Abstract
Intracellular Na activity (aiNa) was measured with recessed-tip, Na-selective micro-electrodes in voltage-clamped sheep cardiac Purkinje fibres. Tension was measured simultaneously. aiNa was increased reversibly either by exposing the preparation to K-free, Rb-free solution of by adding the cardioactive steroid strophanthidin. An increase of aiNa produced an increase of tonic tension which was larger at depolarized membrane potentials. At sufficiently negative membrane potentials, changes of aiNa (over the range 6-30 mM) had no effect on tonic tension. Therefore, both an increase of aiNa and a depolarization are required to increase tonic tension. It is concluded that either a low level of aiNa or a large negative membrane potential is sufficient to maintain a low intracellular Ca concentration. Tonic tension was measured as a function of aiNa. At a given membrane potential the relationship can be described empirically by an equation of the form: tonic tension = b(aiNa)y, where y is a constant and b depends on membrane potential. In five experiments y was found to be 3.7 +/- 0.7 (mean +/- S.E.M.) over a range of potentials from -60 to -10 mV. Tonic tension was measured as a function of membrane potential. At a given aiNa the relationship can be described approximately as: tonic tension = k exp (aV), where a is a constant and k depends on aiNa. In five experiments a was found to be 0.06 +/- 0.01 mV-1 (mean +/- S.E.M.). A depolarization of 10 mV increases tonic tension by the same amount as does an increase of aiNa that is equivalent to a 3.7 mV change of the Na equilibrium potential, ENa. Hence ENa is nearly 3 times more effective than membrane potential in controlling tonic tension. During a prolonged depolarization (several minutes) the initial increase of tonic tension decays gradually. This is associated with a fall of aiNa. The relationship between tonic