Abstract
The rate of unidirectional D-[14C]glucose efflux from human red blood cells was determined at self-exchange and net-efflux conditions by means of the Millipore-Swinnex filtering technique and the rapid continuous flow tube technique with which initial rates can be measured within fractions of a second. Determinations at 38, 25 and 10 degrees C of the concentration dependence of glucose self-exchange flux and net efflux showed that both self exchange and net efflux followed simple Michaelis-Menten kinetics at all temperatures. At 38 degrees C the maximal self-exchange flux and the maximal net efflux were identical (6 X 10(-10) mol/cm2.sec). The cellular glucose concentration for half-maximal flux (K1/2) was 6.7 mM for self exchange and 8.2 mM for net efflux. By lowering temperature the maximal glucose self-exchange flux progressively exceeded the maximal net efflux, and was about three times larger at 10 degrees C. K1/2 for self exchange increased to 12.6 mM at 10 degrees C, while K1/2 for net efflux decreased to 4.4 mM. At 38 degrees C the glucose permeability at self exchange at a constant extracellular glucose concentration of 40 mM showed a bell-shaped pH dependence between pH 6 and pH 9. A maximum was found at pH 7.2, whereas the apparent permeability coefficient was halved both at pH 6 and pH 9. The temperature dependence of glucose transport was determined between 47 and 0 degrees C at a cellular glucose concentration of 100 mM which ensured greater than 85% saturation of the glucose transport system within the temperature range. The Arrhenius activation energy