Abstract
The increase in K+ conductance induced by repetitive stimulation in metabolically poisoned sartorius muscle fibres of the frog was investigated, using a two-micro-electrode voltage-clamp technique. After the inhibition of creatine kinase by 0.4 mM-1-fluoro-2,4-dinitrobenzene (FDNB) and a complete and irreversible exhaustion of contractility, a nearly linear current-voltage relation was measured between -100 and 0 mV. In the presence of CN- (4 mM) an 'intermediate state' could be established by repetitive stimulation towards complete mechanical exhaustion. In this labile state, the high and potential-independent K+ conductance could be induced by repetitive voltage-clamp pulses (100 ms duration) from -85 to 0 mV membrane potential. After the pulses had ceased, fibres regained their original membrane conductance within several minutes. After the electrophoretic injection of the Ca2+-chelating agent H2EGTA2- into fibres in the intermediate state, an increase in membrane conductance by repetitive voltage-clamp pulses could no longer be induced. Fibres in the intermediate state into which H2EGTA2- -buffered Ca2+ (free Ca2+ approximately 10(-5) M) was injected, or to which external caffeine (1.5 mM) was applied, showed a spontaneous and reversible increase in membrane conductance. In metabolically poisoned and mechanically exhausted sartorius muscles the concentrations of creatine phosphate (CP) and ATP were estimated using biochemical standard methods. The concentration of CP remained basically unchanged after FDNB poisoning. In solutions containing CN- plus iodoacetate CP fell below