tension and aiNa is similar to that seen when aiNa is increased by inhibiting the Na pump. It is concluded that the fall of aiNa is responsible for the decay of tonic tension. The changes of tonic tension reported in this paper are consistent with the effects of aiNa and membrane potential on a voltage-dependent Na-Ca exchange. The possibility that a voltage-dependent Ca channel contributes to tonic tension is also discussed.
Full text
PDF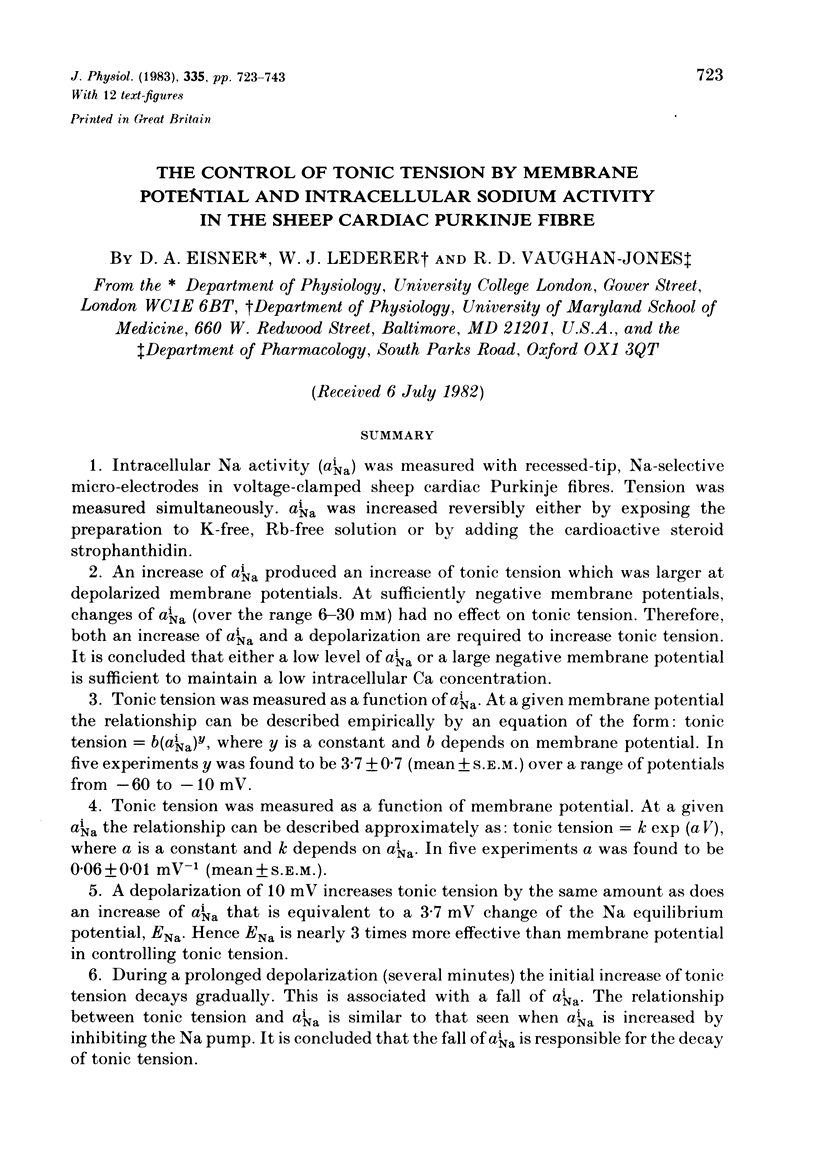
