Abstract
We used a patch clamp to measure Na currents across 10-15 micron diameter circular patches of frog and rat skeletal muscle membrane. We tested for electrophoretic mobility of Na channels, by applying steady lateral fields (of the order of 10 mV micron-1) across the wall of the patch pipette. Application of steady negative potentials to the inside of the pipette resulted in a fall in the number of functional Na channels in the patch. This fall took several minutes to complete and was reversible. It was assayed by applying suitable depolarizations at approximately 11 sec intervals. When a steady lateral field was applied in the absence of changes in membrane potential of the patch, the loss of Na current was virtually abolished. Thus it was not due to electrophoretic movement of channels, but instead to depolarization of the sarcolemma. Evidently, a very slow inactivation of Na conductance operates in skeletal muscle. In frog muscle, the rate constants for loss and recovery of Na current were about 0.1 min-1 (17 degrees C) at resting potential. Rate constants were higher at more positive and at more negative membrane potentials. Current amplitude was reduced to 0.5 at about -76 mV. Roughly similar results were found in rat omohyoid muscle. A further inactivation mechanism, whose rate was intermediate between conventional fast inactivation and the very slow process described here, was present also in both rat and frog muscle. In frog muscle, lateral fields do not alter the potential dependence of fast inactivation. Either the surface charge due to membrane lipids does not influence inactivation or the lipids immediately surrounding the Na channel are restricted in their mobility.
Full text
PDF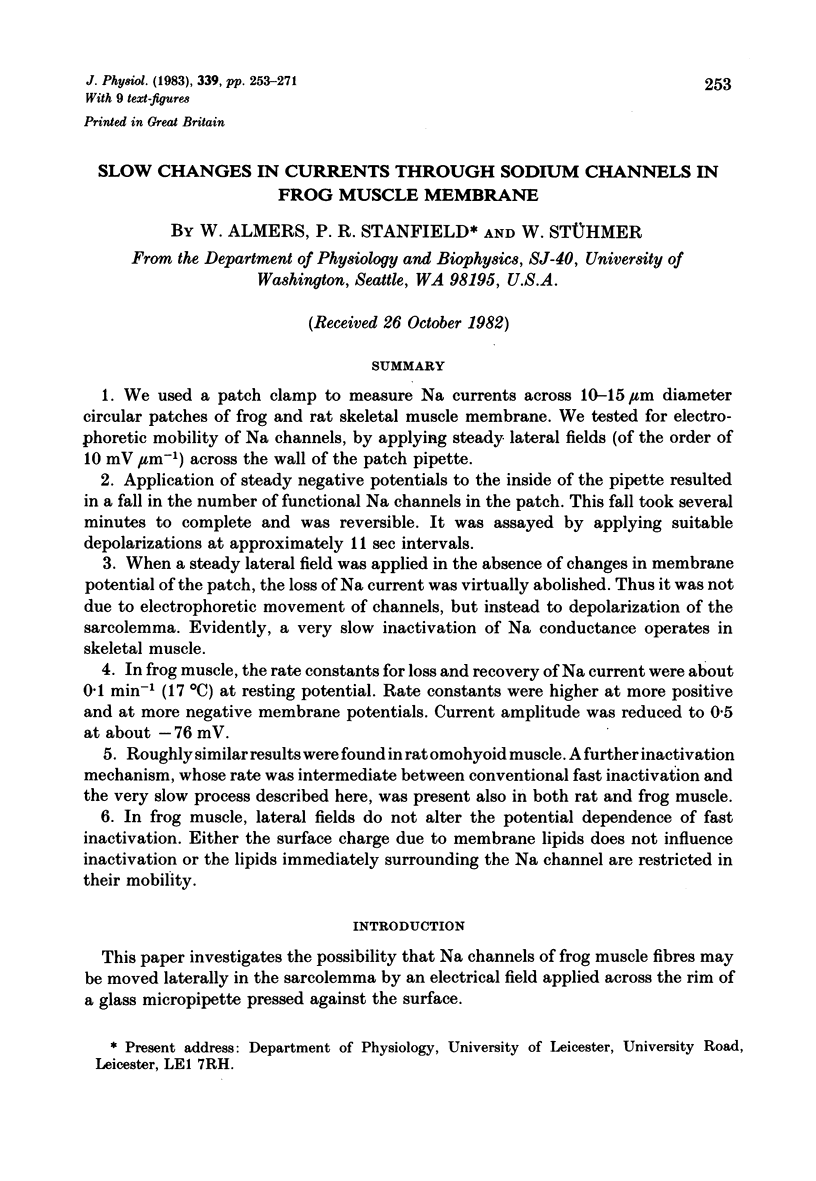
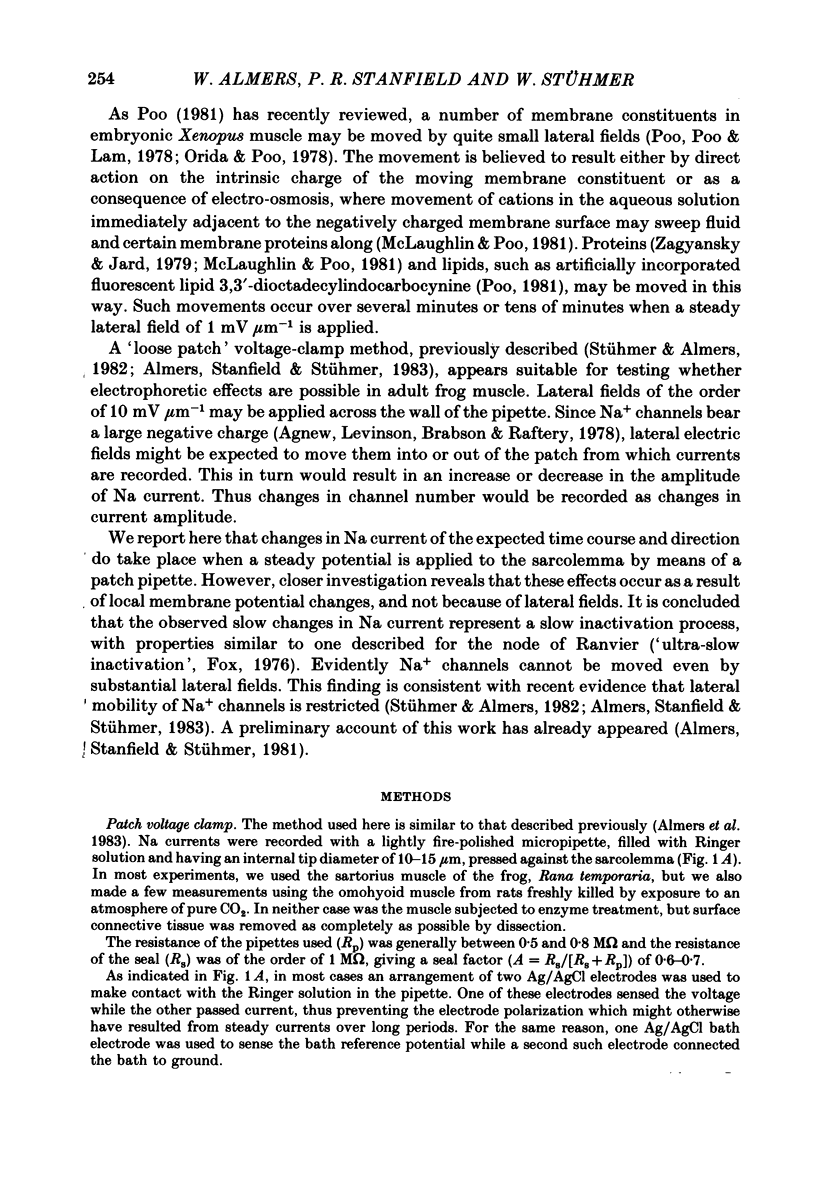
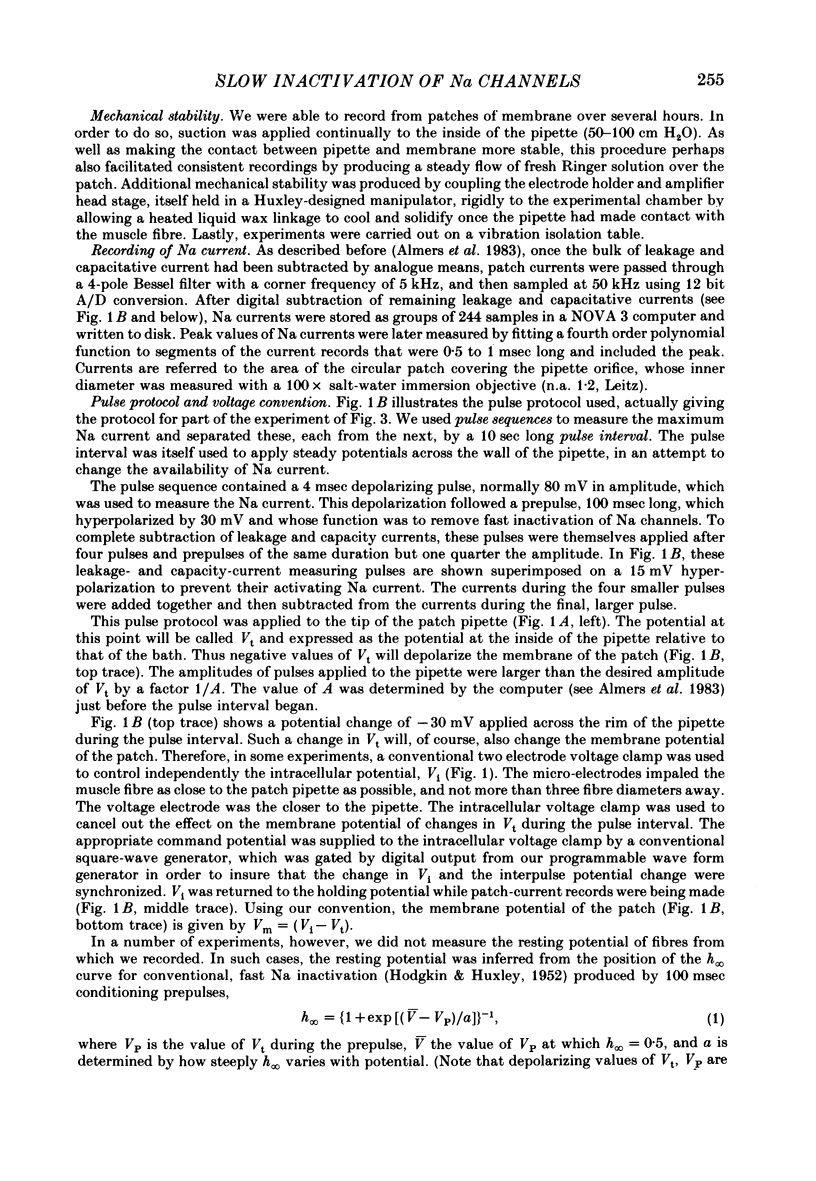
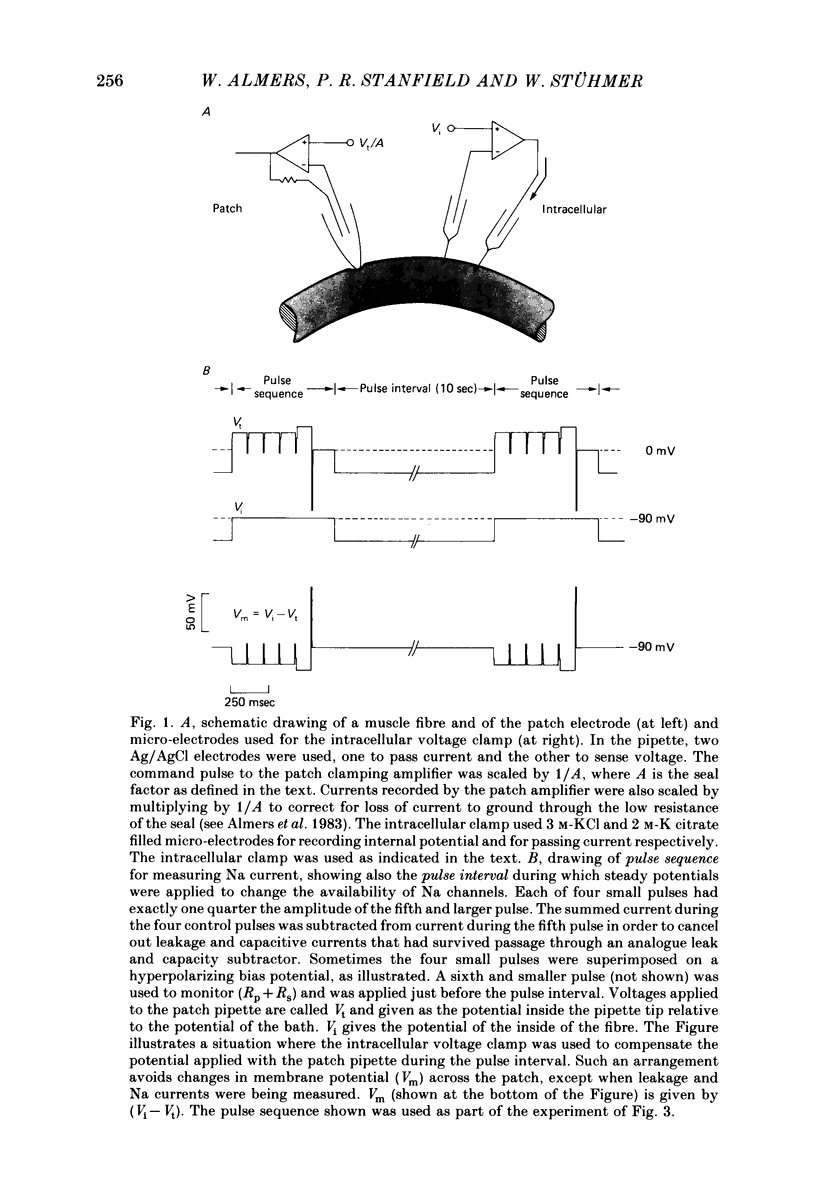