the detectable concentration of about 0.5-1% of the normal value. ATP decreased to slightly less than 20% under both conditions. It is concluded that internal free Ca2+ promotes the activation of the K+ conductance in exhausted muscle fibres, and that a shortage of energy reserves increases the 'sensitivity' of K+ channels to Ca2+ ions.
Full text
PDF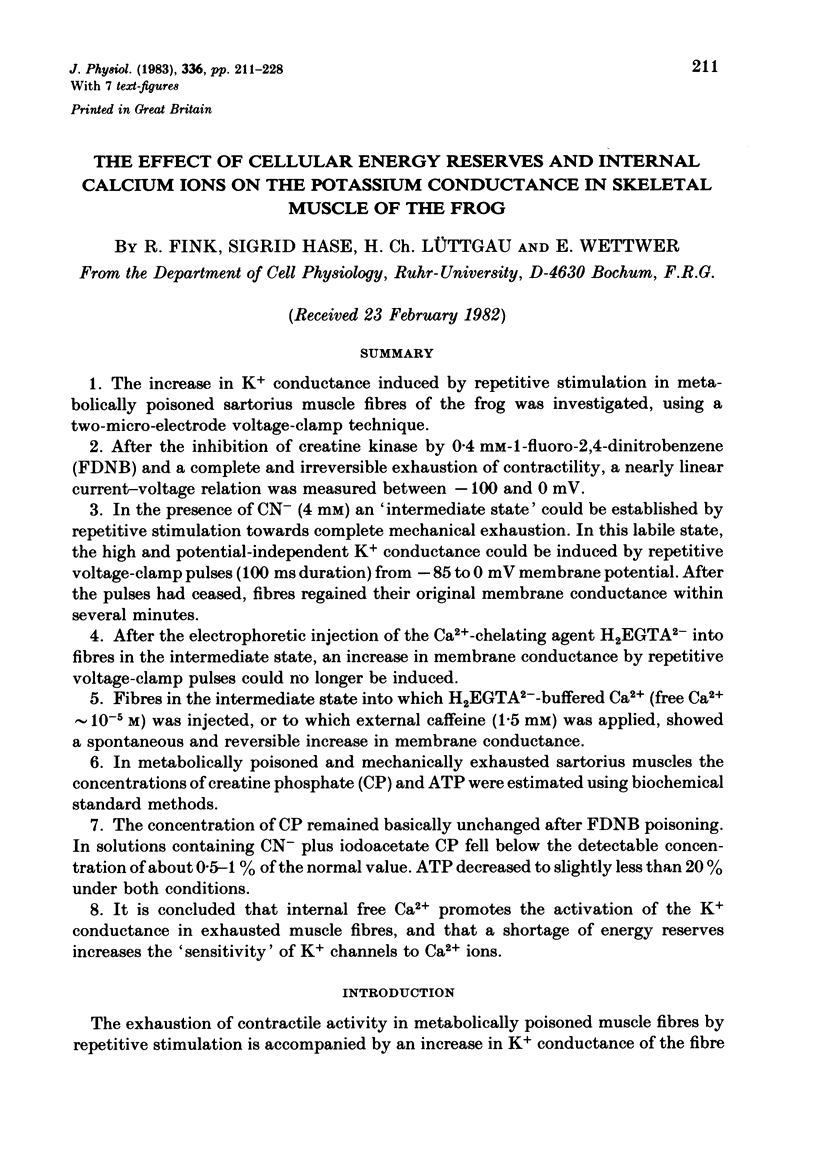
