Abstract
1. The type of metabolism adopted by Pseudomonas oxalaticus during growth on a variety of carbon sources was studied. 2. The only substrate upon which autotrophic growth was observed is formate. 3. In mixtures of formate and those substrates upon which the organism can grow faster than on formate, e.g. succinate, lactate or citrate, heterotrophic metabolism results. 4. In mixtures of formate and those substrates upon which the organism can grow at a similar rate to that on formate, e.g. glycollate or glyoxylate, the predominant mode of metabolism adopted is heterotrophic utilization of the C2 substrate coupled with oxidation of formate as ancillary energy source. 5. P. oxalaticus grows on oxalate 30% slower than on formate. In mixtures of formate and oxalate, the predominant mode of metabolism adopted is autotrophic utilization of formate coupled with oxidation of oxalate as ancillary energy source. 6. In mixtures of formate and those substrates upon which the organism grows at a much lower rate than on formate, e.g. glycerol and malonate, the predominant mode of metabolism adopted is autotrophic utilization of formate. 7. It is concluded that synthesis of the enzymes involved in autotrophic metabolism is controlled by a combination of induction and metabolite repression.
Full text
PDF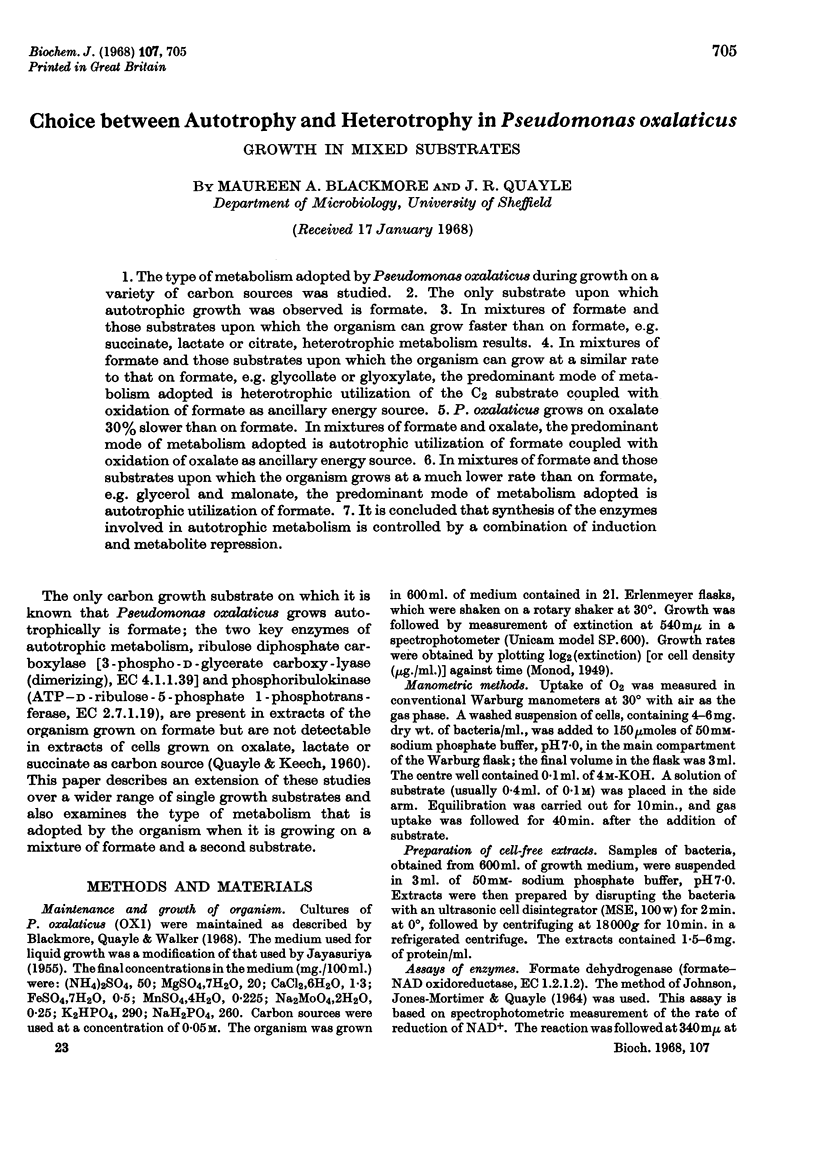
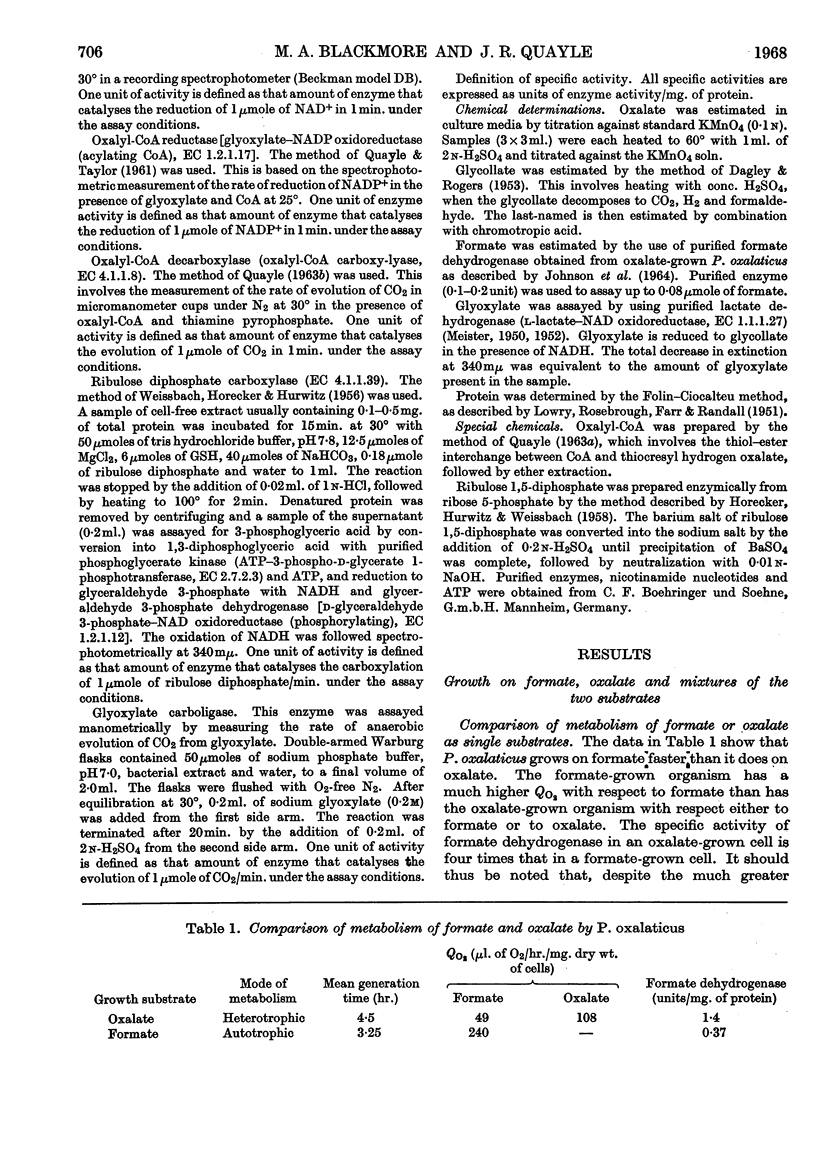
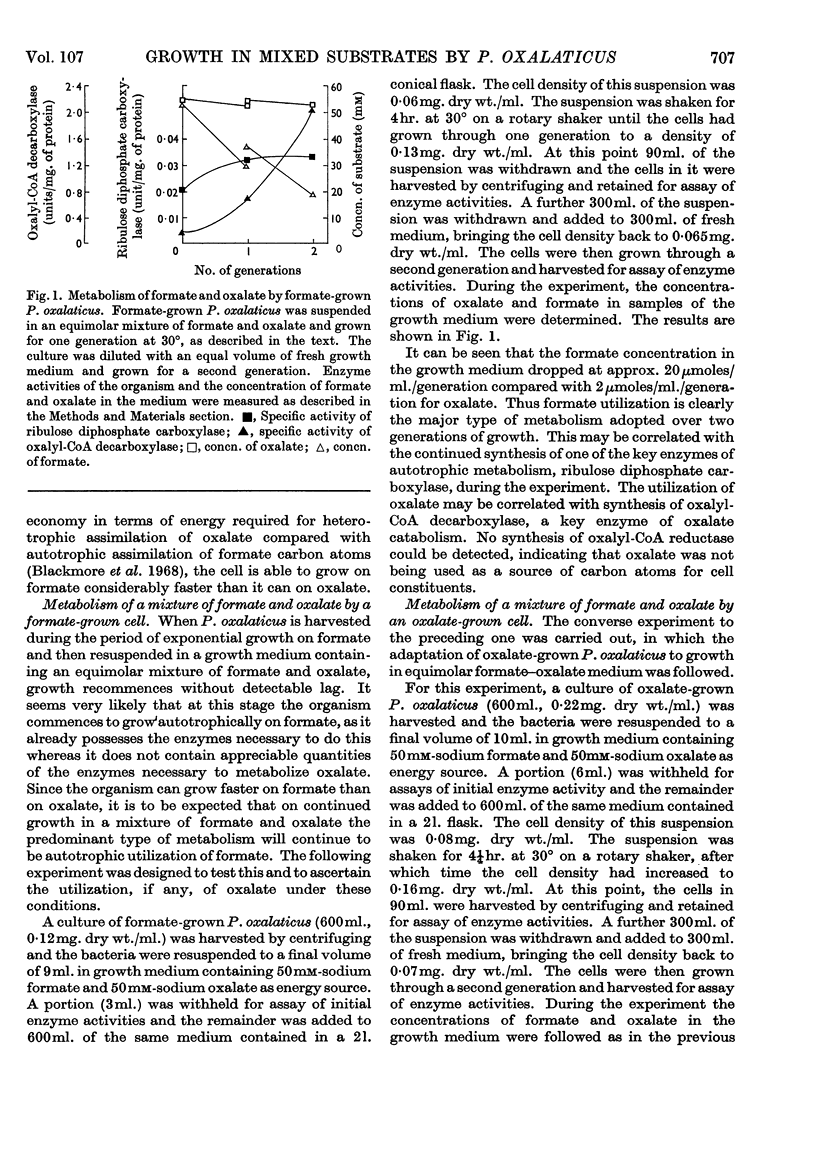
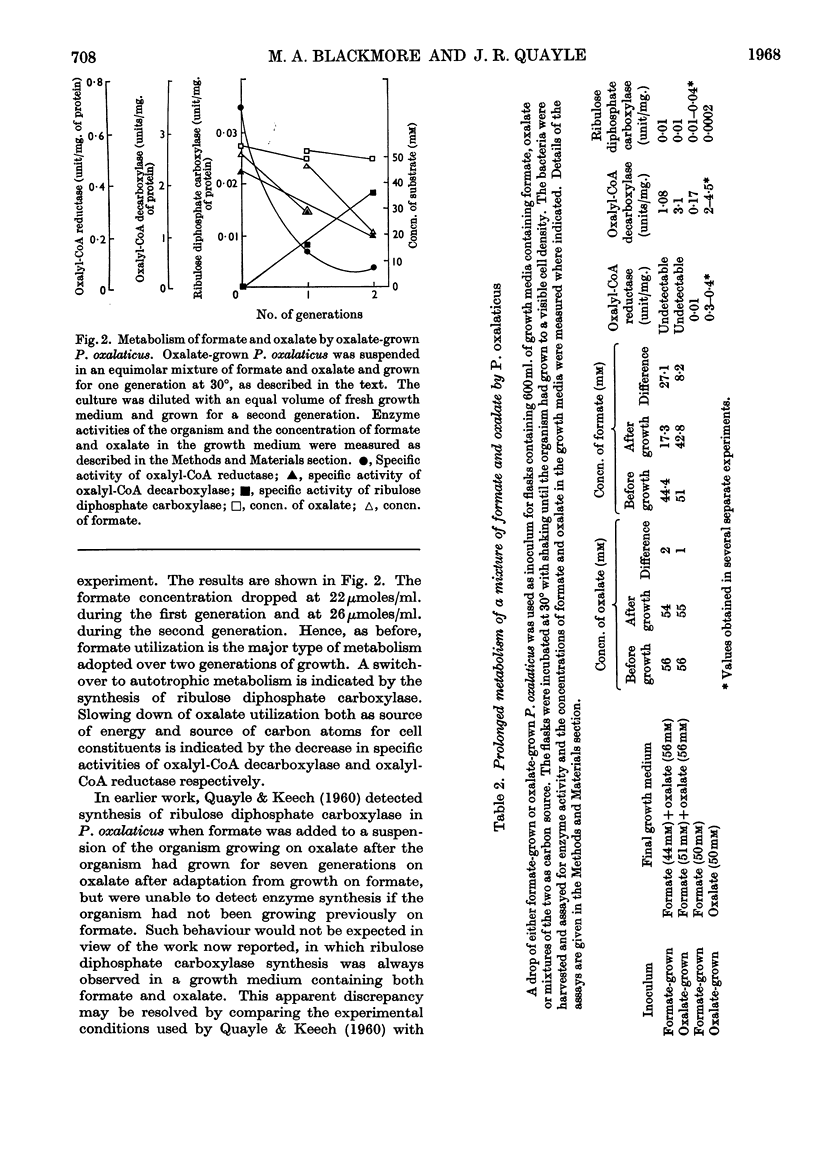
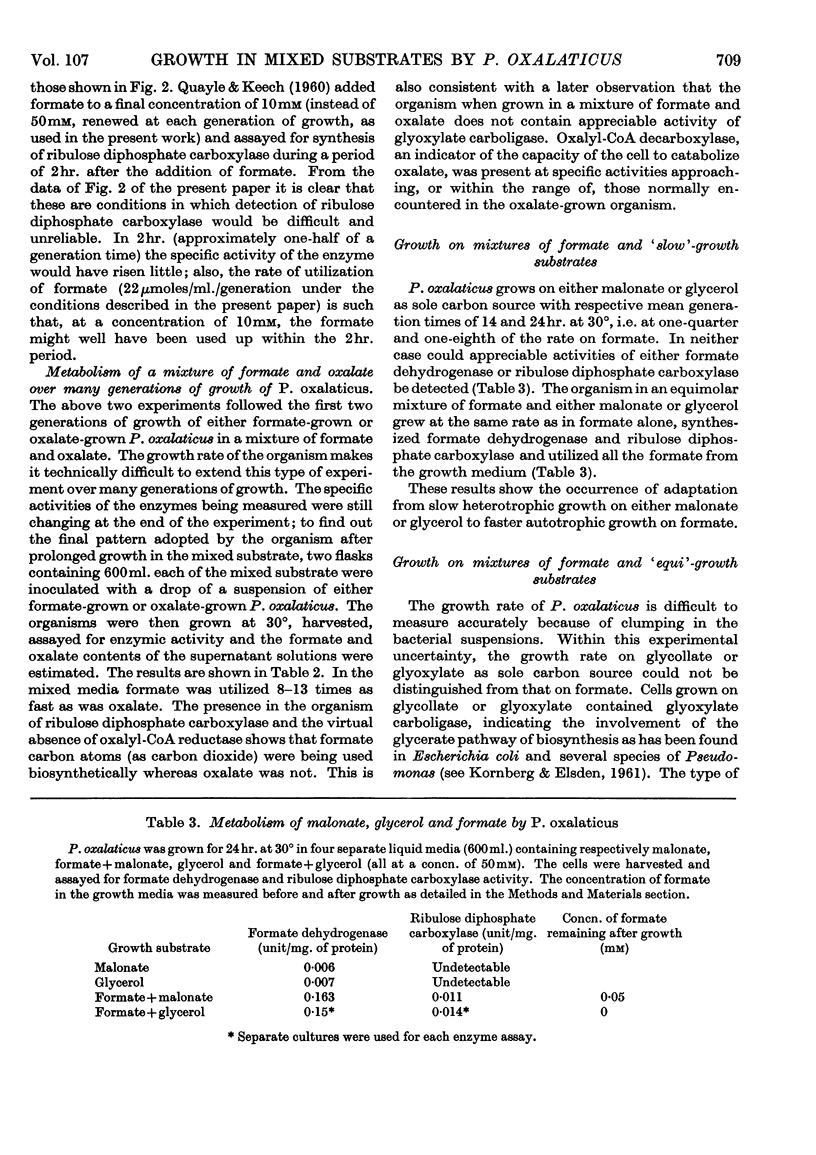