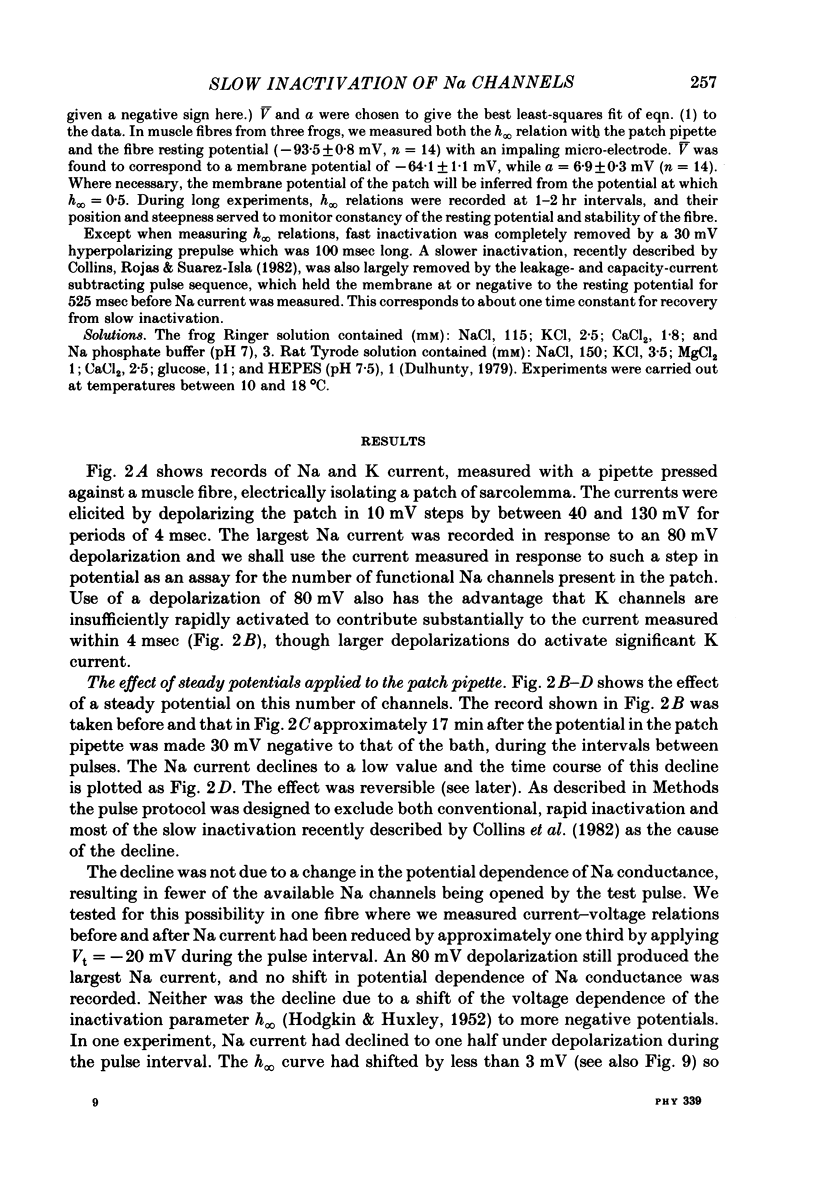
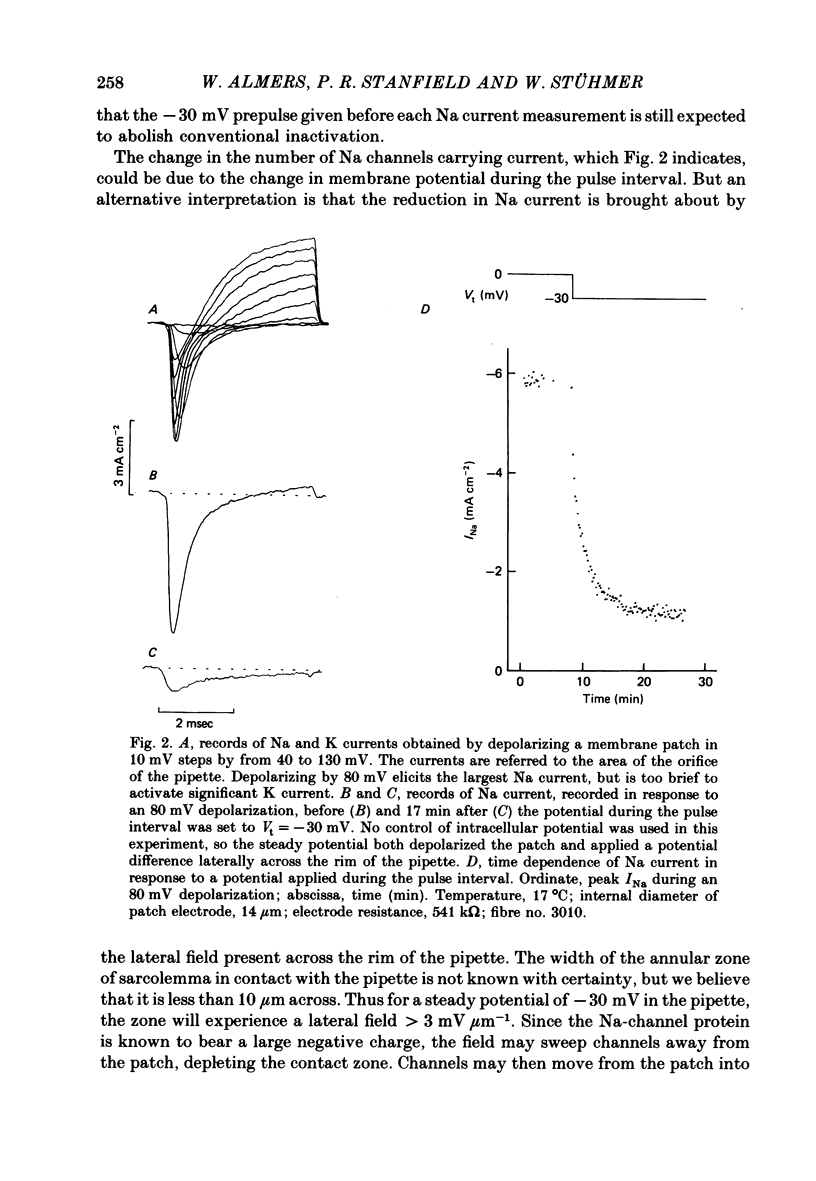
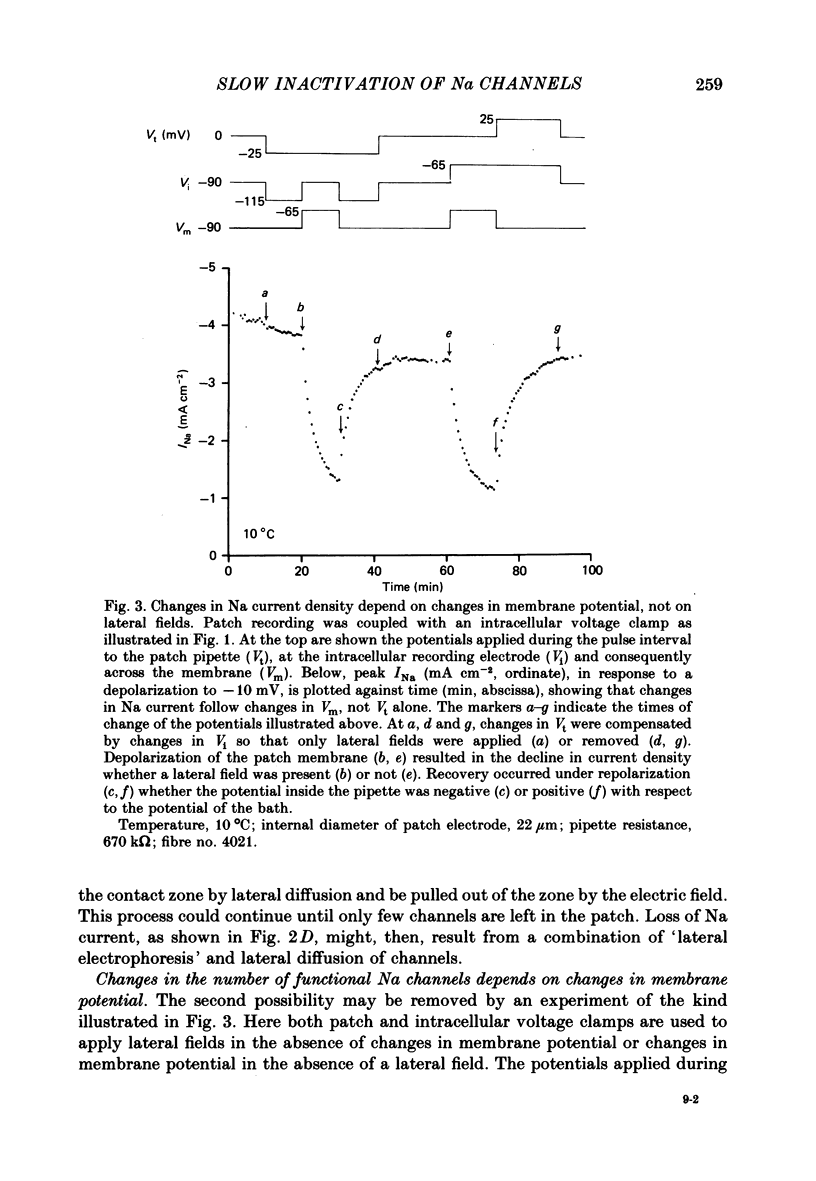
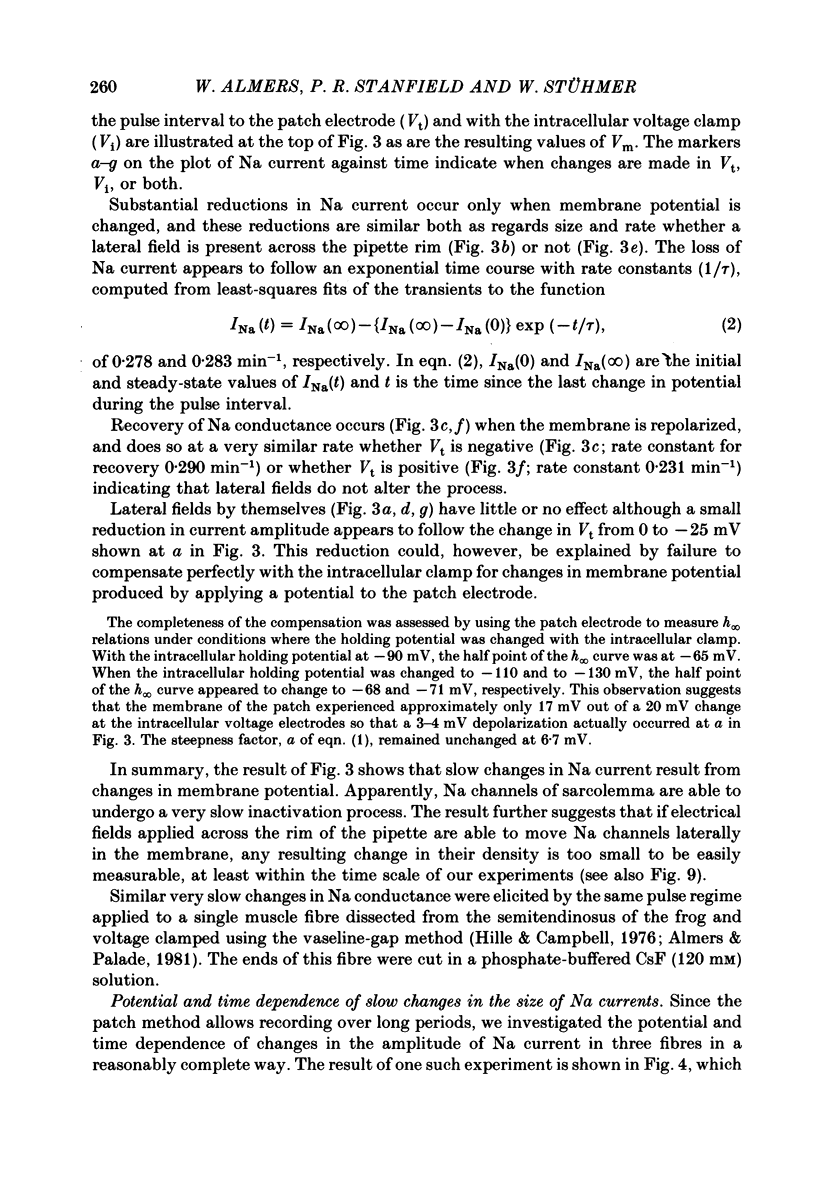
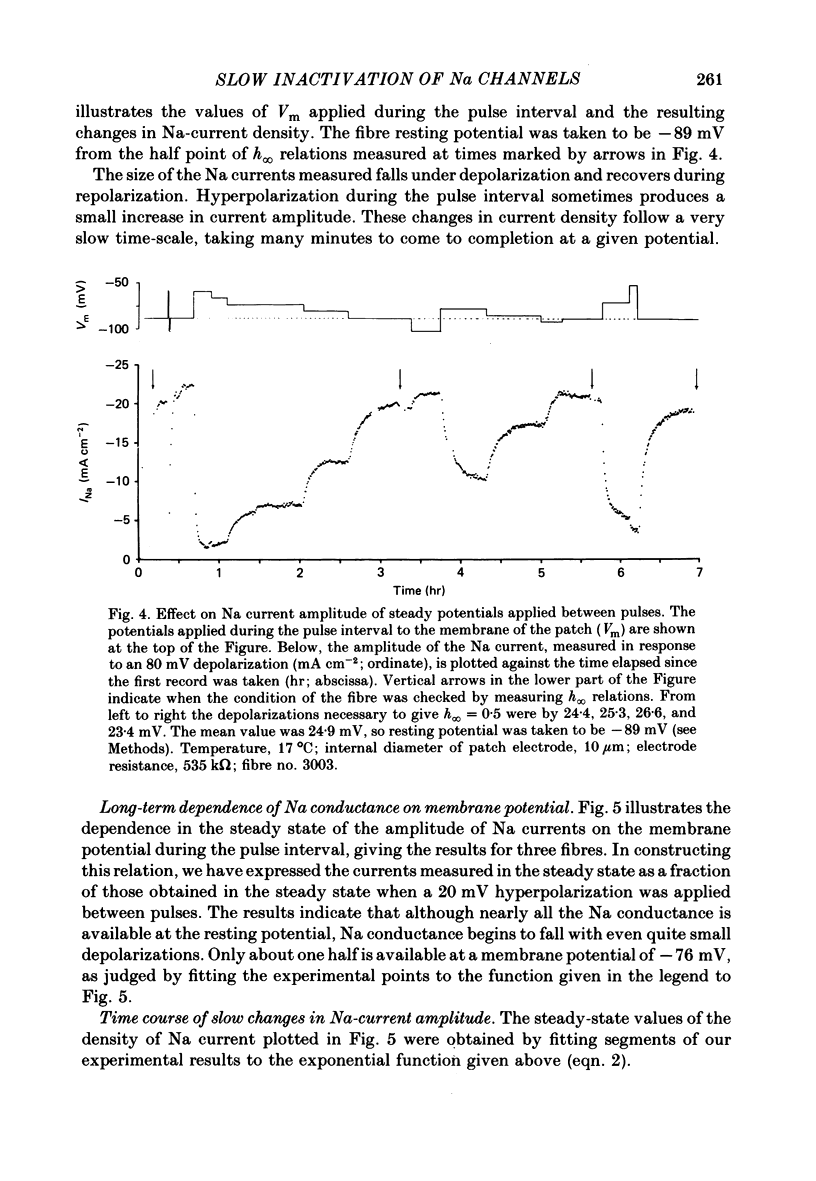
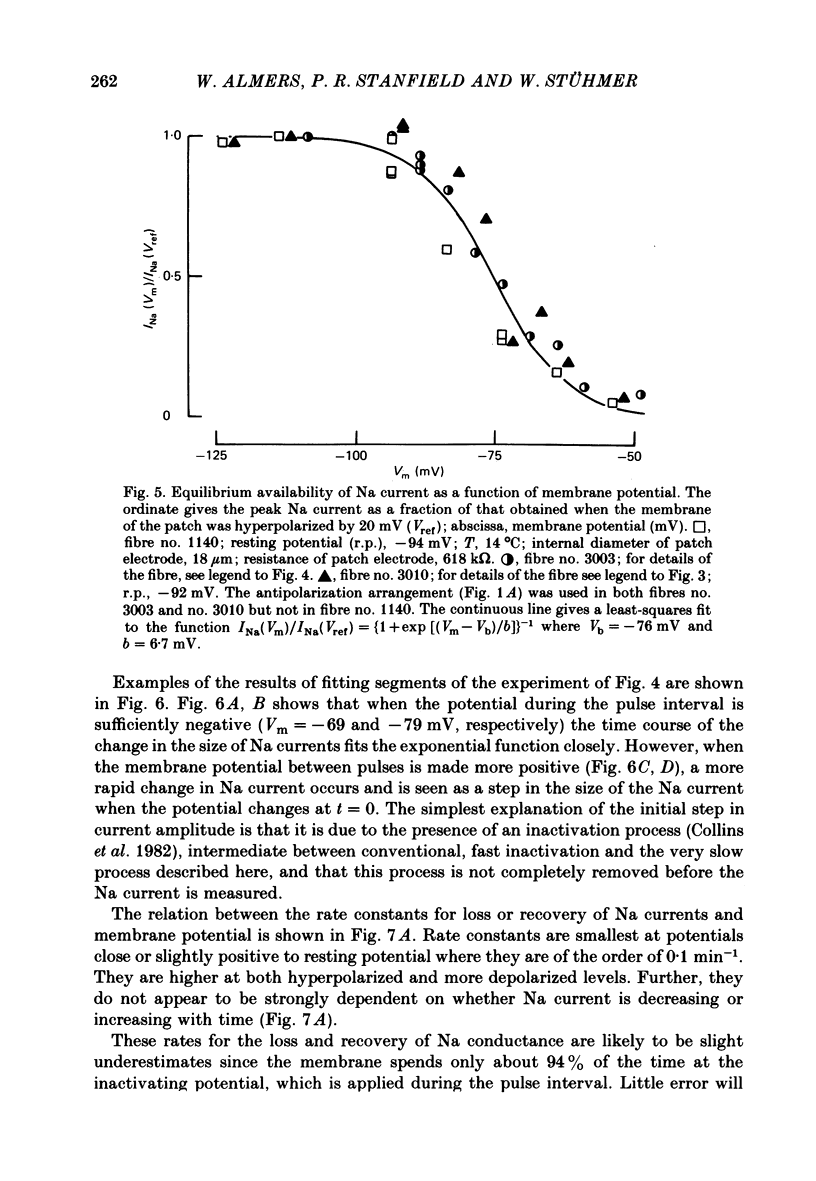
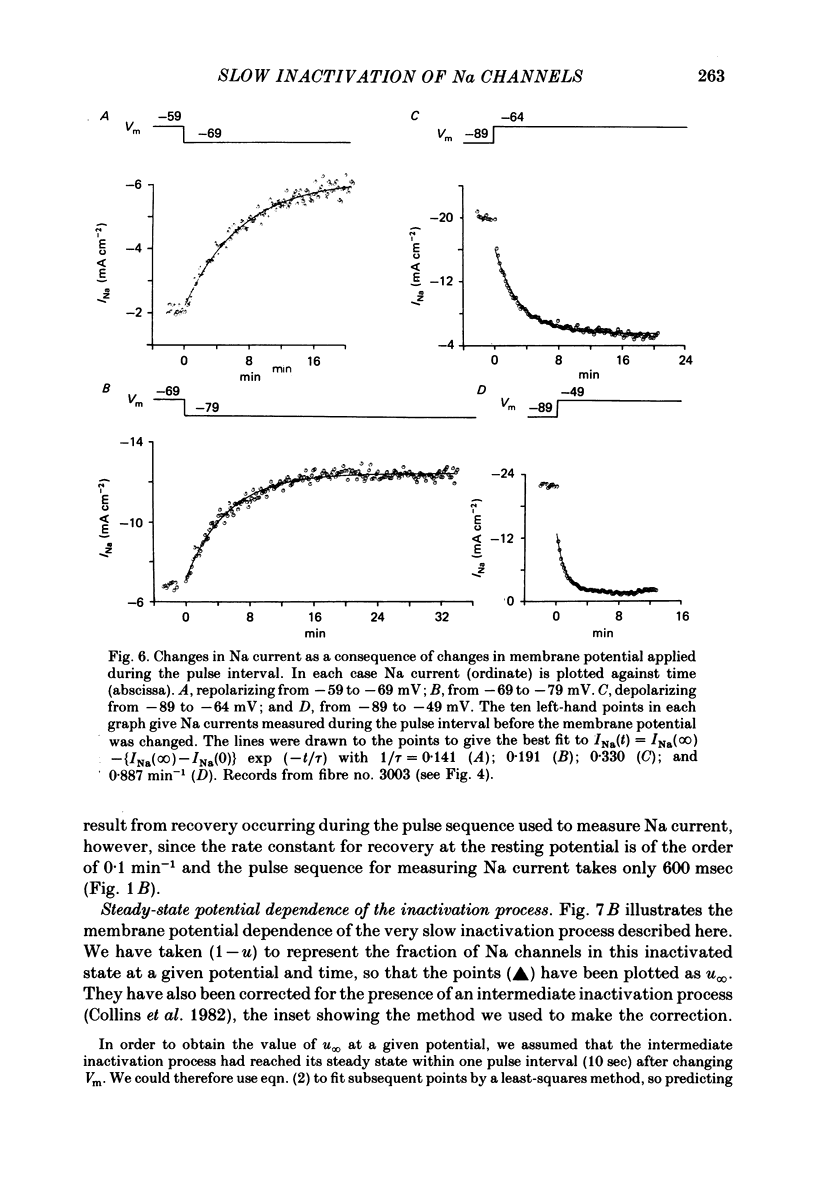
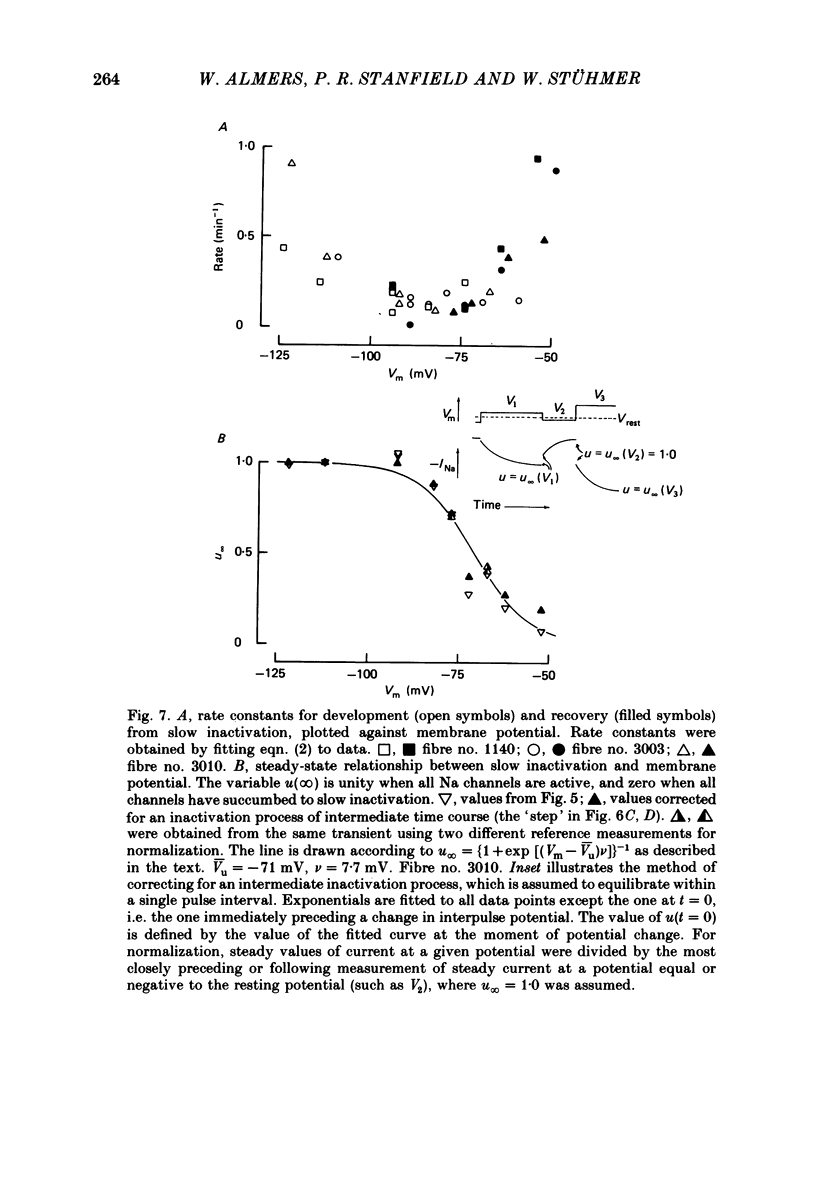
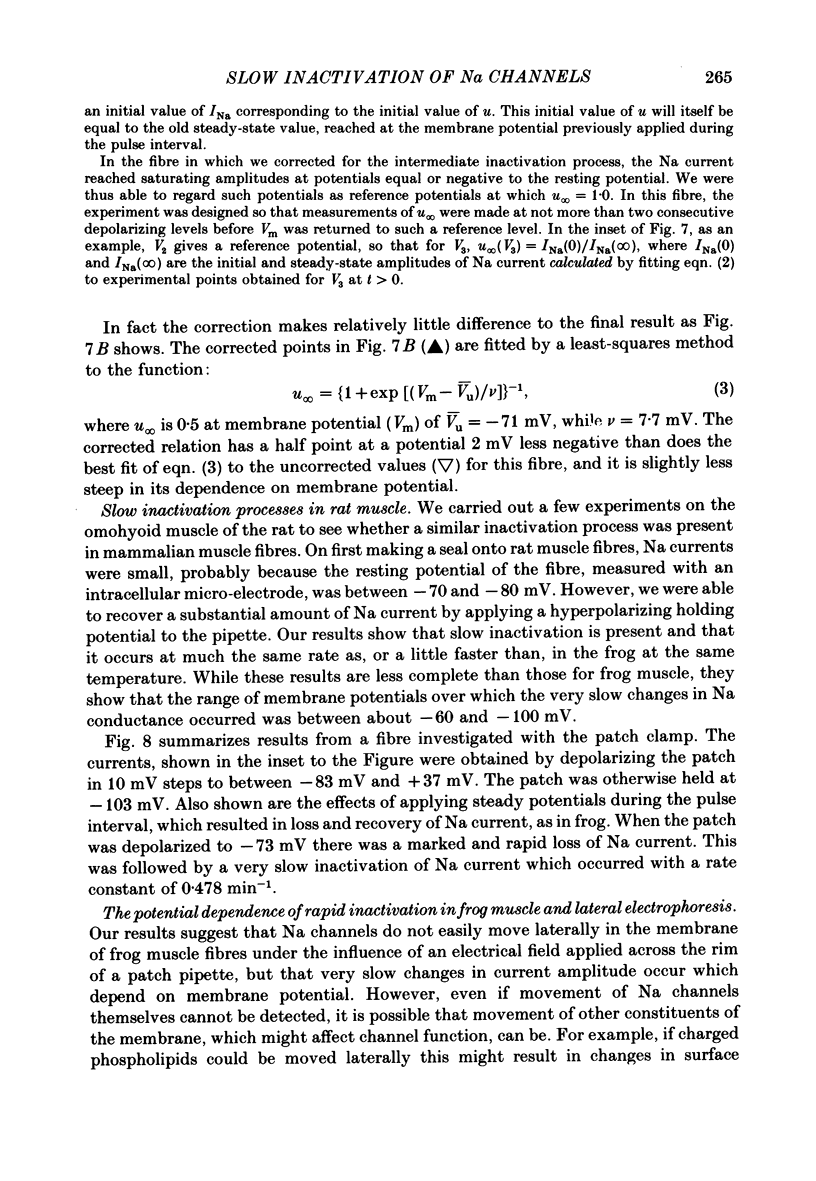
