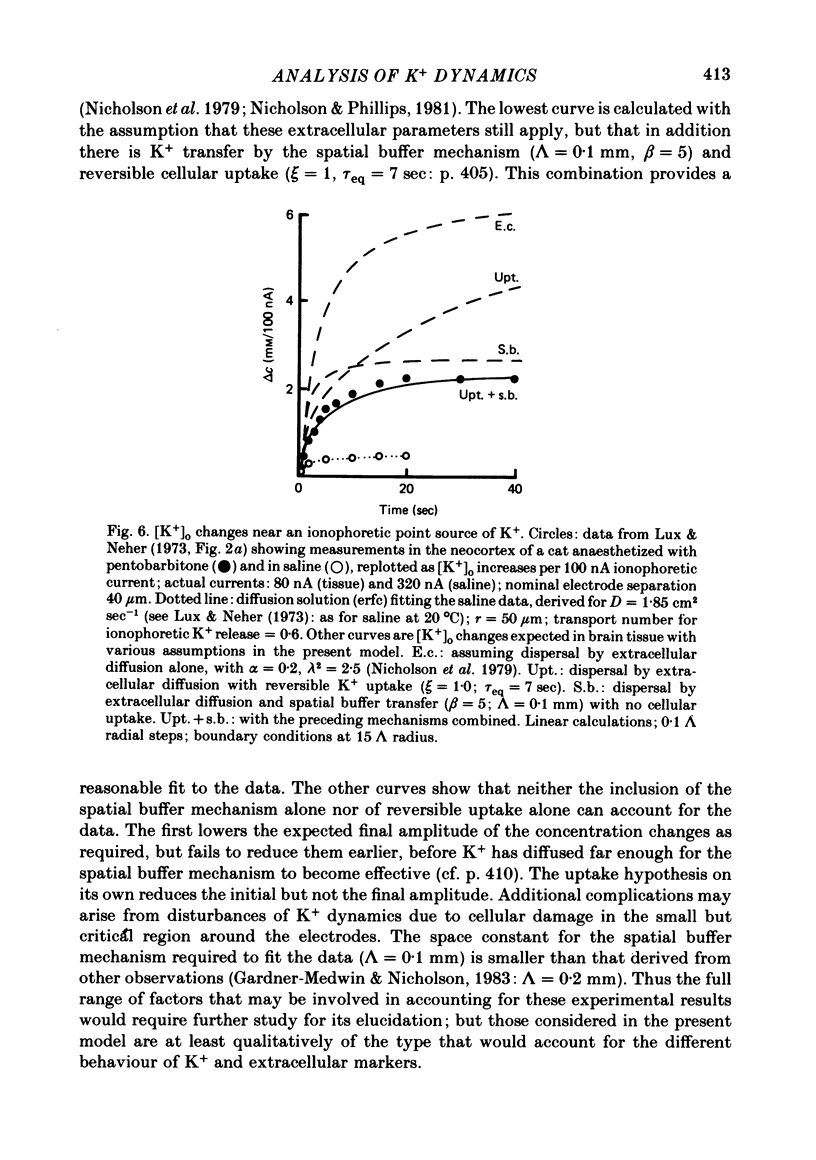
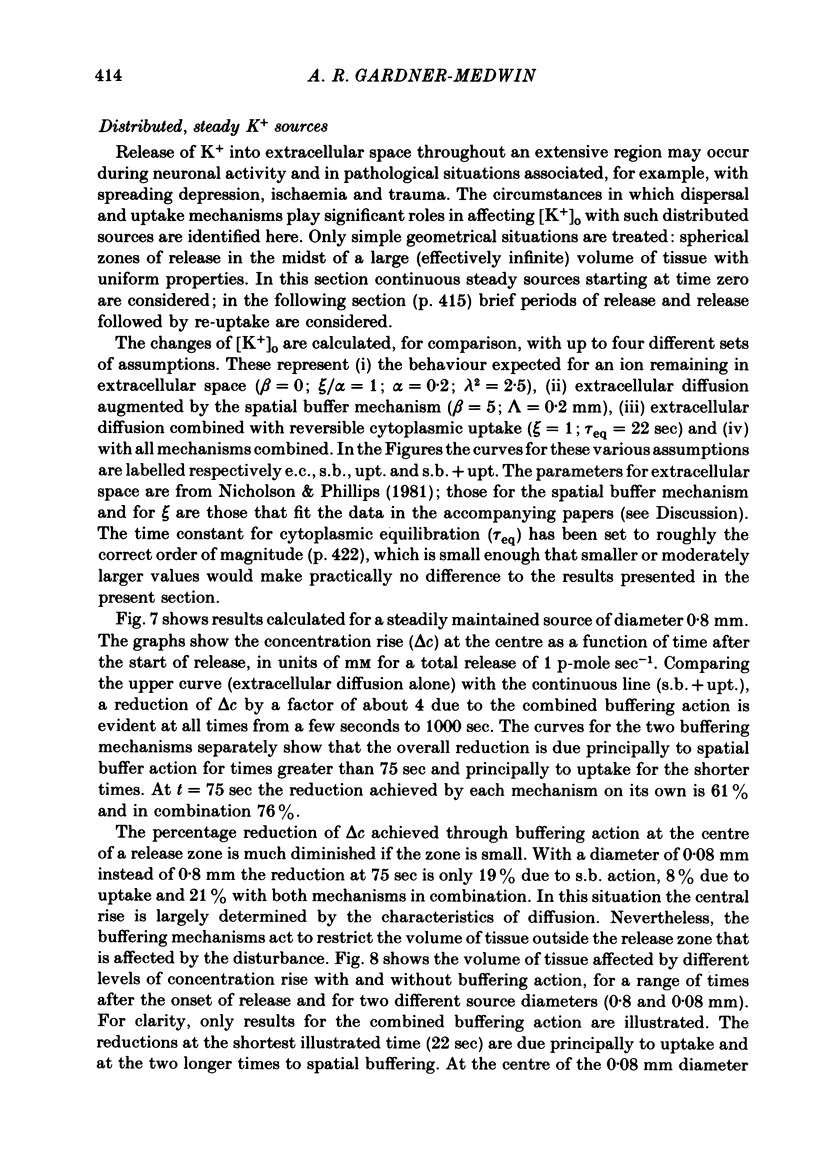