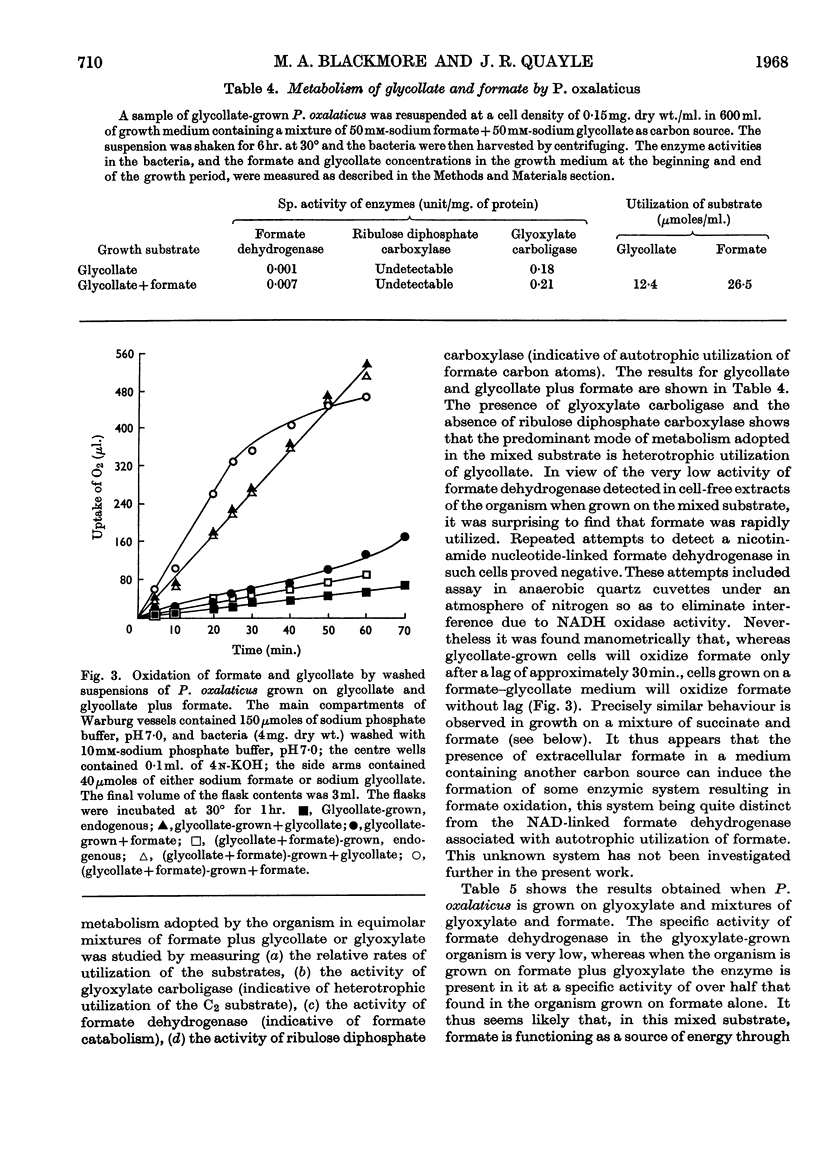
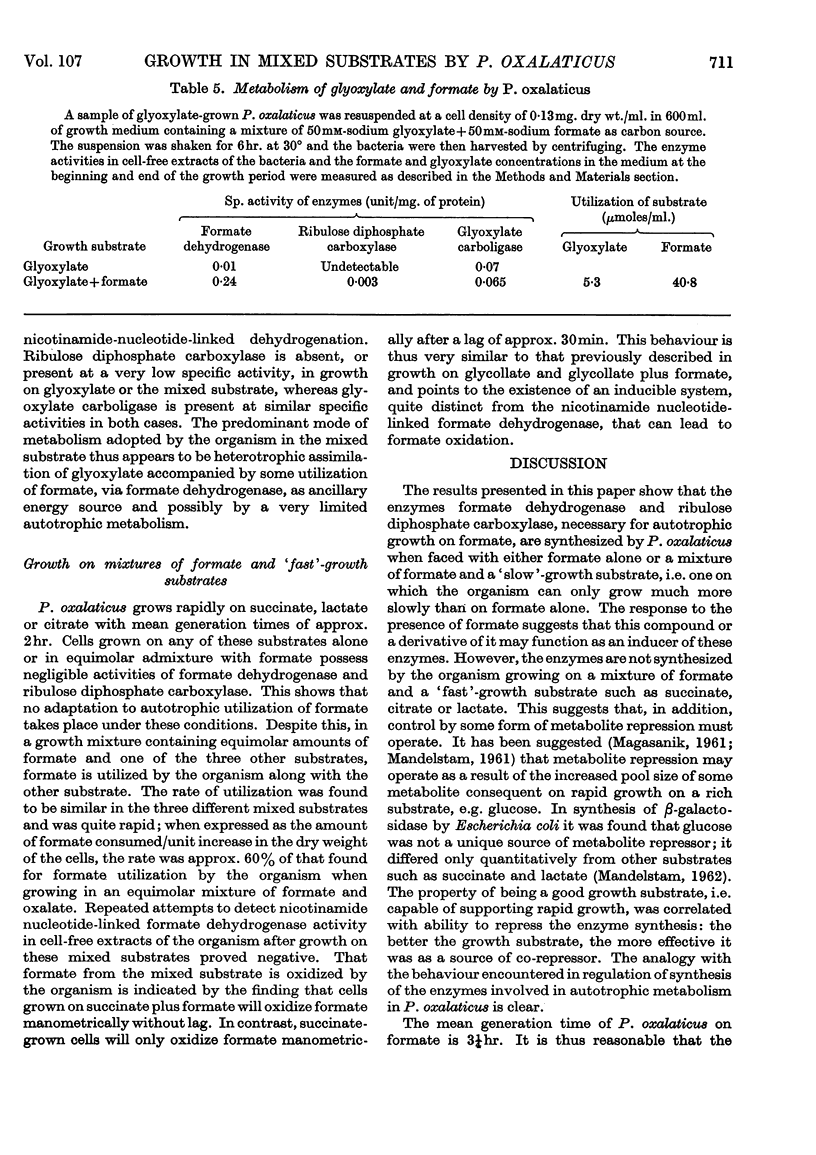
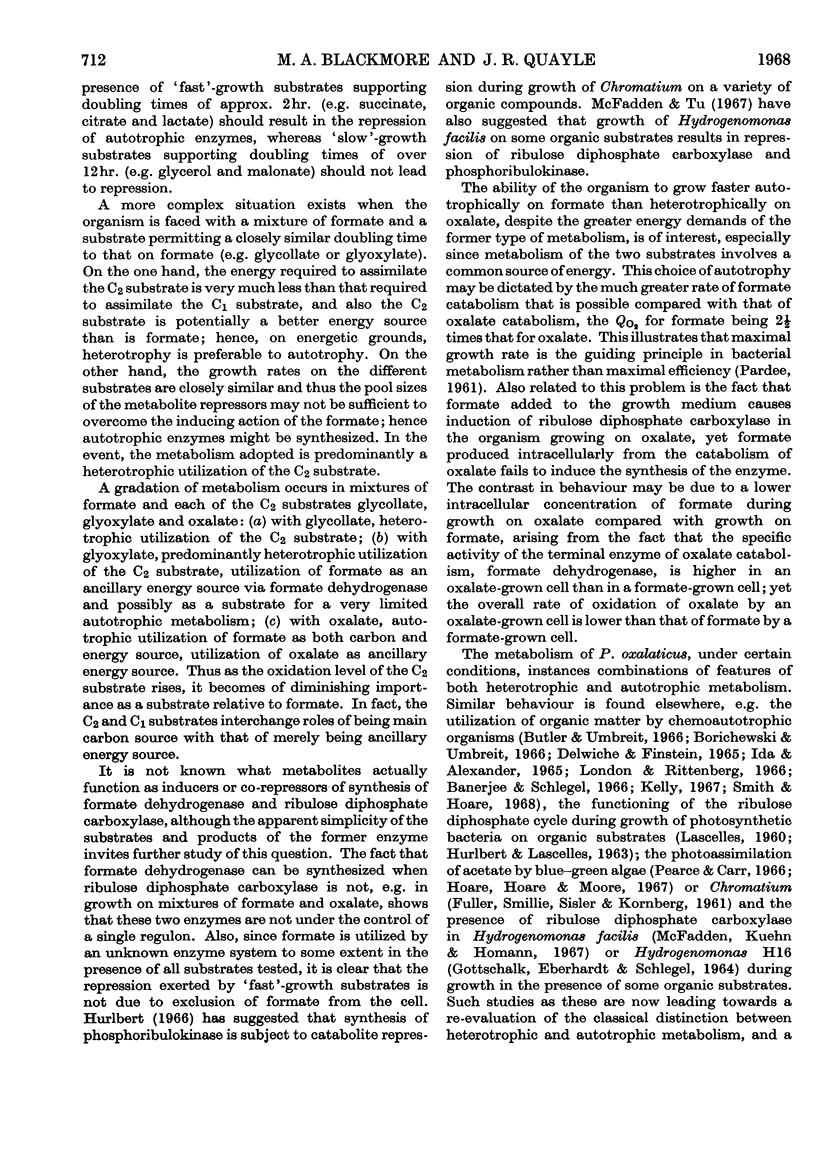
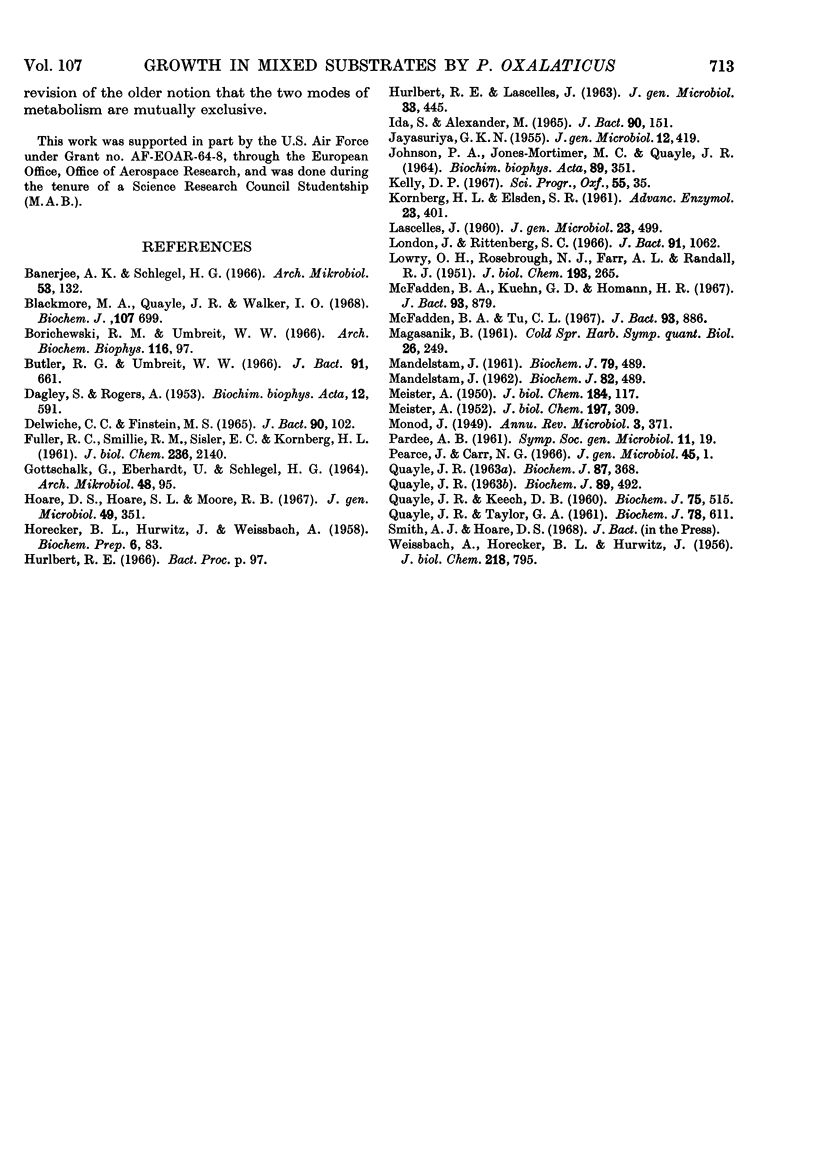
Selected References
These references are in PubMed. This may not be the complete list of references from this article.