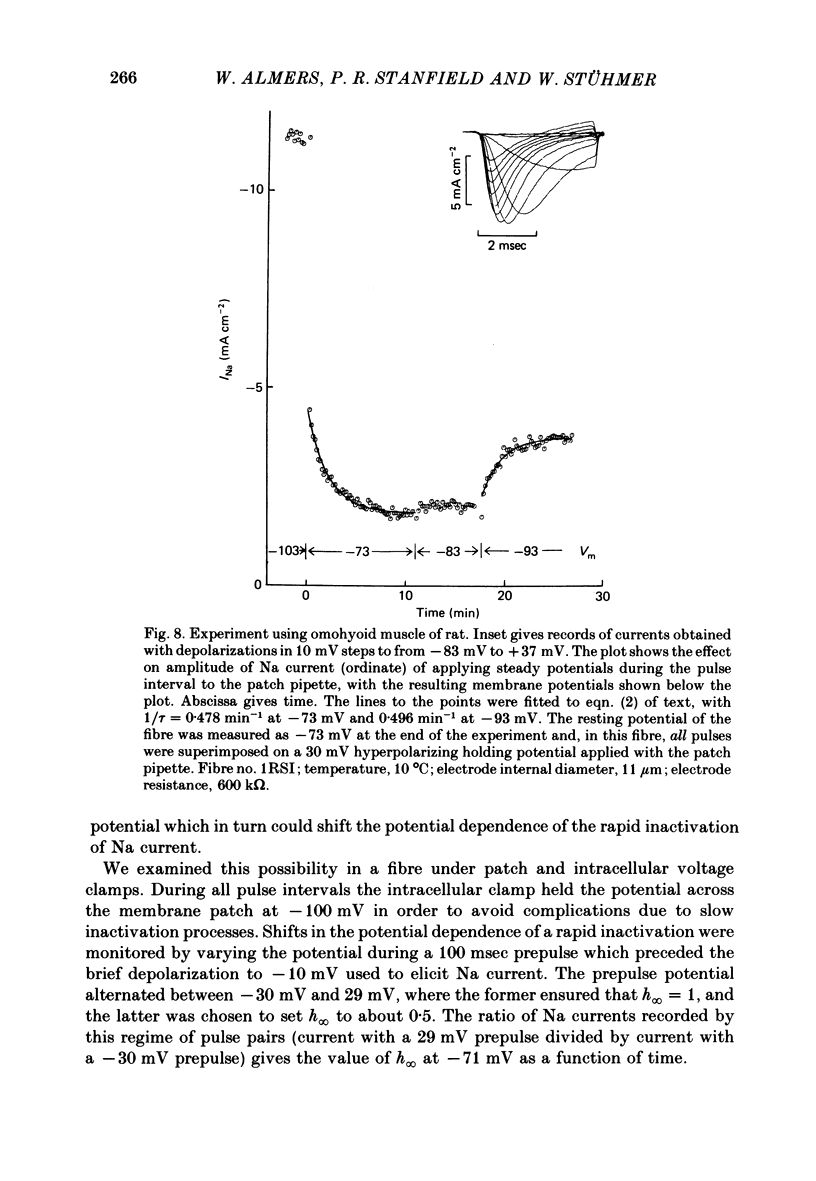
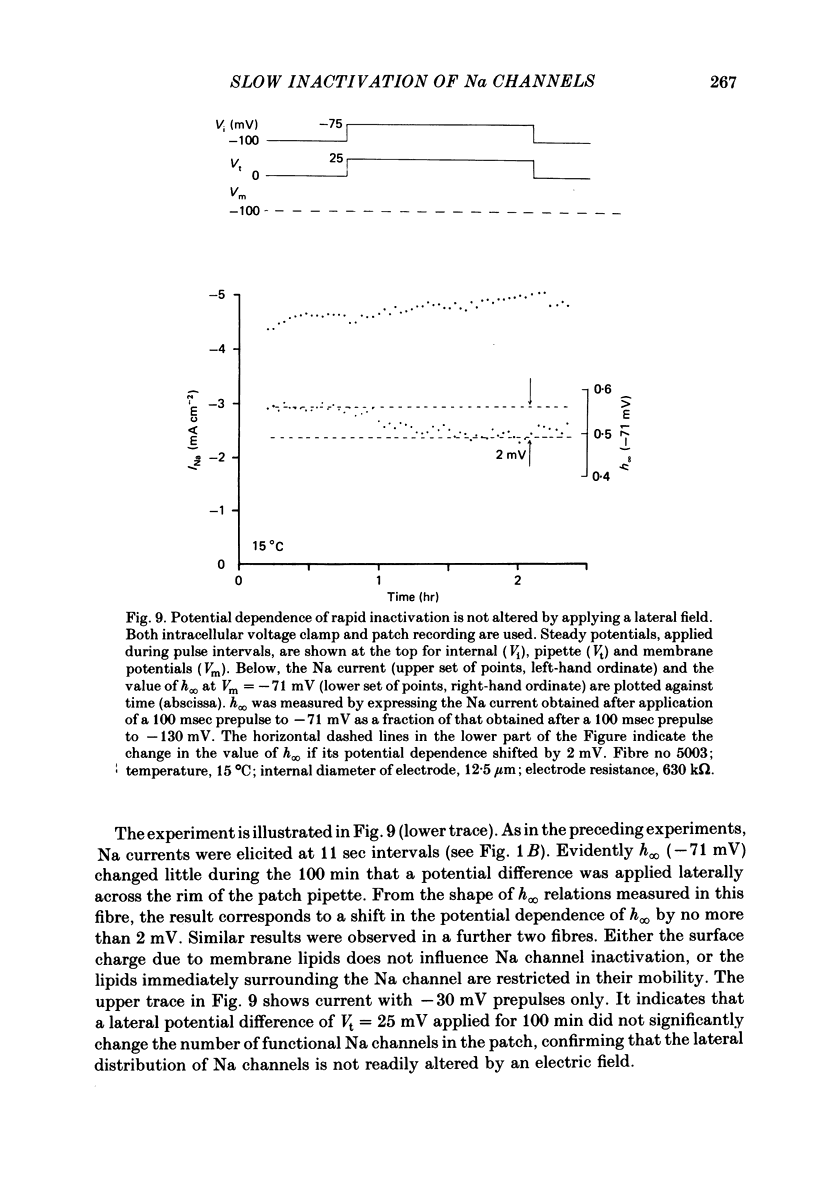
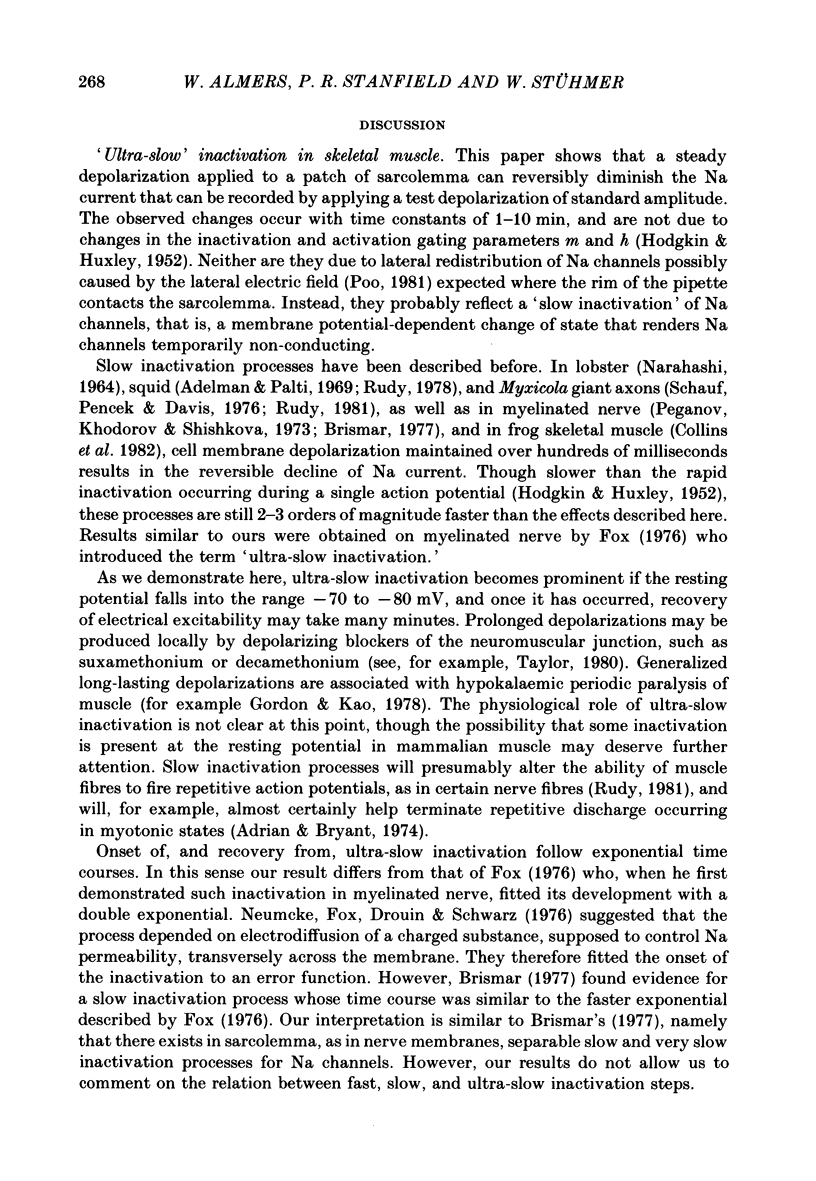
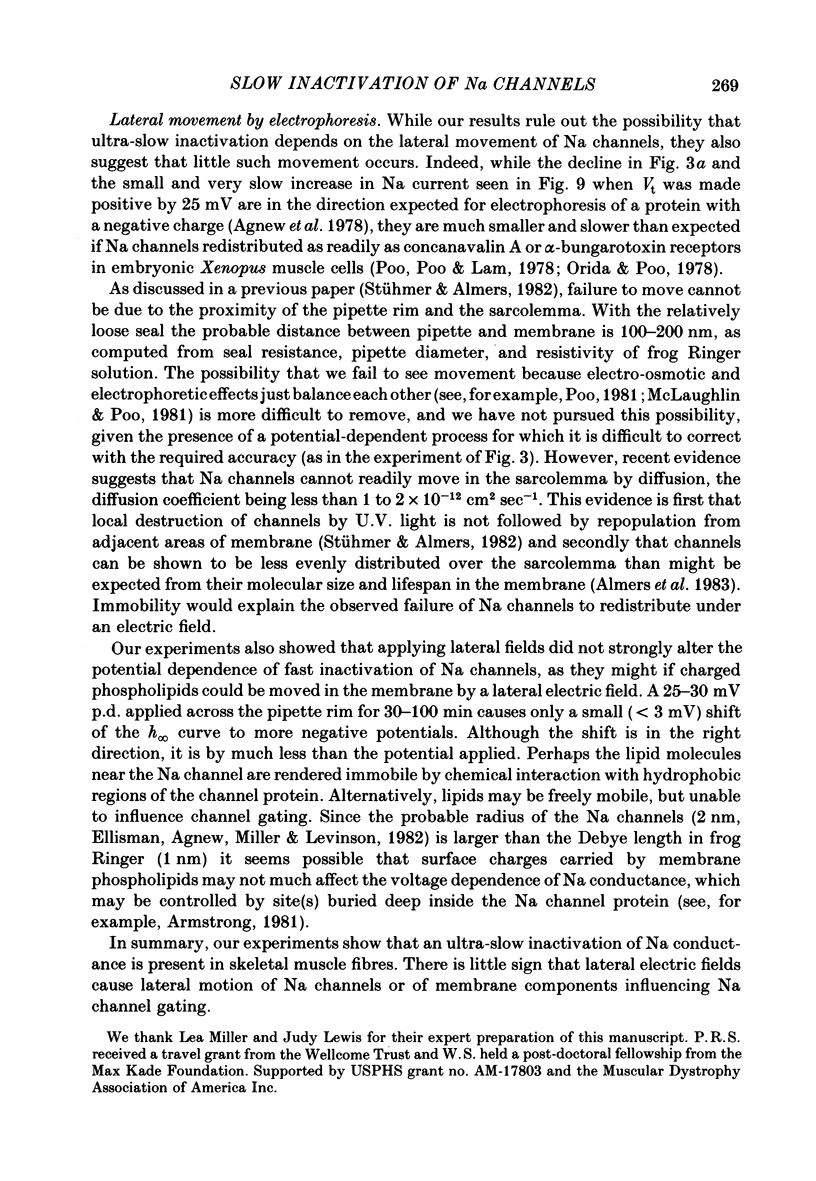
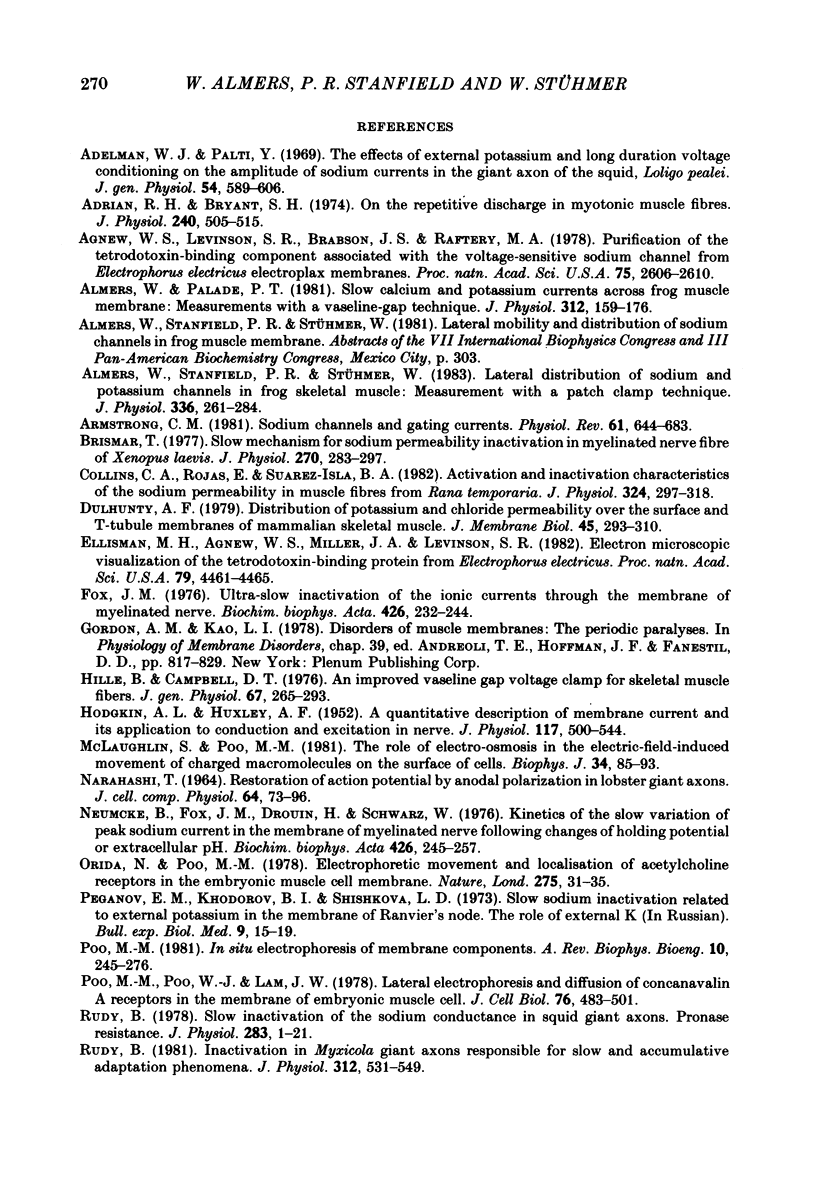
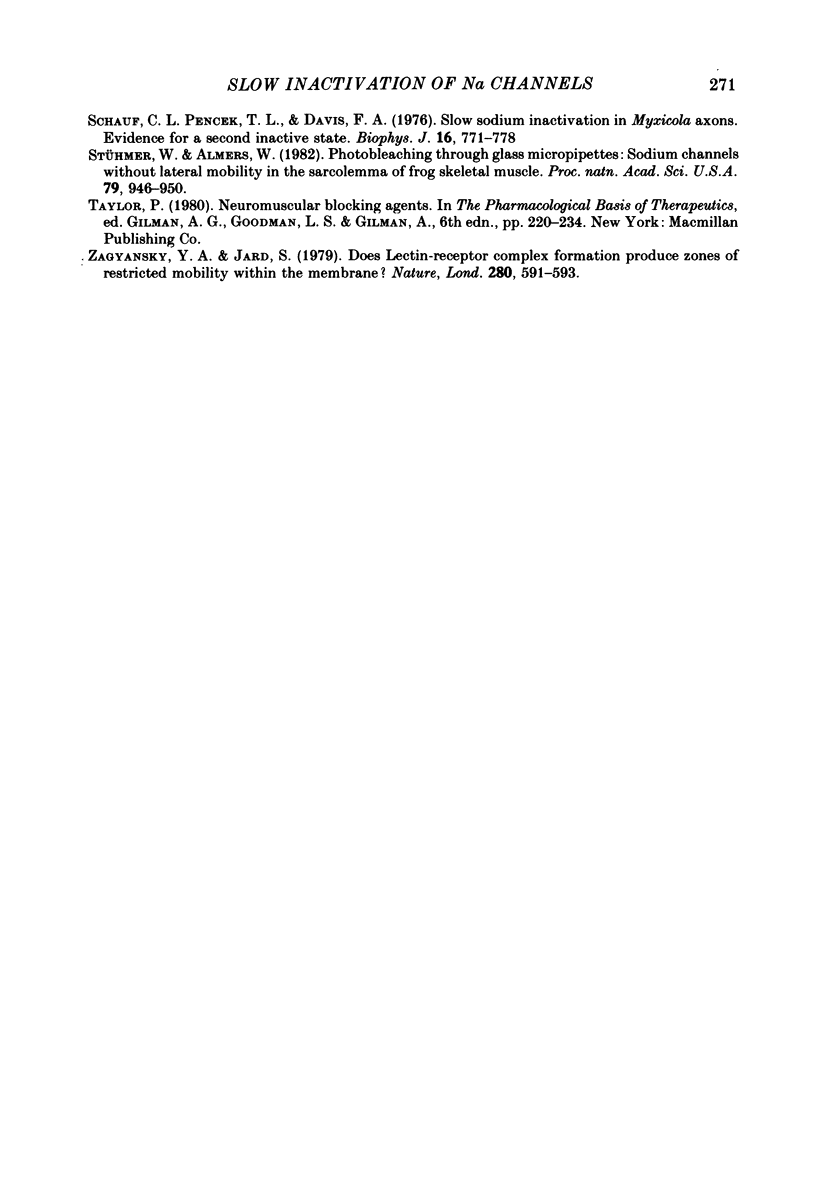
Images in this article
Selected References
These references are in PubMed. This may not be the complete list of references from this article.