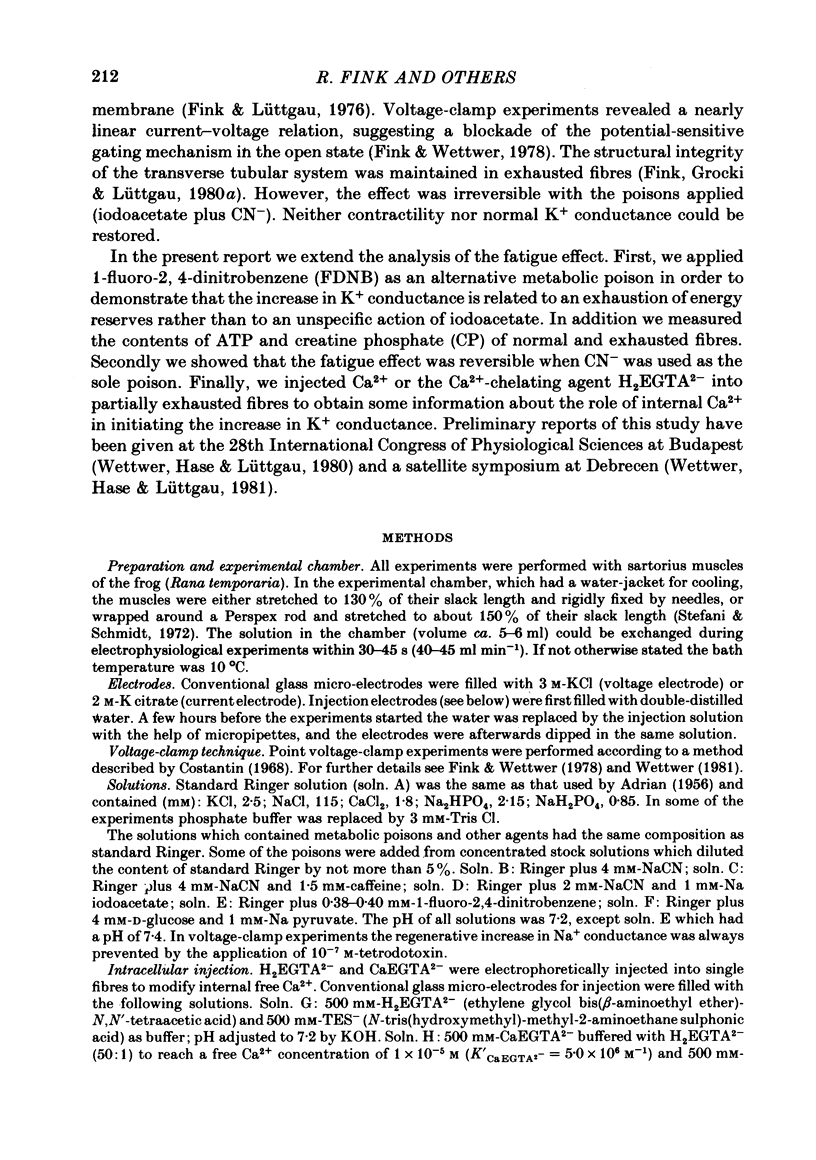
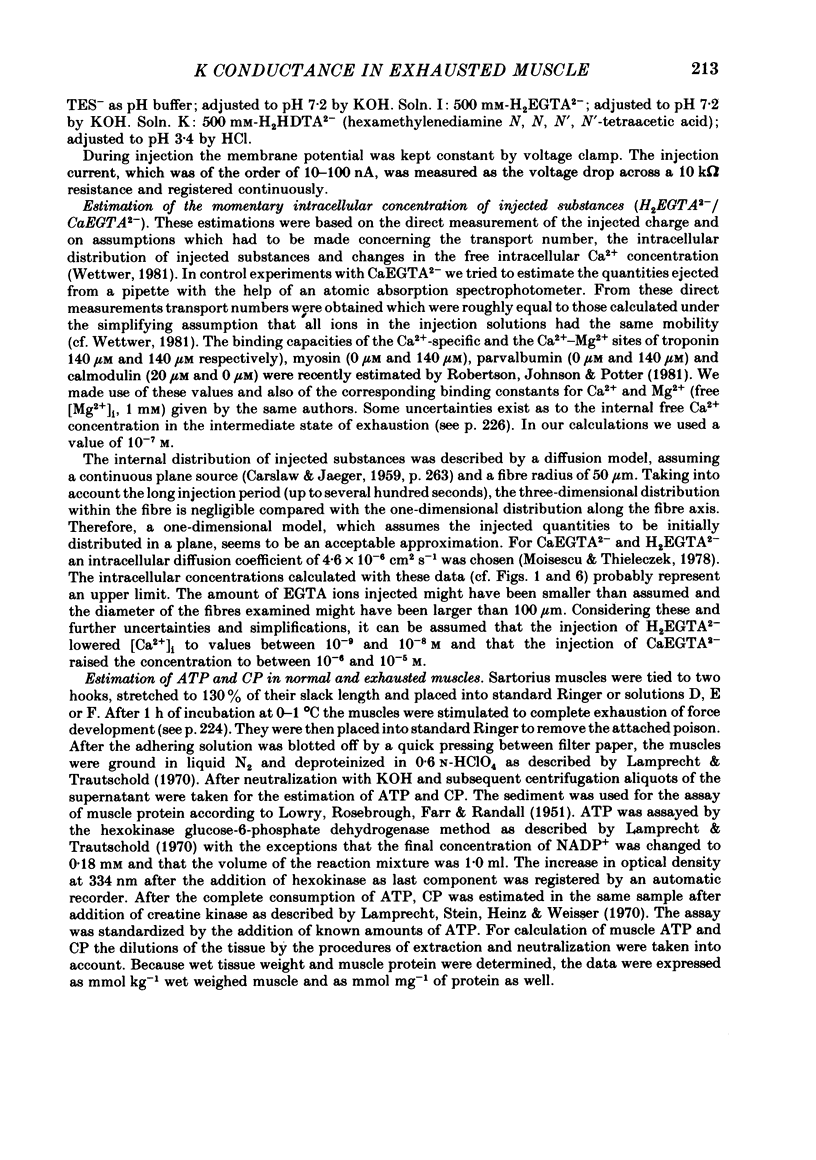
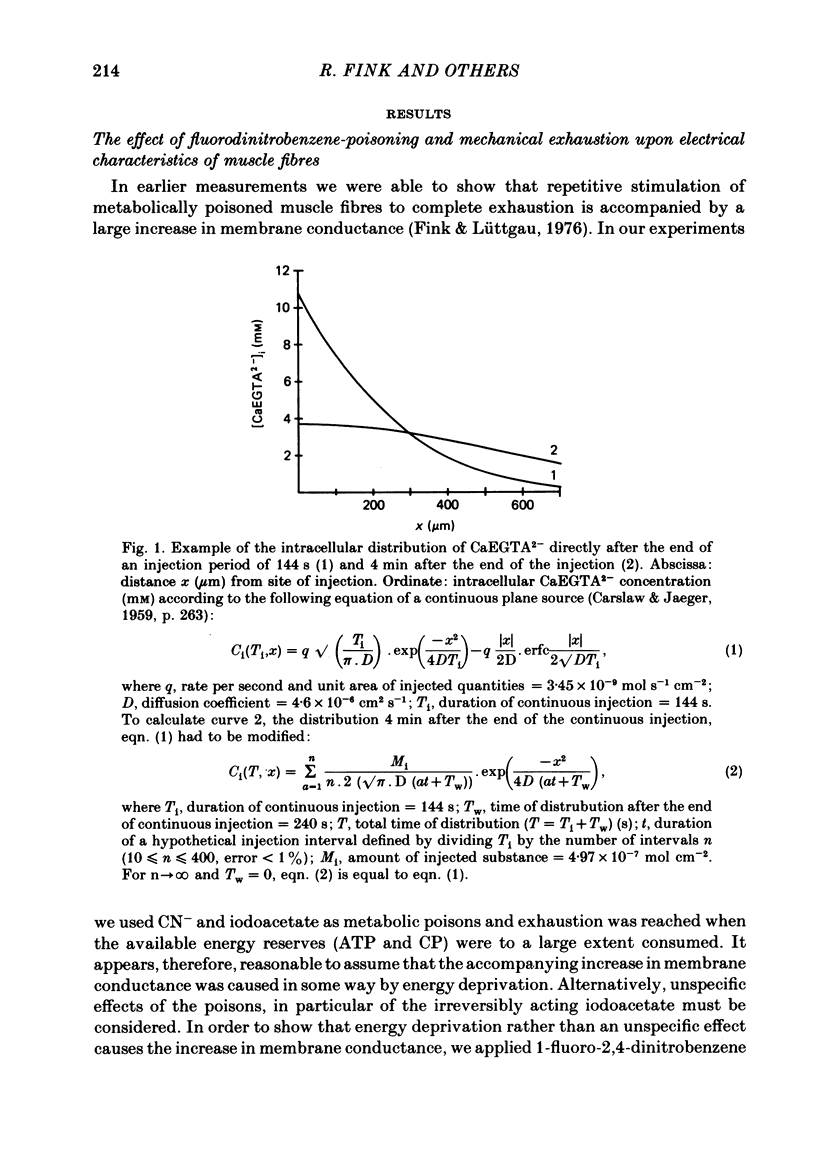
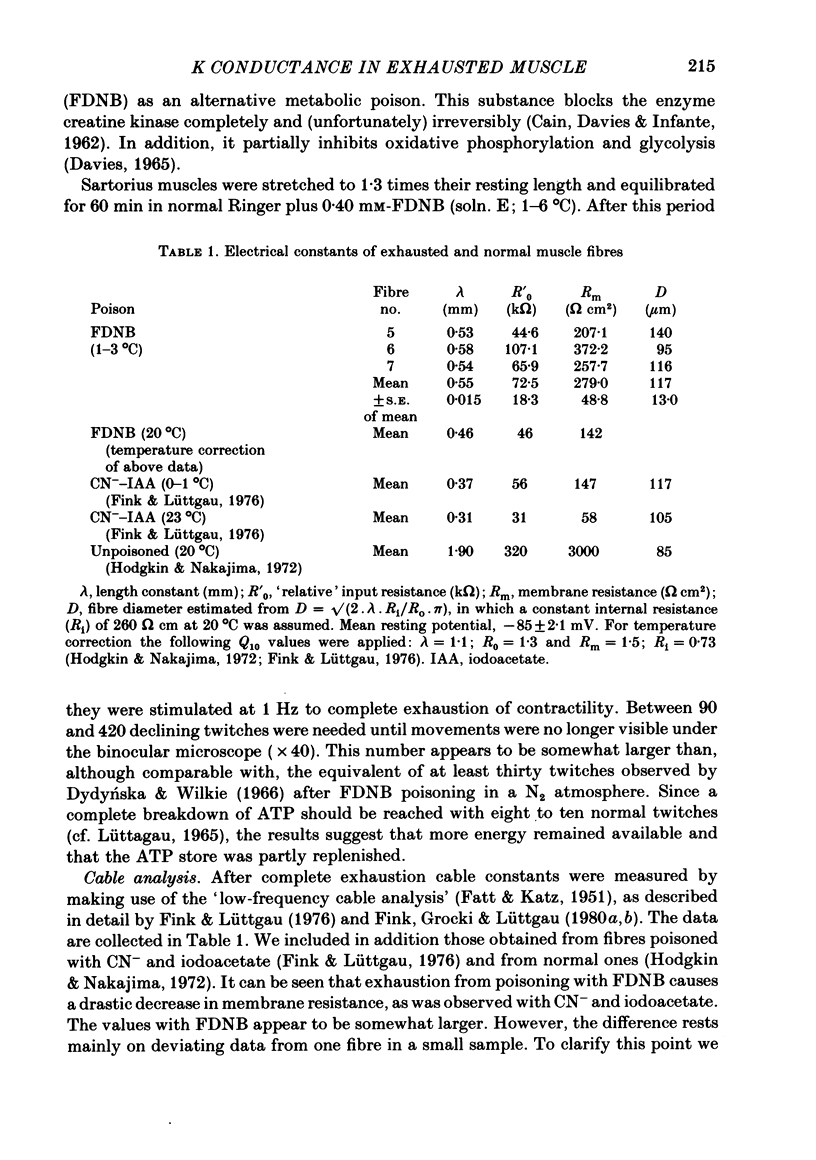
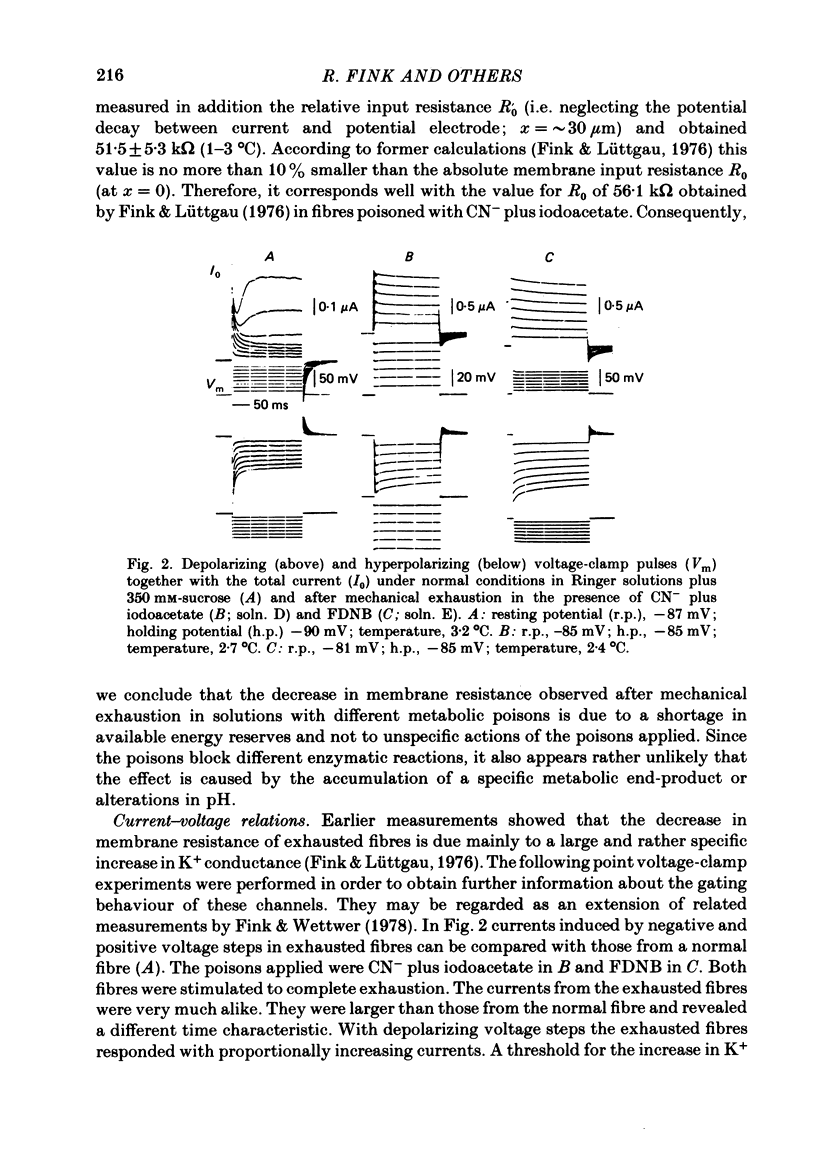
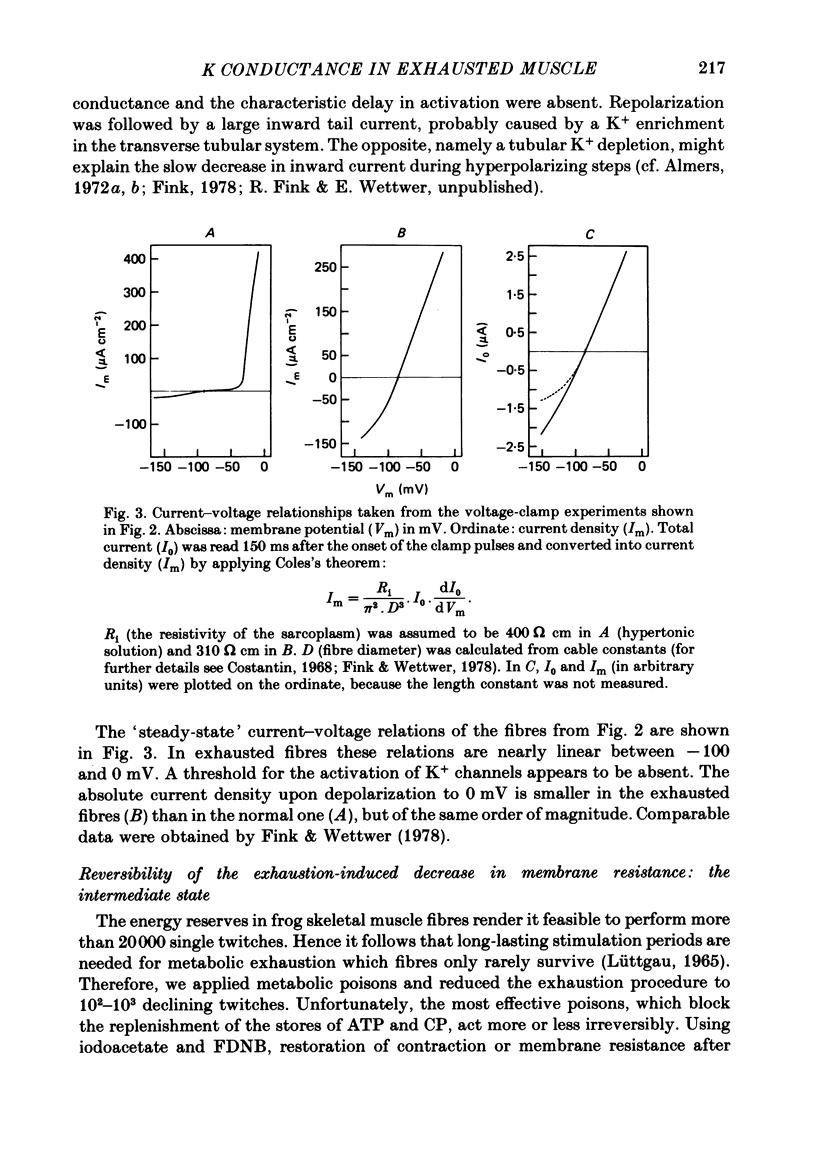
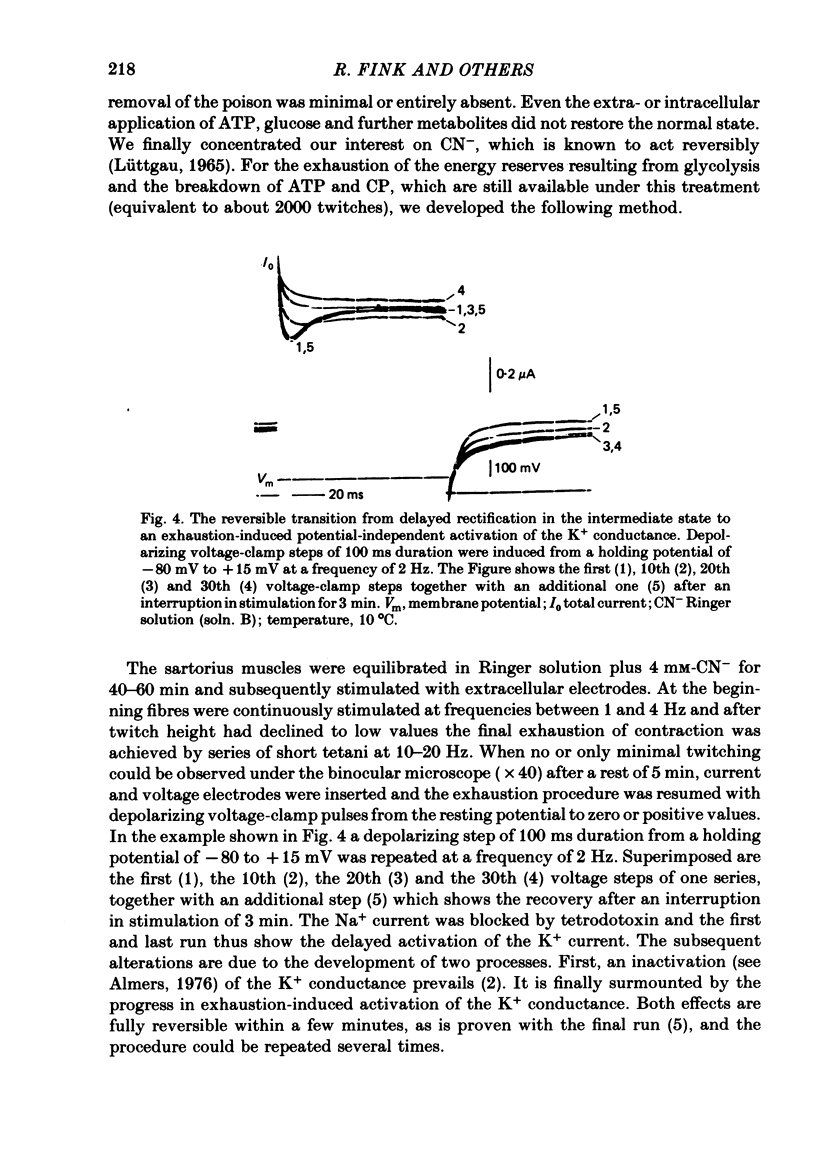
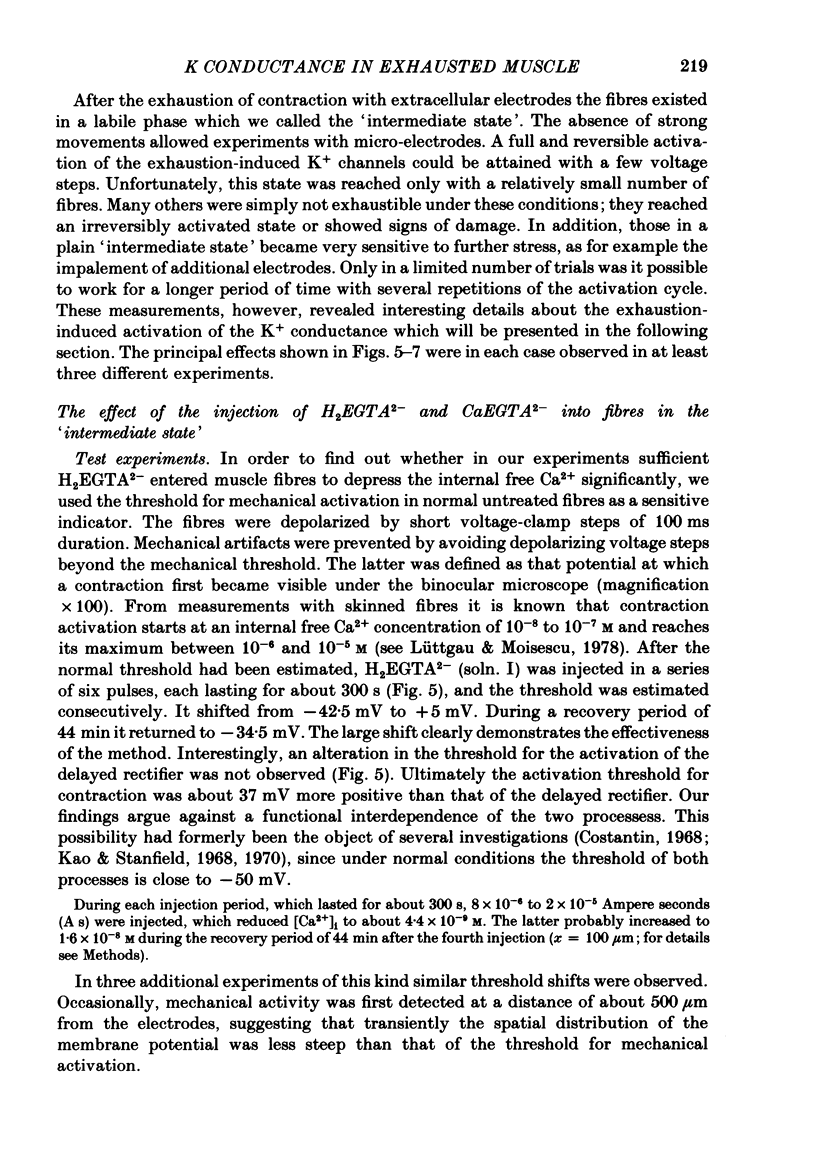
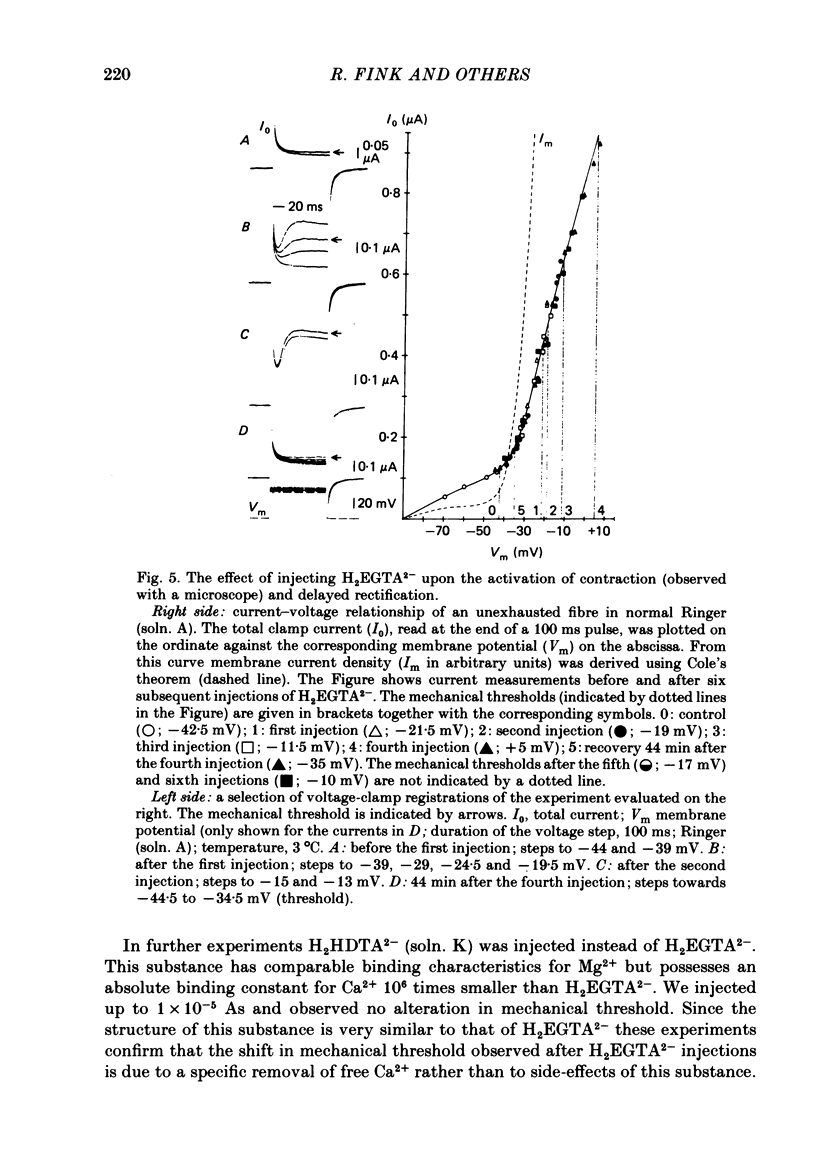
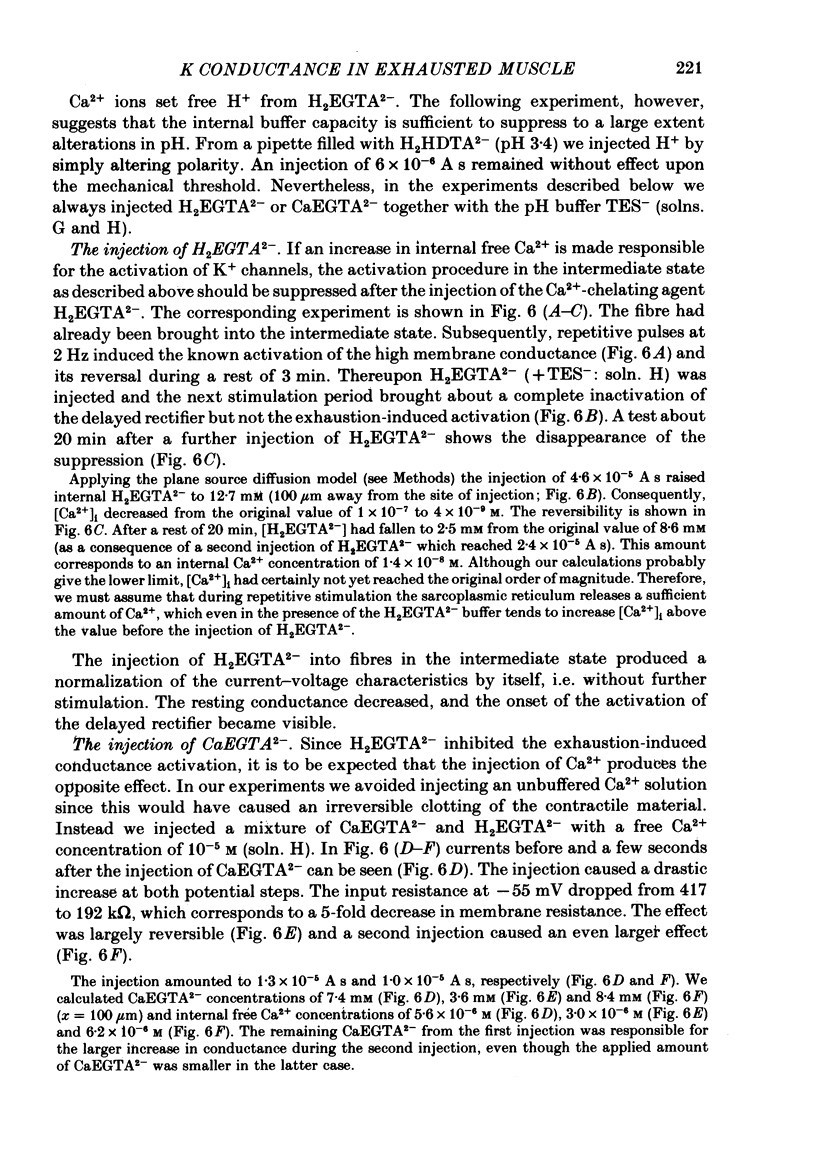