- Banerjee A. K., Schlegel H. G. Zur Rolle des Hefeextraktes während des chemolithotrophen Wachstums von Micrococcus denitrificans. Arch Mikrobiol. 1966 Mar 8;53(2):132–153. [PubMed] [Google Scholar]
- Blackmore M. A., Quayle J. R., Walker I. O. Choice between autotrophy and heterotrophy in Pseudomonas oxalaticus. Utilization of oxalate by cells after adaptation from growth on formate to growth on oxalate. Biochem J. 1968 May;107(5):699–704. doi: 10.1042/bj1070699. [DOI] [PMC free article] [PubMed] [Google Scholar]
- Borichewski R. M., Umbreit W. W. Growth of Thiobacillus thiooxidans on glucose. Arch Biochem Biophys. 1966 Sep 26;116(1):97–102. doi: 10.1016/0003-9861(66)90017-8. [DOI] [PubMed] [Google Scholar]
- Butler R. G., Umbreit W. W. Absorption and utilization of organic matter by the strict autotroph, Thiobacillus thiooxidans, with special reference to aspartic acid. J Bacteriol. 1966 Feb;91(2):661–666. doi: 10.1128/jb.91.2.661-666.1966. [DOI] [PMC free article] [PubMed] [Google Scholar]
- DAGLEY S., RODGERS A. Estimation of glycollic acid. Biochim Biophys Acta. 1953 Dec;12(4):591–591. doi: 10.1016/0006-3002(53)90196-6. [DOI] [PubMed] [Google Scholar]
- Delwiche C. C., Finstein M. S. Carbon and Energy Sources for the Nitrifying Autotroph Nitrobacter. J Bacteriol. 1965 Jul;90(1):102–107. doi: 10.1128/jb.90.1.102-107.1965. [DOI] [PMC free article] [PubMed] [Google Scholar]
- FULLER R. C., SMILLIE R. M., SISLER E. C., KORNBERG H. L. Carbon metabolism in Chromatium. J Biol Chem. 1961 Jul;236:2140–2149. [PubMed] [Google Scholar]
- GOTTSCHALK G., EBERHARDT U., SCHLEGEL H. G. VERWERTUNG VON FRUCTOSE DURCH HYDROGENOMONAS H 16. (I.) Arch Mikrobiol. 1964 Apr 2;48:95–108. [PubMed] [Google Scholar]
- HURLBERT R. E., LASCELLES J. RIBULOSE DIPHOSPHATE CARBOXYLASE IN THIORHODACEAE. J Gen Microbiol. 1963 Dec;33:445–458. doi: 10.1099/00221287-33-3-445. [DOI] [PubMed] [Google Scholar]
- Ida S., Alexander M. Permeability of Nitrobacter agilis to Organic Compounds. J Bacteriol. 1965 Jul;90(1):151–156. doi: 10.1128/jb.90.1.151-156.1965. [DOI] [PMC free article] [PubMed] [Google Scholar]
- JAYASURIYA G. C. The isolation and characteristics of an oxalate-decomposing organism. J Gen Microbiol. 1955 Jun;12(3):419–428. doi: 10.1099/00221287-12-3-419. [DOI] [PubMed] [Google Scholar]
- JOHNSON P. A., JONES-MORTIMER M. C., QUAYLE J. R. USE OF A PURIFIED BACTERIAL FORMATE DEHYDROGENASE FOR THE MICRO-ESTIMATION OF FORMATE. Biochim Biophys Acta. 1964 Aug 26;89:351–353. doi: 10.1016/0926-6569(64)90225-1. [DOI] [PubMed] [Google Scholar]
- KORNBERG H. L., ELSDEN S. R. The metabolism of 2-carbon compounds by microorganisms. Adv Enzymol Relat Subj Biochem. 1961;23:401–470. doi: 10.1002/9780470122686.ch8. [DOI] [PubMed] [Google Scholar]
- LASCELLES J. The formation of ribulose 1:5-diphosphate carboxylase by growing cultures of Athiorhodaceae. J Gen Microbiol. 1960 Dec;23:499–510. doi: 10.1099/00221287-23-3-499. [DOI] [PubMed] [Google Scholar]