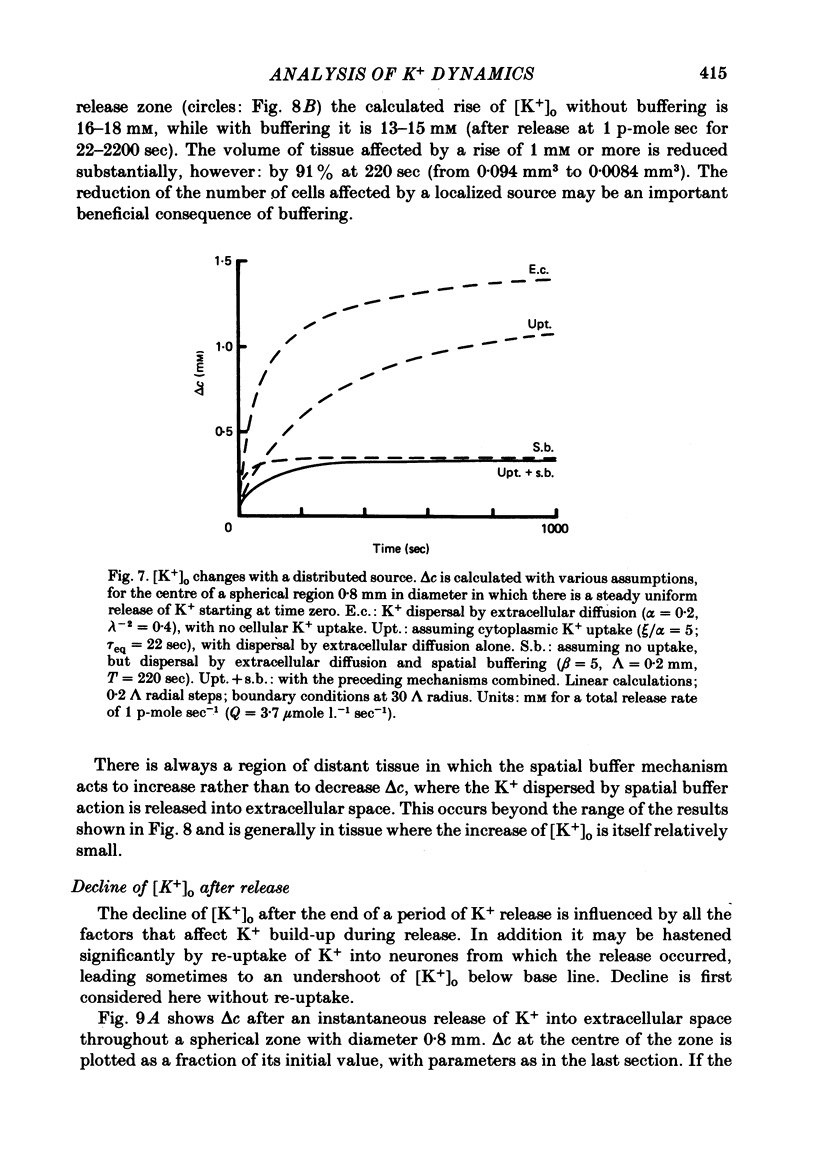
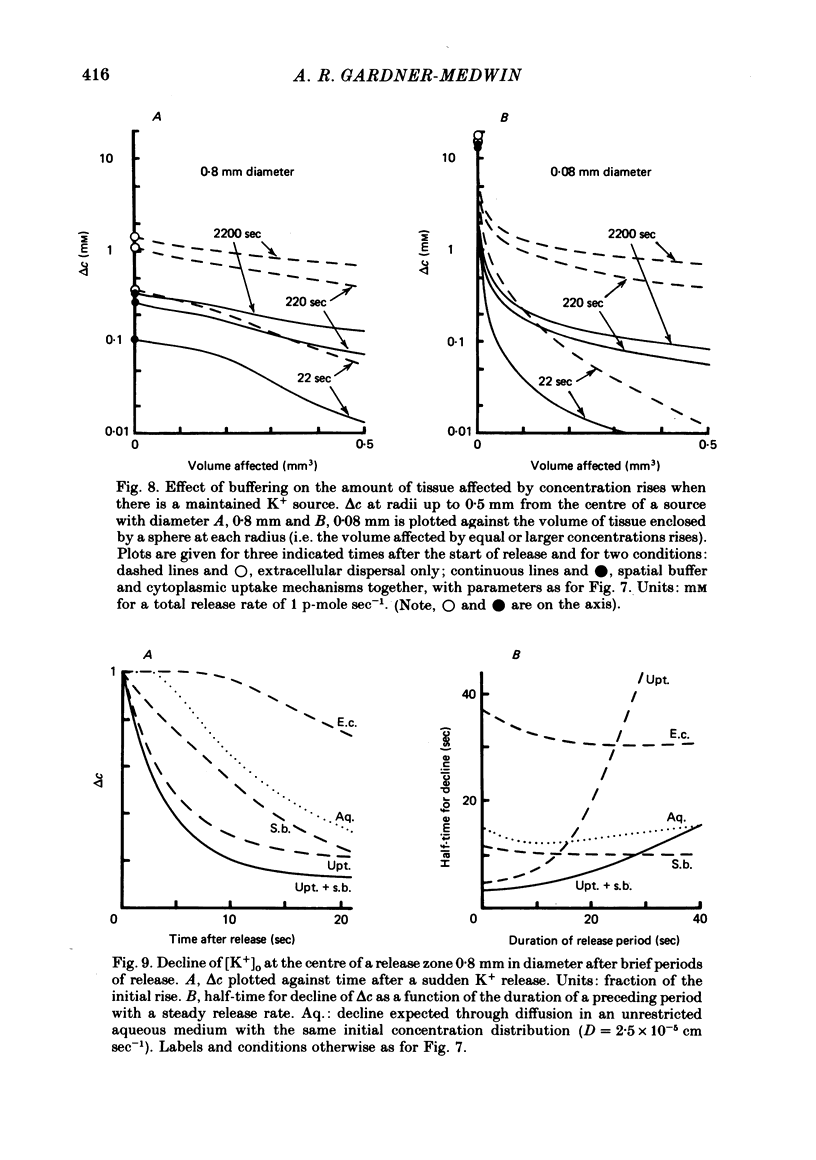
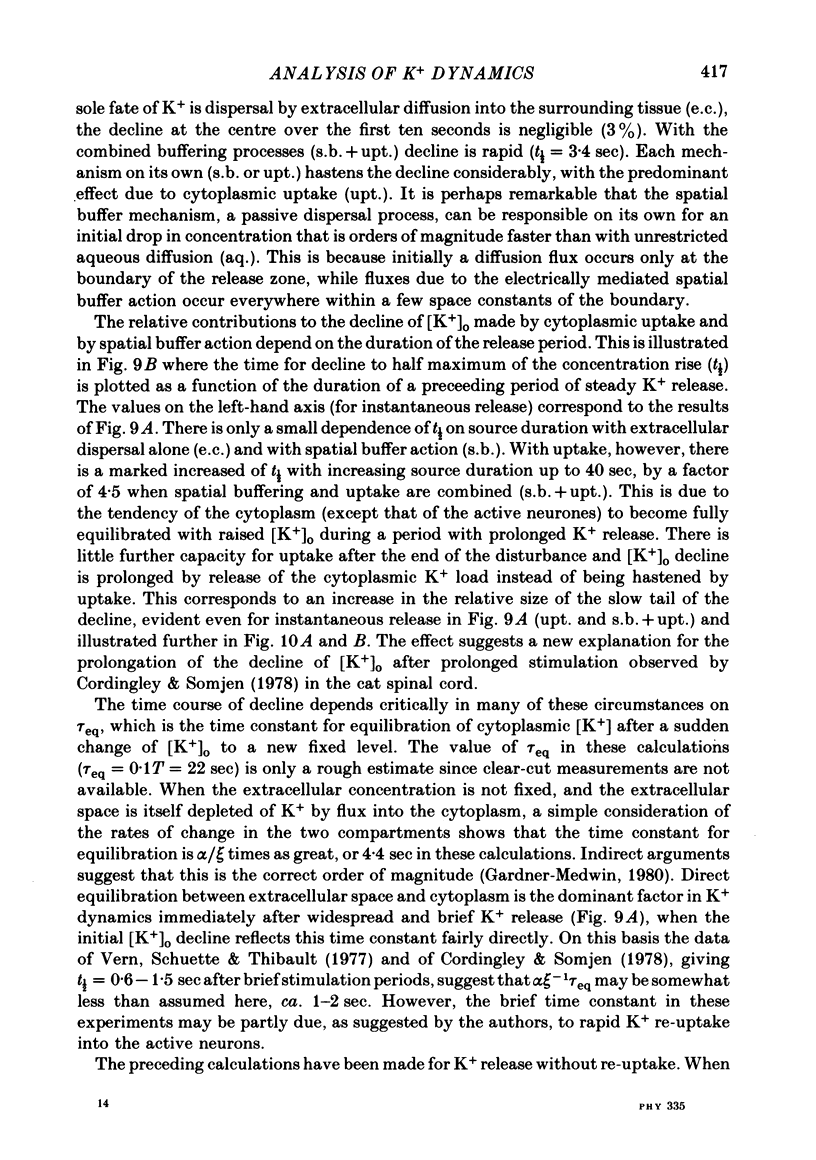