of glucose transport was not constant. By lowering the temperature, the activation energy increased gradually for net efflux from 55 kJ/mole between 38 and 47 degrees C to 151 kJ/mole between 0 and 10 degrees C. The temperature dependence of self-exchange flux showed a more pronounced change around 10 degrees C. The Arrhenius activation energy was found to be 61 kJ/mole above and 120 kJ/mole below 10 degrees C.
Full text
PDF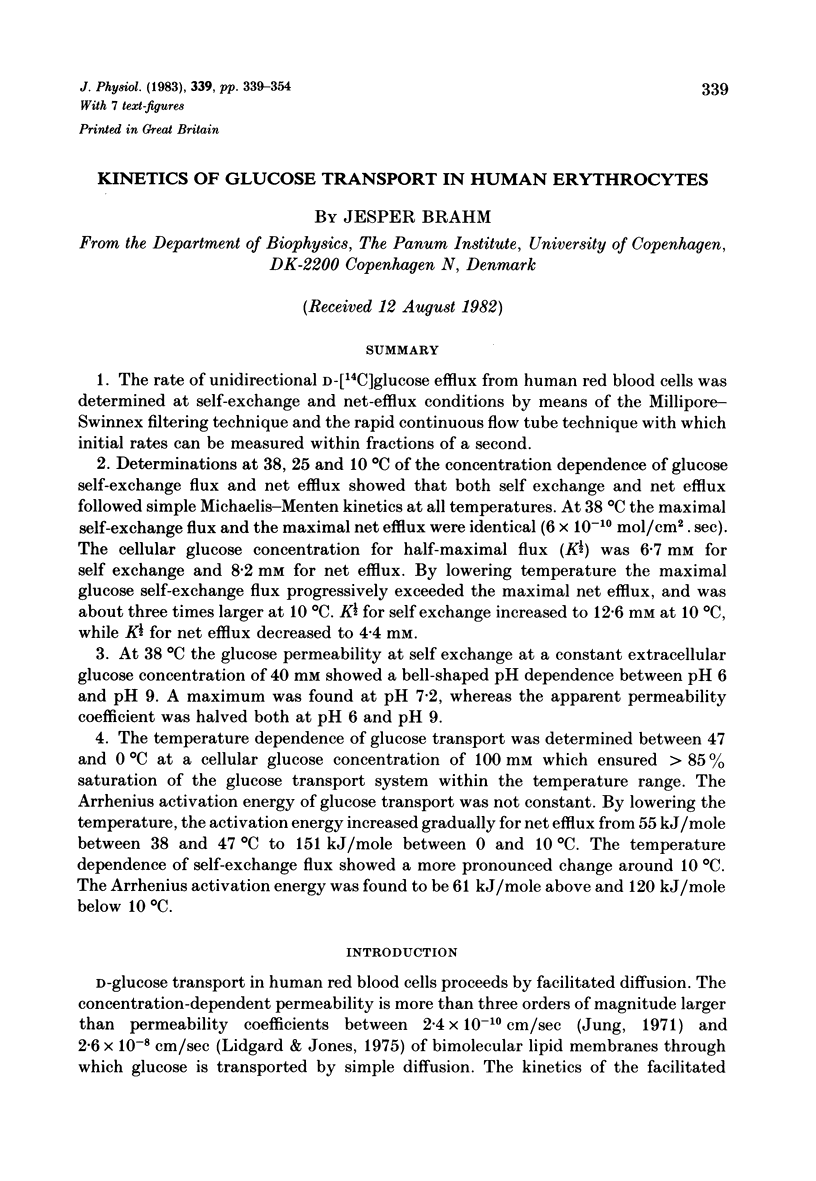
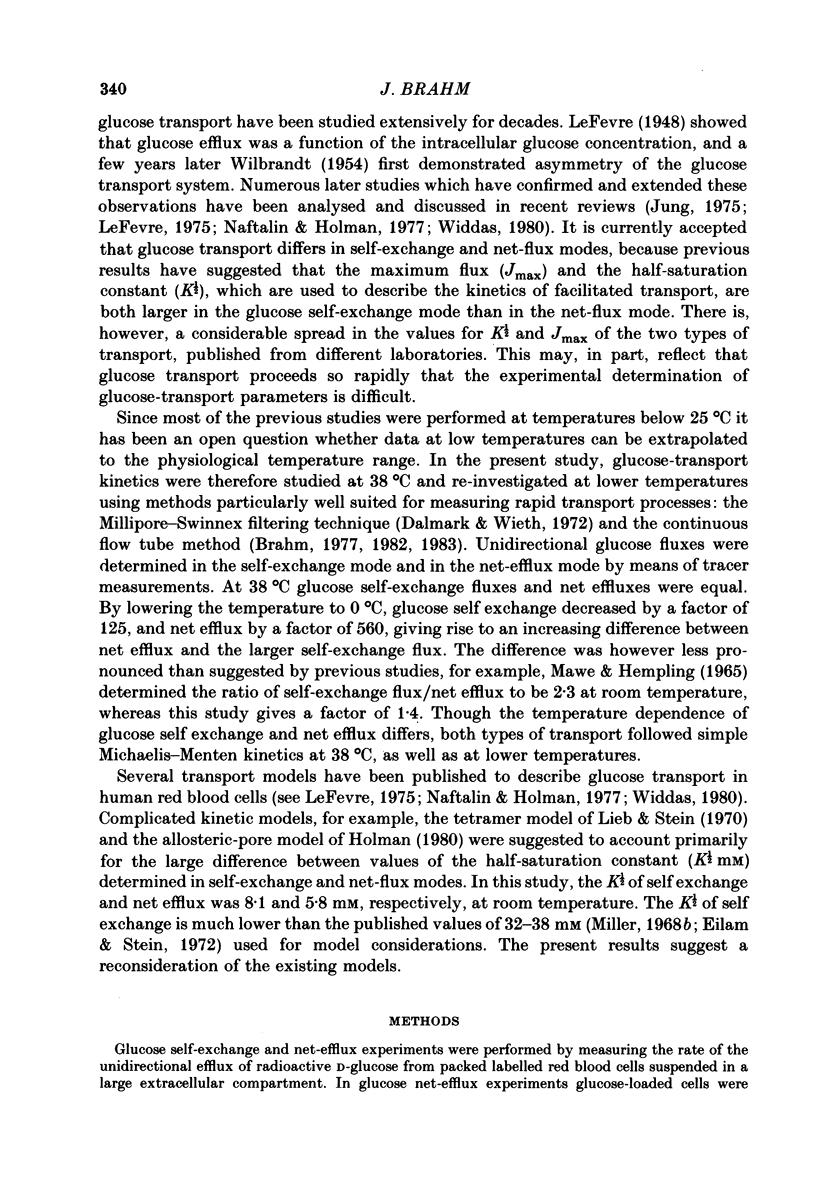
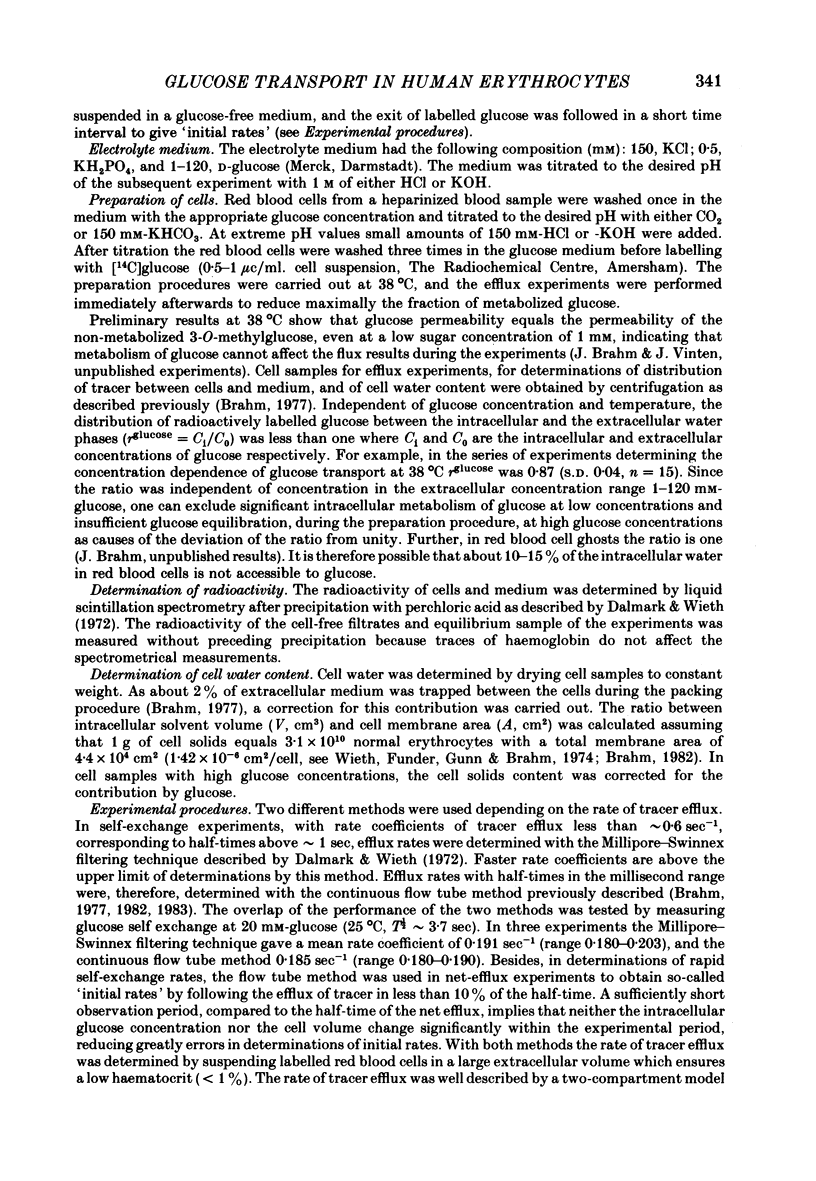
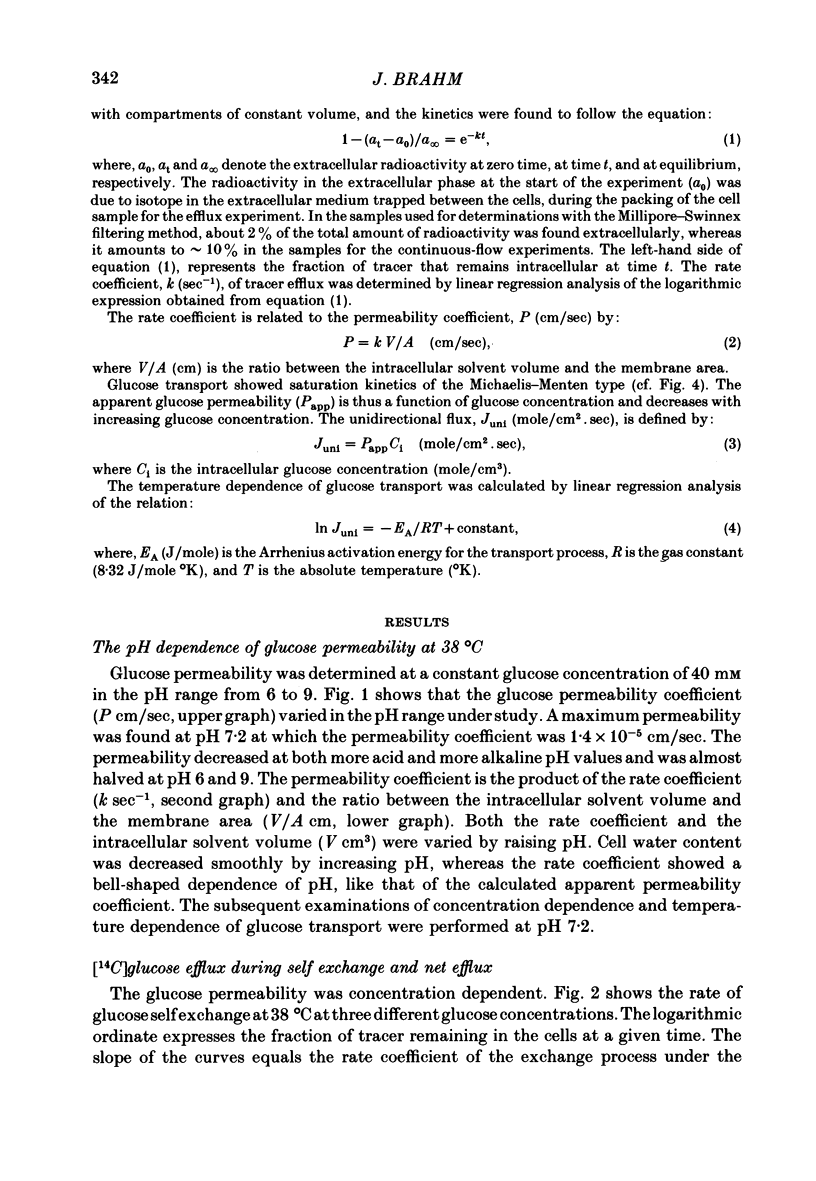
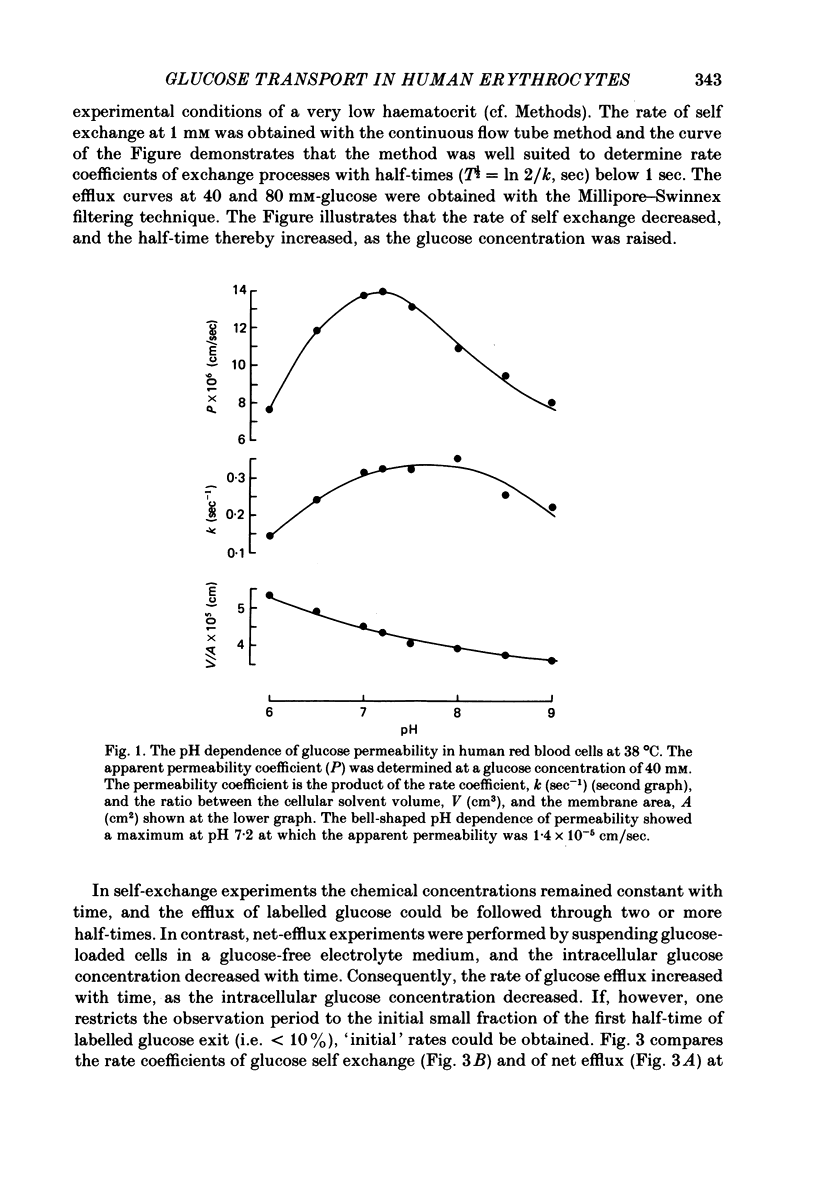
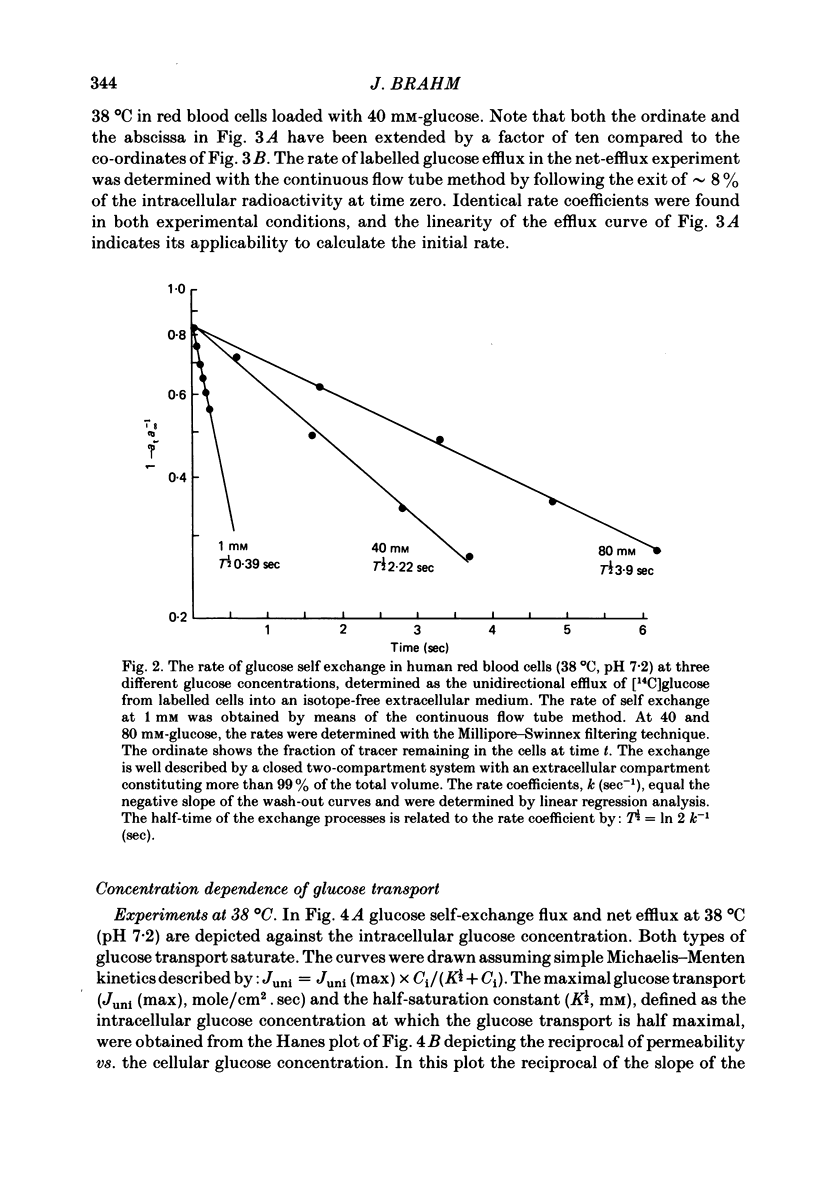
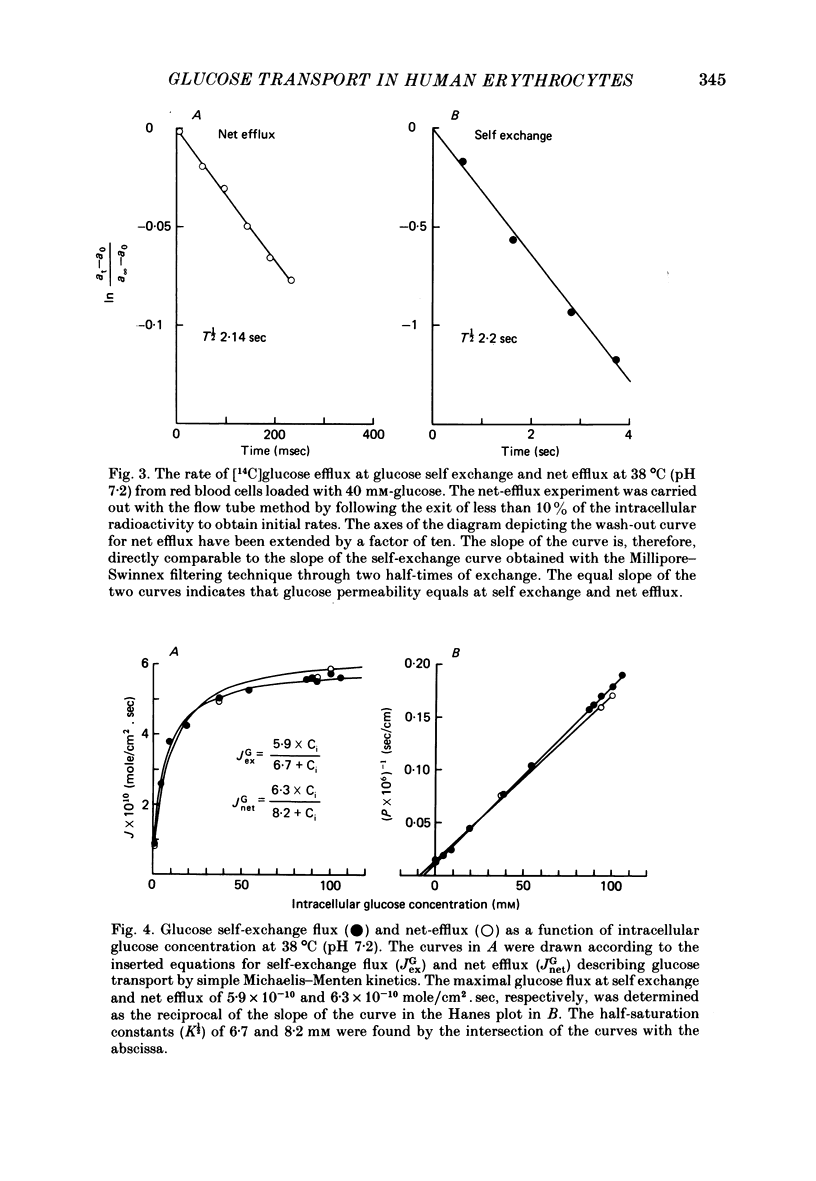
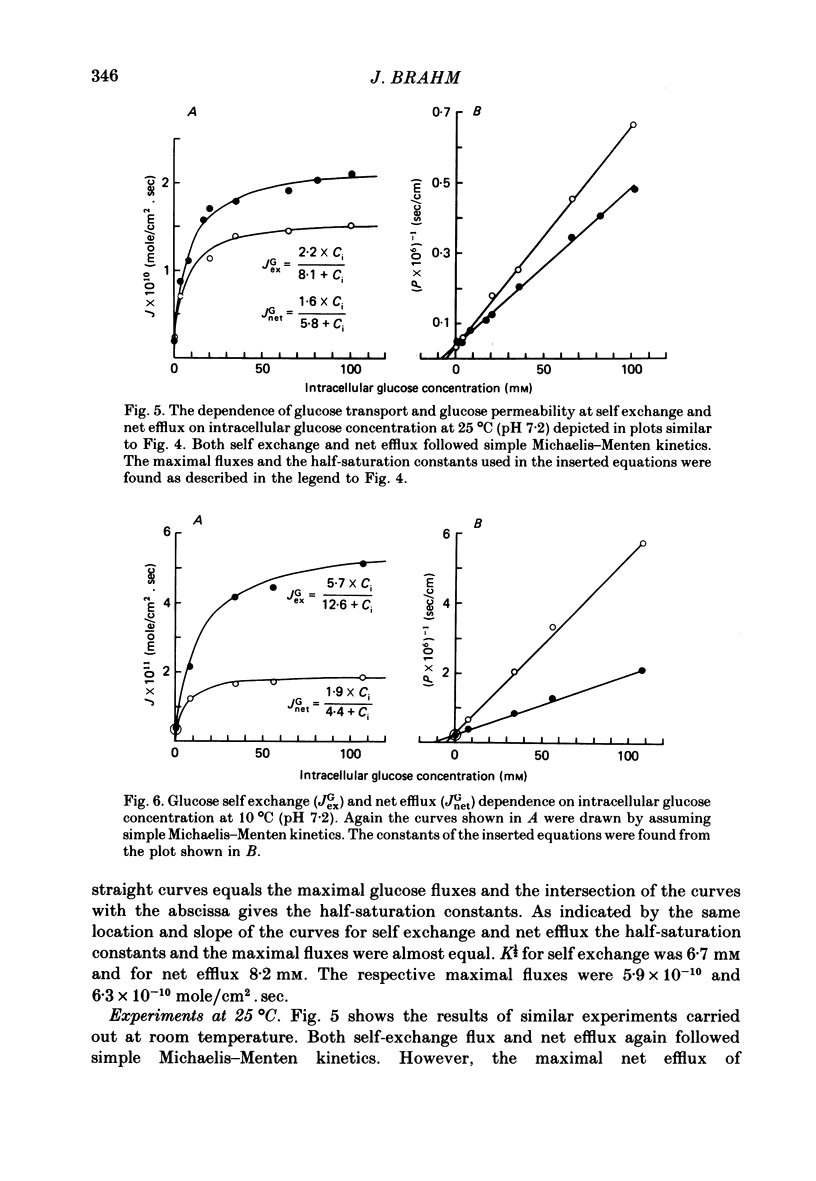
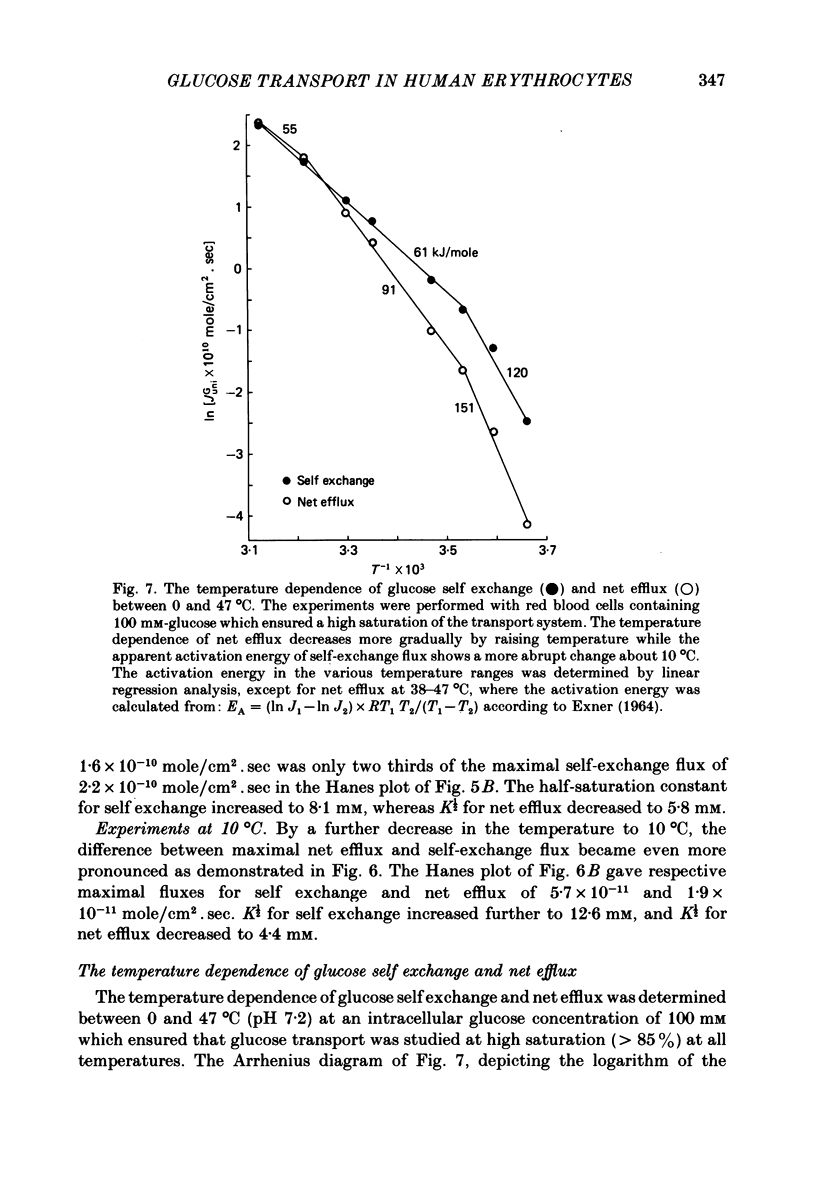
