Abstract
The deoxygenation rates of transformed erythrocytes were compared with those of normal discocytes by both stopped-flow and continuous-flow methods. Echinocytic and spherostomatocytic transformations were induced by various anionic and cationic drugs, respectively, without altering the oxygen affinity of haemoglobin, the cell volume or the membrane fluidity. The echinocytic transformation reduced the deoxygenation rate at slow-flow velocities (50 cm/sec), as detected by the continuous-flow method. However, at higher flow velocities (150 cm/sec) the rate was similar to that seen in normal discocytes. A close correlation between the degree of echinocytosis, the retardation of deoxygenation rate and the increase of suspension viscosity were observed. Microscopic observation of flowing erythrocytes revealed that the echinocytes scarcely deformed at the slower flow velocity, but clearly deformed at the higher flow velocity to various shapes resembling the flowing discocytes. Transformation to spherostomatocytes had no effect on the deoxygenation rate, which was comparable with that of the discocytes, and even the higher flow force did not induce any deformation. The retarded deoxygenation and the increased viscosity of echinocytes was probably due to an augmented stagnant layer around the cells (i.e. an increase of the hydrodynamic effective volume); this layer was reduced when the echinocytes were deformed with increasing flow force.
Full text
PDF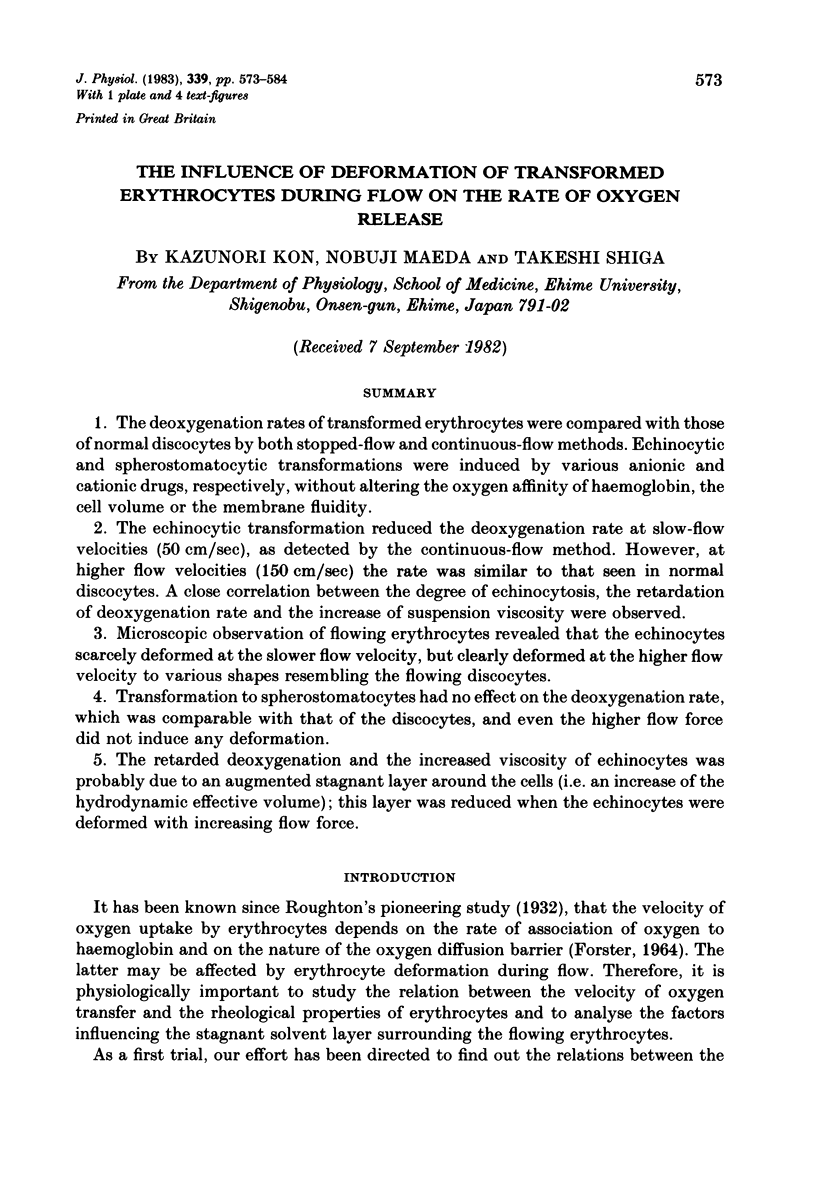
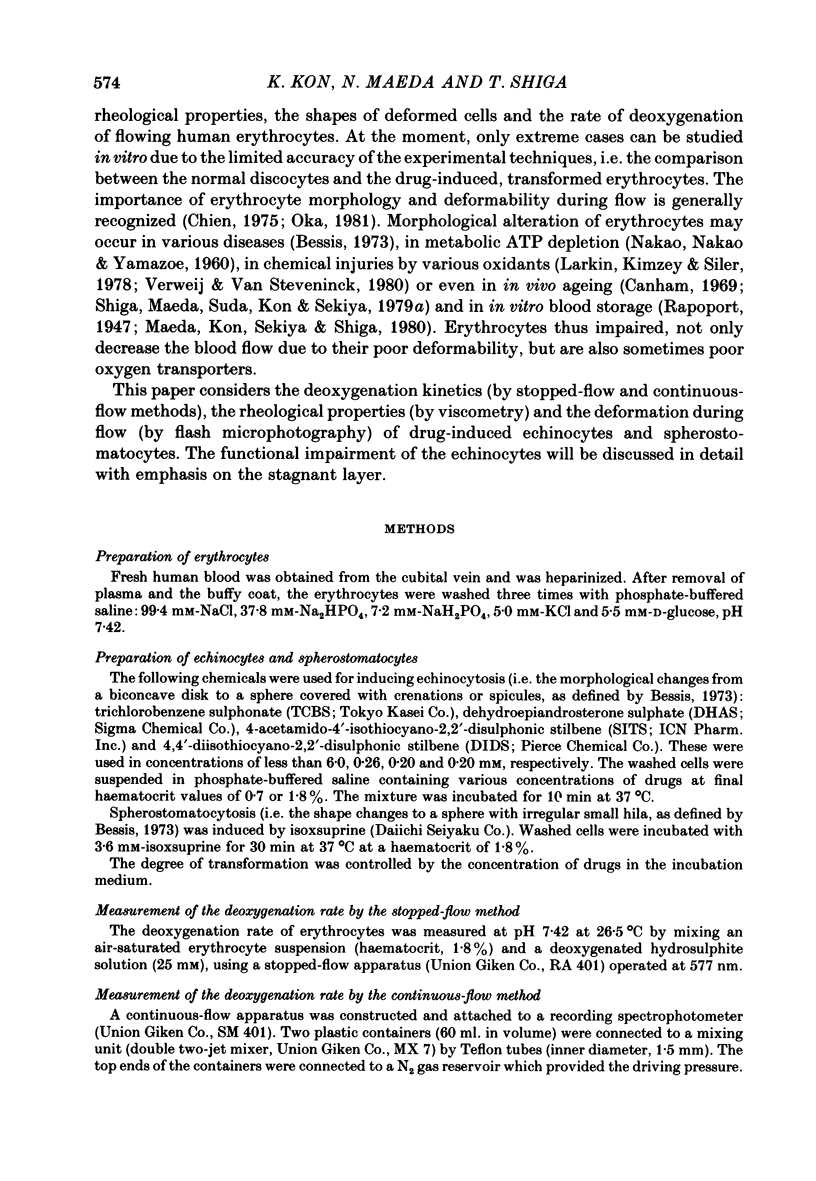
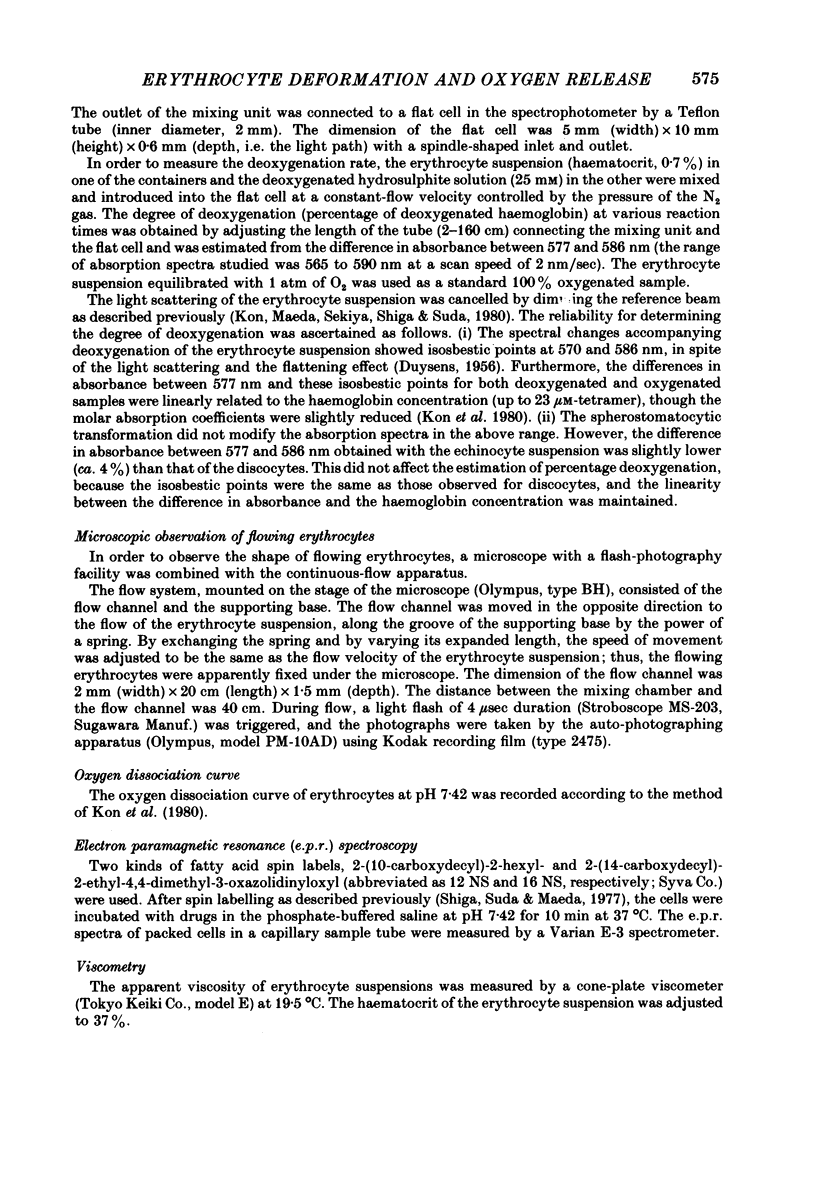
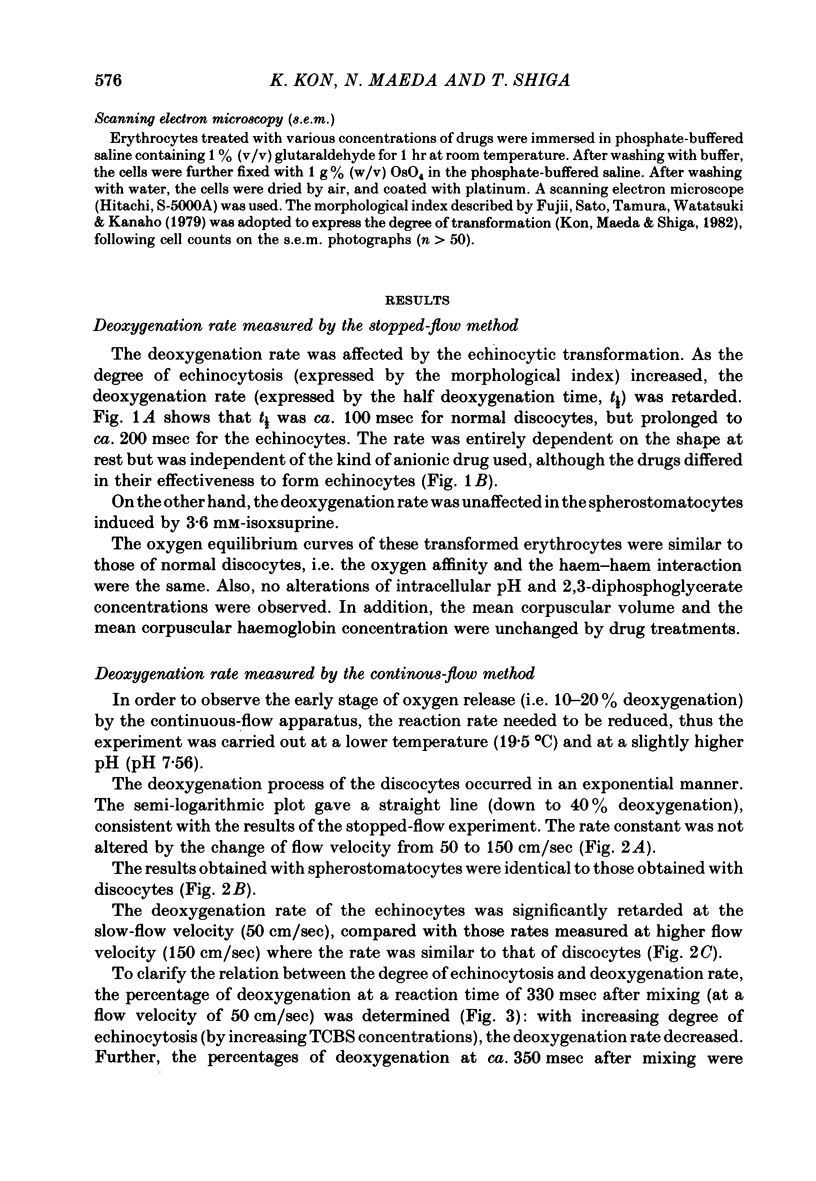
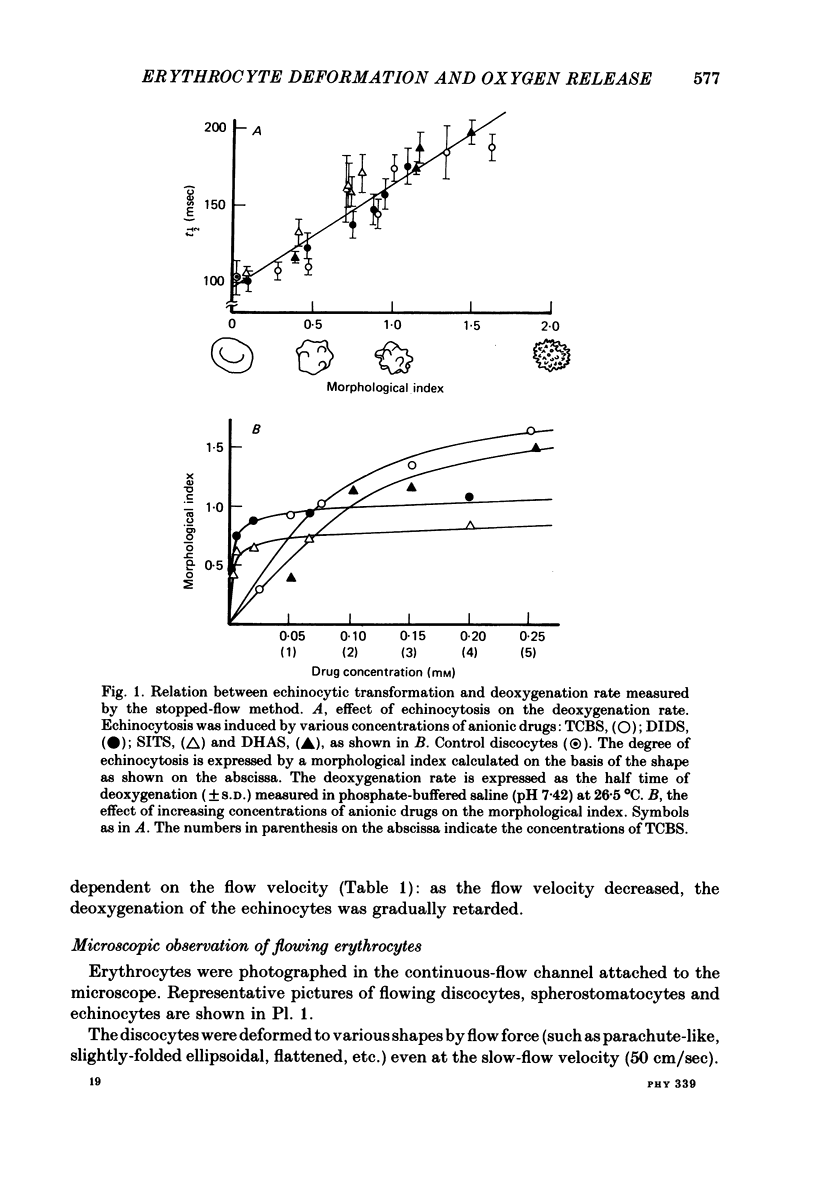
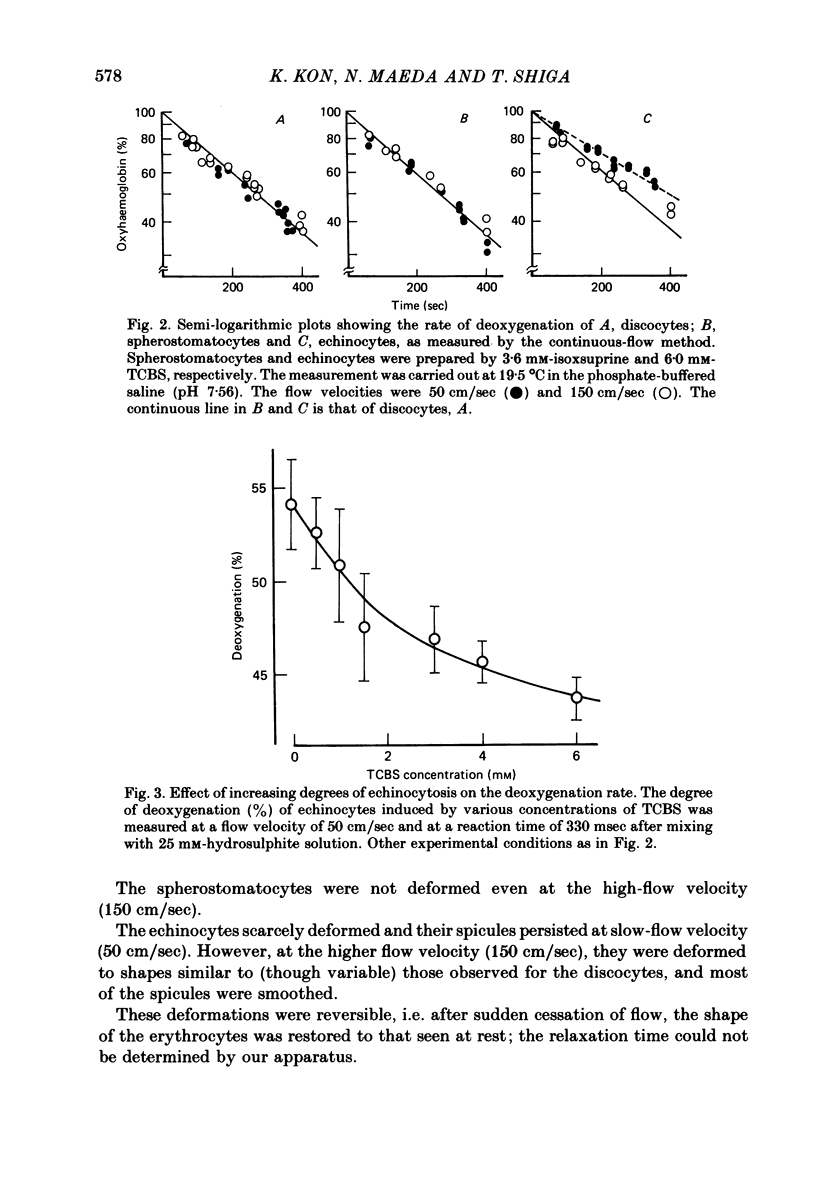
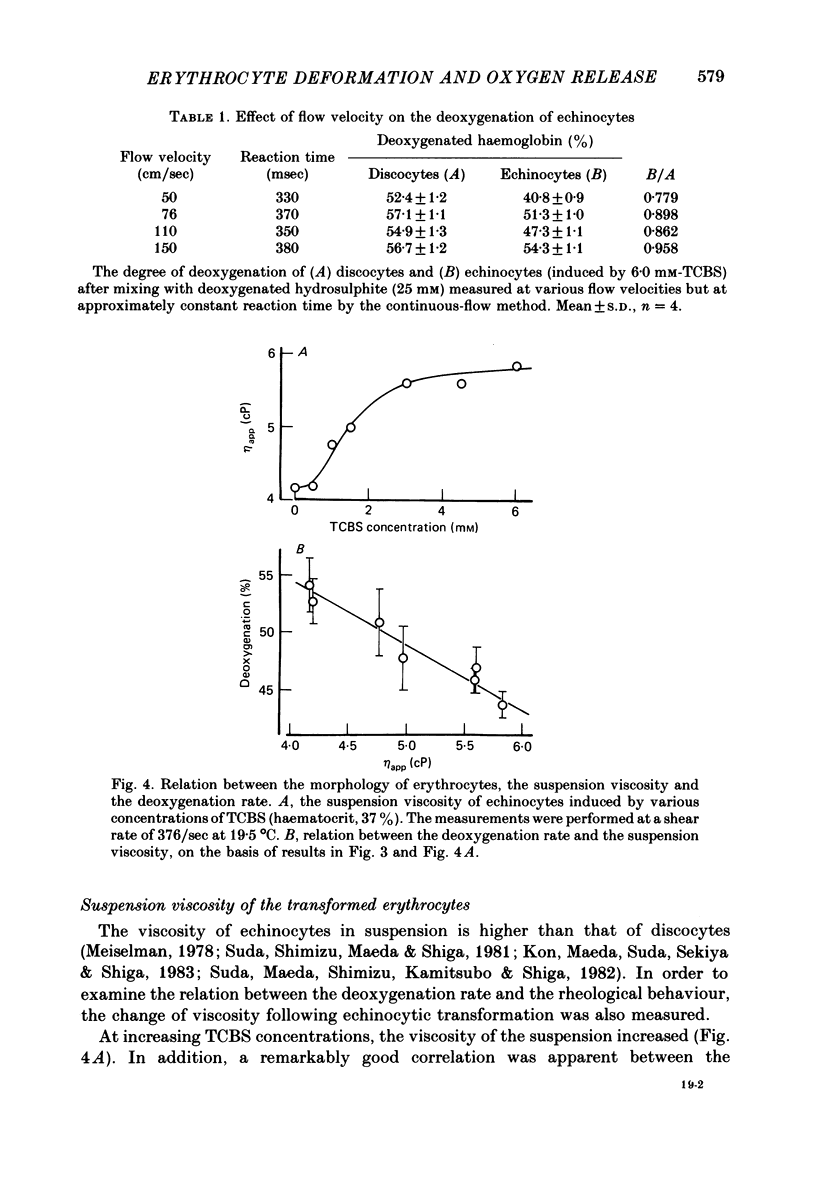
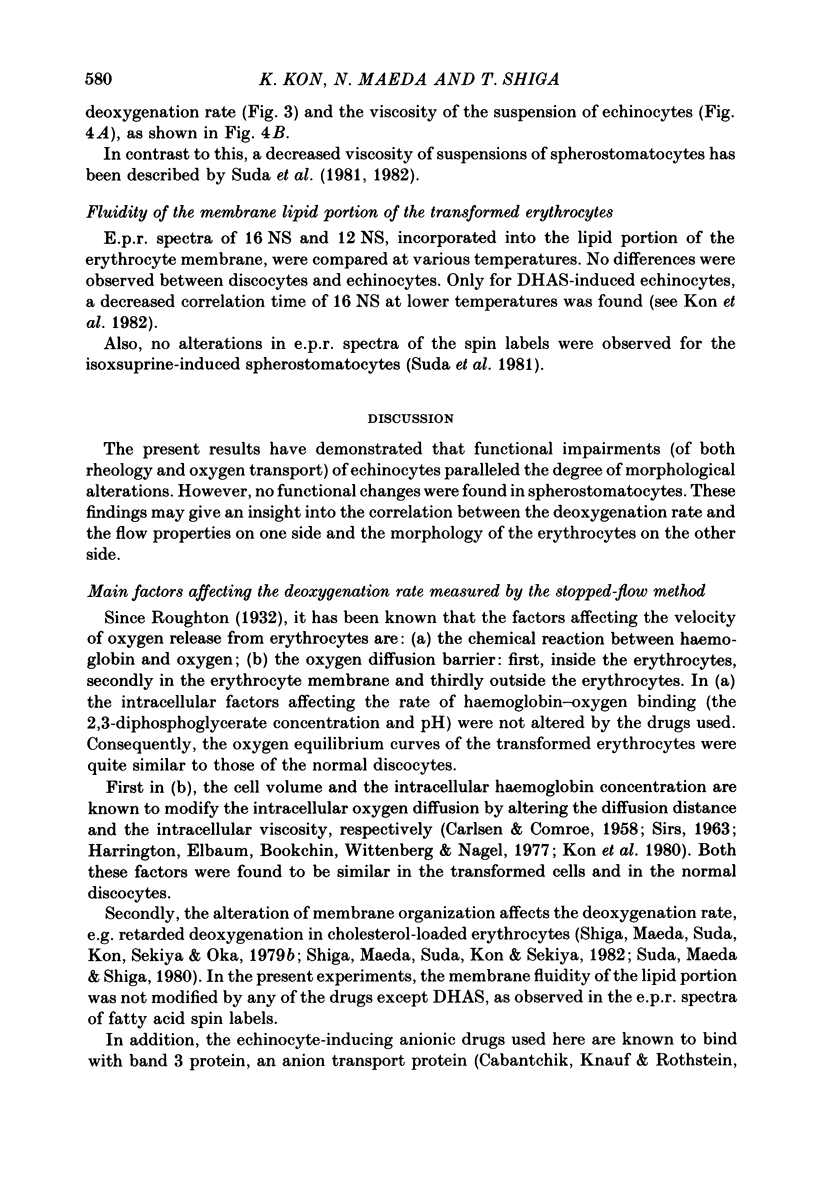
