Abstract
Intracellular recordings were made in photoreceptors and glial cells (outer pigment cells) of the superfused cut head of the honey-bee drone (Apis mellifera male). When the [K+] in the superfusate was abruptly increased from 3.2 mM to 17.9 mM both photoreceptors and glial cells depolarized. The time course of the depolarization of the photoreceptors was slower with increasing depth from the surface. Half time of depolarization was plotted against depth: this graph was compatible with the arrival of K+ being exclusively by diffusion through the extracellular clefts. However, as we then showed, this interpretation is inadequate. The time course of depolarization of the glial cells was almost the same at all depths. This indicates that they are electrically coupled. Consequently, current-mediated K+ flux (spatial buffering) through glial cells will contribute to the transport of K+ through the tissue: K+ ions enter the glial syncytium in the region of high external potassium concentration, [K+]0, and an equivalent quantity of K+ ions leave in regions of low [K+]0. Intracellular K+ activity (aiK) was measured with double-barrelled K+-sensitive micro-electrodes in slices of retina superfused on both faces. When [K+] in the superfusate was increased from 7.5 mM to 17.9 mM an increase in aiK was observed in glial cells at all depths in the slice (initial rate 1.7 mM min-1, S.E. of the mean = 0.2 mM min-1), but there was little increase in the photoreceptors (0.3 +/- 0.2 mM min-1). The increase in aiK in glial cells near the centre of the slice could not have been caused by spatial buffering; it presumably resulted from net uptake. We conclude that when [K+] is increased at the surface of this tissue, the build up of K+ in the extracellular clefts depends on extracellular diffusion, spatial buffering and net uptake. The