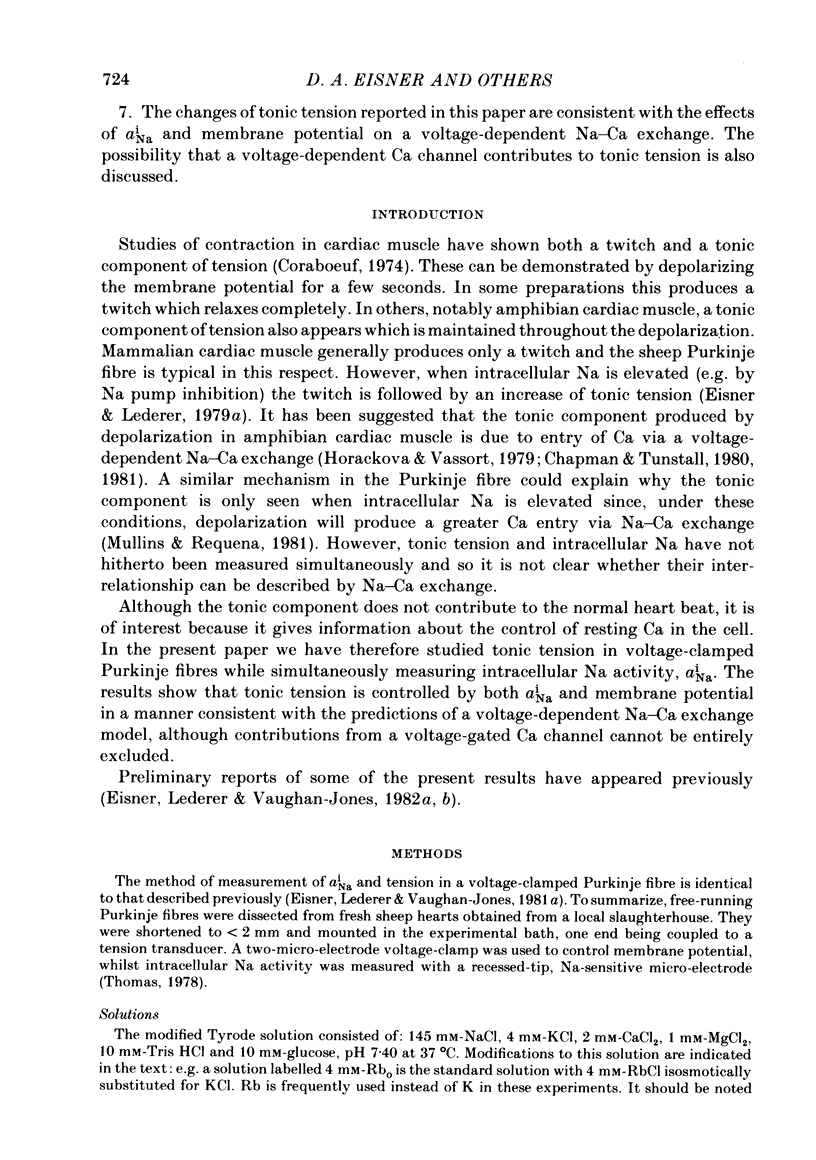
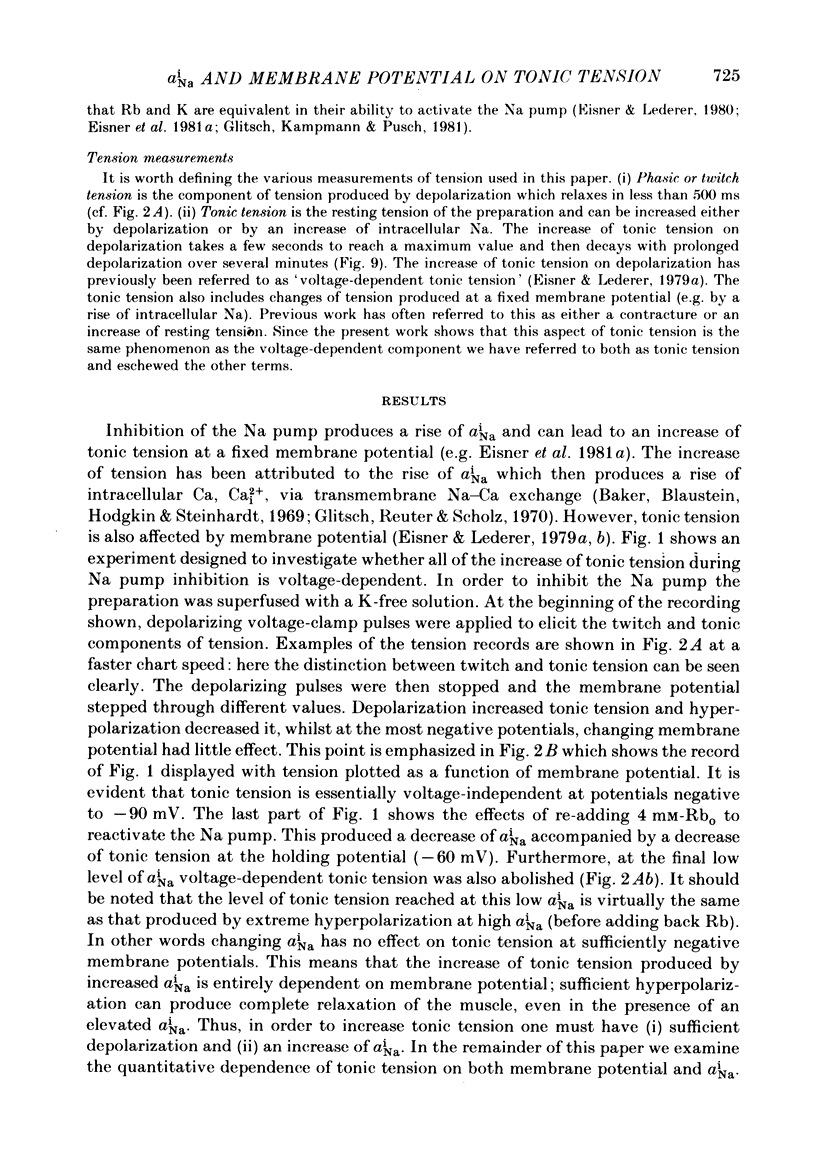
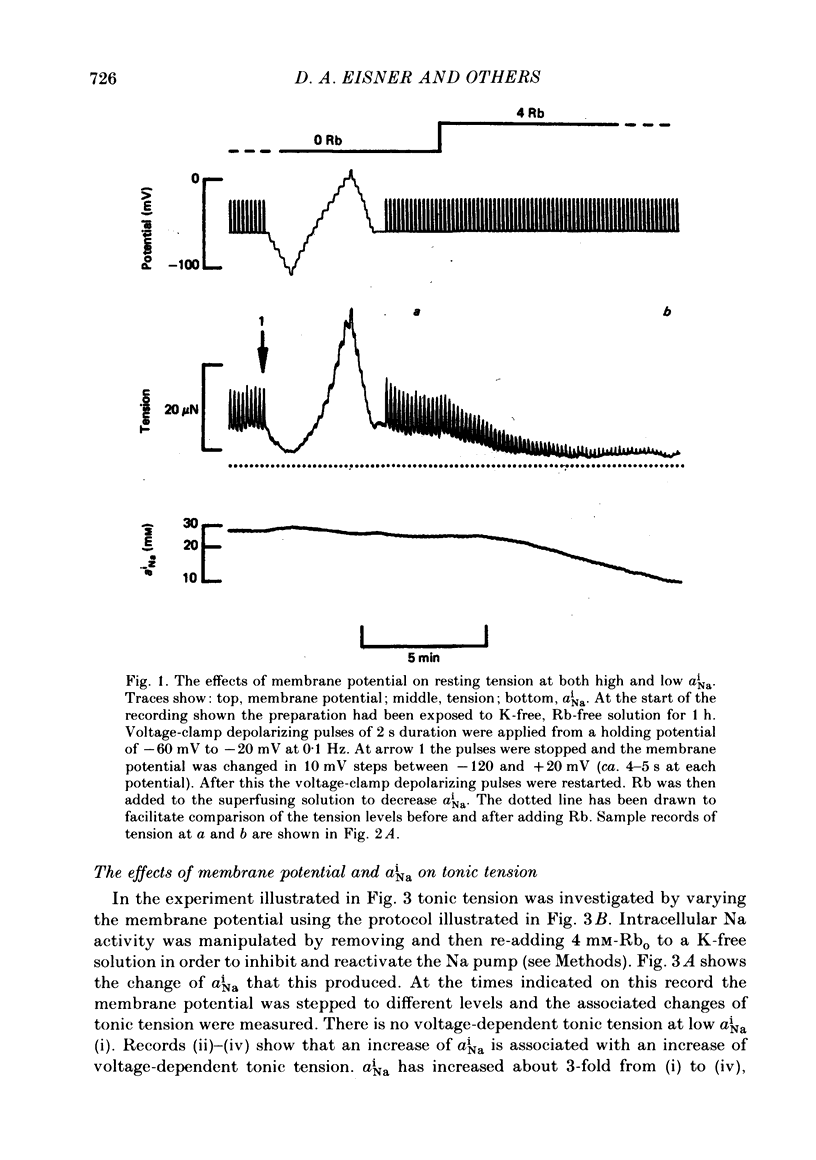
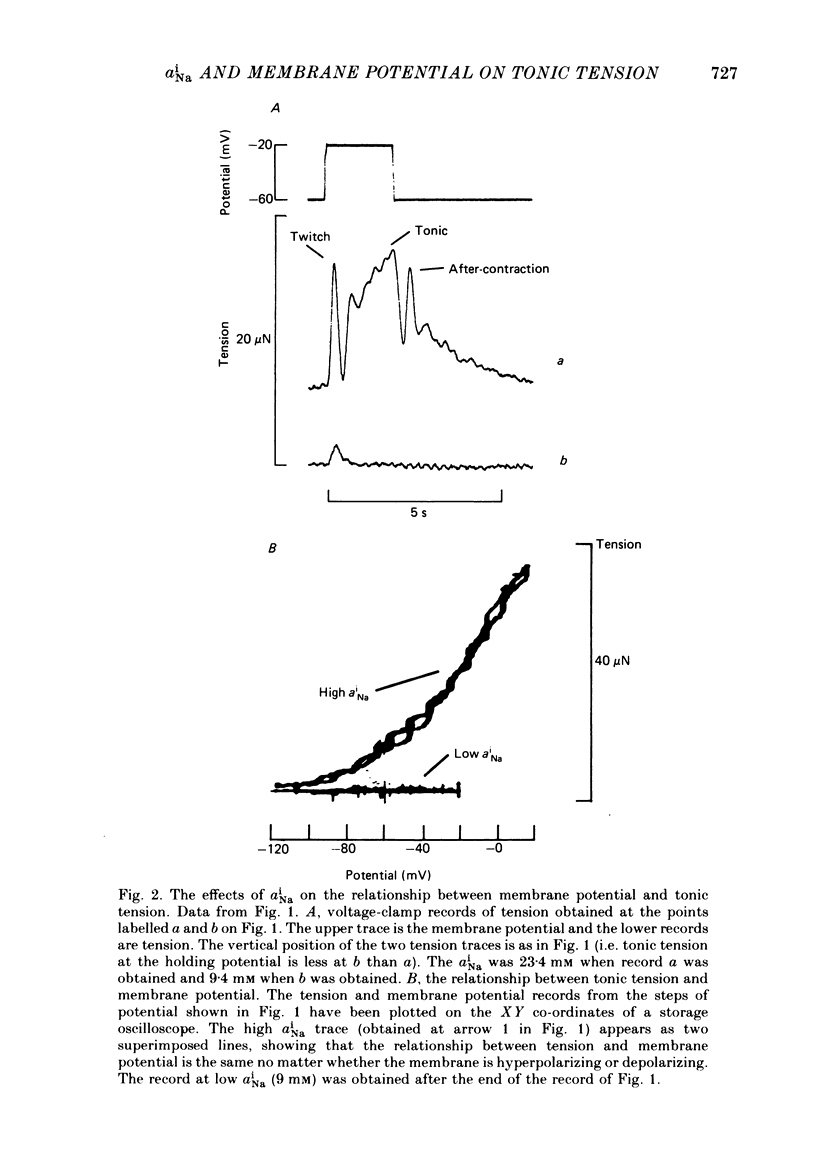
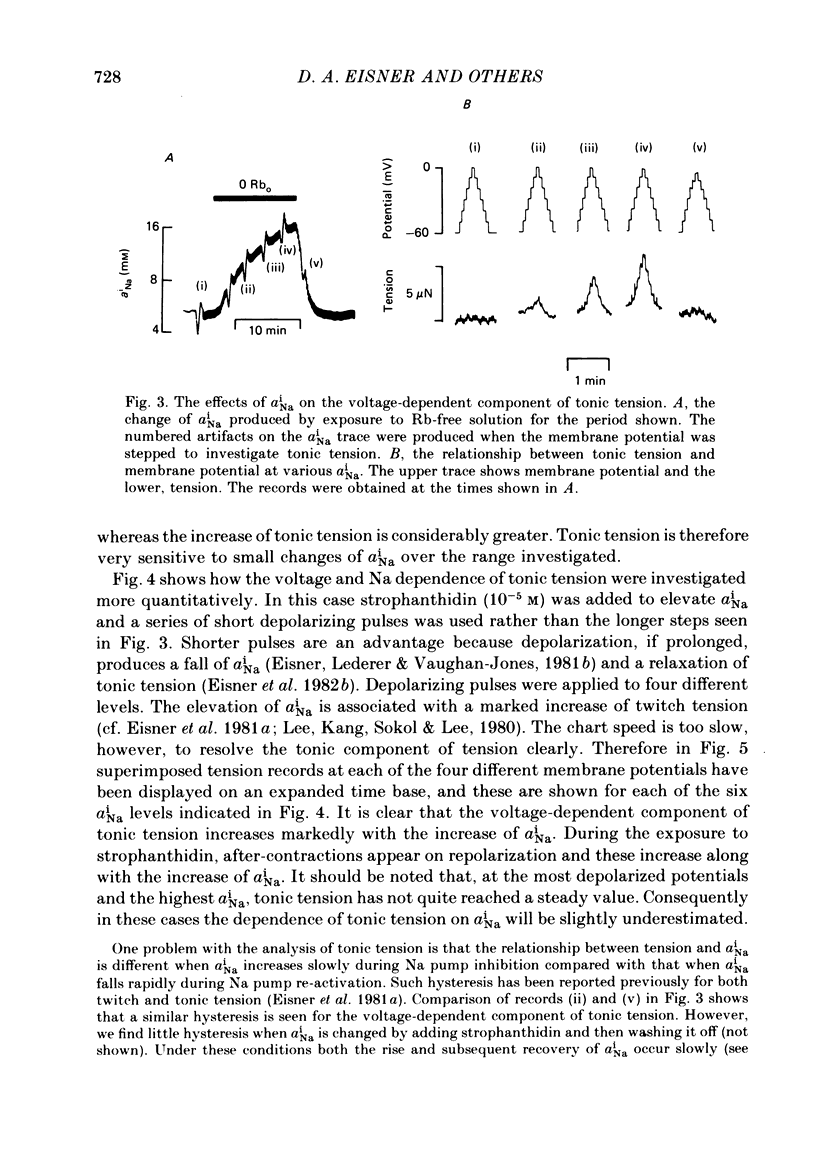
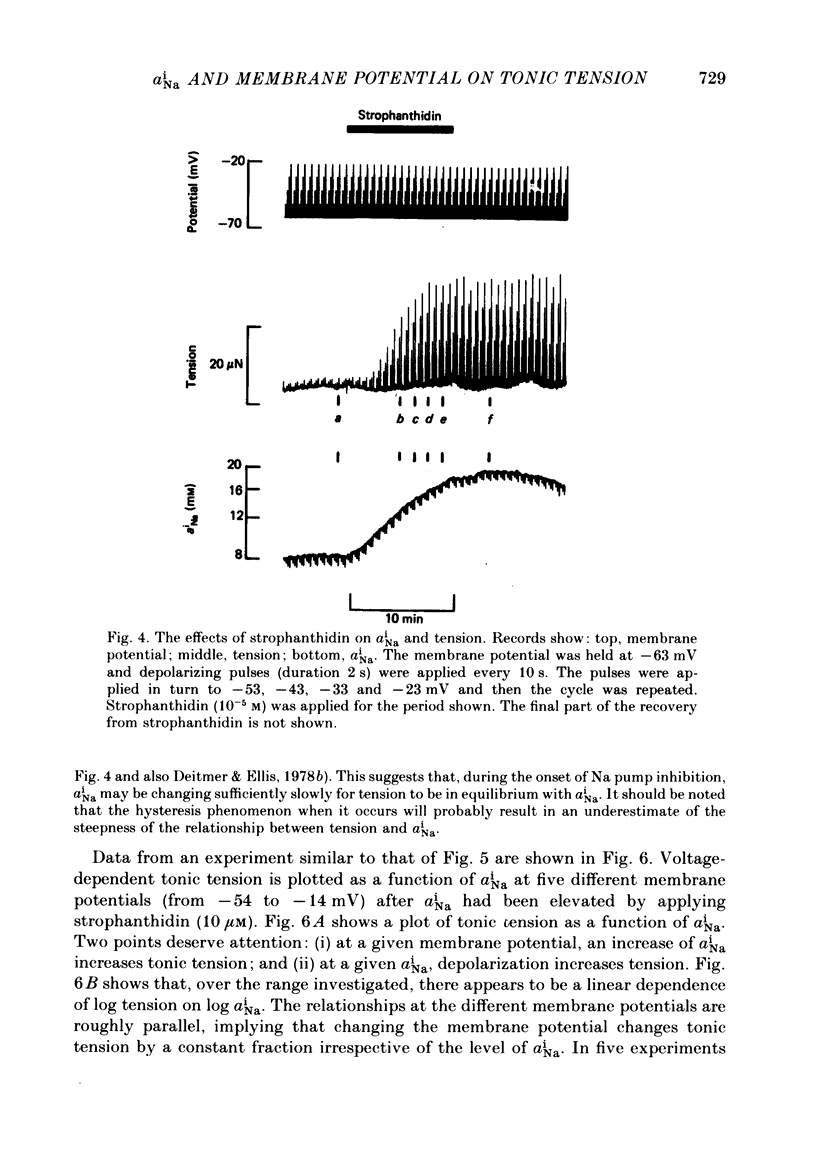
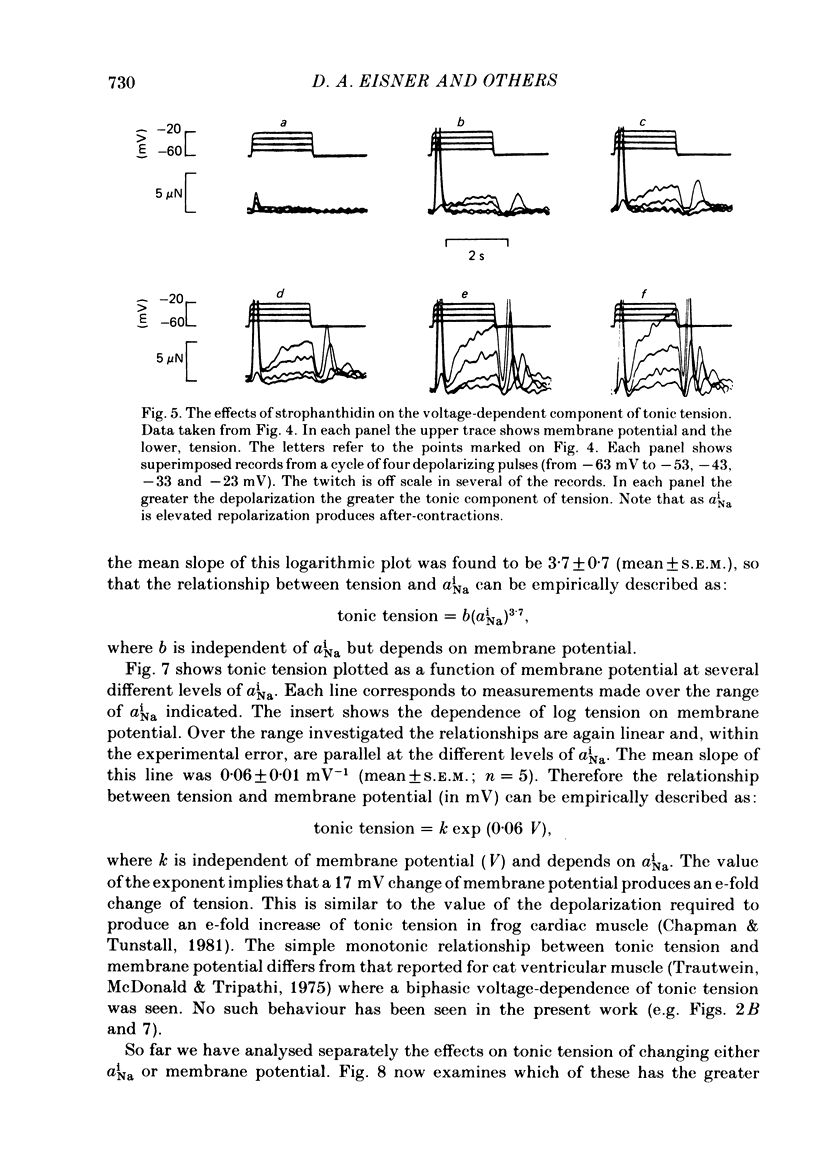
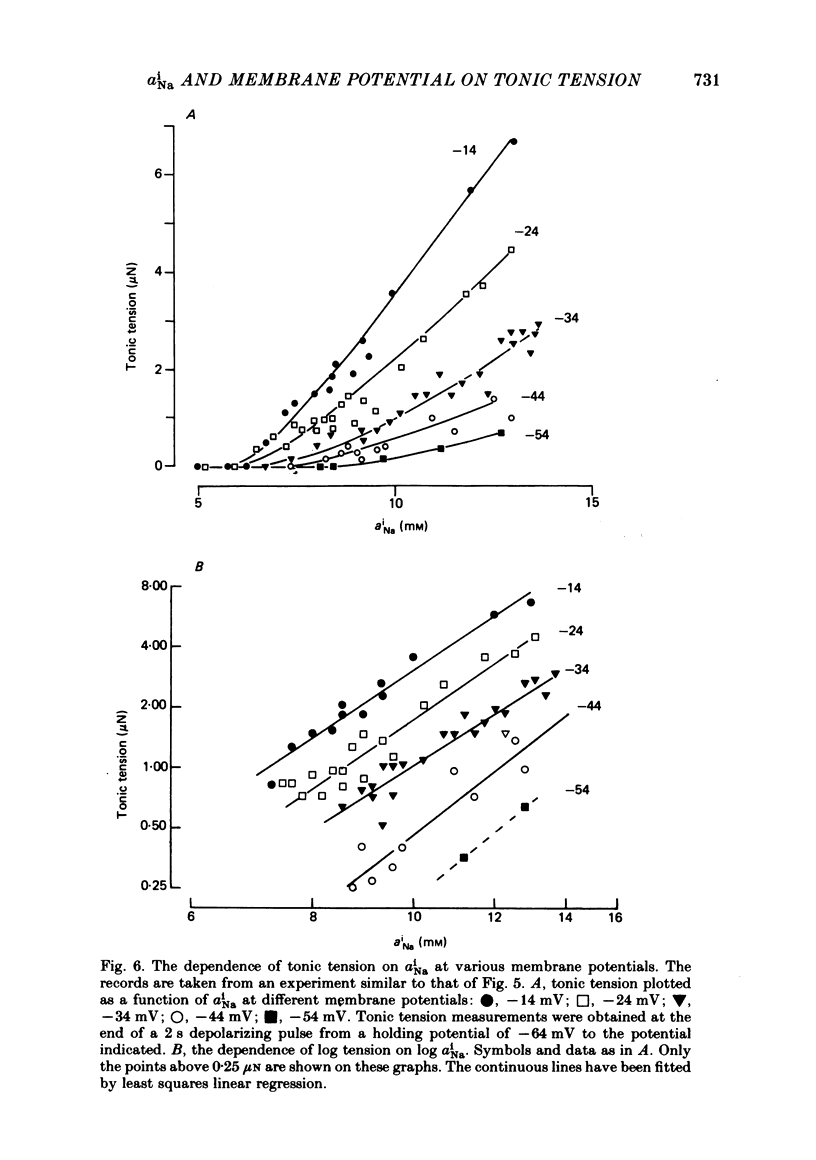
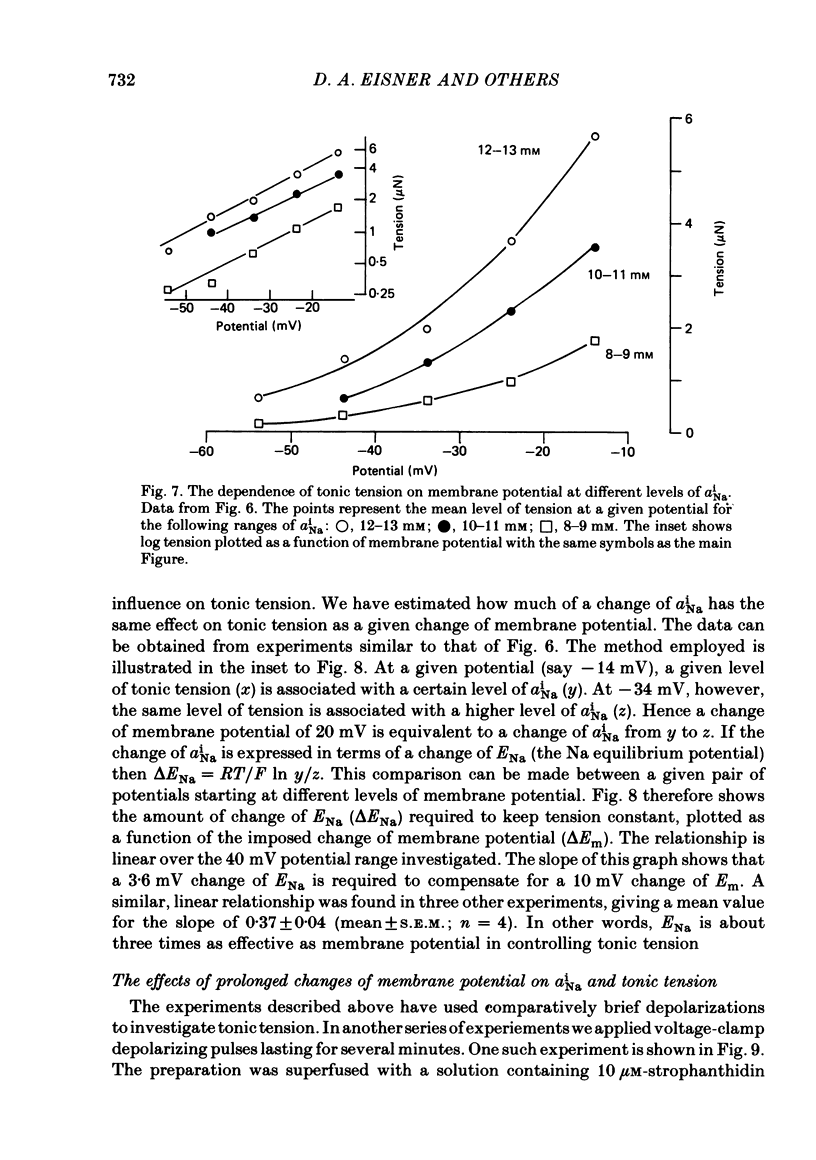
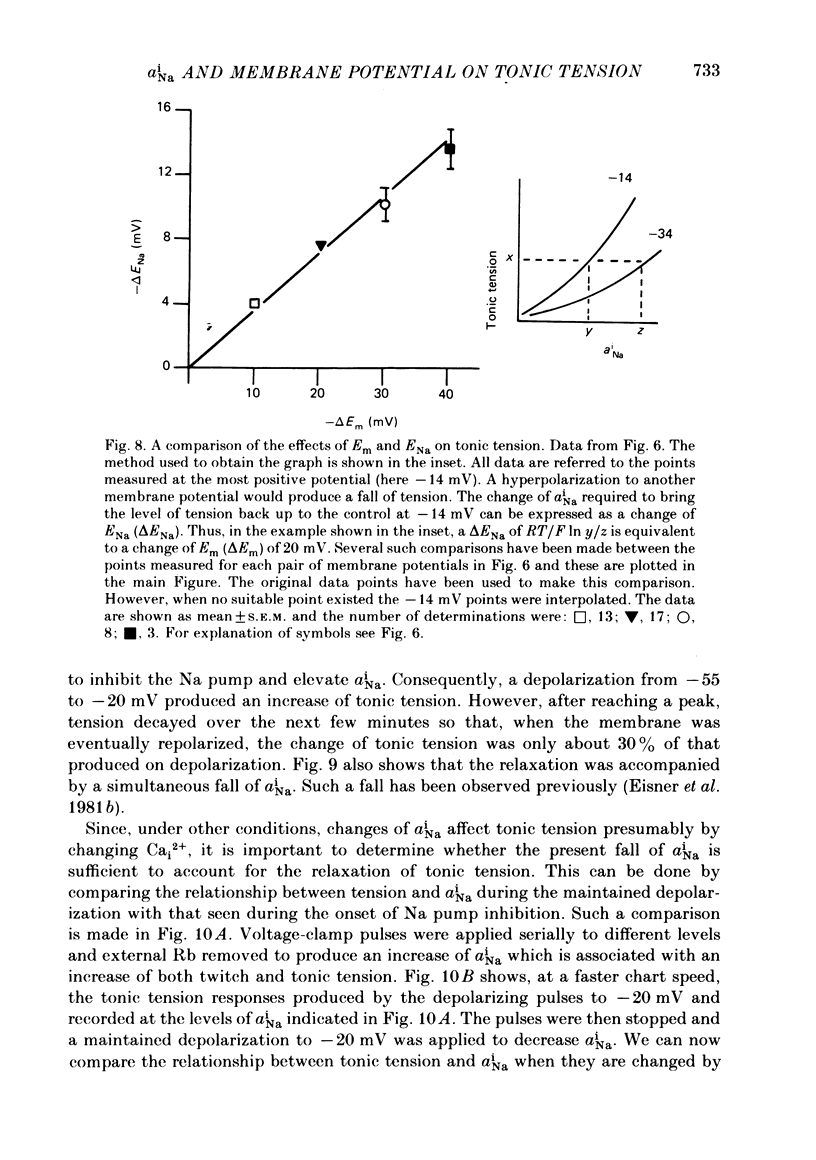
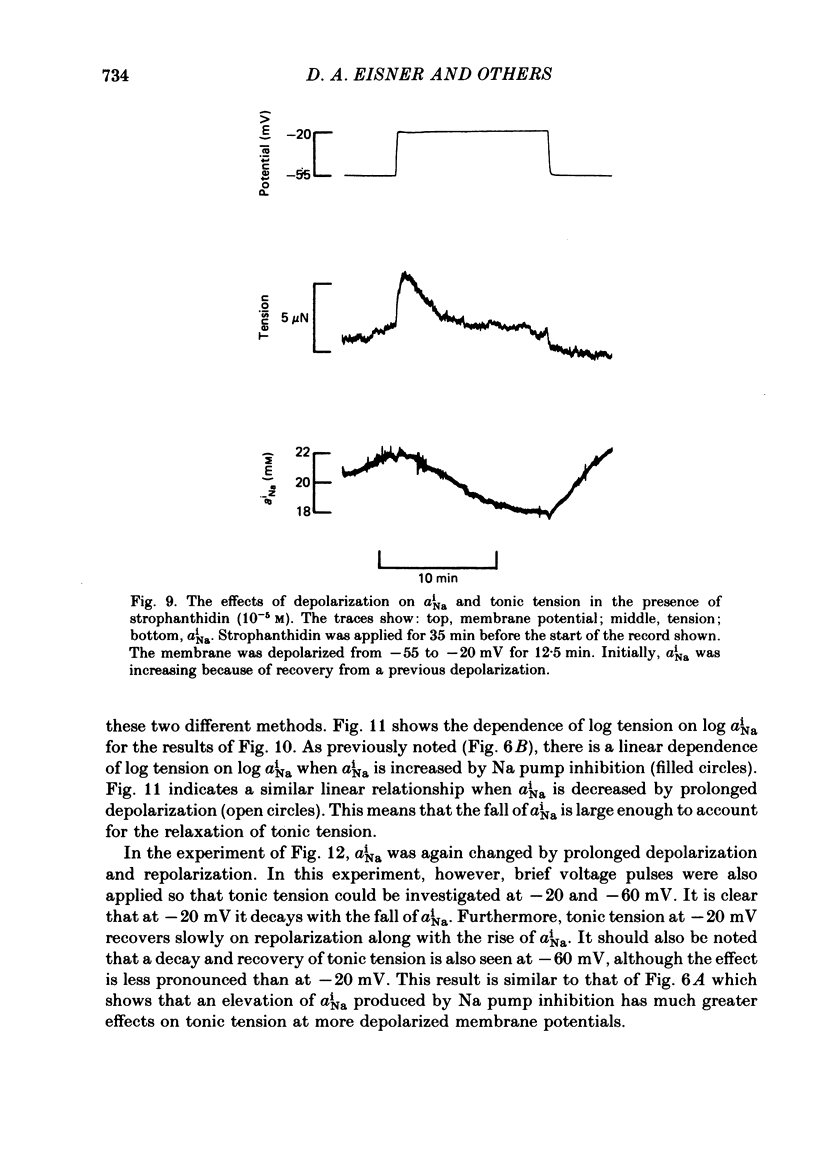