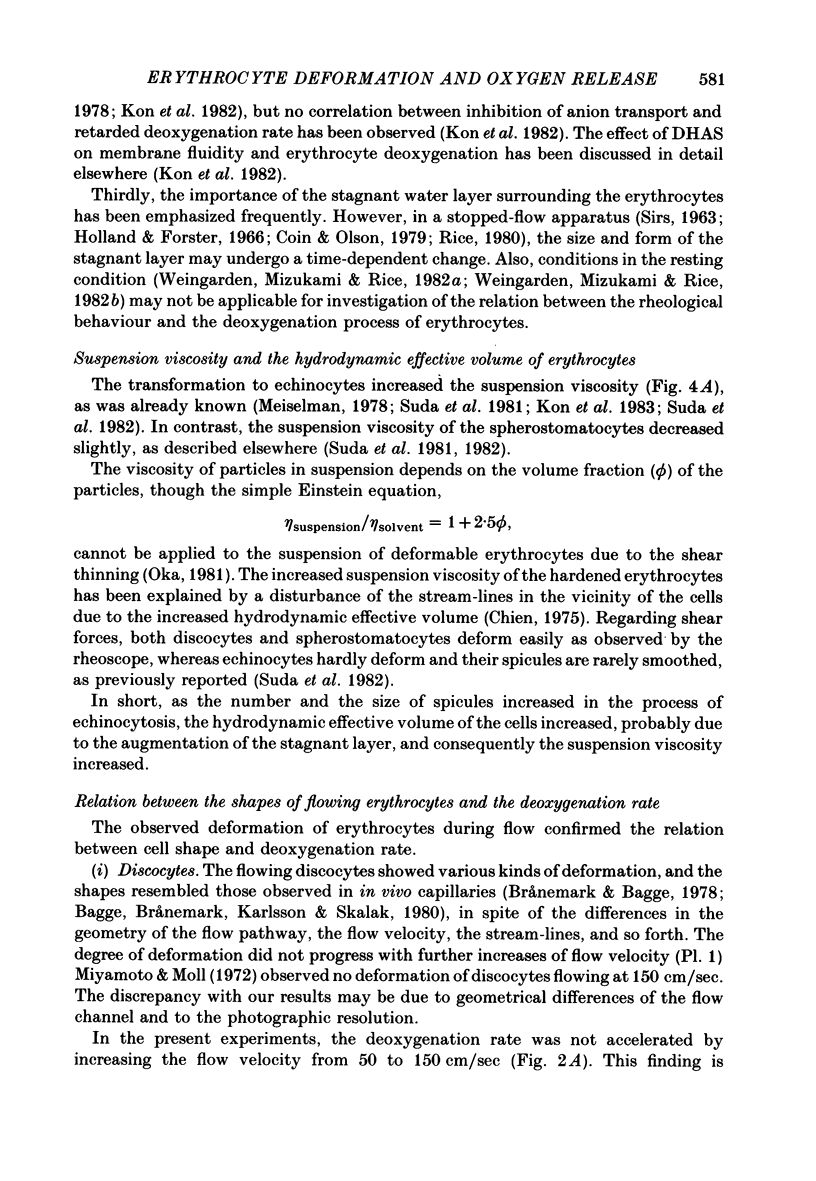
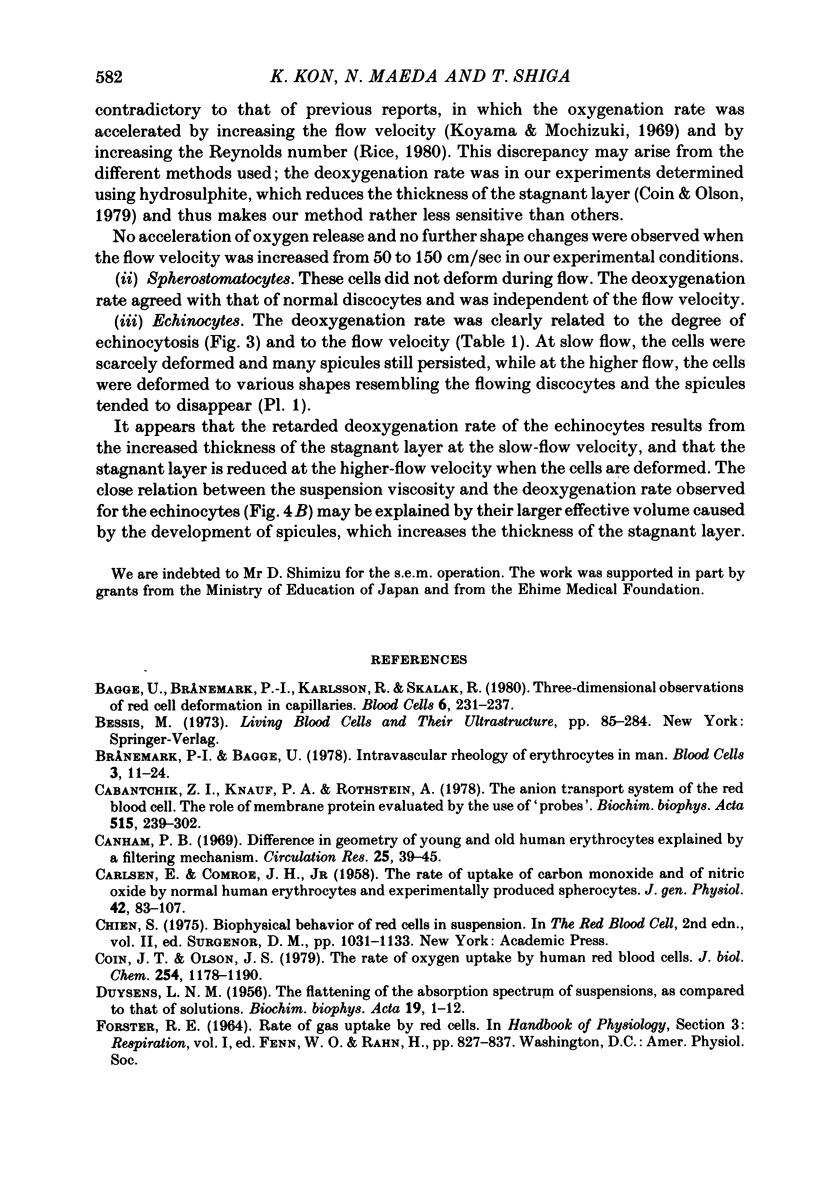
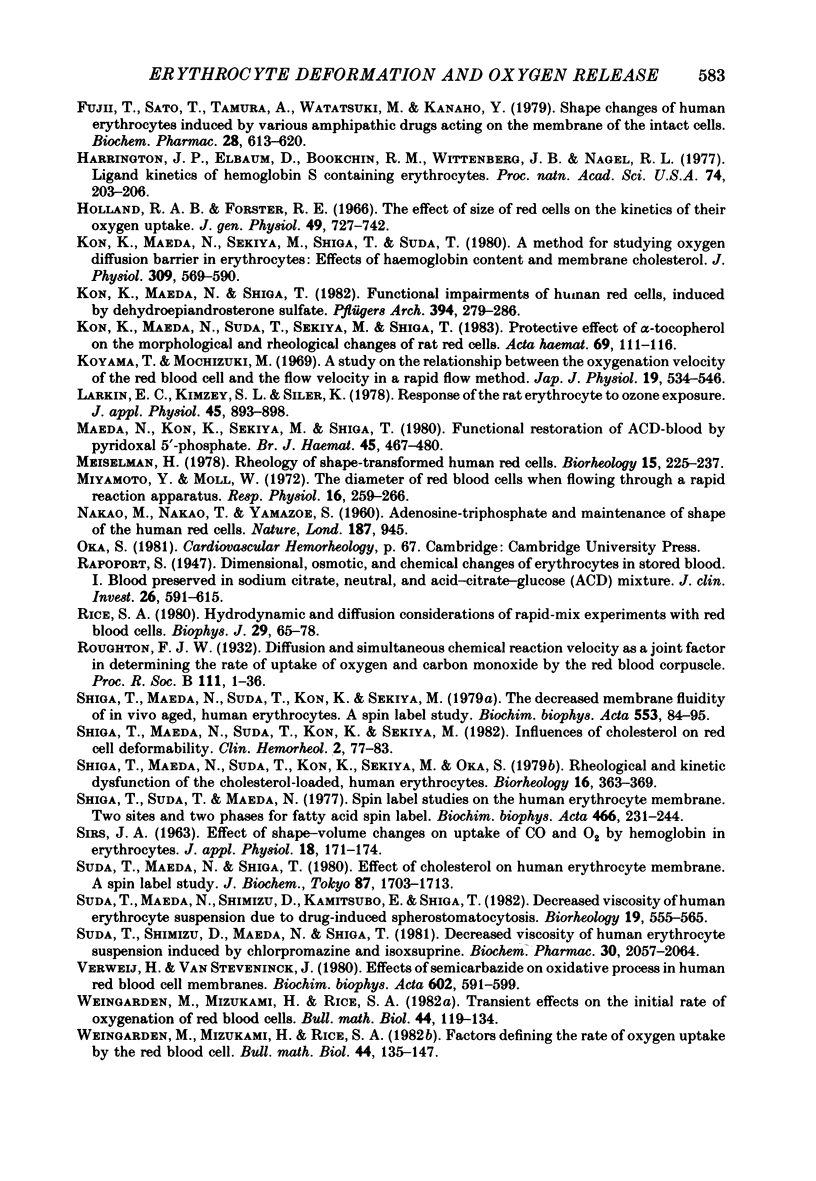
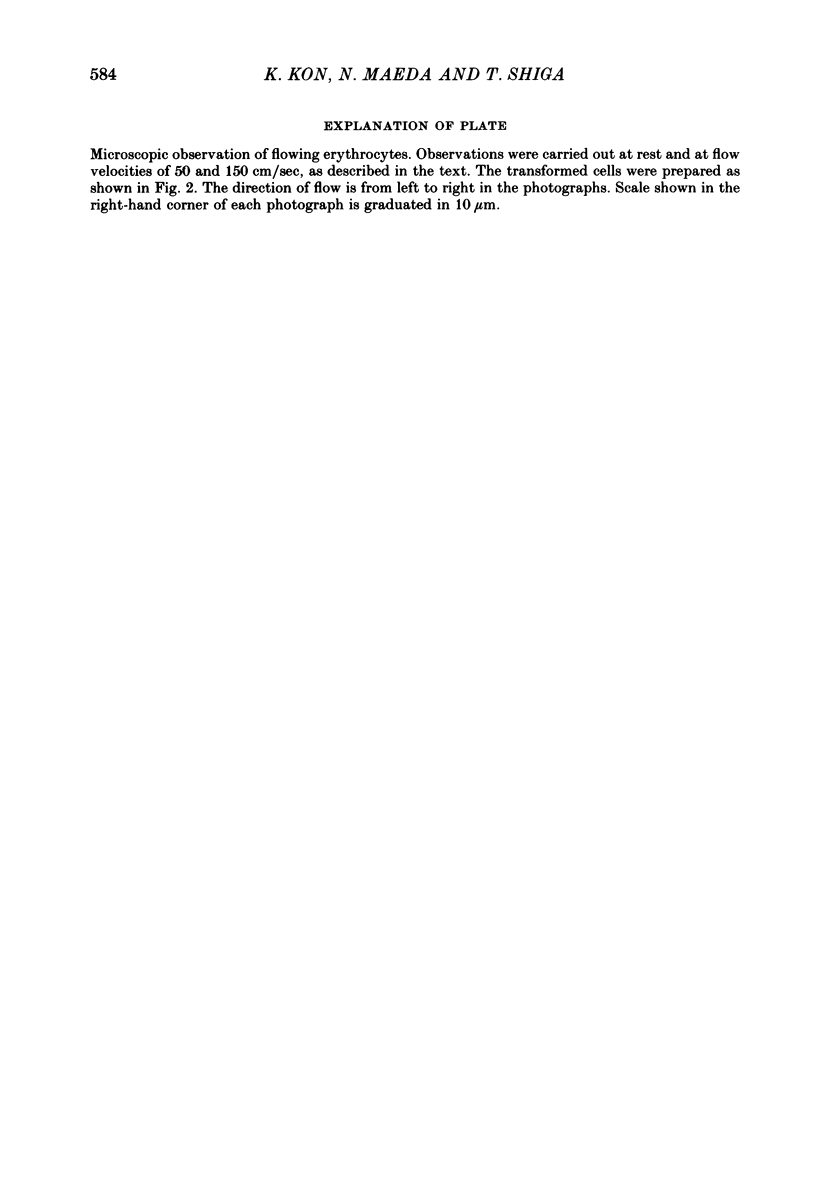
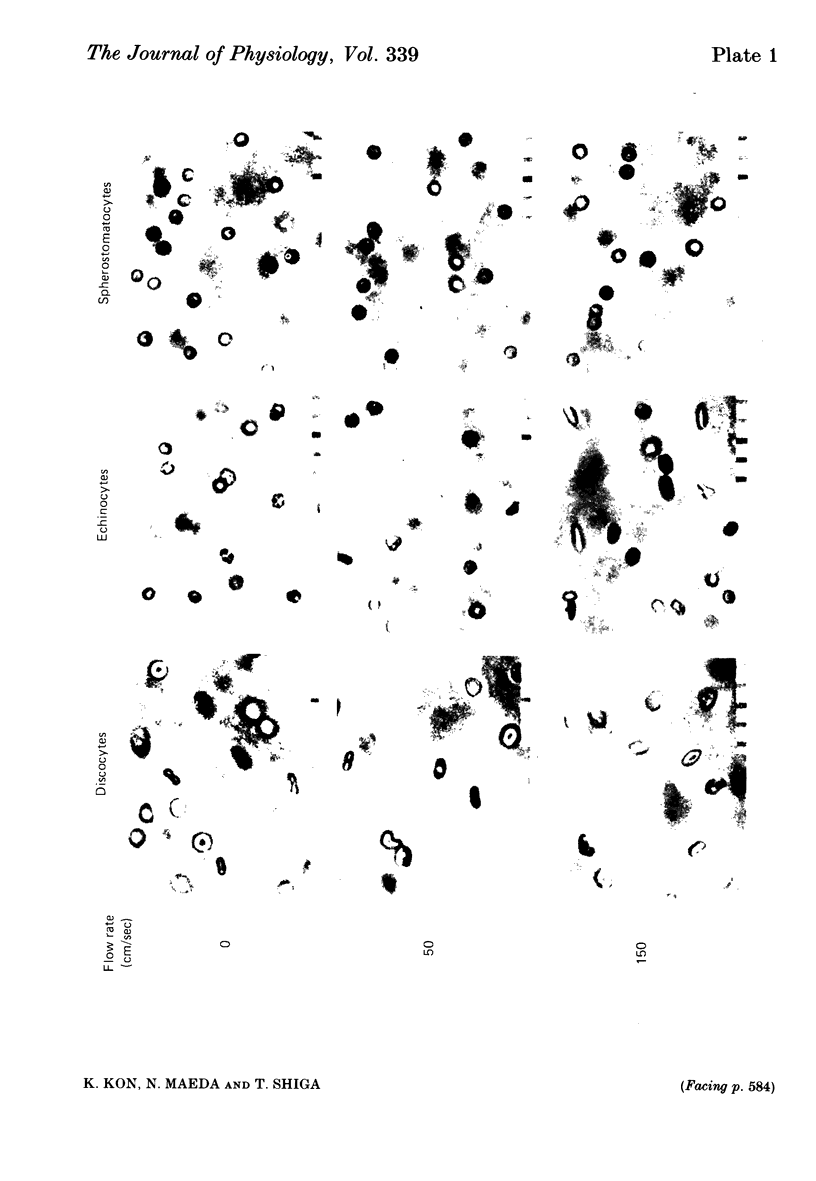
Images in this article
Selected References
These references are in PubMed. This may not be the complete list of references from this article.