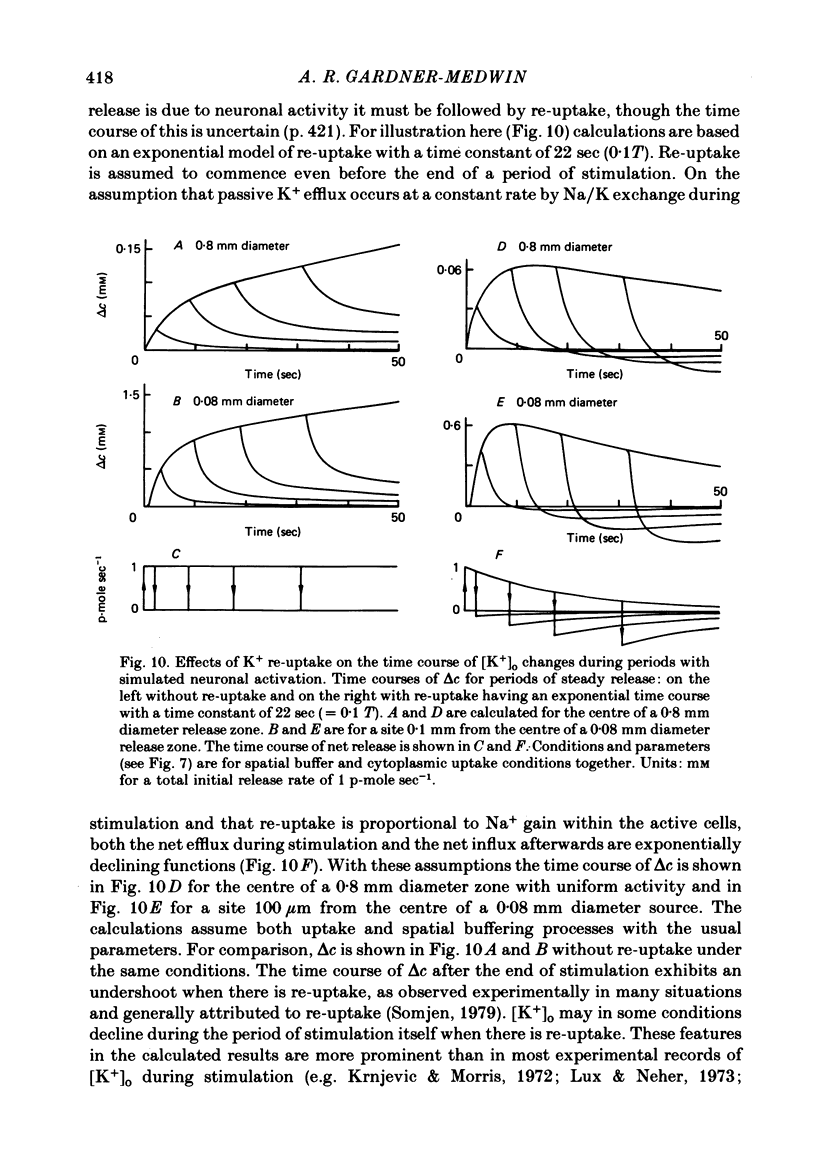
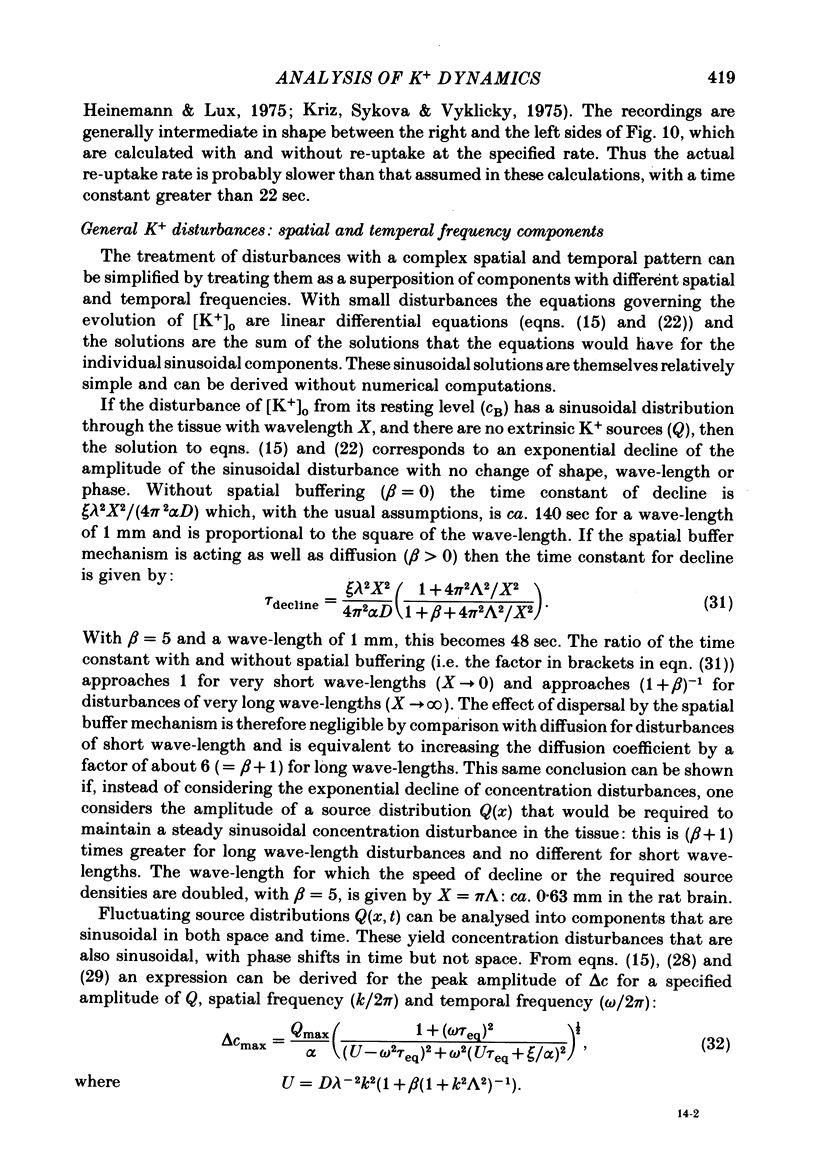