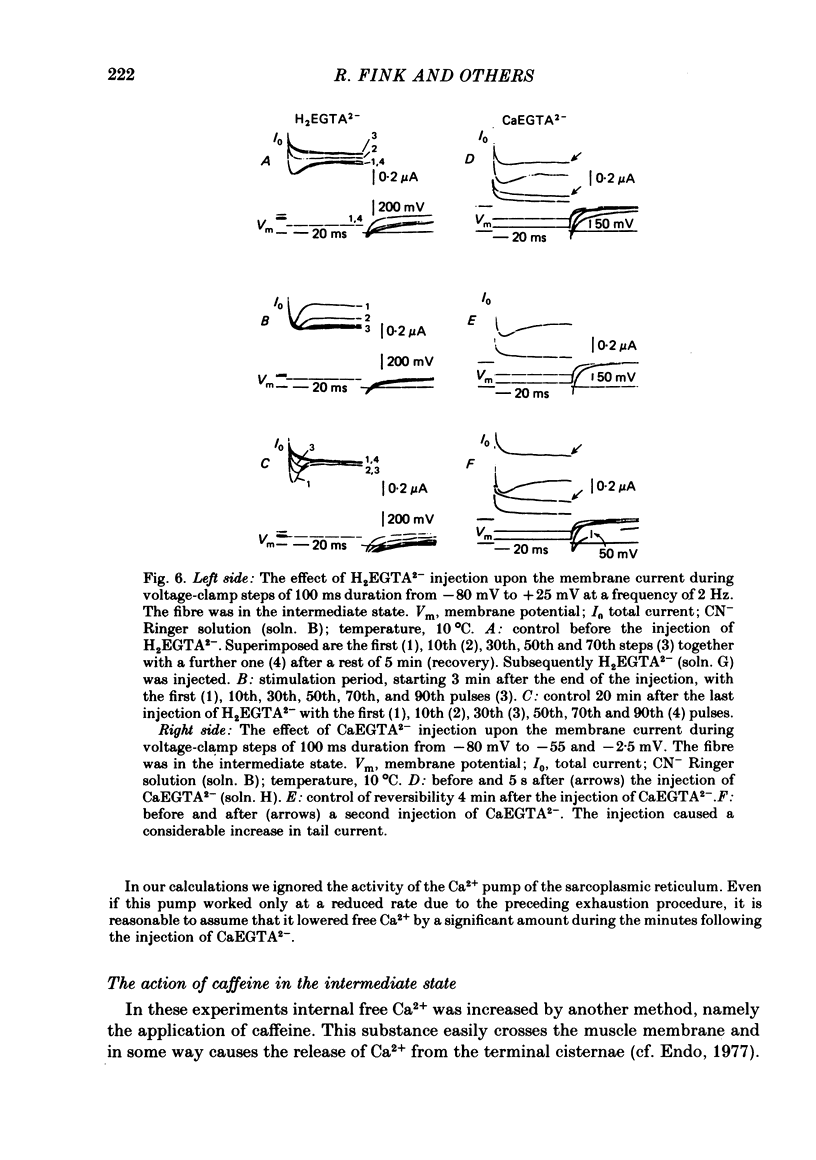
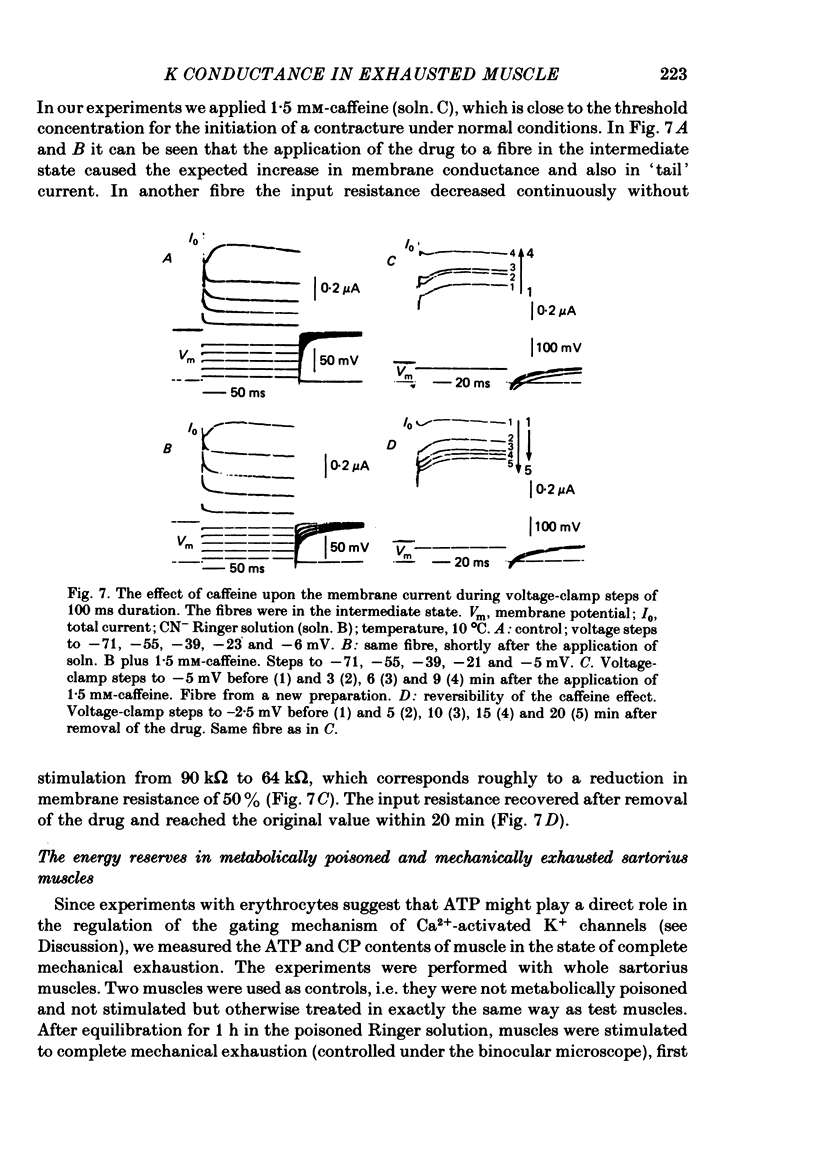
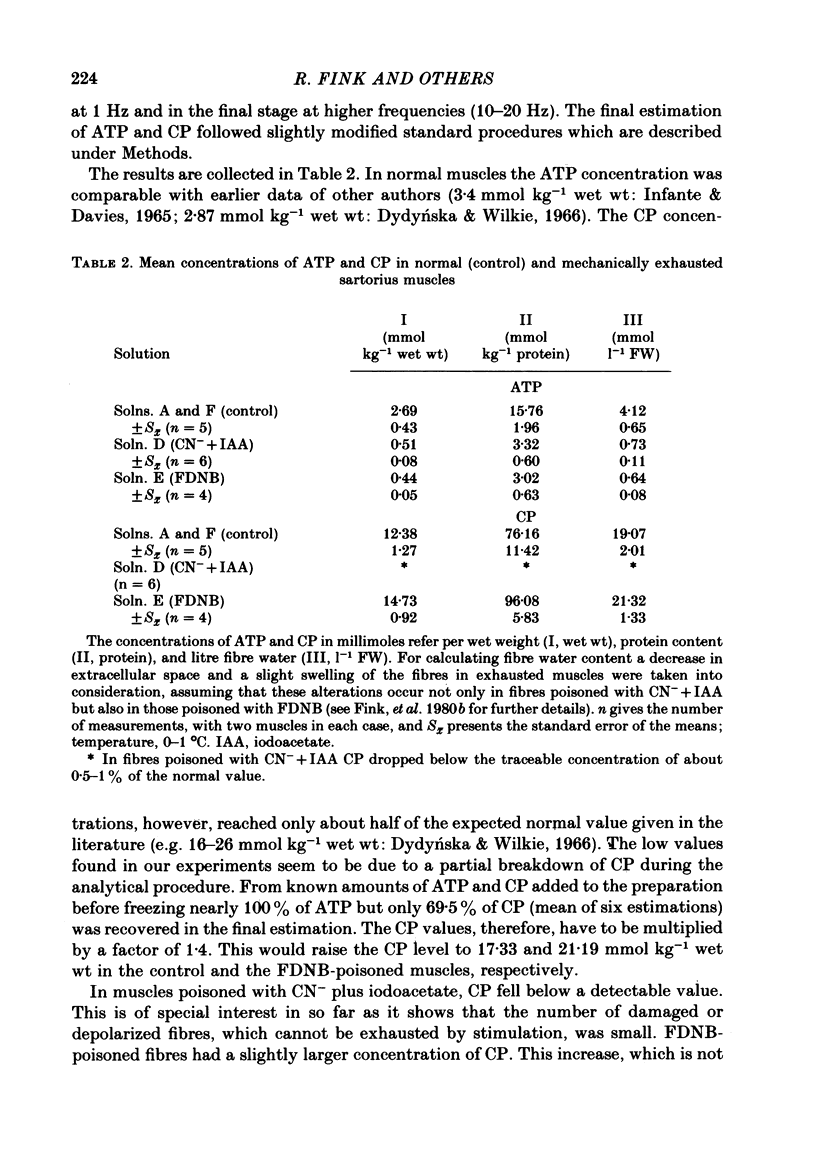
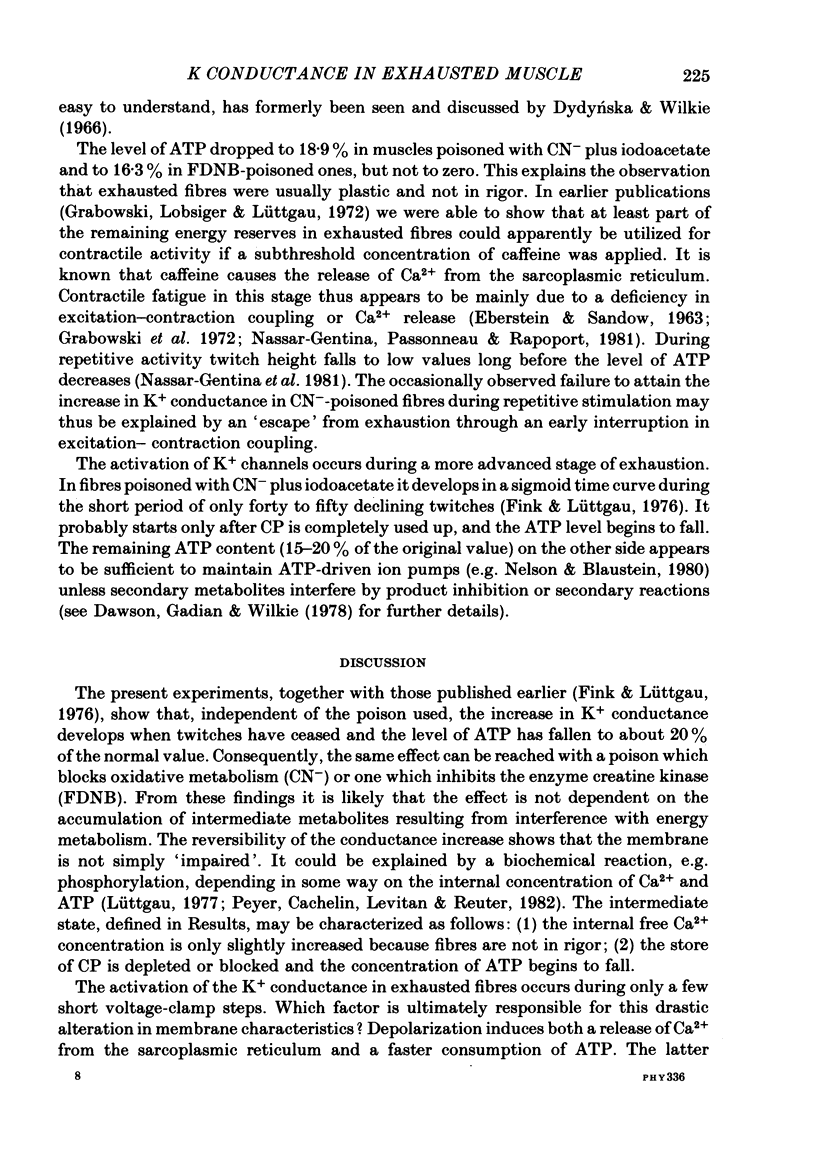
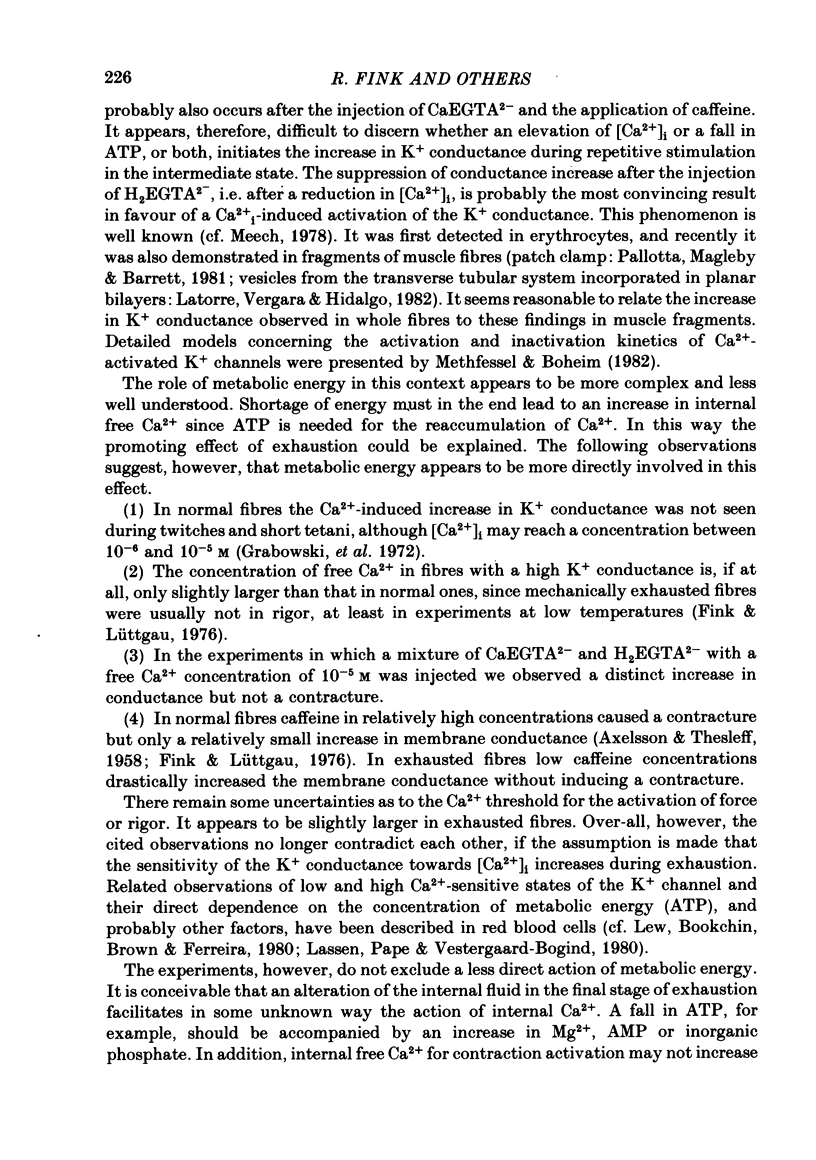
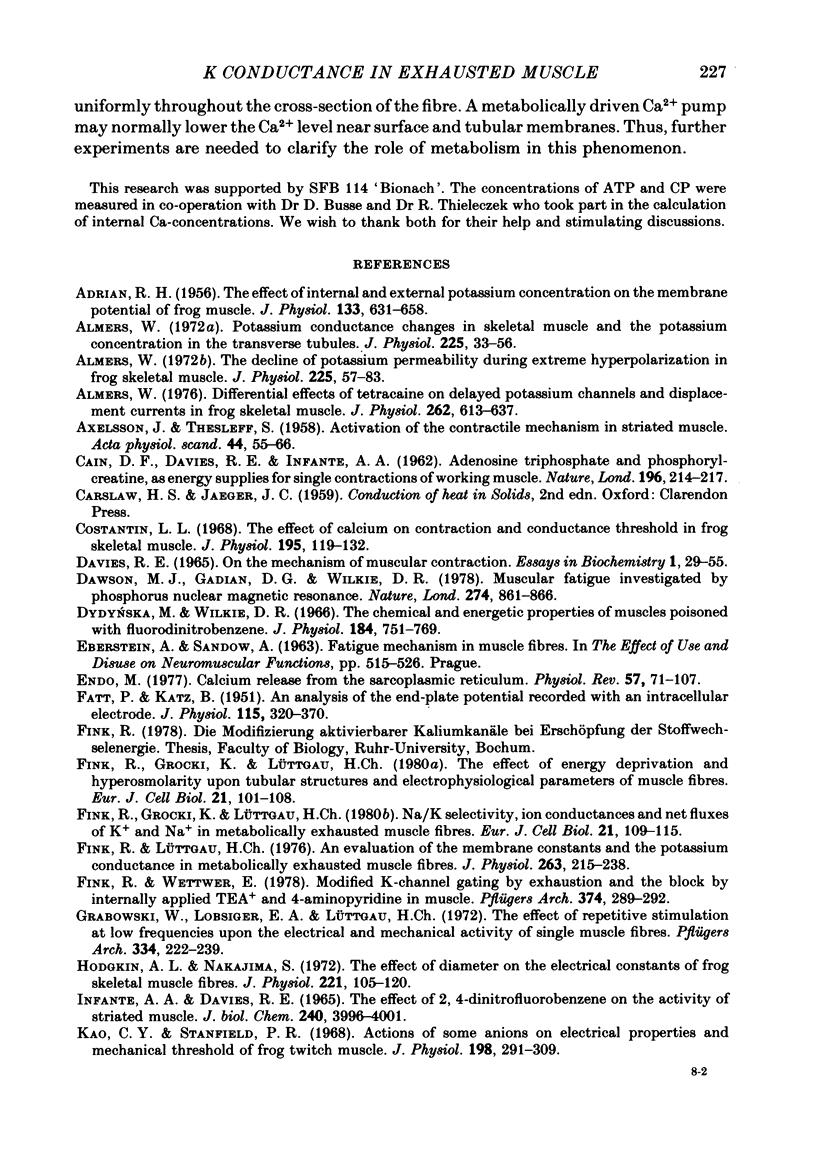
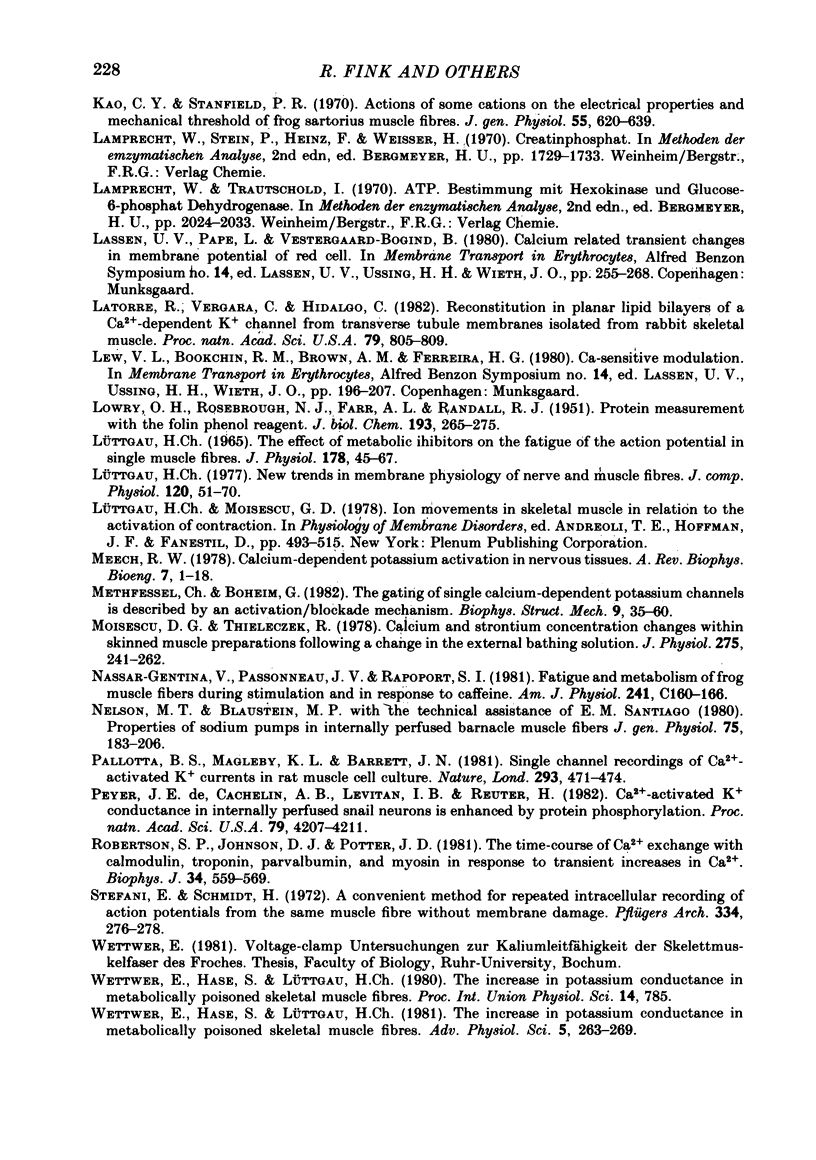
Selected References
These references are in PubMed. This may not be the complete list of references from this article.