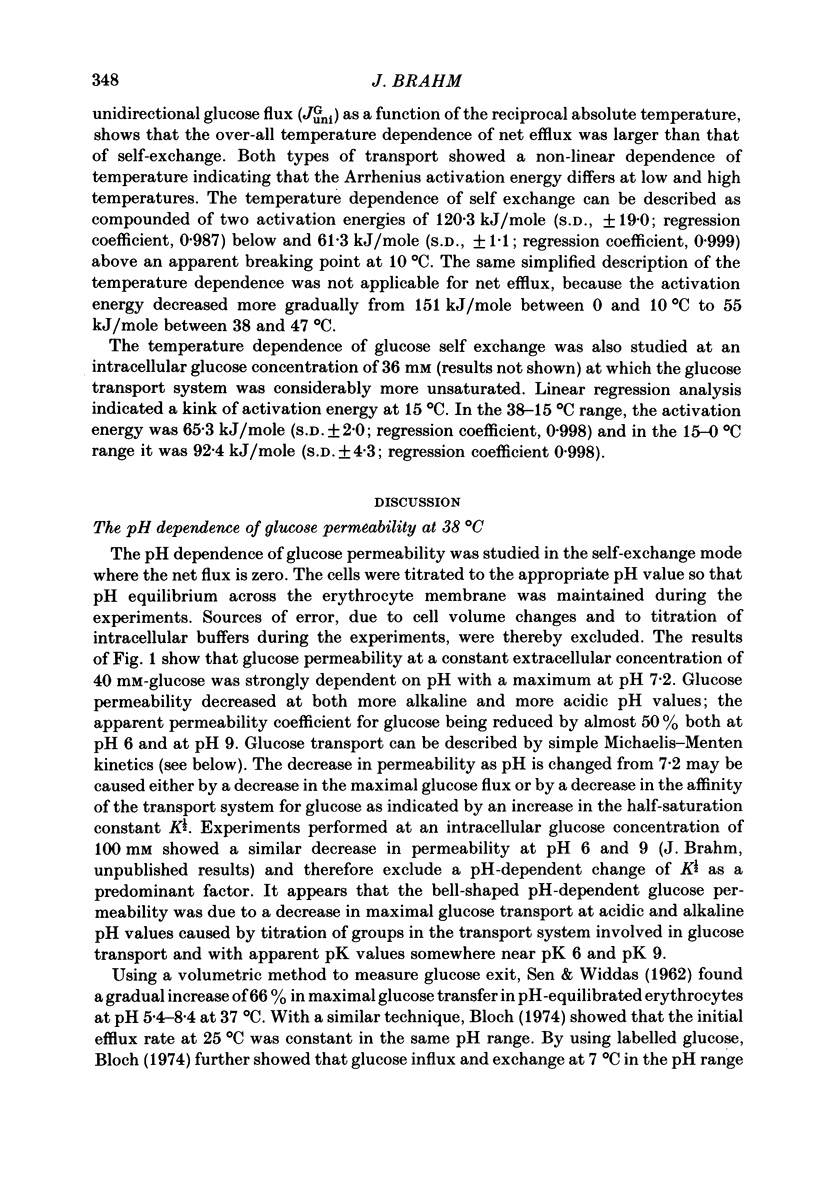
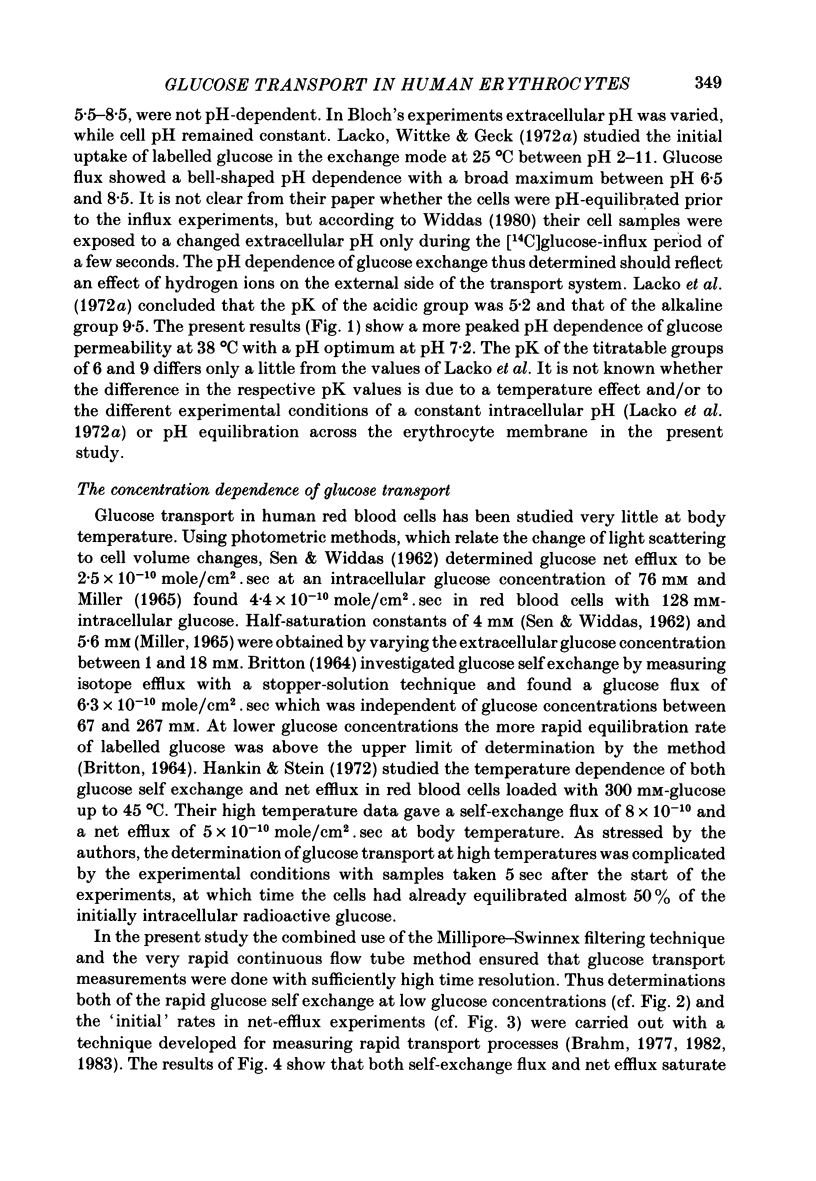
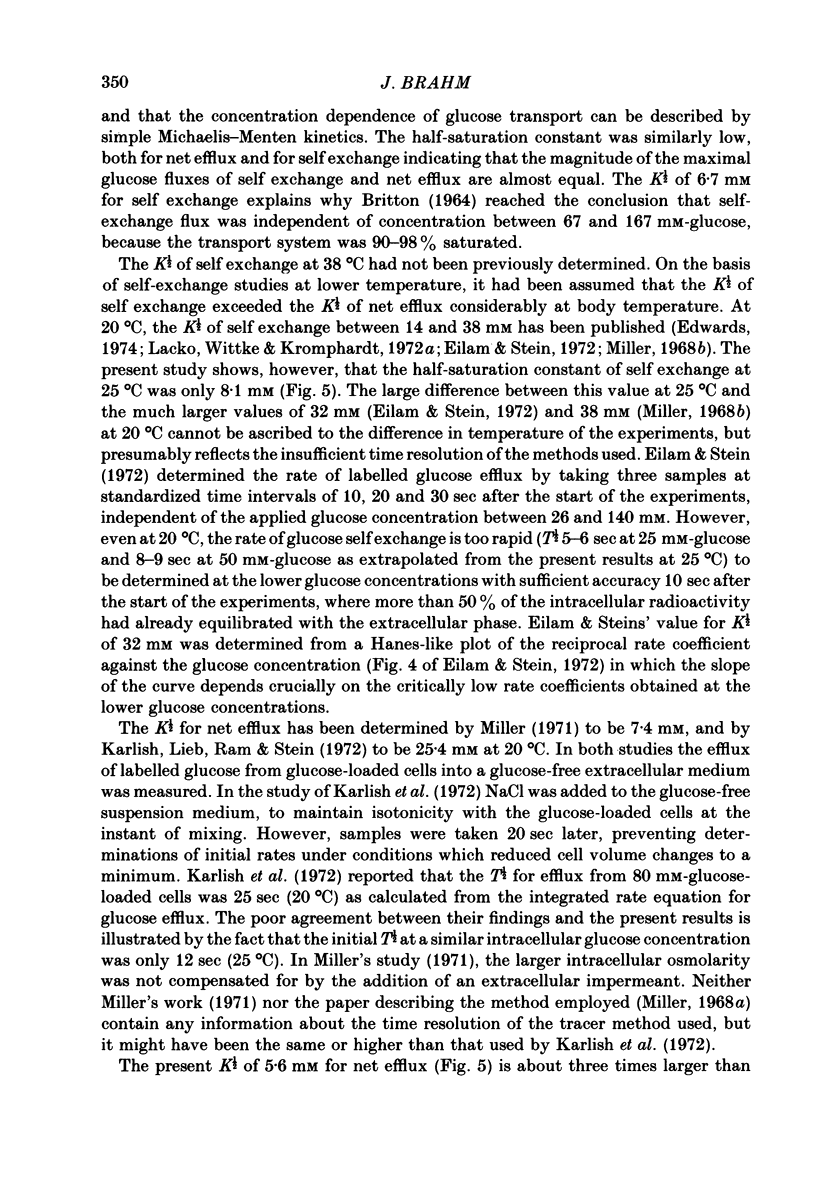
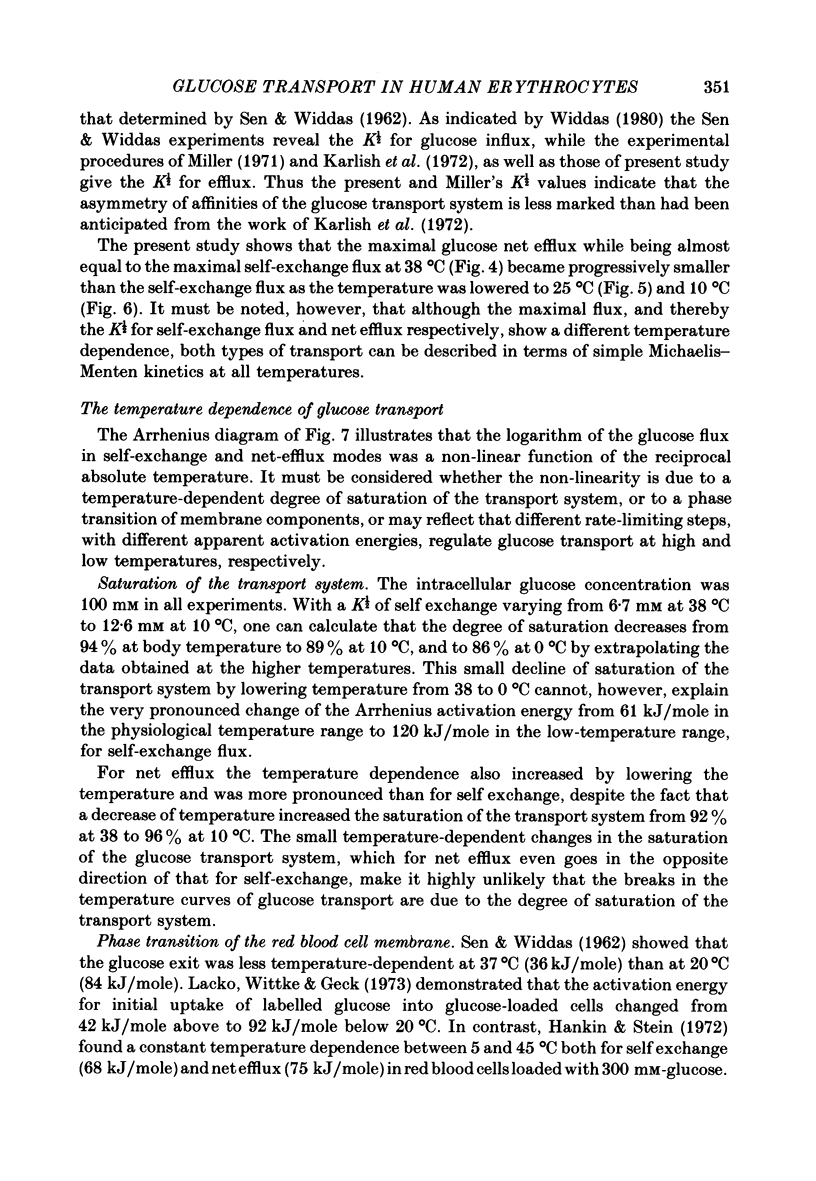
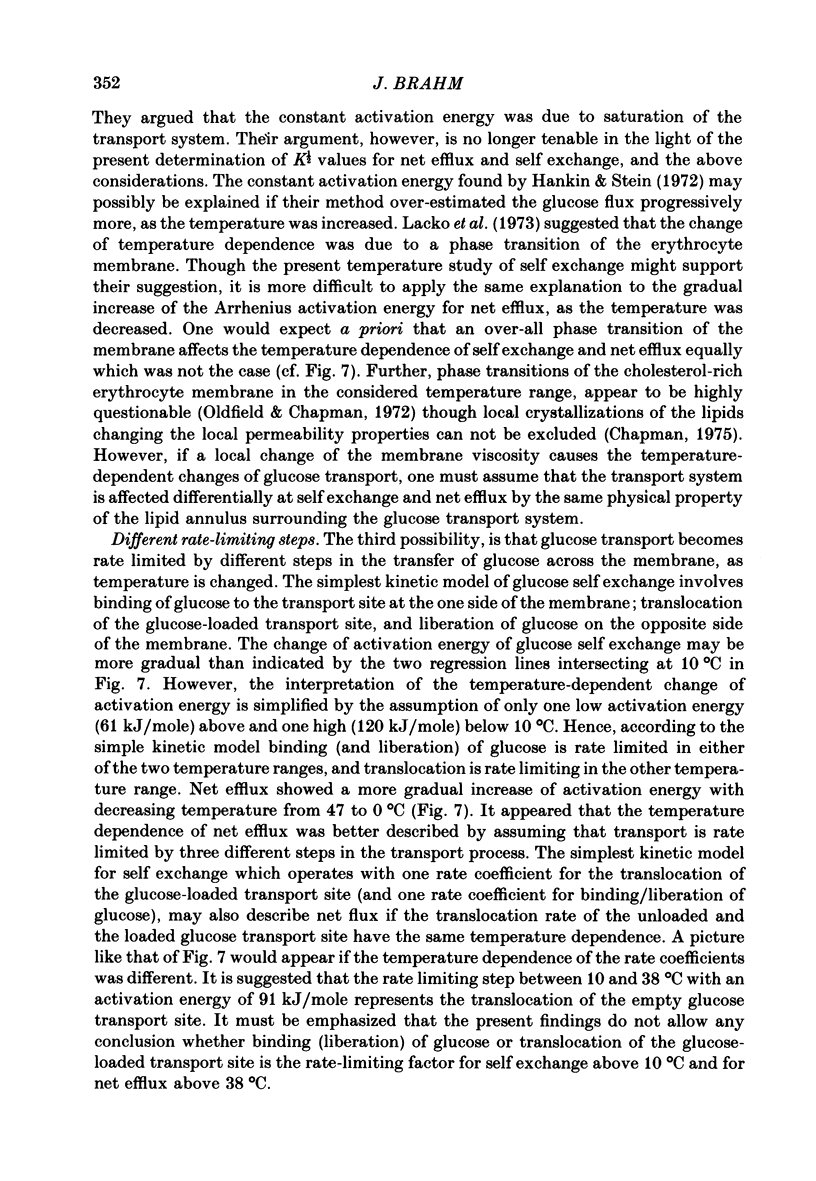
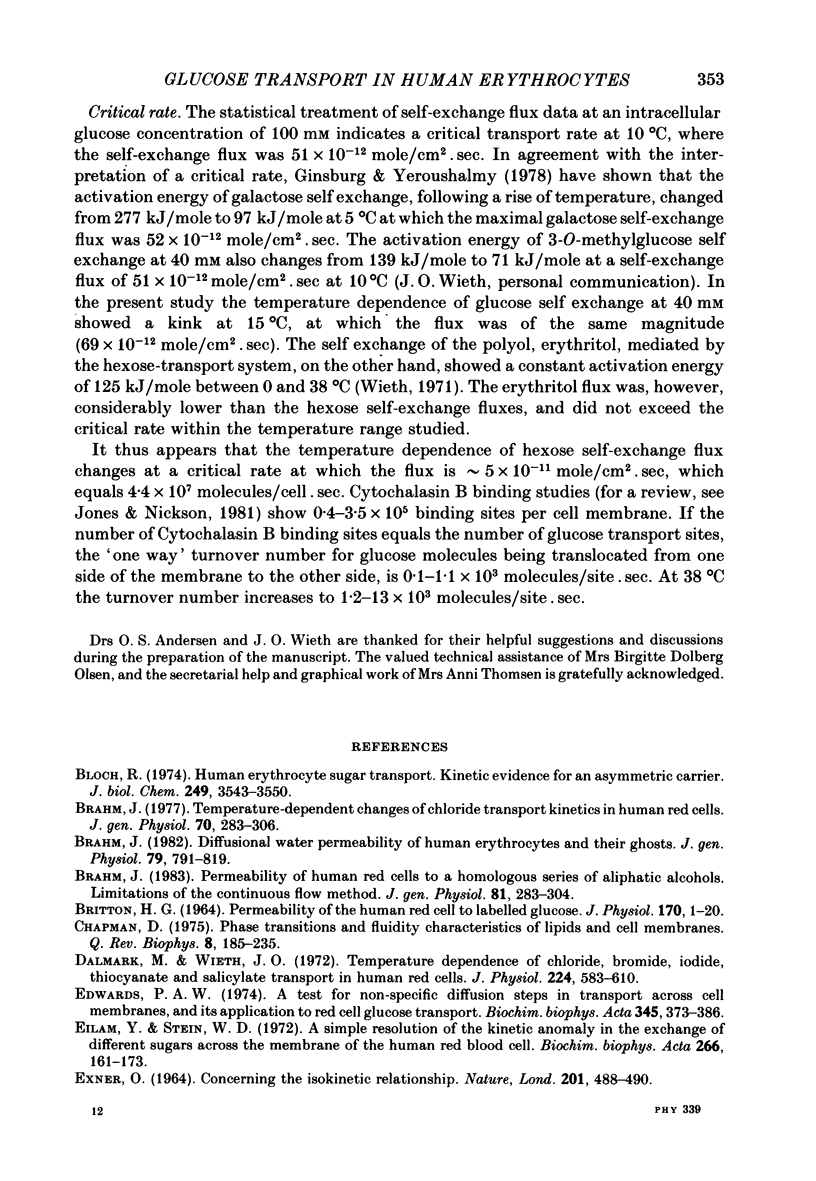
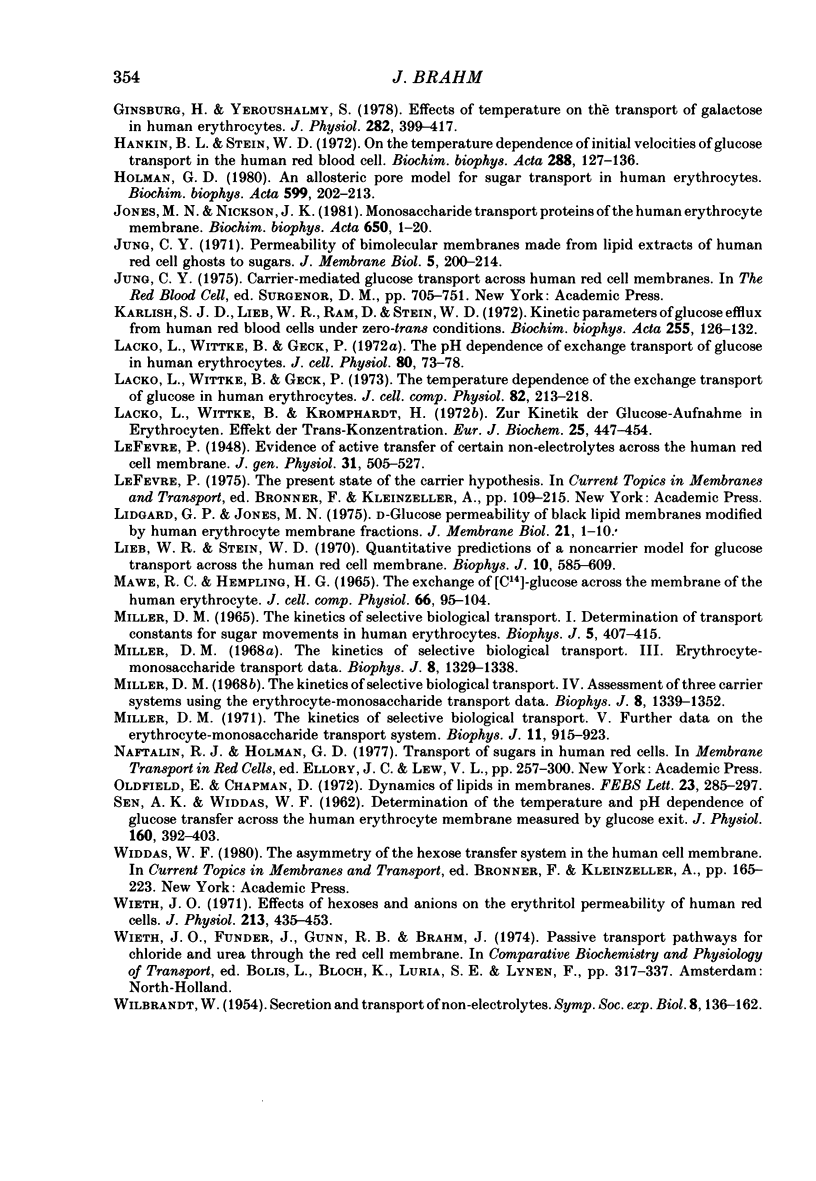
Selected References
These references are in PubMed. This may not be the complete list of references from this article.