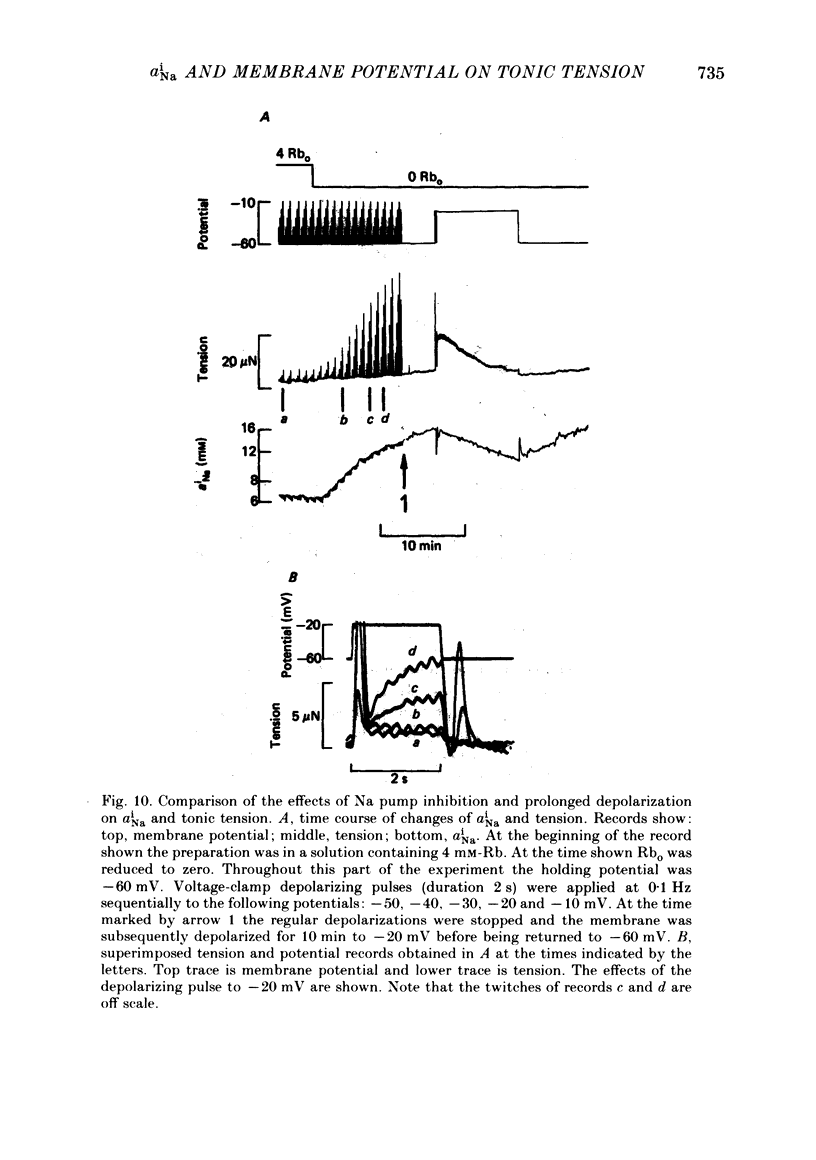
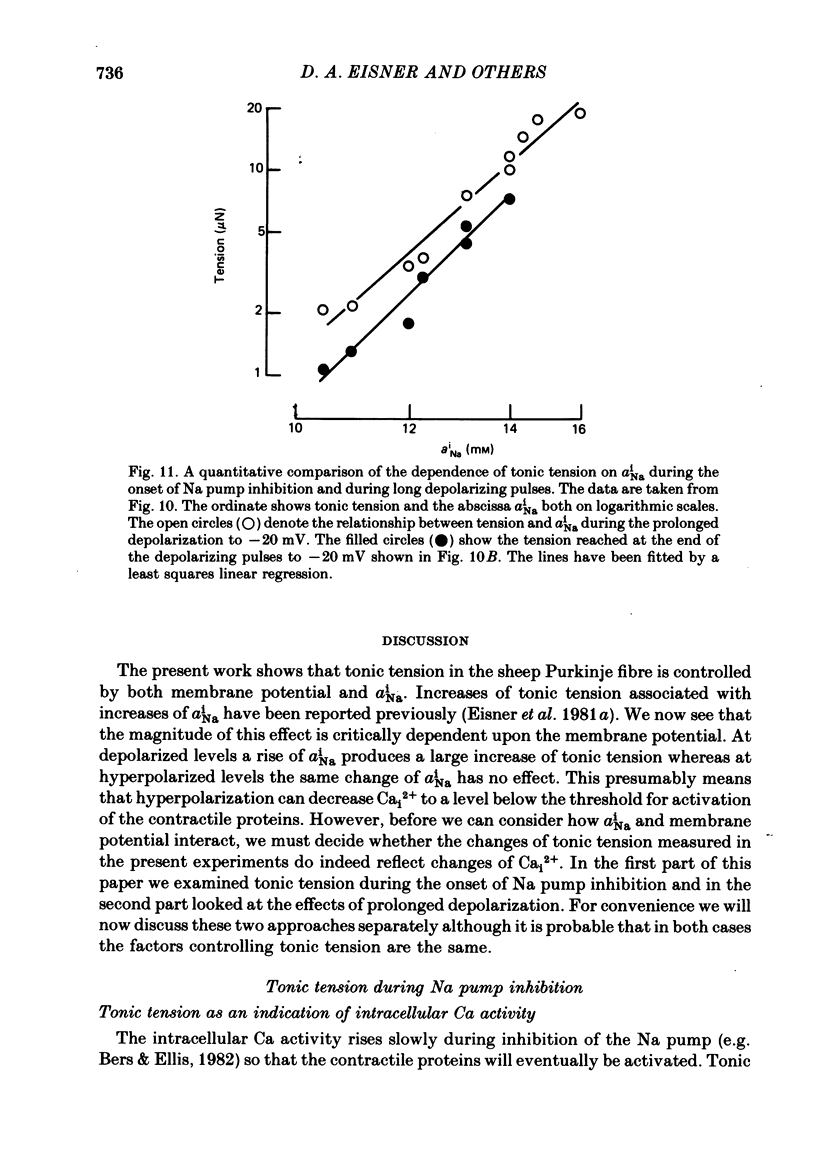
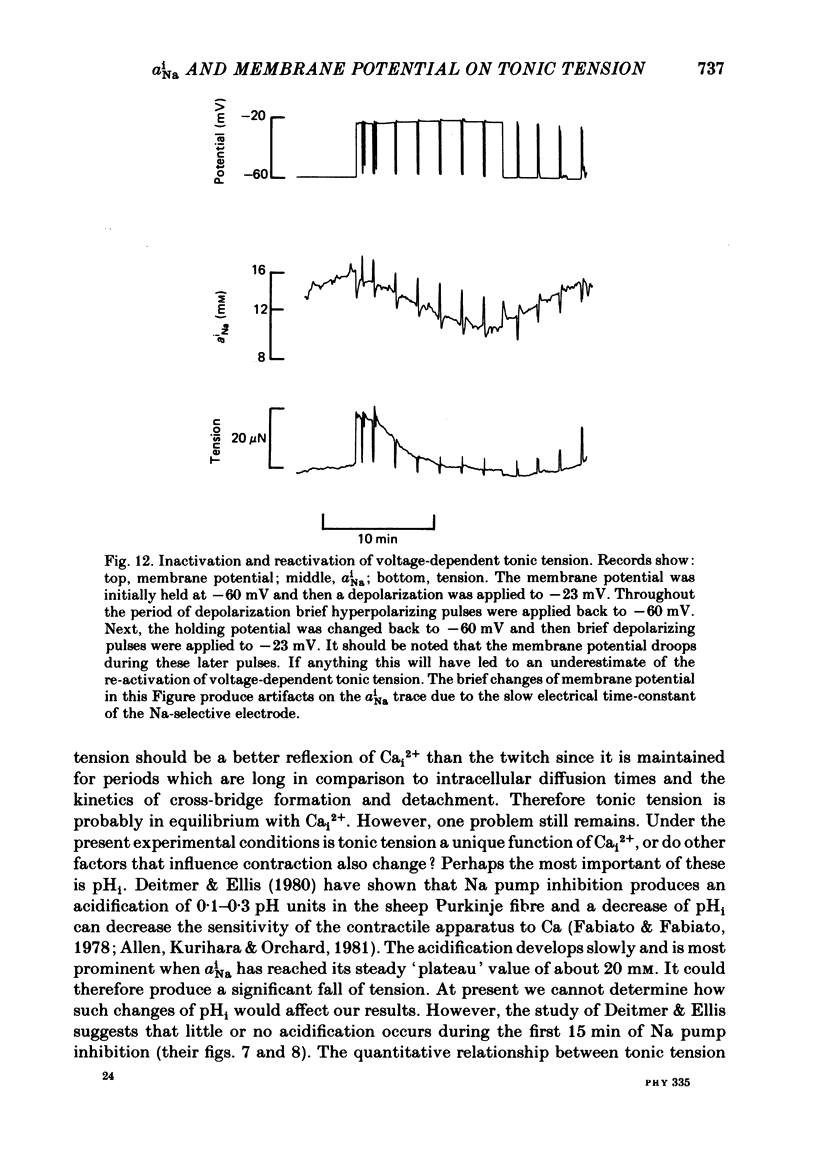
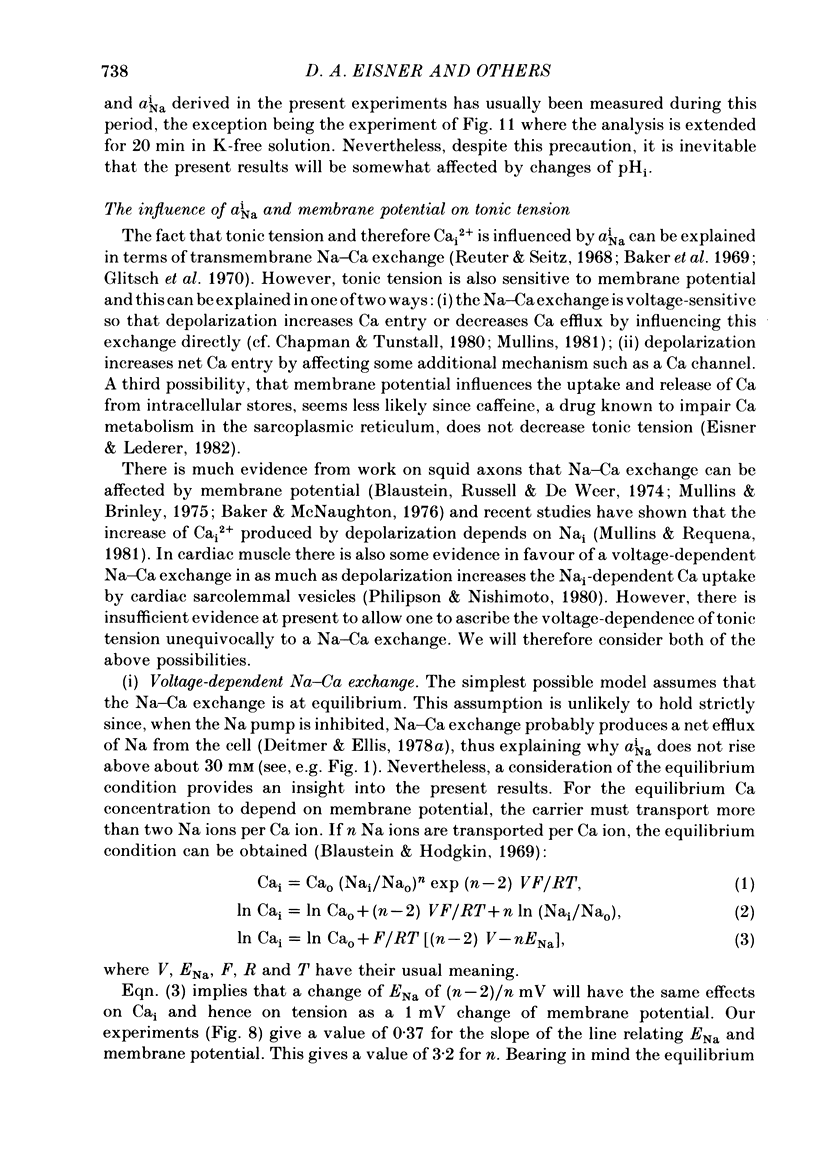
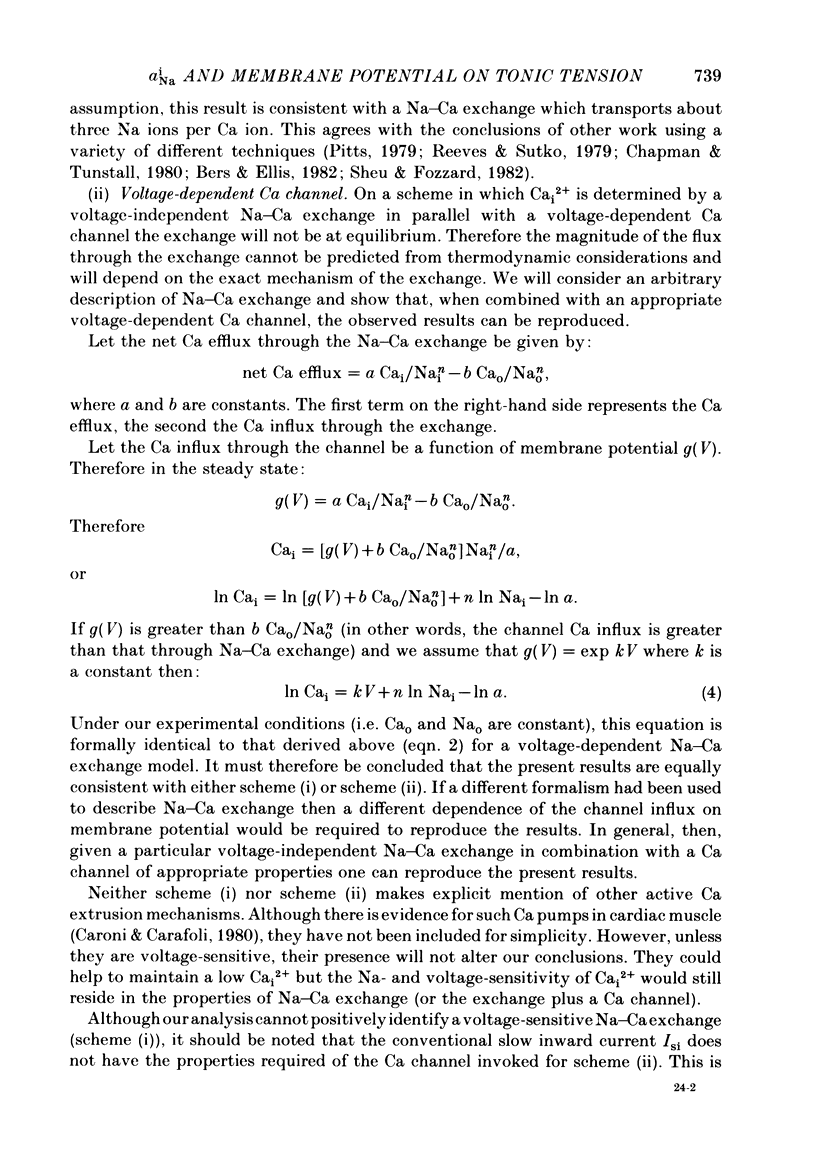
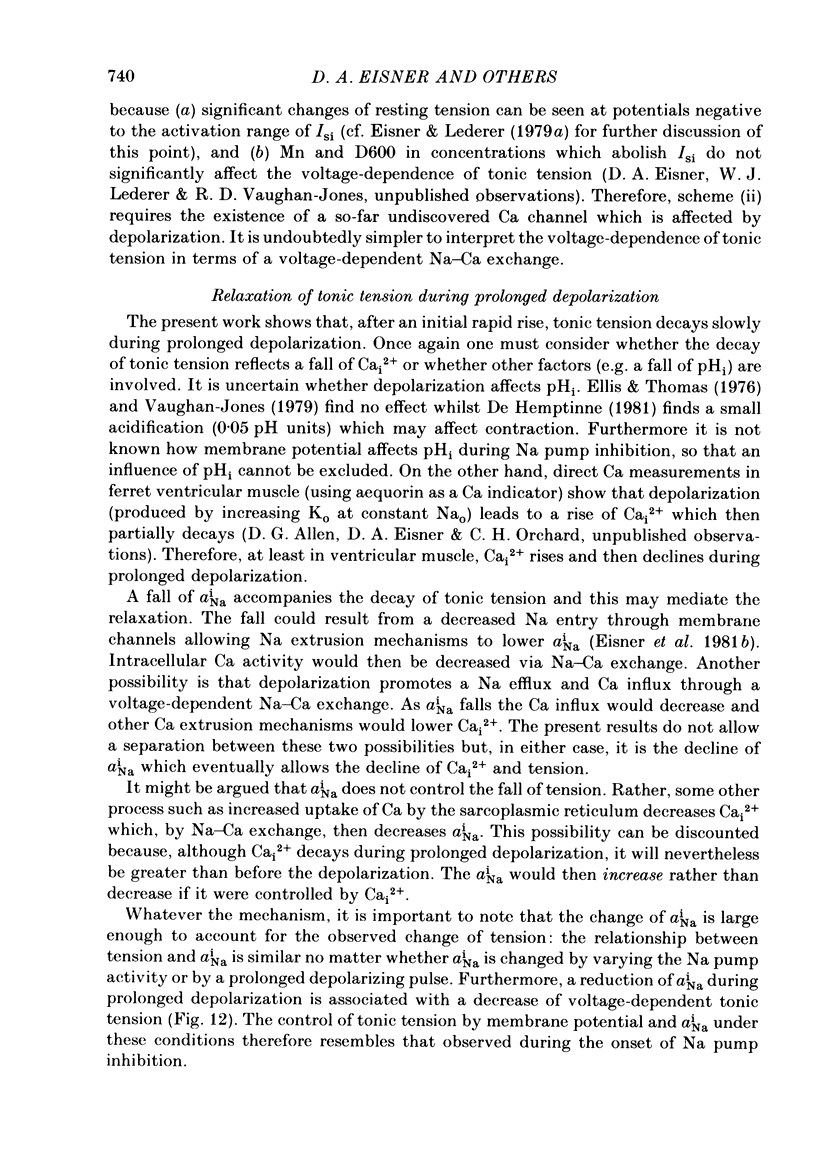
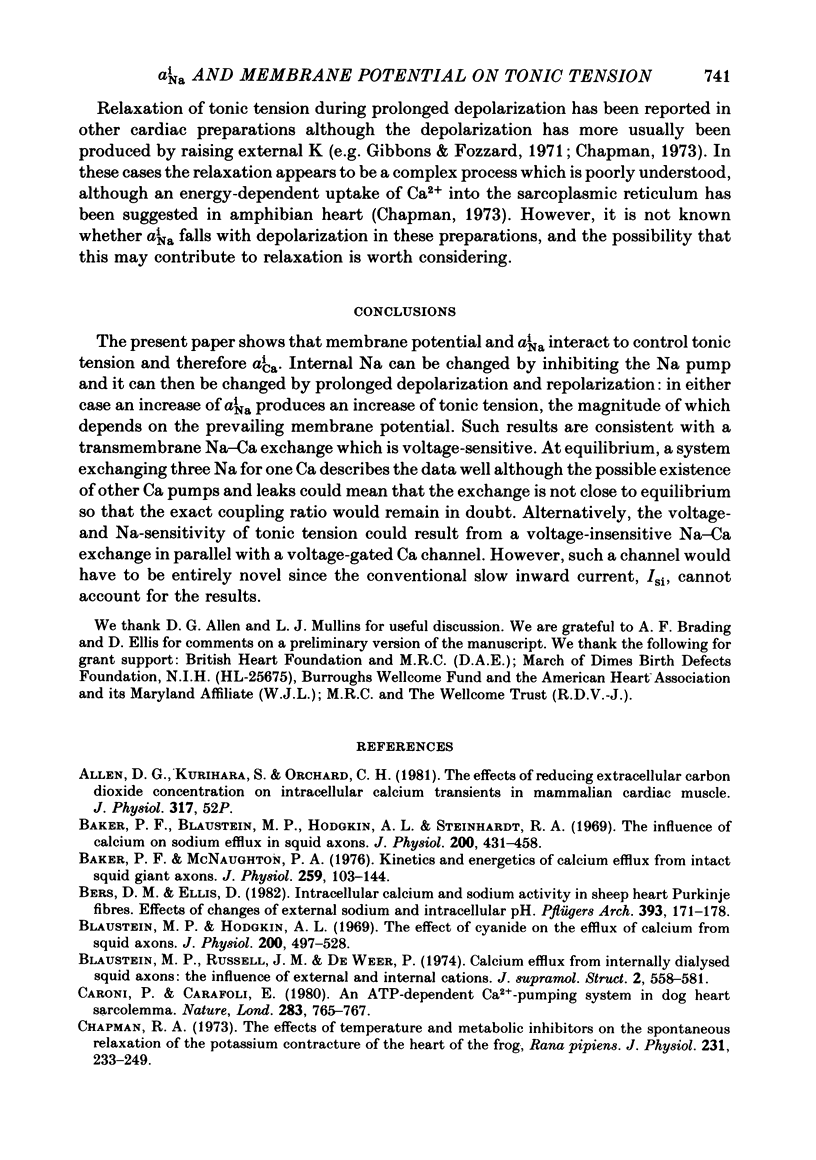
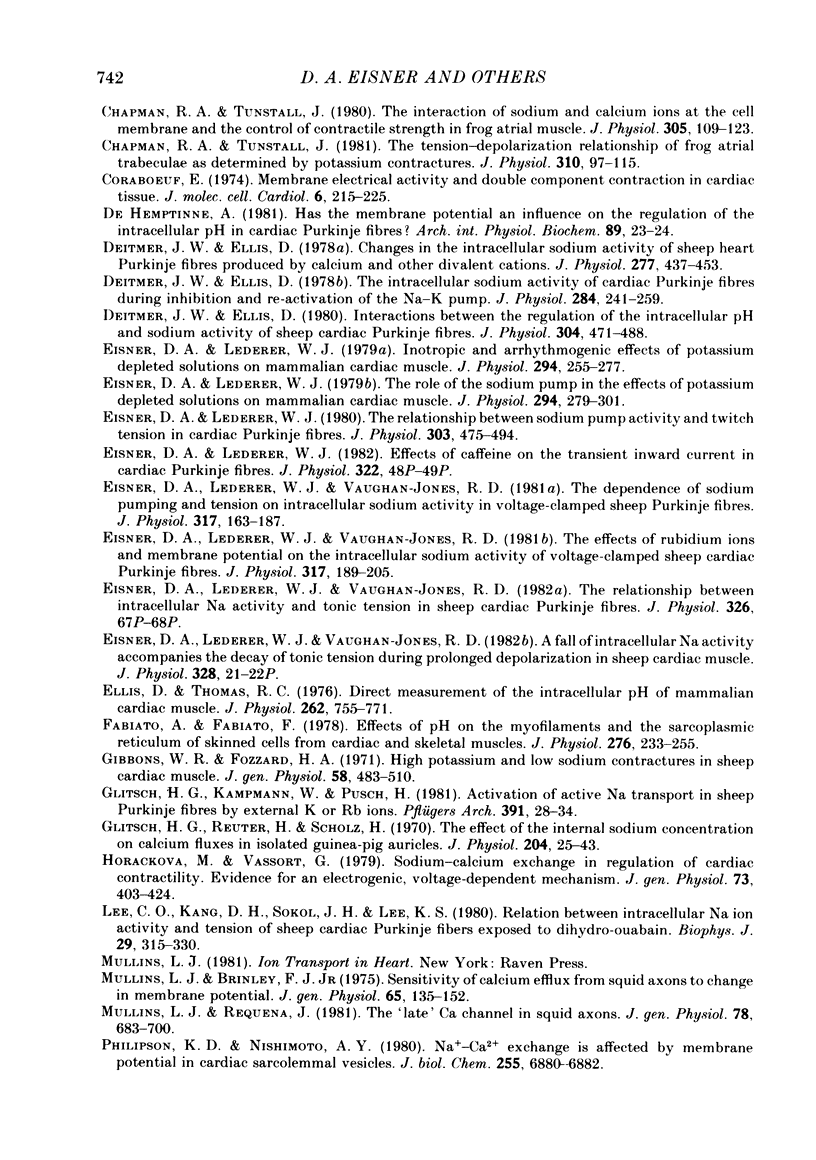
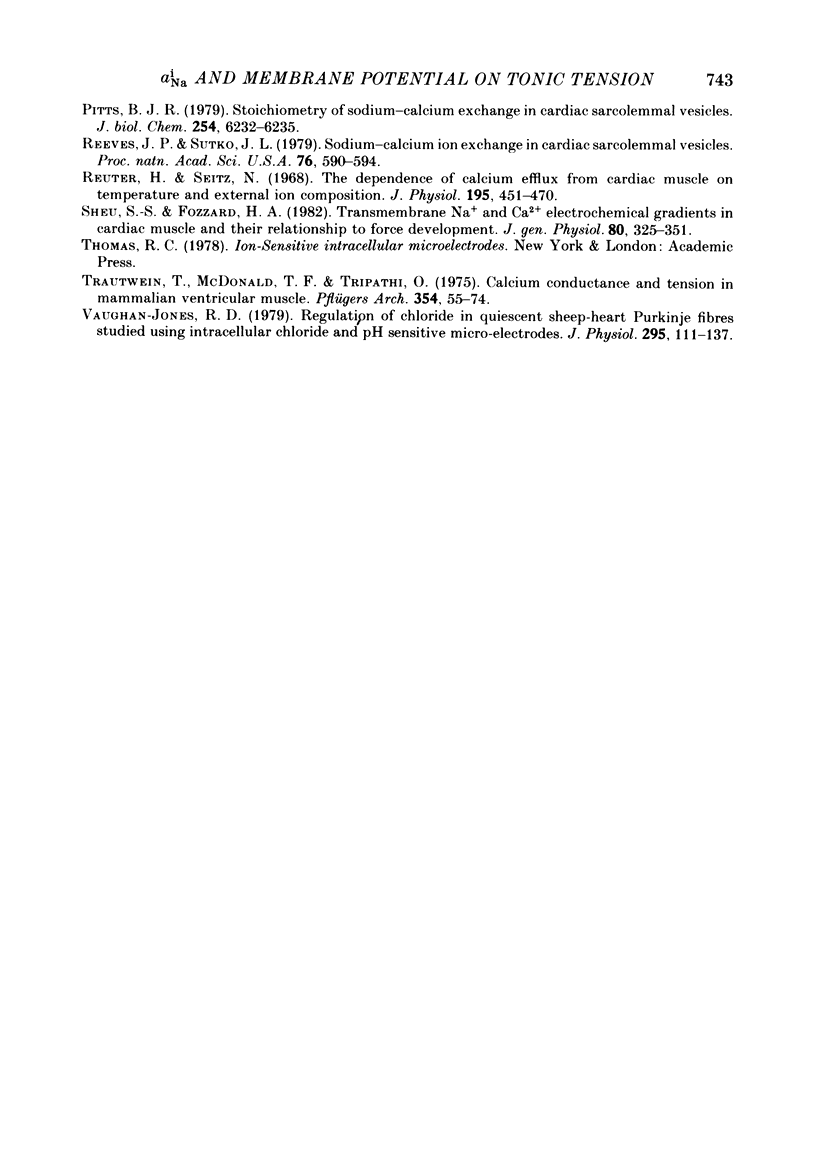
Selected References
These references are in PubMed. This may not be the complete list of references from this article.