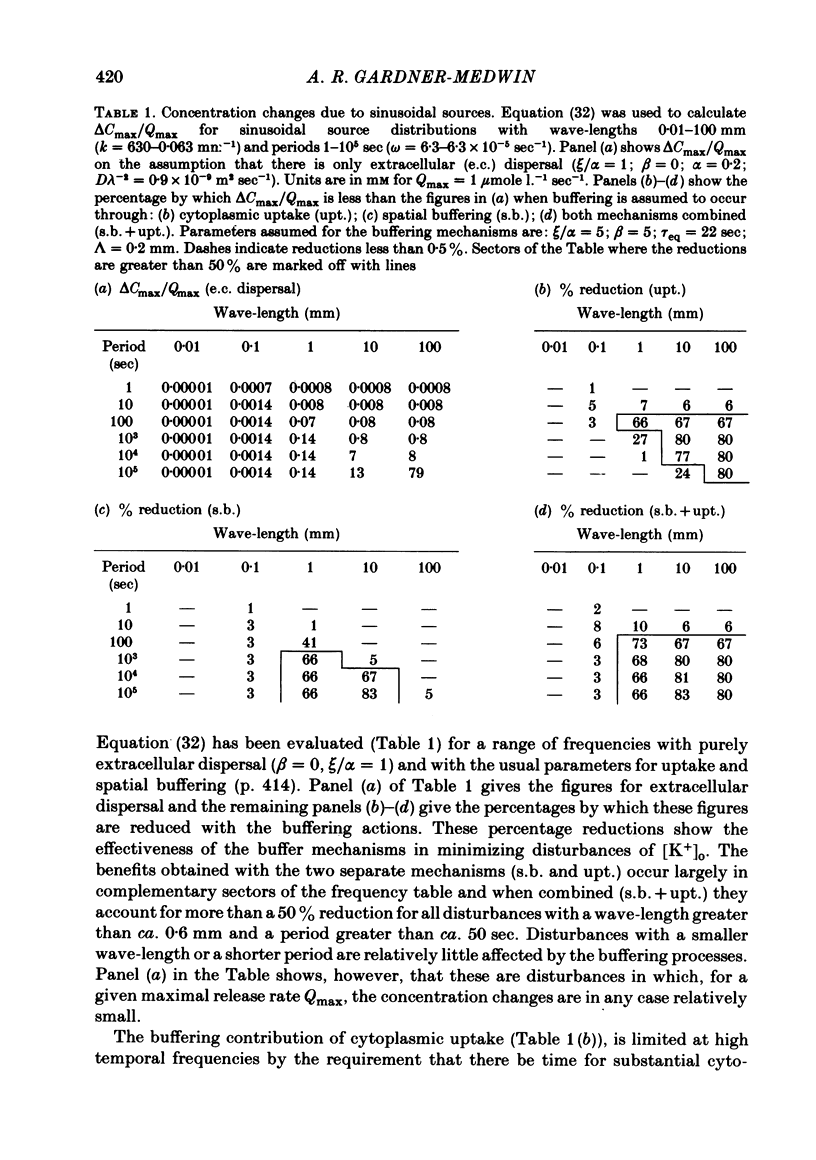
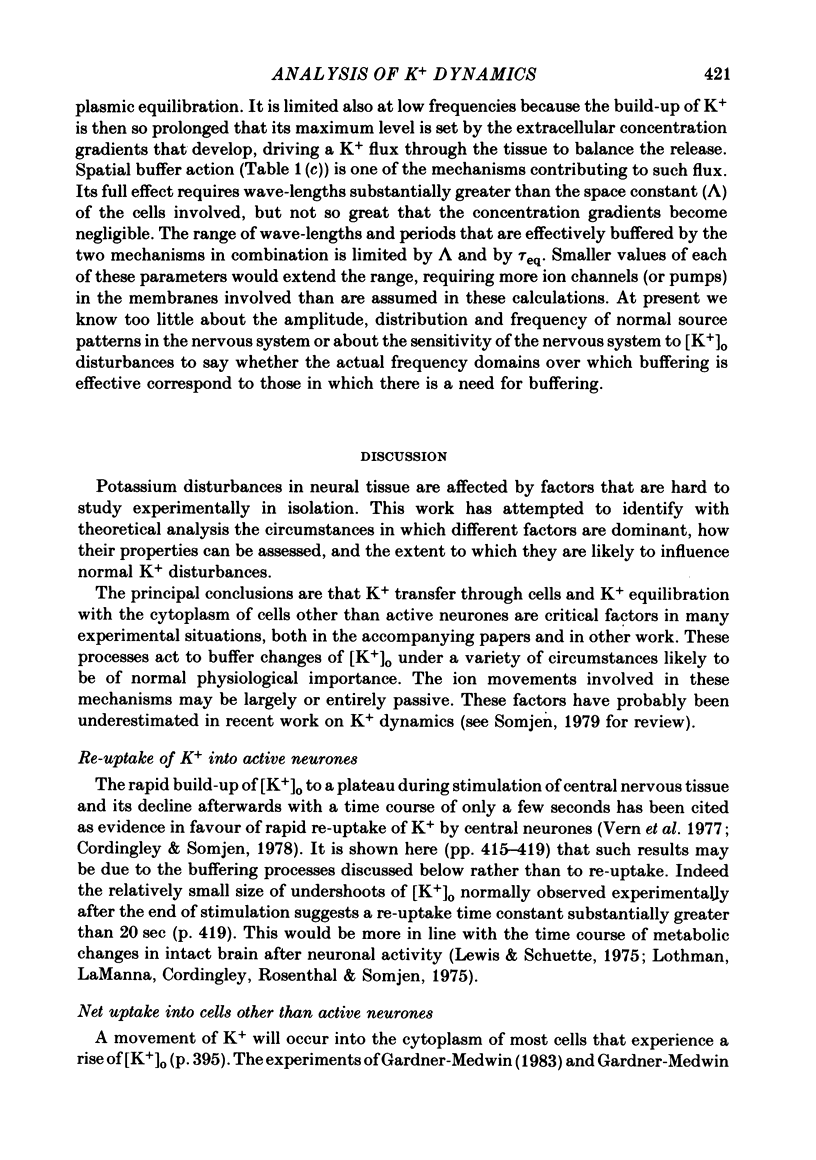
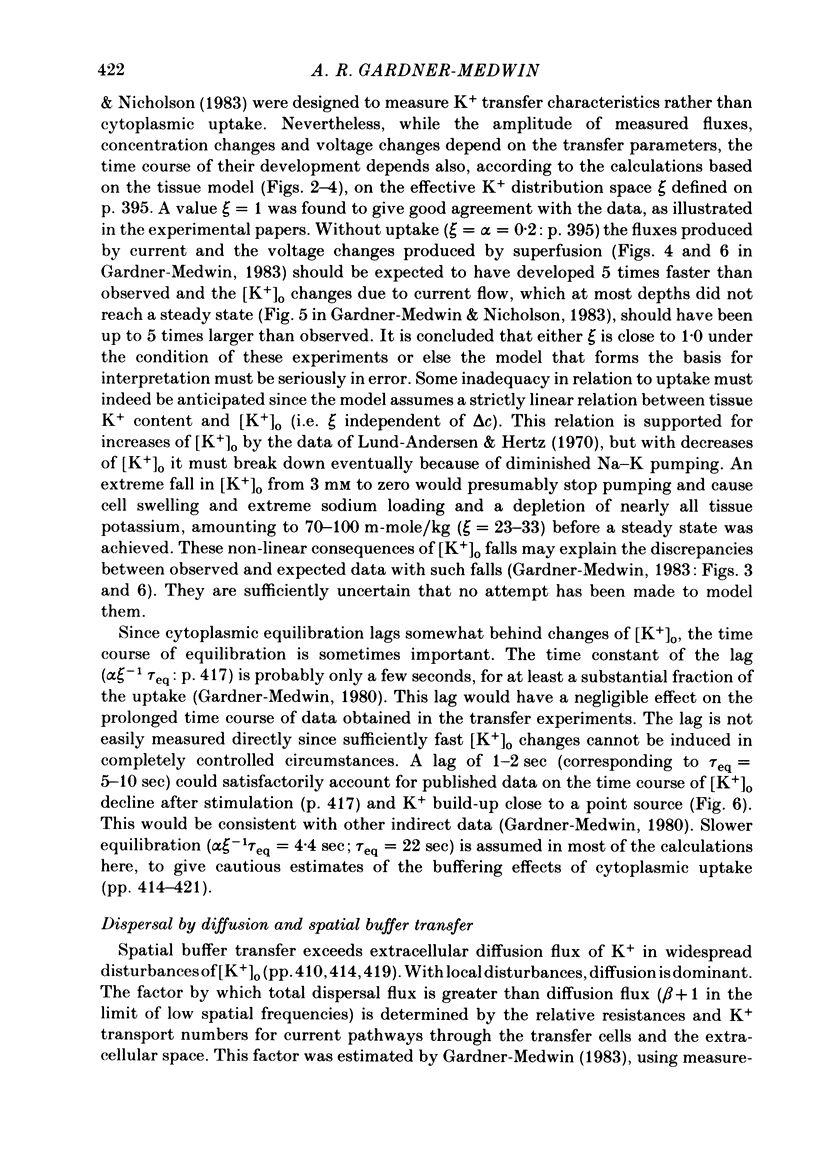