- BRITTON H. G. PERMEABILITY OF THE HUMAN RED CELL TO LABELLED GLUCOSE. J Physiol. 1964 Jan;170:1–20. doi: 10.1113/jphysiol.1964.sp007310. [DOI] [PMC free article] [PubMed] [Google Scholar]
- Bloch R. Human erythrocyte sugar transport. Kinetic evidence for an asymmetric carrier. J Biol Chem. 1974 Jun 10;249(11):3543–3550. [PubMed] [Google Scholar]
- Brahm J. Diffusional water permeability of human erythrocytes and their ghosts. J Gen Physiol. 1982 May;79(5):791–819. doi: 10.1085/jgp.79.5.791. [DOI] [PMC free article] [PubMed] [Google Scholar]
- Brahm J. Permeability of human red cells to a homologous series of aliphatic alcohols. Limitations of the continuous flow-tube method. J Gen Physiol. 1983 Feb;81(2):283–304. doi: 10.1085/jgp.81.2.283. [DOI] [PMC free article] [PubMed] [Google Scholar]
- Brahm J. Temperature-dependent changes of chloride transport kinetics in human red cells. J Gen Physiol. 1977 Sep;70(3):283–306. doi: 10.1085/jgp.70.3.283. [DOI] [PMC free article] [PubMed] [Google Scholar]
- Chapman D. Phase transitions and fluidity characteristics of lipids and cell membranes. Q Rev Biophys. 1975 May;8(2):185–235. doi: 10.1017/s0033583500001797. [DOI] [PubMed] [Google Scholar]
- Dalmark M., Wieth J. O. Temperature dependence of chloride, bromide, iodide, thiocyanate and salicylate transport in human red cells. J Physiol. 1972 Aug;224(3):583–610. doi: 10.1113/jphysiol.1972.sp009914. [DOI] [PMC free article] [PubMed] [Google Scholar]
- Eilam Y., Stein W. D. A simple resolution of the kinetic anomaly in the exchange of different sugars across the membrane of the human red blood cell. Biochim Biophys Acta. 1972 Apr 14;266(1):161–173. doi: 10.1016/0005-2736(72)90132-0. [DOI] [PubMed] [Google Scholar]
- Ginsburg H., Yeroushalmy S. Effects of temperature on the transport of galactose in human erythrocytes. J Physiol. 1978 Sep;282:399–417. doi: 10.1113/jphysiol.1978.sp012471. [DOI] [PMC free article] [PubMed] [Google Scholar]
- Hankin B. L., Stein W. D. On the temperature dependence of initial velocities of glucose transport in the human red blood cell. Biochim Biophys Acta. 1972 Oct 23;288(1):127–136. doi: 10.1016/0005-2736(72)90230-1. [DOI] [PubMed] [Google Scholar]
- Holman G. D. An allosteric pore model for sugar transport in human erythrocytes. Biochim Biophys Acta. 1980 Jun 20;599(1):202–213. doi: 10.1016/0005-2736(80)90068-1. [DOI] [PubMed] [Google Scholar]
- Jones M. N., Nickson J. K. Monosaccharide transport proteins of the human erythrocyte membrane. Biochim Biophys Acta. 1981 Jun 16;650(1):1–20. doi: 10.1016/0304-4157(81)90006-x. [DOI] [PubMed] [Google Scholar]
- Karlish S. J., Lieb W. R., Ram D., Stein W. D. Kinetic parameters of glucose efflux from human red blood cells under zero-trans conditions. Biochim Biophys Acta. 1972 Jan 17;255(1):126–132. doi: 10.1016/0005-2736(72)90014-4. [DOI] [PubMed] [Google Scholar]
- Lacko L., Wittke B., Geck P. The pH dependence of exchange transport of glucose in human erythrocytes. J Cell Physiol. 1972 Aug;80(1):73–78. doi: 10.1002/jcp.1040800109. [DOI] [PubMed] [Google Scholar]
- Lacko L., Wittke B., Geck P. The temperature dependence of the exchange transport of glucose in human erythrocytes. J Cell Physiol. 1973 Oct;82(2):213–218. doi: 10.1002/jcp.1040820209. [DOI] [PubMed] [Google Scholar]
- Lacko L., Wittke B., Kromphardt H. Zur Kinetik der Glucose-Aufnahme in Erythrocyten. Effekt der Trans-Konzentraion. Eur J Biochem. 1972 Feb;25(3):447–454. doi: 10.1111/j.1432-1033.1972.tb01714.x. [DOI] [PubMed] [Google Scholar]
- LeFEVRE P. G. Evidence of active transfer of certain non-electrolytes across the human red cell membrane. J Gen Physiol. 1948 Jul 20;31(6):505–527. doi: 10.1085/jgp.31.6.505. [DOI] [PMC free article] [PubMed] [Google Scholar]
- Lidgard G. P., Jones M. N. D-glucose permeability of black lipid membranes modified by human erythrocyte membrane fractions. J Membr Biol. 1975 Apr 23;21(1-2):1–10. doi: 10.1007/BF01941058. [DOI] [PubMed] [Google Scholar]
- Lieb W. R., Stein W. D. Quantitative predictions of a noncarrier model for glucose transport across the human red cell membrane. Biophys J. 1970 Jul;10(7):585–609. doi: 10.1016/s0006-3495(70)86322-6. [DOI] [PMC free article] [PubMed] [Google Scholar]
- Mawe R. C., Hempling H. G. The exchange of C14 glucose across the membrane of the human erythrocyte. J Cell Physiol. 1965 Aug;66(1):95–103. doi: 10.1002/jcp.1030660110. [DOI] [PubMed] [Google Scholar]
- Miller D. M. The kinetics of selective biological transport. 3. Erythrocyte-monosaccharide transport data. Biophys J. 1968 Nov;8(11):1329–1338. doi: 10.1016/s0006-3495(68)86559-2. [DOI] [PMC free article] [PubMed] [Google Scholar]
- Miller D. M. The kinetics of selective biological transport. I. Determination of transport constants for sugar movements in human erythrocytes. Biophys J. 1965 Jul;5(4):407–415. doi: 10.1016/S0006-3495(65)86725-X. [DOI] [PMC free article] [PubMed] [Google Scholar]
- Miller D. M. The kinetics of selective biological transport. IV. Assessment of three carrier systems using the erythrocyte-monosaccharide transport data. Biophys J. 1968 Nov;8(11):1339–1352. doi: 10.1016/S0006-3495(68)86560-9. [DOI] [PMC free article] [PubMed] [Google Scholar]
- Miller D. M. The kinetics of selective biological transport. V. Further data on the erythrocyte-monosaccharide transport system. Biophys J. 1971 Nov;11(11):915–923. doi: 10.1016/S0006-3495(71)86263-X. [DOI] [PMC free article] [PubMed] [Google Scholar]
- Oldfield E., Chapman D. Dynamics of lipids in membranes: Heterogeneity and the role of cholesterol. FEBS Lett. 1972 Jul 1;23(3):285–297. doi: 10.1016/0014-5793(72)80300-4. [DOI] [PubMed] [Google Scholar]
- SEN A. K., WIDDAS W. F. Determination of the temperature and pH dependence of glucose transfer across the human erythrocyte membrane measured by glucose exit. J Physiol. 1962 Mar;160:392–403. doi: 10.1113/jphysiol.1962.sp006854. [DOI] [PMC free article] [PubMed] [Google Scholar]
- Wieth J. O. Effects of hexoses and anions on the erythritol permeability of human red cells. J Physiol. 1971 Mar;213(2):435–453. doi: 10.1113/jphysiol.1971.sp009392. [DOI] [PMC free article] [PubMed] [Google Scholar]