- Adelman W. J., Jr, Palti Y. The effects of external potassium and long duration voltage conditioning on the amplitude of sodium currents in the giant axon of the squid, Loligo pealei. J Gen Physiol. 1969 Nov;54(5):589–606. doi: 10.1085/jgp.54.5.589. [DOI] [PMC free article] [PubMed] [Google Scholar]
- Adrian R. H., Bryant S. H. On the repetitive discharge in myotonic muscle fibres. J Physiol. 1974 Jul;240(2):505–515. doi: 10.1113/jphysiol.1974.sp010620. [DOI] [PMC free article] [PubMed] [Google Scholar]
- Agnew W. S., Levinson S. R., Brabson J. S., Raftery M. A. Purification of the tetrodotoxin-binding component associated with the voltage-sensitive sodium channel from Electrophorus electricus electroplax membranes. Proc Natl Acad Sci U S A. 1978 Jun;75(6):2606–2610. doi: 10.1073/pnas.75.6.2606. [DOI] [PMC free article] [PubMed] [Google Scholar]
- Almers W., Palade P. T. Slow calcium and potassium currents across frog muscle membrane: measurements with a vaseline-gap technique. J Physiol. 1981 Mar;312:159–176. doi: 10.1113/jphysiol.1981.sp013622. [DOI] [PMC free article] [PubMed] [Google Scholar]
- Almers W., Stanfield P. R., Stühmer W. Lateral distribution of sodium and potassium channels in frog skeletal muscle: measurements with a patch-clamp technique. J Physiol. 1983 Mar;336:261–284. doi: 10.1113/jphysiol.1983.sp014580. [DOI] [PMC free article] [PubMed] [Google Scholar]
- Armstrong C. M. Sodium channels and gating currents. Physiol Rev. 1981 Jul;61(3):644–683. doi: 10.1152/physrev.1981.61.3.644. [DOI] [PubMed] [Google Scholar]
- Brismar T. Slow mechanism for sodium permeability inactivation in myelinated nerve fibre of Xenopus laevis. J Physiol. 1977 Sep;270(2):283–297. doi: 10.1113/jphysiol.1977.sp011952. [DOI] [PMC free article] [PubMed] [Google Scholar]
- Collins C. A., Rojas E., Suarez-Isla B. A. Activation and inactivation characteristics of the sodium permeability in muscle fibres from Rana temporaria. J Physiol. 1982 Mar;324:297–318. doi: 10.1113/jphysiol.1982.sp014114. [DOI] [PMC free article] [PubMed] [Google Scholar]
- Dulhunty A. F. Distribution of potassium and chloride permeability over the surface and T-tubule membranes of mammalian skeletal muscle. J Membr Biol. 1979 Apr 9;45(3-4):293–310. doi: 10.1007/BF01869290. [DOI] [PubMed] [Google Scholar]
- Ellisman M. H., Agnew W. S., Miller J. A., Levinson S. R. Electron microscopic visualization of the tetrodotoxin-binding protein from Electrophorus electricus. Proc Natl Acad Sci U S A. 1982 Jul;79(14):4461–4465. doi: 10.1073/pnas.79.14.4461. [DOI] [PMC free article] [PubMed] [Google Scholar]
- Fox J. M. Ultra-slow inactivation of the ionic currents through the membrane of myelinated nerve. Biochim Biophys Acta. 1976 Mar 5;426(2):232–244. doi: 10.1016/0005-2736(76)90334-5. [DOI] [PubMed] [Google Scholar]
- HODGKIN A. L., HUXLEY A. F. A quantitative description of membrane current and its application to conduction and excitation in nerve. J Physiol. 1952 Aug;117(4):500–544. doi: 10.1113/jphysiol.1952.sp004764. [DOI] [PMC free article] [PubMed] [Google Scholar]
- Hille B., Campbell D. T. An improved vaseline gap voltage clamp for skeletal muscle fibers. J Gen Physiol. 1976 Mar;67(3):265–293. doi: 10.1085/jgp.67.3.265. [DOI] [PMC free article] [PubMed] [Google Scholar]
- McLaughlin S., Poo M. M. The role of electro-osmosis in the electric-field-induced movement of charged macromolecules on the surfaces of cells. Biophys J. 1981 Apr;34(1):85–93. doi: 10.1016/S0006-3495(81)84838-2. [DOI] [PMC free article] [PubMed] [Google Scholar]
- Neumcke B., Fox J. M., Drouin H., Schwarz W. Kinetics of the slow variation of peak sodium current in the membrane of myelinated nerve following changes of holding potential or extracellular pH. Biochim Biophys Acta. 1976 Mar 5;426(2):245–257. doi: 10.1016/0005-2736(76)90335-7. [DOI] [PubMed] [Google Scholar]
- Orida N., Poo M. M. Electrophoretic movement and localisation of acetylcholine receptors in the embryonic muscle cell membrane. Nature. 1978 Sep 7;275(5675):31–35. doi: 10.1038/275031a0. [DOI] [PubMed] [Google Scholar]
- Peganov E. M., Khodorov B. I., Shishkova L. D. Medlennaia natrievaia inaktivatsiia v membrane perekhvata Ranve. Rol' naruzhnogo kaliia. Biull Eksp Biol Med. 1973 Sep;76(9):15–19. [PubMed] [Google Scholar]
- Poo M. M., Poo W. J., Lam J. W. Lateral electrophoresis and diffusion of Concanavalin A receptors in the membrane of embryonic muscle cell. J Cell Biol. 1978 Feb;76(2):483–501. doi: 10.1083/jcb.76.2.483. [DOI] [PMC free article] [PubMed] [Google Scholar]
- Poo M. In situ electrophoresis of membrane components. Annu Rev Biophys Bioeng. 1981;10:245–276. doi: 10.1146/annurev.bb.10.060181.001333. [DOI] [PubMed] [Google Scholar]
- Rudy B. Inactivation in Myxicola giant axons responsible for slow and accumulative adaptation phenomena. J Physiol. 1981 Mar;312:531–549. doi: 10.1113/jphysiol.1981.sp013642. [DOI] [PMC free article] [PubMed] [Google Scholar]
- Rudy B. Slow inactivation of the sodium conductance in squid giant axons. Pronase resistance. J Physiol. 1978 Oct;283:1–21. doi: 10.1113/jphysiol.1978.sp012485. [DOI] [PMC free article] [PubMed] [Google Scholar]
- Schauf C. L., Pencek T. L., Davis F. A. Slow sodium inactivation in Myxicola axons. Evidence for a second inactive state. Biophys J. 1976 Jul;16(7):771–778. doi: 10.1016/S0006-3495(76)85727-X. [DOI] [PMC free article] [PubMed] [Google Scholar]
- Stühmer W., Almers W. Photobleaching through glass micropipettes: sodium channels without lateral mobility in the sarcolemma of frog skeletal muscle. Proc Natl Acad Sci U S A. 1982 Feb;79(3):946–950. doi: 10.1073/pnas.79.3.946. [DOI] [PMC free article] [PubMed] [Google Scholar]
- Zagyansky Y. A., Jard S. Does lectin-receptor complex formation produce zones of restricted mobility within the membrane? Nature. 1979 Aug 16;280(5723):591–593. doi: 10.1038/280591a0. [DOI] [PubMed] [Google Scholar]