- Baker P. F., Blaustein M. P., Hodgkin A. L., Steinhardt R. A. The influence of calcium on sodium efflux in squid axons. J Physiol. 1969 Feb;200(2):431–458. doi: 10.1113/jphysiol.1969.sp008702. [DOI] [PMC free article] [PubMed] [Google Scholar]
- Baker P. F., McNaughton P. A. Kinetics and energetics of calcium efflux from intact squid giant axons. J Physiol. 1976 Jul;259(1):103–144. doi: 10.1113/jphysiol.1976.sp011457. [DOI] [PMC free article] [PubMed] [Google Scholar]
- Bers D. M., Ellis D. Intracellular calcium and sodium activity in sheep heart Purkinje fibres. Effect of changes of external sodium and intracellular pH. Pflugers Arch. 1982 Apr;393(2):171–178. doi: 10.1007/BF00582941. [DOI] [PubMed] [Google Scholar]
- Blaustein M. P., Hodgkin A. L. The effect of cyanide on the efflux of calcium from squid axons. J Physiol. 1969 Feb;200(2):497–527. doi: 10.1113/jphysiol.1969.sp008704. [DOI] [PMC free article] [PubMed] [Google Scholar]
- Blaustein M. P., Russell J. M., Weer P. Calcium efflux from internally dialyzed squid axons: the influence of external and internal cations. J Supramol Struct. 1974;2(5-6):558–581. doi: 10.1002/jss.400020505. [DOI] [PubMed] [Google Scholar]
- Caroni P., Carafoli E. An ATP-dependent Ca2+-pumping system in dog heart sarcolemma. Nature. 1980 Feb 21;283(5749):765–767. doi: 10.1038/283765a0. [DOI] [PubMed] [Google Scholar]
- Chapman R. A. The effects of temperature and metabolic inhibitors on the spontaneous relaxation of the potassium contracture of the heart of the frog Rana pipiens. J Physiol. 1973 Jun;231(2):233–249. doi: 10.1113/jphysiol.1973.sp010230. [DOI] [PMC free article] [PubMed] [Google Scholar]
- Chapman R. A., Tunstall J. The interaction of sodium and calcium ions at the cell membrane and the control of contractile strength in frog atrial muscle. J Physiol. 1980 Aug;305:109–123. doi: 10.1113/jphysiol.1980.sp013353. [DOI] [PMC free article] [PubMed] [Google Scholar]
- Chapman R. A., Tunstall J. The tension-depolarization relationship of frog atrial trabeculae as determined by potassium contractures. J Physiol. 1981 Jan;310:97–115. doi: 10.1113/jphysiol.1981.sp013539. [DOI] [PMC free article] [PubMed] [Google Scholar]
- Coraboeuf E. Editorial: Membrane electrical activity and double component contraction in cardiac tissue. J Mol Cell Cardiol. 1974 Jun;6(3):215–225. doi: 10.1016/0022-2828(74)90051-0. [DOI] [PubMed] [Google Scholar]
- Deitmer J. W., Ellis D. Changes in the intracellular sodium activity of sheep heart Purkinje fibres produced by calcium and other divalent cations. J Physiol. 1978 Apr;277:437–453. doi: 10.1113/jphysiol.1978.sp012283. [DOI] [PMC free article] [PubMed] [Google Scholar]
- Deitmer J. W., Ellis D. Interactions between the regulation of the intracellular pH and sodium activity of sheep cardiac Purkinje fibres. J Physiol. 1980 Jul;304:471–488. doi: 10.1113/jphysiol.1980.sp013337. [DOI] [PMC free article] [PubMed] [Google Scholar]
- Deitmer J. W., Ellis D. The intracellular sodium activity of cardiac Purkinje fibres during inhibition and re-activation of the Na-K pump. J Physiol. 1978 Nov;284:241–259. doi: 10.1113/jphysiol.1978.sp012539. [DOI] [PMC free article] [PubMed] [Google Scholar]
- Eisner D. A., Lederer W. J. Inotropic and arrhythmogenic effects of potassium-depleted solutions on mammalian cardiac muscle. J Physiol. 1979 Sep;294:255–277. doi: 10.1113/jphysiol.1979.sp012929. [DOI] [PMC free article] [PubMed] [Google Scholar]
- Eisner D. A., Lederer W. J. The relationship between sodium pump activity and twitch tension in cardiac Purkinje fibres. J Physiol. 1980 Jun;303:475–494. doi: 10.1113/jphysiol.1980.sp013299. [DOI] [PMC free article] [PubMed] [Google Scholar]
- Eisner D. A., Lederer W. J. The role of the sodium pump in the effects of potassium-depleted solutions on mammalian cardiac muscle. J Physiol. 1979 Sep;294:279–301. doi: 10.1113/jphysiol.1979.sp012930. [DOI] [PMC free article] [PubMed] [Google Scholar]
- Eisner D. A., Lederer W. J., Vaughan-Jones R. D. The dependence of sodium pumping and tension on intracellular sodium activity in voltage-clamped sheep Purkinje fibres. J Physiol. 1981 Aug;317:163–187. doi: 10.1113/jphysiol.1981.sp013819. [DOI] [PMC free article] [PubMed] [Google Scholar]
- Eisner D. A., Lederer W. J., Vaughan-Jones R. D. The effects of rubidium ions and membrane potentials on the intracellular sodium activity of sheep Purkinje fibres. J Physiol. 1981 Aug;317:189–205. doi: 10.1113/jphysiol.1981.sp013820. [DOI] [PMC free article] [PubMed] [Google Scholar]
- Ellis D., Thomas R. C. Direct measurement of the intracellular pH of mammalian cardiac muscle. J Physiol. 1976 Nov;262(3):755–771. doi: 10.1113/jphysiol.1976.sp011619. [DOI] [PMC free article] [PubMed] [Google Scholar]
- Fabiato A., Fabiato F. Effects of pH on the myofilaments and the sarcoplasmic reticulum of skinned cells from cardiace and skeletal muscles. J Physiol. 1978 Mar;276:233–255. doi: 10.1113/jphysiol.1978.sp012231. [DOI] [PMC free article] [PubMed] [Google Scholar]
- Gibbons W. R., Fozzard H. A. High potassium and low sodium contractures in sheep cardiac muscle. J Gen Physiol. 1971 Nov;58(5):483–510. doi: 10.1085/jgp.58.5.483. [DOI] [PMC free article] [PubMed] [Google Scholar]
- Glitsch H. G., Kampmann W., Pusch H. Activation of active Na transport in sheep Purkinje fibres by external K or Rb ions. Pflugers Arch. 1981 Jul;391(1):28–34. doi: 10.1007/BF00580690. [DOI] [PubMed] [Google Scholar]
- Glitsch H. G., Reuter H., Scholz H. The effect of the internal sodium concentration on calcium fluxes in isolated guinea-pig auricles. J Physiol. 1970 Jul;209(1):25–43. doi: 10.1113/jphysiol.1970.sp009153. [DOI] [PMC free article] [PubMed] [Google Scholar]
- Horackova M., Vassort G. Sodium-calcium exchange in regulation of cardiac contractility. Evidence for an electrogenic, voltage-dependent mechanism. J Gen Physiol. 1979 Apr;73(4):403–424. doi: 10.1085/jgp.73.4.403. [DOI] [PMC free article] [PubMed] [Google Scholar]
- Lee C. O., Kang D. H., Sokol J. H., Lee K. S. Relation between intracellular Na ion activity and tension of sheep cardiac Purkinje fibers exposed to dihydro-ouabain. Biophys J. 1980 Feb;29(2):315–330. doi: 10.1016/S0006-3495(80)85135-6. [DOI] [PMC free article] [PubMed] [Google Scholar]
- Mullins L. J., Brinley F. J., Jr Sensitivity of calcium efflux from squid axons to changes in membrane potential. J Gen Physiol. 1975 Feb;65(2):135–152. doi: 10.1085/jgp.65.2.135. [DOI] [PMC free article] [PubMed] [Google Scholar]
- Mullins L. J., Requena J. The "late" Ca channel in squid axons. J Gen Physiol. 1981 Dec;78(6):683–700. doi: 10.1085/jgp.78.6.683. [DOI] [PMC free article] [PubMed] [Google Scholar]
- Philipson K. D., Nishimoto A. Y. Na+-Ca2+ exchange is affected by membrane potential in cardiac sarcolemmal vesicles. J Biol Chem. 1980 Jul 25;255(14):6880–6882. [PubMed] [Google Scholar]
- Pitts B. J. Stoichiometry of sodium-calcium exchange in cardiac sarcolemmal vesicles. Coupling to the sodium pump. J Biol Chem. 1979 Jul 25;254(14):6232–6235. [PubMed] [Google Scholar]
- Reeves J. P., Sutko J. L. Sodium-calcium ion exchange in cardiac membrane vesicles. Proc Natl Acad Sci U S A. 1979 Feb;76(2):590–594. doi: 10.1073/pnas.76.2.590. [DOI] [PMC free article] [PubMed] [Google Scholar]
- Reuter H., Seitz N. The dependence of calcium efflux from cardiac muscle on temperature and external ion composition. J Physiol. 1968 Mar;195(2):451–470. doi: 10.1113/jphysiol.1968.sp008467. [DOI] [PMC free article] [PubMed] [Google Scholar]
- Sheu S. S., Fozzard H. A. Transmembrane Na+ and Ca2+ electrochemical gradients in cardiac muscle and their relationship to force development. J Gen Physiol. 1982 Sep;80(3):325–351. doi: 10.1085/jgp.80.3.325. [DOI] [PMC free article] [PubMed] [Google Scholar]
- Trautwein W., McDonald T. F., Tripathi O. Calcium conductance and tension in mammalian ventricular muscle. Pflugers Arch. 1975;354(1):55–74. doi: 10.1007/BF00584503. [DOI] [PubMed] [Google Scholar]
- Vaughan-Jones R. D. Regulation of chloride in quiescent sheep-heart Purkinje fibres studied using intracellular chloride and pH-sensitive micro-electrodes. J Physiol. 1979 Oct;295:111–137. doi: 10.1113/jphysiol.1979.sp012957. [DOI] [PMC free article] [PubMed] [Google Scholar]