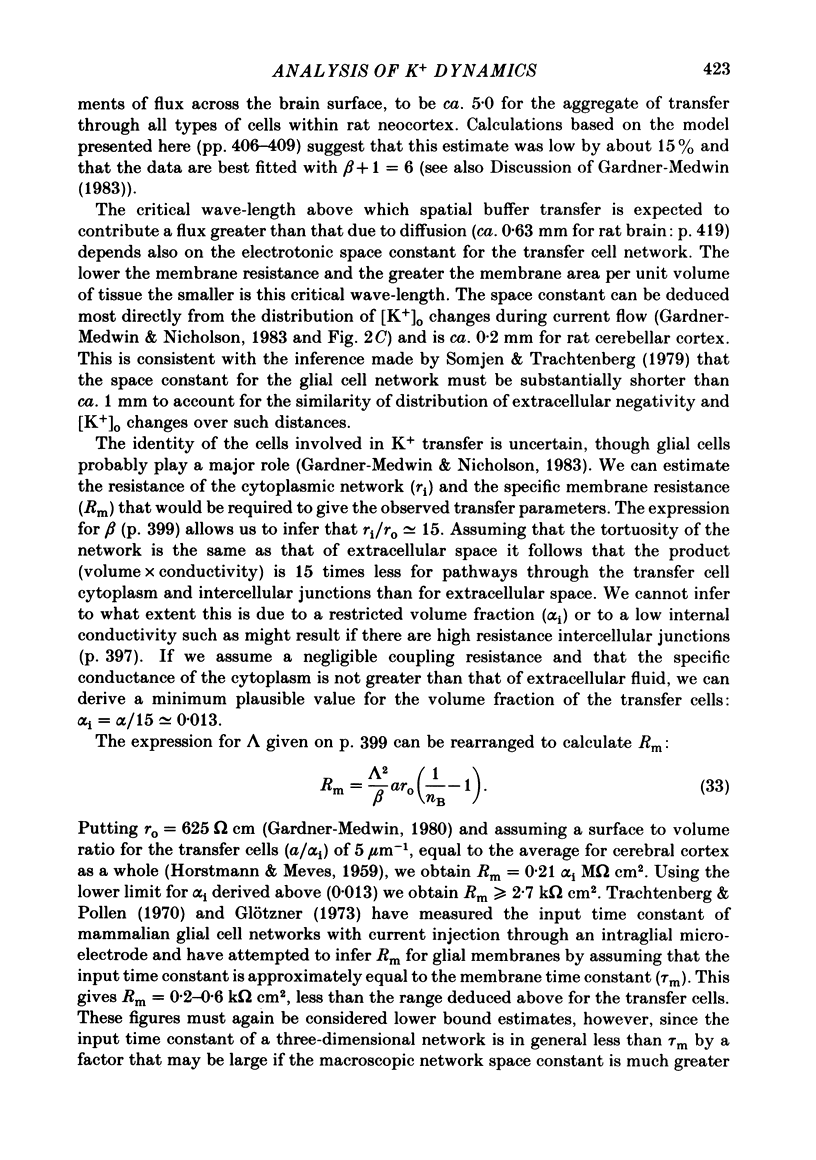
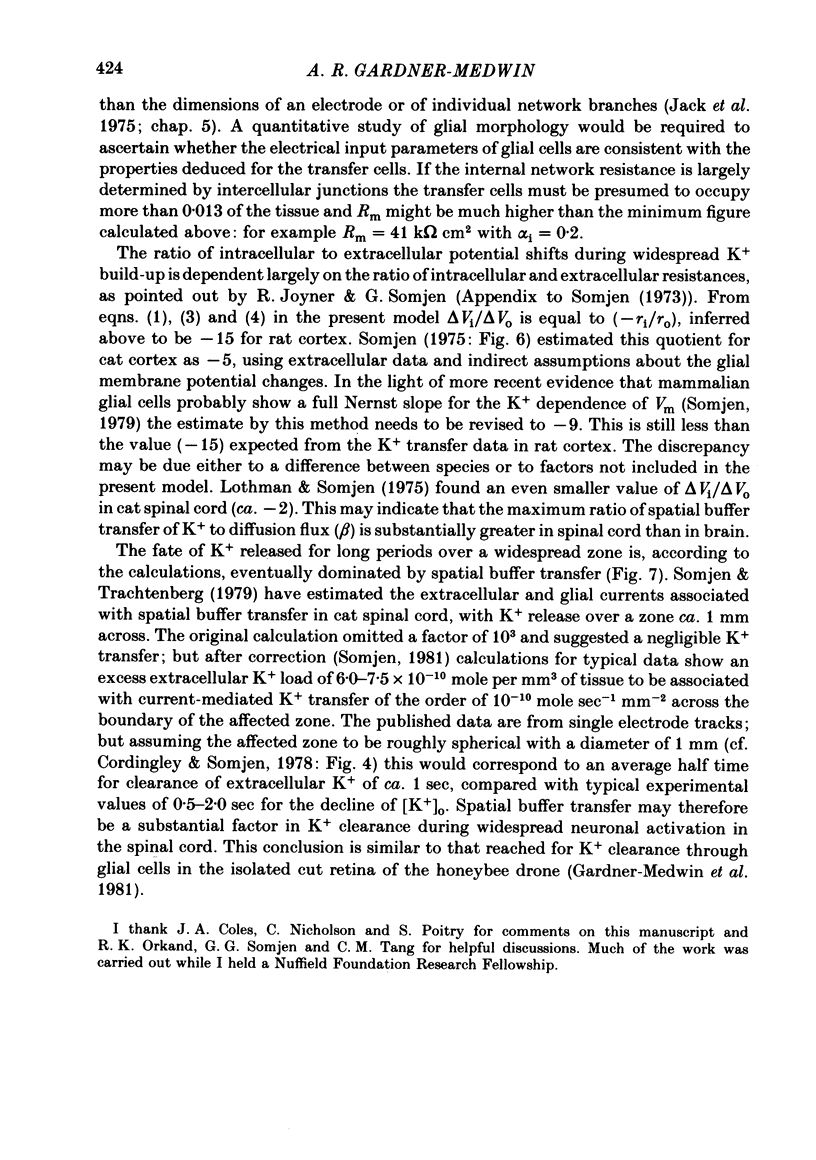