- Bagge U., Brånemark P. I., Karlsson R., Skalak R. Three-dimensional observations of red blood cell deformation in capillaries. Blood Cells. 1980;6(2):231–239. [PubMed] [Google Scholar]
- CARLSEN E., COMROE J. H., Jr The rate of uptake of carbon monoxide and of nitric oxide by normal human erythrocytes and experimentally produced spherocytes. J Gen Physiol. 1958 Sep 20;42(1):83–107. doi: 10.1085/jgp.42.1.83. [DOI] [PMC free article] [PubMed] [Google Scholar]
- Cabantchik Z. I., Knauf P. A., Rothstein A. The anion transport system of the red blood cell. The role of membrane protein evaluated by the use of 'probes'. Biochim Biophys Acta. 1978 Sep 29;515(3):239–302. doi: 10.1016/0304-4157(78)90016-3. [DOI] [PubMed] [Google Scholar]
- Canham P. B. Difference in geometry of young and old human erythrocytes explained by a filtering mechanism. Circ Res. 1969 Jul;25(1):39–45. doi: 10.1161/01.res.25.1.39. [DOI] [PubMed] [Google Scholar]
- Carvounis C. P., Carvounis G., Arbeit L. A. Role of the endogenous kallikrein-kinin system in modulating vasopressin-stimulated water flow and urea permeability in the toad urinary bladder. J Clin Invest. 1981 Jun;67(6):1792–1796. doi: 10.1172/JCI110219. [DOI] [PMC free article] [PubMed] [Google Scholar]
- Coin J. T., Olson J. S. The rate of oxygen uptake by human red blood cells. J Biol Chem. 1979 Feb 25;254(4):1178–1190. [PubMed] [Google Scholar]
- Colina-Chourio J., McGiff J. C., Miller M. P., Nasjletti A. Possible influence of intrarenal generation of kinins on prostaglandin release from the rabbit perfused kidney. Br J Pharmacol. 1976 Oct;58(2):165–172. doi: 10.1111/j.1476-5381.1976.tb10392.x. [DOI] [PMC free article] [PubMed] [Google Scholar]
- DUYSENS L. N. The flattening of the absorption spectrum of suspensions, as compared to that of solutions. Biochim Biophys Acta. 1956 Jan;19(1):1–12. doi: 10.1016/0006-3002(56)90380-8. [DOI] [PubMed] [Google Scholar]
- Dunn M. J., Greely H. P., Valtin H., Kintner L. B., Beeuwkes R., 3rd Renal excretion of prostaglandins E2 and F2alpha in diabetes insipidus rats. Am J Physiol. 1978 Dec;235(6):E624–E627. doi: 10.1152/ajpendo.1978.235.6.E624. [DOI] [PubMed] [Google Scholar]
- Fejes-Tóth G., Zahajszky T., Filep J. Effect of vasopressin on renal kallikrein excretion. Am J Physiol. 1980 Oct;239(4):F388–F392. doi: 10.1152/ajprenal.1980.239.4.F388. [DOI] [PubMed] [Google Scholar]
- Fujii T., Sato T., Tamura A., Wakatsuki M., Kanaho Y. Shape changes of human erythrocytes induced by various amphipathic drugs acting on the membrane of the intact cells. Biochem Pharmacol. 1979 Mar 1;28(5):613–620. doi: 10.1016/0006-2952(79)90144-8. [DOI] [PubMed] [Google Scholar]
- Gellai M., Valtin H. Chronic vascular constrictions and measurements of renal function in conscious rats. Kidney Int. 1979 Apr;15(4):419–426. doi: 10.1038/ki.1979.54. [DOI] [PubMed] [Google Scholar]
- Harrington J. P., Elbaum D., Bookchin R. M., Wittenberg J. B., Nagel R. L. Ligand kinetics of hemoglobin S containing erythrocytes. Proc Natl Acad Sci U S A. 1977 Jan;74(1):203–206. doi: 10.1073/pnas.74.1.203. [DOI] [PMC free article] [PubMed] [Google Scholar]
- Holland R. A., Forster R. E. The effect of size of red cells on the kinetics of their oxygen uptake. J Gen Physiol. 1966 Mar;49(4):727–742. doi: 10.1085/jgp.49.4.727. [DOI] [PMC free article] [PubMed] [Google Scholar]
- Kon K., Maeda N., Sekiya M., Shiga T., Suda T. A method for studying oxygen diffusion barrier in erythrocytes: effects of haemoglobin content and membrane cholesterol. J Physiol. 1980 Dec;309:569–590. doi: 10.1113/jphysiol.1980.sp013528. [DOI] [PMC free article] [PubMed] [Google Scholar]
- Kon K., Maeda N., Shiga T. Functional impairments of human red cells, induced by dehydroepiandrosterone sulfate. Pflugers Arch. 1982 Oct 1;394(4):279–286. doi: 10.1007/BF00583691. [DOI] [PubMed] [Google Scholar]
- Kon K., Maeda N., Suda T., Sekiya M., Shiga T. Protective effect of alpha-tocopherol on the morphological and rheological changes of rat red cells. Acta Haematol. 1983;69(2):111–116. doi: 10.1159/000206864. [DOI] [PubMed] [Google Scholar]
- Koyama T., Mochizuki M. A study on the relationship between the oxygenation velocity of the red blood cell and the flow velocity in a rapid flow method. Jpn J Physiol. 1969 Aug;19(4):534–546. doi: 10.2170/jjphysiol.19.534. [DOI] [PubMed] [Google Scholar]
- Larkin E. C., Kimzey S. L., Siler K. Response of the rat erythrocyte to ozone exposure. J Appl Physiol Respir Environ Exerc Physiol. 1978 Dec;45(6):893–898. doi: 10.1152/jappl.1978.45.6.893. [DOI] [PubMed] [Google Scholar]
- Maeda N., Kon K., Sekiya M., Shiga T. Functional restoration of ACD-blood by pyrodoxal 5'-phosphate. Br J Haematol. 1980 Jul;45(3):467–480. doi: 10.1111/j.1365-2141.1980.tb07166.x. [DOI] [PubMed] [Google Scholar]