- LOWRY O. H., ROSEBROUGH N. J., FARR A. L., RANDALL R. J. Protein measurement with the Folin phenol reagent. J Biol Chem. 1951 Nov;193(1):265–275. [PubMed] [Google Scholar]
- London J., Rittenberg S. C. Effects of organic matter on the growth of Thiobacillus intermedius. J Bacteriol. 1966 Mar;91(3):1062–1069. doi: 10.1128/jb.91.3.1062-1069.1966. [DOI] [PMC free article] [PubMed] [Google Scholar]
- MAGASANIK B. Catabolite repression. Cold Spring Harb Symp Quant Biol. 1961;26:249–256. doi: 10.1101/sqb.1961.026.01.031. [DOI] [PubMed] [Google Scholar]
- MANDELSTAM J. Induction and repression of beta-galactosidase in non-growing Escherichia coli. Biochem J. 1961 Jun;79:489–496. doi: 10.1042/bj0790489. [DOI] [PMC free article] [PubMed] [Google Scholar]
- MANDELSTAM J. The repression of constitutive beta-galactosidase in Escherichia coli by glucose and other carbon sources. Biochem J. 1962 Mar;82:489–493. doi: 10.1042/bj0820489. [DOI] [PMC free article] [PubMed] [Google Scholar]
- MEISTER A. Enzymatic preparation of alpha-keto acids. J Biol Chem. 1952 May;197(1):309–317. [PubMed] [Google Scholar]
- MEISTER A. Reduction of alpha gamma-diketo and alpha-keto acids catalyzed by muscle preparations and by crystalline lactic dehydrogenase. J Biol Chem. 1950 May;184(1):117–129. [PubMed] [Google Scholar]
- McFadden B. A., Kuehn G. D., Homann H. R. CO(2) Fixation, Glutamate Labeling, and the Krebs Cycle in Ribose-grown Hydrogenomonas facilis. J Bacteriol. 1967 Mar;93(3):879–885. doi: 10.1128/jb.93.3.879-885.1967. [DOI] [PMC free article] [PubMed] [Google Scholar]
- McFadden B. A., Tu C. C. Regulation of autotrophic and heterotrophic carbon dioxide fixation in Hydrogenomonas facilis. J Bacteriol. 1967 Mar;93(3):886–893. doi: 10.1128/jb.93.3.886-893.1967. [DOI] [PMC free article] [PubMed] [Google Scholar]
- QUAYLE J. R. CARBON ASSIMILATION BY PSEUDOMONAS OXALATICUS (OX1). 7. DECARBOXYLATION OF OXALYL-COENZYME A TO FORMYL-COENZYME A. Biochem J. 1963 Dec;89:492–503. doi: 10.1042/bj0890492. [DOI] [PMC free article] [PubMed] [Google Scholar]
- QUAYLE J. R. Carbon assimilation by Pseudomonas oxalaticus (OX1). 6. Reactions of oxalyl-coenzyme A. Biochem J. 1963 May;87:368–373. doi: 10.1042/bj0870368. [DOI] [PMC free article] [PubMed] [Google Scholar]
- QUAYLE J. R., KEECH D. B. Carbon assimilation by Pseudomonas oxalaticus (OX1). 343. Oxalate utilization during growth on oxalate. Biochem J. 1960 Jun;75:515–523. doi: 10.1042/bj0750515. [DOI] [PMC free article] [PubMed] [Google Scholar]
- QUAYLE J. R., TAYLOR G. A. Carbon assimilation by Pseudomonas oxalaticus (OXI). 5. Purification and properties of glyoxylic dehydrogenase. Biochem J. 1961 Mar;78:611–615. doi: 10.1042/bj0780611. [DOI] [PMC free article] [PubMed] [Google Scholar]
- WEISSBACH A., HORECKER B. L., HURWITZ J. The enzymatic formation of phosphoglyceric acid from ribulose diphosphate and carbon dioxide. J Biol Chem. 1956 Feb;218(2):795–810. [PubMed] [Google Scholar]