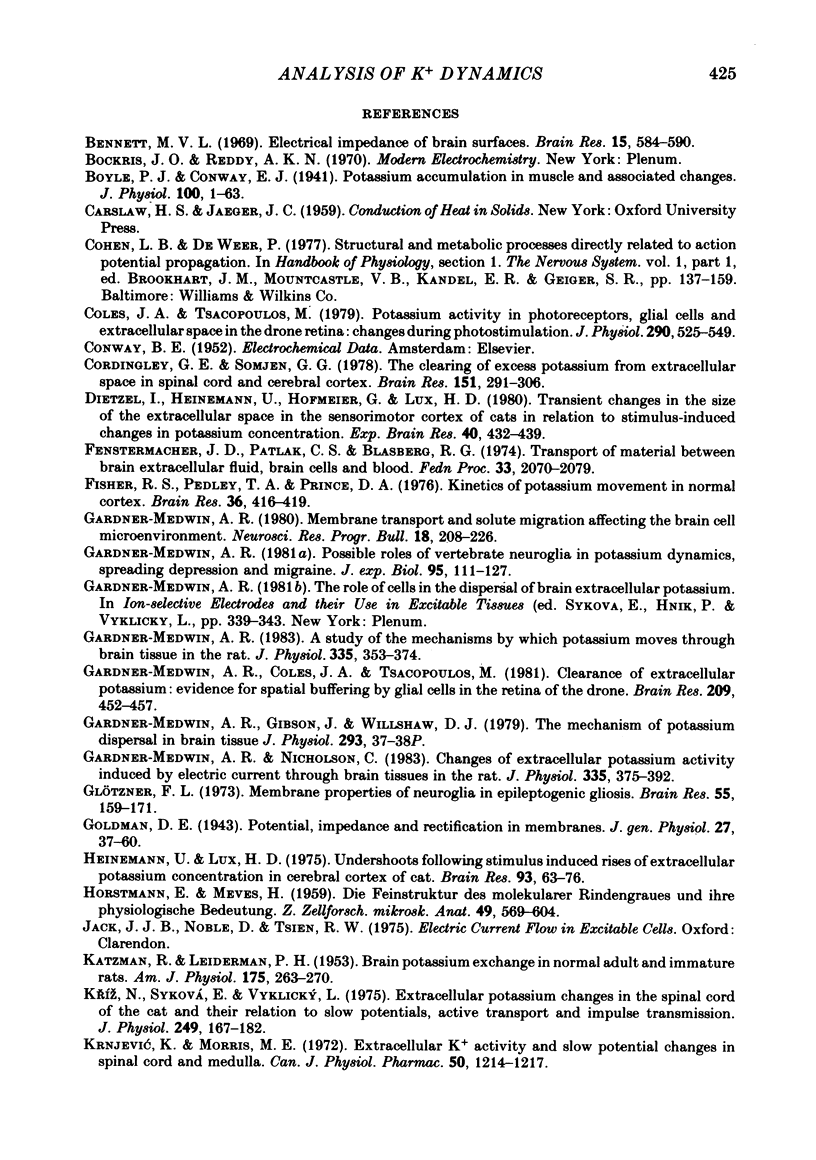
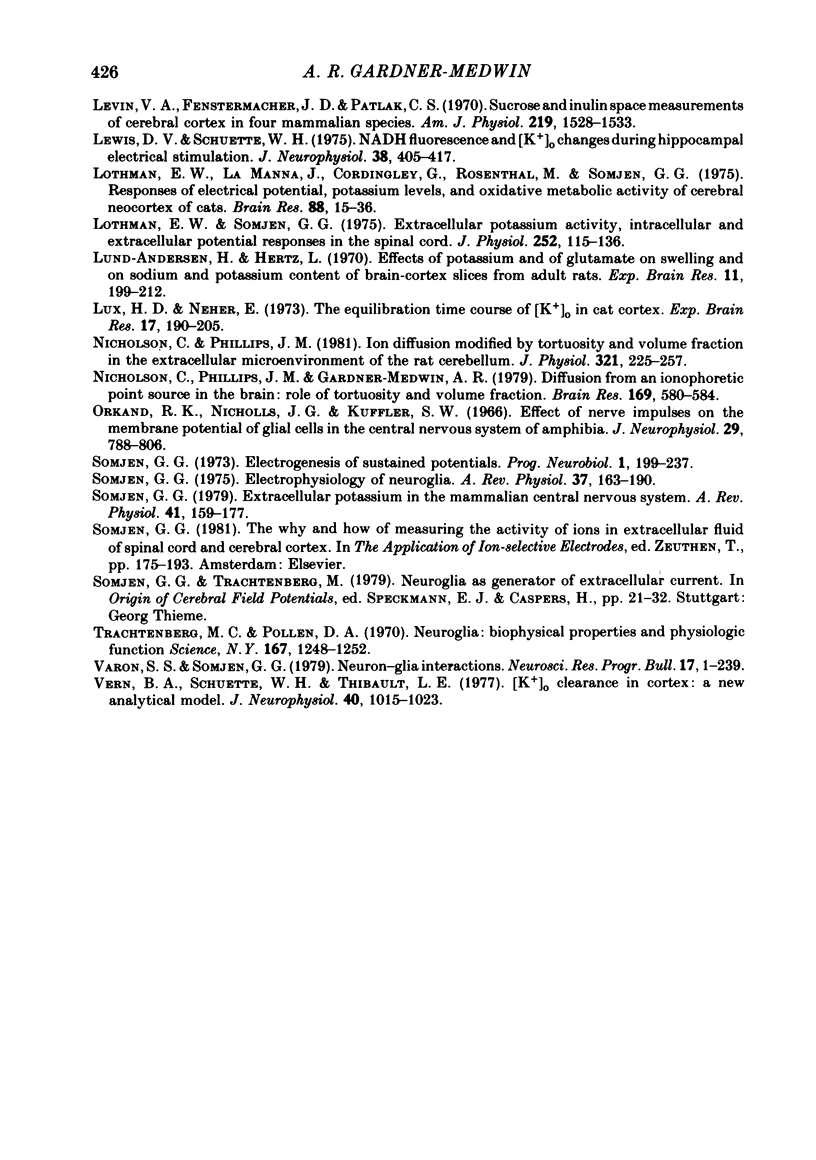
Selected References
These references are in PubMed. This may not be the complete list of references from this article.