- Meiselman H. J. Rheology of shape-transformed human red cells. Biorheology. 1978;15(3-4):225–237. doi: 10.3233/bir-1978-153-410. [DOI] [PubMed] [Google Scholar]
- Miyamoto Y., Moll W. The diameter of red blood cells when flowing through a rapid reaction apparatus. Respir Physiol. 1972 Oct;16(2):259–266. doi: 10.1016/0034-5687(72)90055-2. [DOI] [PubMed] [Google Scholar]
- NAKAO M., NAKAO T., YAMAZOE S. Adenosine triphosphate and maintenance of shape of the human red cells. Nature. 1960 Sep 10;187:945–946. doi: 10.1038/187945a0. [DOI] [PubMed] [Google Scholar]
- Nasjletti A., McGiff J. C., Colina-Chourio J. Interrelations of the renal kallikrein-kinin system and renal prostaglandins in the conscious rat. Influence of mineralocorticoids. Circ Res. 1978 Nov;43(5):799–807. doi: 10.1161/01.res.43.5.799. [DOI] [PubMed] [Google Scholar]
- Olsen U. B., Ahnfelt-Ronne I. Bumetanide induced increase of renal blood flow in conscious dogs and its relation to local renal hormones (PGE, kallikrein and renin). Acta Pharmacol Toxicol (Copenh) 1976 Mar;38(3):219–228. doi: 10.1111/j.1600-0773.1976.tb03114.x. [DOI] [PubMed] [Google Scholar]
- Rapoport S., Wing M. DIMENSIONAL, OSMOTIC, AND CHEMICAL CHANGES OF ERYTHROCYTES IN STORED BLOOD. I. BLOOD PRESERVED IN SODIUM CITRATE, NEUTRAL, AND ACID CITRATE-GLUCOSE (ACD) MIXTURES. J Clin Invest. 1947 Jul;26(4):591–615. doi: 10.1172/JCI101843. [DOI] [PMC free article] [PubMed] [Google Scholar]
- Rice S. A. Hydrodynamic and diffusion considerations of rapid-mix experiments with red blood cells. Biophys J. 1980 Jan;29(1):65–77. doi: 10.1016/S0006-3495(80)85118-6. [DOI] [PMC free article] [PubMed] [Google Scholar]
- SIRS J. A. Effect of shape-volume changes on uptake of CO and O2 by hemoglobin in erythrmcytes. J Appl Physiol. 1963 Jan;18:171–174. doi: 10.1152/jappl.1963.18.1.171. [DOI] [PubMed] [Google Scholar]
- Shiga T., Maeda N., Suda T., Kon K., Sekiya M., Oka S. Rheological and kinetic dysfunctions of the cholesterol-loaded, human erythrocytes. Biorheology. 1979;16(4-5):363–369. doi: 10.3233/bir-1979-164-511. [DOI] [PubMed] [Google Scholar]
- Shiga T., Maeda N., Suda T., Kon K., Sekiya M. The decreased membrane fluidity of in vivo aged, human erythrocytes. A spin label study. Biochim Biophys Acta. 1979 May 3;553(1):84–95. doi: 10.1016/0005-2736(79)90032-4. [DOI] [PubMed] [Google Scholar]
- Shiga T., Suda T., Maeda N. Spin label studies on the human erythrocyte membrane. Two sites and two phases for fatty acid spin labels. Biochim Biophys Acta. 1977 Apr 18;466(2):231–244. doi: 10.1016/0005-2736(77)90221-8. [DOI] [PubMed] [Google Scholar]
- Suda T., Maeda N., Shiga T. Effect of cholesterol on human erythrocyte membrane. A spin label study. J Biochem. 1980 Jun;87(6):1703–1713. doi: 10.1093/oxfordjournals.jbchem.a132914. [DOI] [PubMed] [Google Scholar]
- Suda T., Maeda N., Shimizu D., Kamitsubo E., Shiga T. Decreased viscosity of human erythrocyte suspension due to drug-induced spherostomatocytosis. Biorheology. 1982;19(4):555–565. doi: 10.3233/bir-1982-19407. [DOI] [PubMed] [Google Scholar]
- Suda T., Shimizu D., Maeda N., Shiga T. Decreased viscosity of human erythrocyte suspension induced by chlorpromazine and isoxsuprine. Biochem Pharmacol. 1981 Aug 1;30(15):2057–2064. doi: 10.1016/0006-2952(81)90223-9. [DOI] [PubMed] [Google Scholar]
- Valtin H. Animal model of human disease: hereditary hypothalamic diabetes insipidus. Am J Pathol. 1976 Jun;83(3):633–636. [PMC free article] [PubMed] [Google Scholar]
- Verweij H., van Steveninck J. Effects of semicarbazide on oxidative processes in human red blood cell membranes. Biochim Biophys Acta. 1980 Nov 18;602(3):591–599. doi: 10.1016/0005-2736(80)90337-5. [DOI] [PubMed] [Google Scholar]
- Walker L. A., Frölich J. C. Dose-dependent stimulation of renal prostaglandin synthesis by deamino-8-D-arginine vasopressin in rats with hereditary diabetes insipidus. J Pharmacol Exp Ther. 1981 Apr;217(1):87–91. [PubMed] [Google Scholar]
- Walker L. A., Whorton A. R., Smigel M., France R., Frölich J. C. Antidiuretic hormone increases renal prostaglandin synthesis in vivo. Am J Physiol. 1978 Sep;235(3):F180–F185. doi: 10.1152/ajprenal.1978.235.3.F180. [DOI] [PubMed] [Google Scholar]
- Weingarden M., Mizukami H., Rice S. A. Factors defining the rate of oxygen uptake by the red blood cell. Bull Math Biol. 1982;44(1):135–147. doi: 10.1007/BF02459424. [DOI] [PubMed] [Google Scholar]
- Weingarden M., Mizukami H., Rice S. A. Transient effects on the initial rate of oxygenation of red blood cells. Bull Math Biol. 1982;44(1):119–134. doi: 10.1007/BF02459423. [DOI] [PubMed] [Google Scholar]