- ADRIAN R. H. The effect of internal and external potassium concentration on the membrane potential of frog muscle. J Physiol. 1956 Sep 27;133(3):631–658. doi: 10.1113/jphysiol.1956.sp005615. [DOI] [PMC free article] [PubMed] [Google Scholar]
- AXELSSON J., THESLEFF S. Activation of the contractile mechanism in striated muscle. Acta Physiol Scand. 1958 Oct 28;44(1):55–66. doi: 10.1111/j.1748-1716.1958.tb01608.x. [DOI] [PubMed] [Google Scholar]
- Almers W. Differential effects of tetracaine on delayed potassium channels and displacement currents in frog skeletal muscle. J Physiol. 1976 Nov;262(3):613–637. doi: 10.1113/jphysiol.1976.sp011612. [DOI] [PMC free article] [PubMed] [Google Scholar]
- Almers W. Potassium conductance changes in skeletal muscle and the potassium concentration in the transverse tubules. J Physiol. 1972 Aug;225(1):33–56. doi: 10.1113/jphysiol.1972.sp009928. [DOI] [PMC free article] [PubMed] [Google Scholar]
- Almers W. The decline of potassium permeability during extreme hyperpolarization in frog skeletal muscle. J Physiol. 1972 Aug;225(1):57–83. doi: 10.1113/jphysiol.1972.sp009929. [DOI] [PMC free article] [PubMed] [Google Scholar]
- CAIN D. F., INFANTE A. A., DAVIES R. E. Chemistry of muscle contraction. Adenosine triphosphate and phosphorylcreatine as energy supplies for single contractions of working muscle. Nature. 1962 Oct 20;196:214–217. doi: 10.1038/196214a0. [DOI] [PubMed] [Google Scholar]
- Costantin L. L. The effect o f calcium on contraction and conductance thresholds in frog skeletal muscle. J Physiol. 1968 Mar;195(1):119–132. doi: 10.1113/jphysiol.1968.sp008450. [DOI] [PMC free article] [PubMed] [Google Scholar]
- Davies R. E. On the mechanism of muscular contraction. Essays Biochem. 1965;1:29–55. [PubMed] [Google Scholar]
- Dawson M. J., Gadian D. G., Wilkie D. R. Muscular fatigue investigated by phosphorus nuclear magnetic resonance. Nature. 1978 Aug 31;274(5674):861–866. doi: 10.1038/274861a0. [DOI] [PubMed] [Google Scholar]
- Dydyńska M., Wilkie D. R. The chemical and energetic properties of muscles poisoned with fluorodinitrobenzene. J Physiol. 1966 Jun;184(3):751–769. doi: 10.1113/jphysiol.1966.sp007946. [DOI] [PMC free article] [PubMed] [Google Scholar]
- Endo M. Calcium release from the sarcoplasmic reticulum. Physiol Rev. 1977 Jan;57(1):71–108. doi: 10.1152/physrev.1977.57.1.71. [DOI] [PubMed] [Google Scholar]
- FATT P., KATZ B. An analysis of the end-plate potential recorded with an intracellular electrode. J Physiol. 1951 Nov 28;115(3):320–370. doi: 10.1113/jphysiol.1951.sp004675. [DOI] [PMC free article] [PubMed] [Google Scholar]
- Fink R., Grocki K., Lüttgau H. C. Na/K selectivity, ion conductances and net fluxes of K+ and Na'n metabolically exhausted muscle fibres. Eur J Cell Biol. 1980 Apr;21(1):109–115. [PubMed] [Google Scholar]
- Fink R., Grocki K., Lüttgau H. C. The effect of energy deprivation and hyperosmolarity upon tubular structures and electrophysiological parameters of muscle fibres. Eur J Cell Biol. 1980 Apr;21(1):101–108. [PubMed] [Google Scholar]
- Fink R., Lüttgau H. C. An evaluation of the membrane constants and the potassium conductance in metabolically exhausted muscle fibres. J Physiol. 1976 Dec;263(2):215–238. doi: 10.1113/jphysiol.1976.sp011629. [DOI] [PMC free article] [PubMed] [Google Scholar]
- Fink R., Wettwer E. Modified K-channel gating by exhaustion and the block by internally applied TEA+ and 4-aminopyridine in muscle. Pflugers Arch. 1978 May 31;374(3):289–292. doi: 10.1007/BF00585607. [DOI] [PubMed] [Google Scholar]
- Grabowski W., Lobsiger E. A., Lüttgau H. C. The effect of repetitive stimulation at low frequencies upon the electrical and mechanical activity of single muscle fibres. Pflugers Arch. 1972;334(3):222–239. doi: 10.1007/BF00626225. [DOI] [PubMed] [Google Scholar]
- Hodgkin A. L., Nakajima S. The effect of diameter on the electrical constants of frog skeletal muscle fibres. J Physiol. 1972 Feb;221(1):105–120. doi: 10.1113/jphysiol.1972.sp009742. [DOI] [PMC free article] [PubMed] [Google Scholar]
- Infante A. A., Davies R. E. The effect of 2,4-dinitrofluorobenzene on the activity of striated muscle. J Biol Chem. 1965 Oct;240(10):3996–4001. [PubMed] [Google Scholar]
- Kao C. Y., Stanfield P. R. Actions of some anions on electrical properties and mechanical threshold of frog twitch muscle. J Physiol. 1968 Sep;198(2):291–309. doi: 10.1113/jphysiol.1968.sp008607. [DOI] [PMC free article] [PubMed] [Google Scholar]
- Kao C. Y., Stanfield P. R. Actions of some cations on the electrical properties and mechanical threshold of frog sartorius muscle fibers. J Gen Physiol. 1970 May;55(5):620–639. doi: 10.1085/jgp.55.5.620. [DOI] [PMC free article] [PubMed] [Google Scholar]
- LOWRY O. H., ROSEBROUGH N. J., FARR A. L., RANDALL R. J. Protein measurement with the Folin phenol reagent. J Biol Chem. 1951 Nov;193(1):265–275. [PubMed] [Google Scholar]
- Latorre R., Vergara C., Hidalgo C. Reconstitution in planar lipid bilayers of a Ca2+-dependent K+ channel from transverse tubule membranes isolated from rabbit skeletal muscle. Proc Natl Acad Sci U S A. 1982 Feb;79(3):805–809. doi: 10.1073/pnas.79.3.805. [DOI] [PMC free article] [PubMed] [Google Scholar]
- Meech R. W. Calcium-dependent potassium activation in nervous tissues. Annu Rev Biophys Bioeng. 1978;7:1–18. doi: 10.1146/annurev.bb.07.060178.000245. [DOI] [PubMed] [Google Scholar]
- Methfessel C., Boheim G. The gating of single calcium-dependent potassium channels is described by an activation/blockade mechanism. Biophys Struct Mech. 1982;9(1):35–60. doi: 10.1007/BF00536014. [DOI] [PubMed] [Google Scholar]
- Moisescu D. G., Thieleczek R. Calcium and strontium concentration changes within skinned muscle preparations following a change in the external bathing solution. J Physiol. 1978 Feb;275:241–262. doi: 10.1113/jphysiol.1978.sp012188. [DOI] [PMC free article] [PubMed] [Google Scholar]
- Nassar-Gentina V., Passonneau J. V., Rapoport S. I. Fatigue and metabolism of frog muscle fibers during stimulation and in response to caffeine. Am J Physiol. 1981 Sep;241(3):C160–C166. doi: 10.1152/ajpcell.1981.241.3.C160. [DOI] [PubMed] [Google Scholar]
- Nelson M. T., Blaustein M. P. Properties of sodium pumps in internally perfused barnacle muscle fibers. J Gen Physiol. 1980 Feb;75(2):183–206. doi: 10.1085/jgp.75.2.183. [DOI] [PMC free article] [PubMed] [Google Scholar]
- Pallotta B. S., Magleby K. L., Barrett J. N. Single channel recordings of Ca2+-activated K+ currents in rat muscle cell culture. Nature. 1981 Oct 8;293(5832):471–474. doi: 10.1038/293471a0. [DOI] [PubMed] [Google Scholar]
- Robertson S. P., Johnson J. D., Potter J. D. The time-course of Ca2+ exchange with calmodulin, troponin, parvalbumin, and myosin in response to transient increases in Ca2+. Biophys J. 1981 Jun;34(3):559–569. doi: 10.1016/S0006-3495(81)84868-0. [DOI] [PMC free article] [PubMed] [Google Scholar]
- Stefani E., Schmidt H. A convenient method for repeated intracellular recording of action potentials from the same muscle fibre without membrane damage. Pflugers Arch. 1972;334(3):276–278. doi: 10.1007/BF00626229. [DOI] [PubMed] [Google Scholar]
- de Peyer J. E., Cachelin A. B., Levitan I. B., Reuter H. Ca2+ -activated K+ conductance in internally perfused snail neurons is enhanced by protein phosphorylation. Proc Natl Acad Sci U S A. 1982 Jul;79(13):4207–4211. doi: 10.1073/pnas.79.13.4207. [DOI] [PMC free article] [PubMed] [Google Scholar]