- Bennett M. V. Electrical impedance of brain surfaces. Brain Res. 1969 Oct;15(2):584–590. doi: 10.1016/0006-8993(69)90191-7. [DOI] [PubMed] [Google Scholar]
- Boyle P. J., Conway E. J. Potassium accumulation in muscle and associated changes. J Physiol. 1941 Aug 11;100(1):1–63. doi: 10.1113/jphysiol.1941.sp003922. [DOI] [PMC free article] [PubMed] [Google Scholar]
- Coles J. A., Tsacopoulos M. Potassium activity in photoreceptors, glial cells and extracellular space in the drone retina: changes during photostimulation. J Physiol. 1979 May;290(2):525–549. doi: 10.1113/jphysiol.1979.sp012788. [DOI] [PMC free article] [PubMed] [Google Scholar]
- Cordingley G. E., Somjen G. G. The clearing of excess potassium from extracellular space in spinal cord and cerebral cortex. Brain Res. 1978 Aug 4;151(2):291–306. doi: 10.1016/0006-8993(78)90886-7. [DOI] [PubMed] [Google Scholar]
- Dietzel I., Heinemann U., Hofmeier G., Lux H. D. Transient changes in the size of the extracellular space in the sensorimotor cortex of cats in relation to stimulus-induced changes in potassium concentration. Exp Brain Res. 1980;40(4):432–439. doi: 10.1007/BF00236151. [DOI] [PubMed] [Google Scholar]
- Fenstermacher J. D., Patlak C. S., Blasberg R. G. Transport of material between brain extracellular fluid, brain cells and blood. Fed Proc. 1974 Sep;33(9):2070–2074. [PubMed] [Google Scholar]
- Gardner-Medwin A. R. A study of the mechanisms by which potassium moves through brain tissue in the rat. J Physiol. 1983 Feb;335:353–374. doi: 10.1113/jphysiol.1983.sp014539. [DOI] [PMC free article] [PubMed] [Google Scholar]
- Gardner-Medwin A. R., Coles J. A., Tsacopoulos M. Clearance of extracellular potassium: evidence for spatial buffering by glial cells in the retina of the drone. Brain Res. 1981 Mar 30;209(2):452–457. doi: 10.1016/0006-8993(81)90169-4. [DOI] [PubMed] [Google Scholar]
- Gardner-Medwin A. R., Gibson J. L., Willshaw D. J. The mechanism of potassium dispersal in brain tissue [proceedings]. J Physiol. 1979 Aug;293:37P–38P. [PubMed] [Google Scholar]
- Gardner-Medwin A. R., Nicholson C. Changes of extracellular potassium activity induced by electric current through brain tissue in the rat. J Physiol. 1983 Feb;335:375–392. doi: 10.1113/jphysiol.1983.sp014540. [DOI] [PMC free article] [PubMed] [Google Scholar]
- Gardner-Medwin A. R. Possible roles of vertebrate neuroglia in potassium dynamics, spreading depression and migraine. J Exp Biol. 1981 Dec;95:111–127. doi: 10.1242/jeb.95.1.111. [DOI] [PubMed] [Google Scholar]
- Heinemann U., Lux H. D. Undershoots following stimulus-induced rises of extracellular potassium concentration in cerebral cortex of cat. Brain Res. 1975 Jul 25;93(1):63–76. doi: 10.1016/0006-8993(75)90286-3. [DOI] [PubMed] [Google Scholar]
- KATZMAN R., LEIDERMAN P. Brain potassium exchange in normal adult and immature rats. Am J Physiol. 1953 Nov;175(2):263–270. doi: 10.1152/ajplegacy.1953.175.2.263. [DOI] [PubMed] [Google Scholar]
- Krnjević K., Morris M. E. Extracellular K + activity and slow potential changes in spinal cord and medulla. Can J Physiol Pharmacol. 1972 Dec;50(12):1214–1217. doi: 10.1139/y72-177. [DOI] [PubMed] [Google Scholar]
- Krív N., Syková E., Vyklický L. Extracellular potassium changes in the spinal cord of the cat and their relation to slow potentials, active transport and impulse transmission. J Physiol. 1975 Jul;249(1):167–182. doi: 10.1113/jphysiol.1975.sp011009. [DOI] [PMC free article] [PubMed] [Google Scholar]
- Levin V. A., Fenstermacher J. D., Patlak C. S. Sucrose and inulin space measurements of cerebral cortex in four mammalian species. Am J Physiol. 1970 Nov;219(5):1528–1533. doi: 10.1152/ajplegacy.1970.219.5.1528. [DOI] [PubMed] [Google Scholar]
- Lewis D. V., Schuette W. H. NADH fluorescence and [K+]o changes during hippocampal electrical stimulation. J Neurophysiol. 1975 Mar;38(2):405–417. doi: 10.1152/jn.1975.38.2.405. [DOI] [PubMed] [Google Scholar]
- Lothman E. W., Somjen G. G. Extracellular potassium activity, intracellular and extracellular potential responses in the spinal cord. J Physiol. 1975 Oct;252(1):115–136. doi: 10.1113/jphysiol.1975.sp011137. [DOI] [PMC free article] [PubMed] [Google Scholar]
- Lothman E., Lamanna J., Cordingley G., Rosenthal M., Somjen G. Responses of electrical potential, potassium levels, and oxidative metabolic activity of the cerebral neocortex of cats. Brain Res. 1975 Apr 25;88(1):15–36. doi: 10.1016/0006-8993(75)90943-9. [DOI] [PubMed] [Google Scholar]
- Lund-Andersen H., Hertz L. Effects of potassium content in brain-cortex slices from adult rats. Exp Brain Res. 1970;11(2):199–212. doi: 10.1007/BF00234323. [DOI] [PubMed] [Google Scholar]
- Lux H. D., Neher E. The equilibration time course of (K + ) 0 in cat cortex. Exp Brain Res. 1973 Apr 30;17(2):190–205. doi: 10.1007/BF00235028. [DOI] [PubMed] [Google Scholar]
- Nicholson C., Phillips J. M., Gardner-Medwin A. R. Diffusion from an iontophoretic point source in the brain: role of tortuosity and volume fraction. Brain Res. 1979 Jun 29;169(3):580–584. doi: 10.1016/0006-8993(79)90408-6. [DOI] [PubMed] [Google Scholar]
- Nicholson C., Phillips J. M. Ion diffusion modified by tortuosity and volume fraction in the extracellular microenvironment of the rat cerebellum. J Physiol. 1981 Dec;321:225–257. doi: 10.1113/jphysiol.1981.sp013981. [DOI] [PMC free article] [PubMed] [Google Scholar]
- Orkand R. K., Nicholls J. G., Kuffler S. W. Effect of nerve impulses on the membrane potential of glial cells in the central nervous system of amphibia. J Neurophysiol. 1966 Jul;29(4):788–806. doi: 10.1152/jn.1966.29.4.788. [DOI] [PubMed] [Google Scholar]
- Somjen G. G. Electrogenesis of sustained potentials. Prog Neurobiol. 1973;1(3):201–237. [PubMed] [Google Scholar]
- Somjen G. G. Electrophysiology of neuroglia. Annu Rev Physiol. 1975;37:163–190. doi: 10.1146/annurev.ph.37.030175.001115. [DOI] [PubMed] [Google Scholar]
- Somjen G. G. Extracellular potassium in the mammalian central nervous system. Annu Rev Physiol. 1979;41:159–177. doi: 10.1146/annurev.ph.41.030179.001111. [DOI] [PubMed] [Google Scholar]
- Trachtenberg M. C., Pollen D. A. Neuroglia: biophysical properties and physiologic function. Science. 1970 Feb 27;167(3922):1248–1252. doi: 10.1126/science.167.3922.1248. [DOI] [PubMed] [Google Scholar]
- Varon S. S., Somjen G. G. Neuron-glia interactions. Neurosci Res Program Bull. 1979 Feb;17(1):1–239. [PubMed] [Google Scholar]
- Vern B. A., Schuette W. H., Thibault L. E. [K+]o clearance in cortex: a new analytical model. J Neurophysiol. 1977 Sep;40(5):1015–1023. doi: 10.1152/jn.1977.40.5.1015. [DOI] [PubMed] [Google Scholar]