latter two processes, which have opposing effects, involve about 10 times as much K+ as the first. This is in rough agreement with less direct experiments on mammalian brain (Gardner-Medwin, 1977, 1983b).
Full text
PDF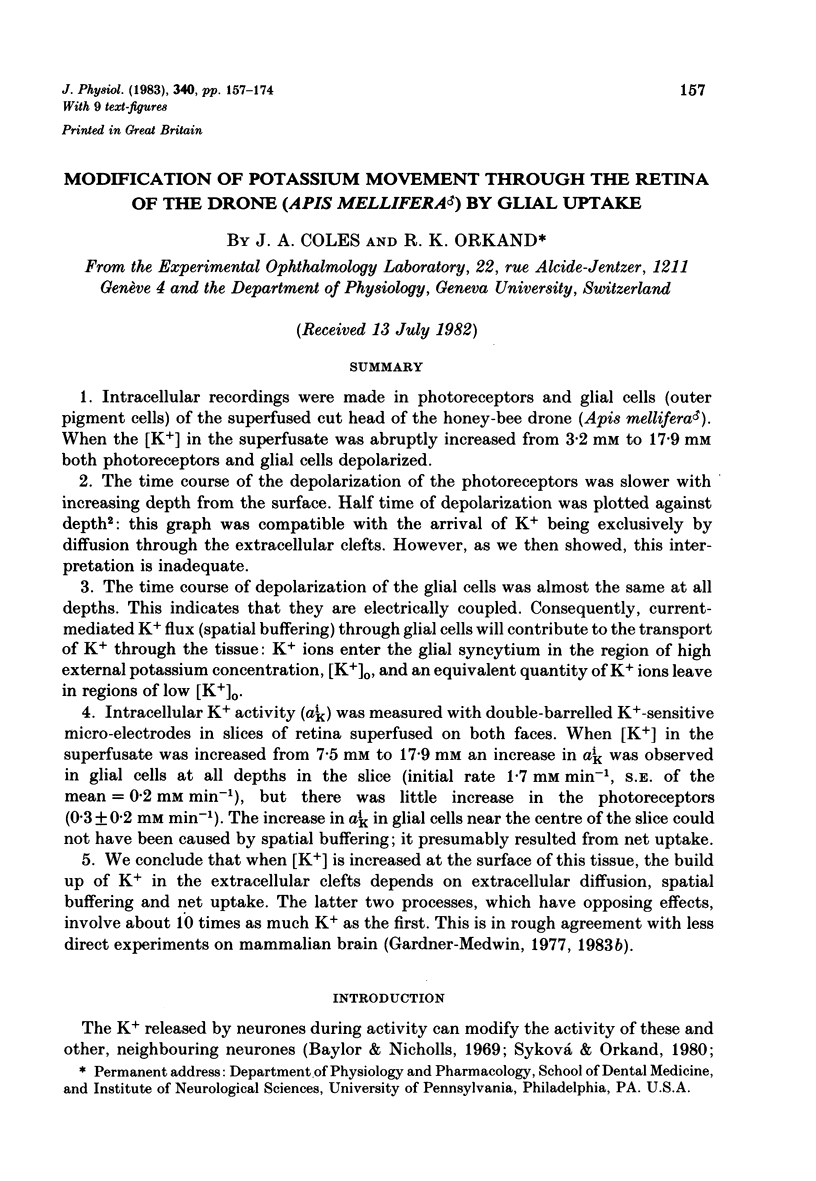
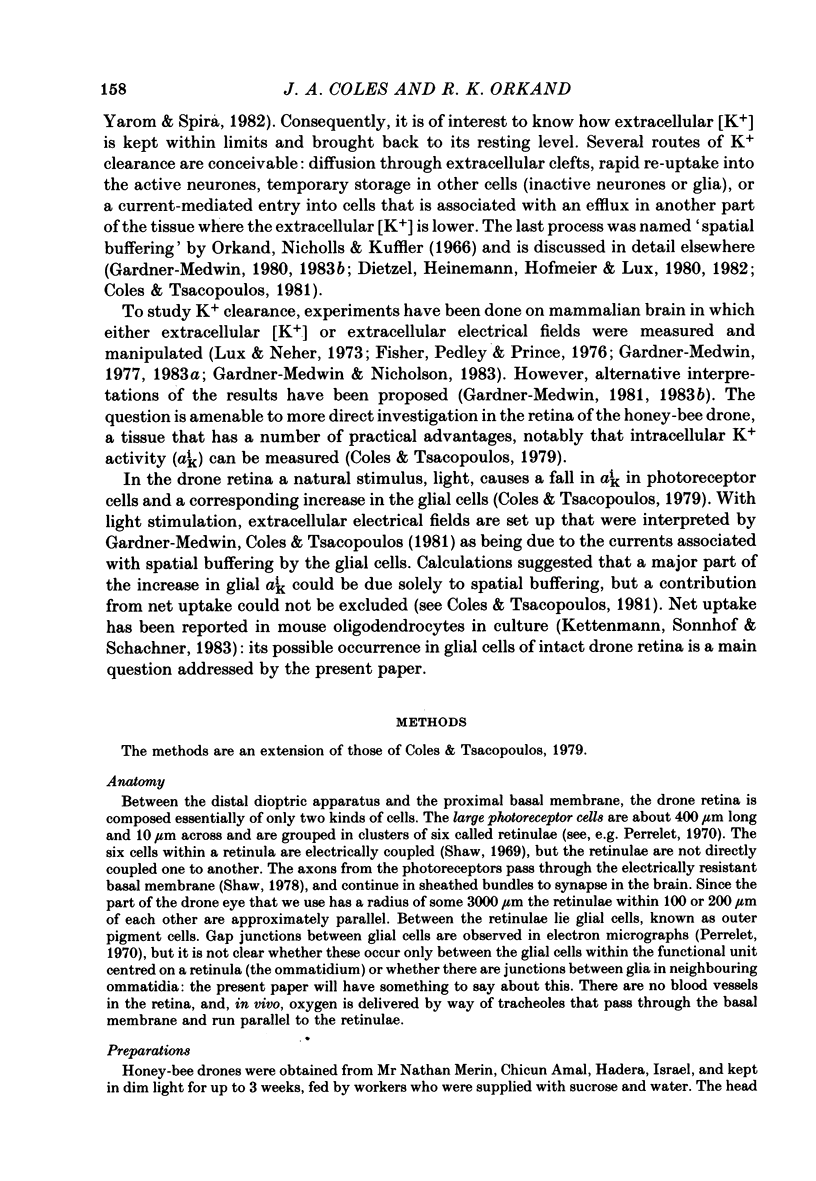
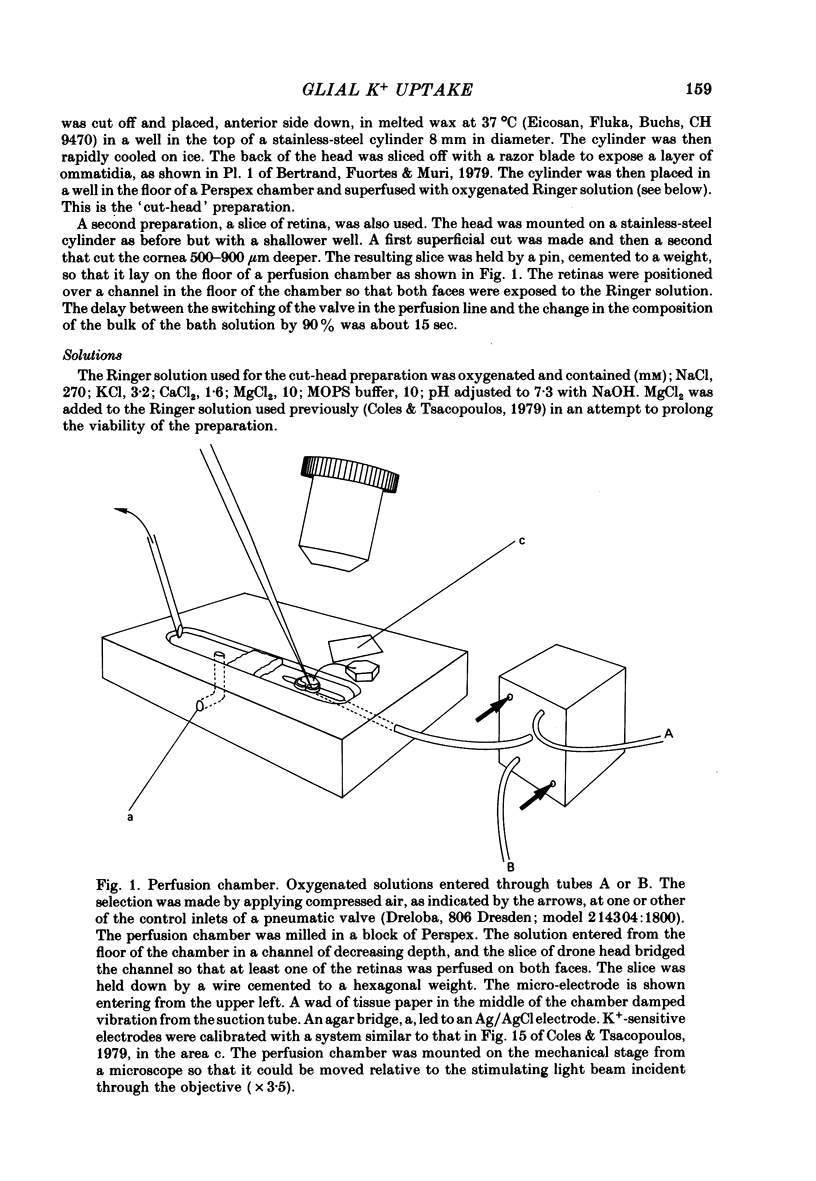
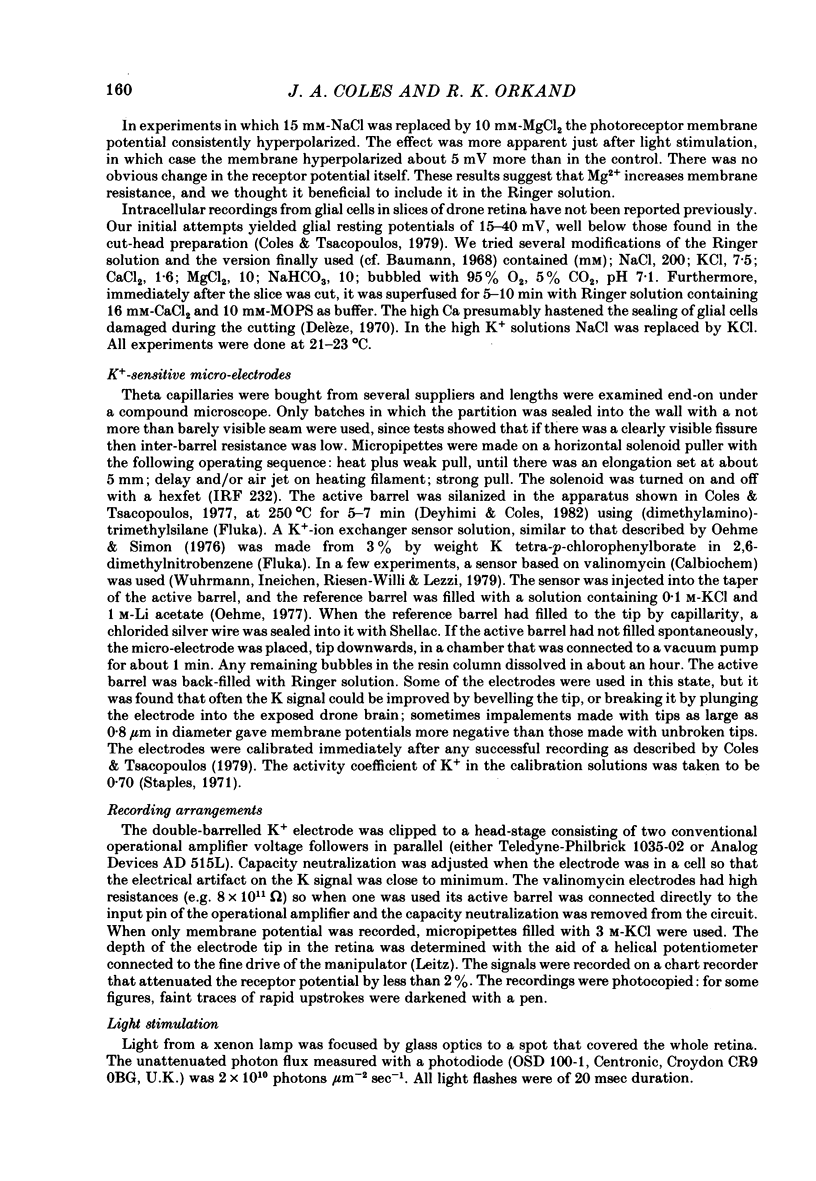
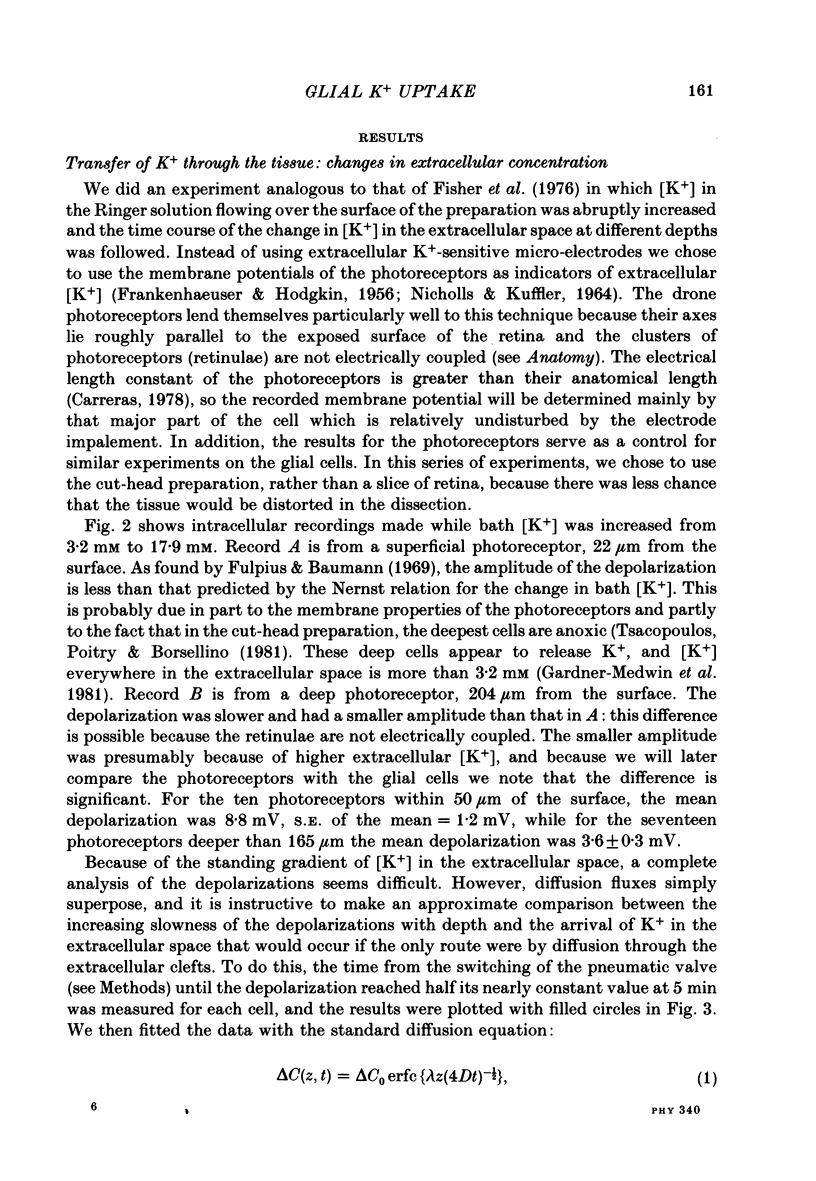
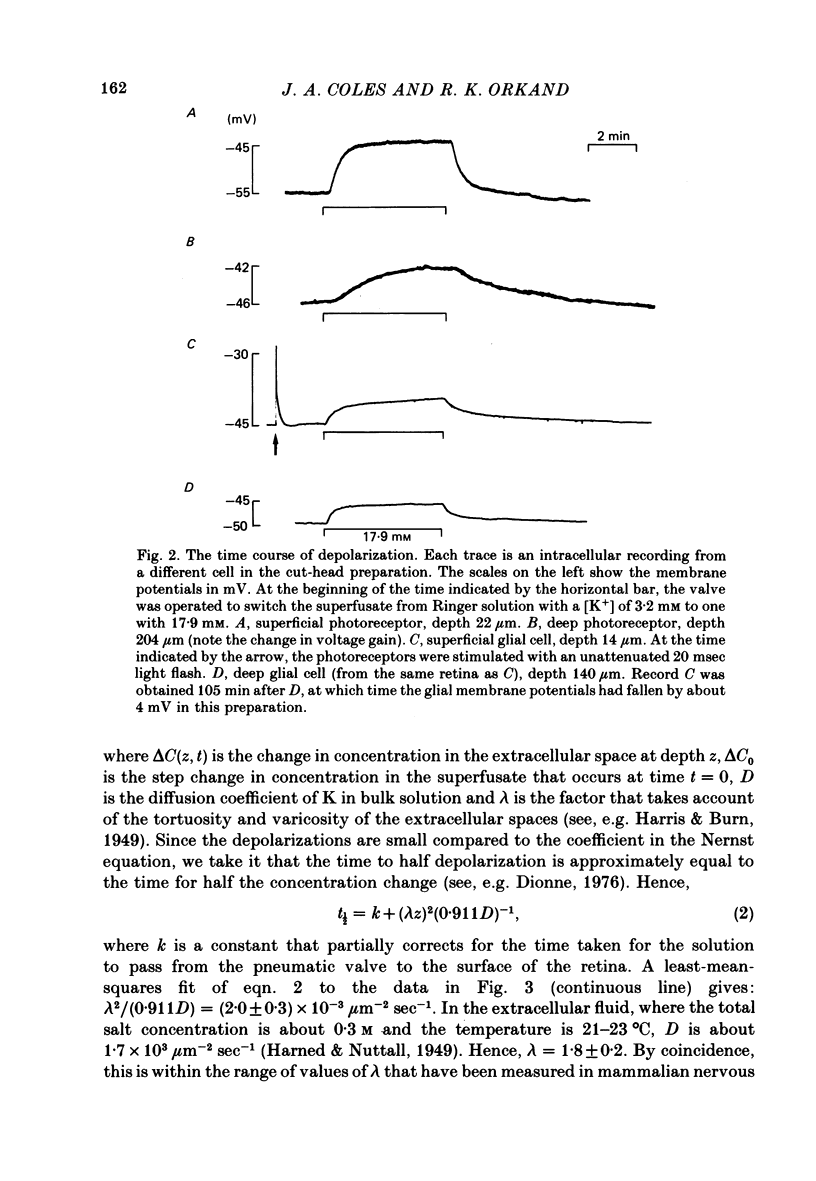
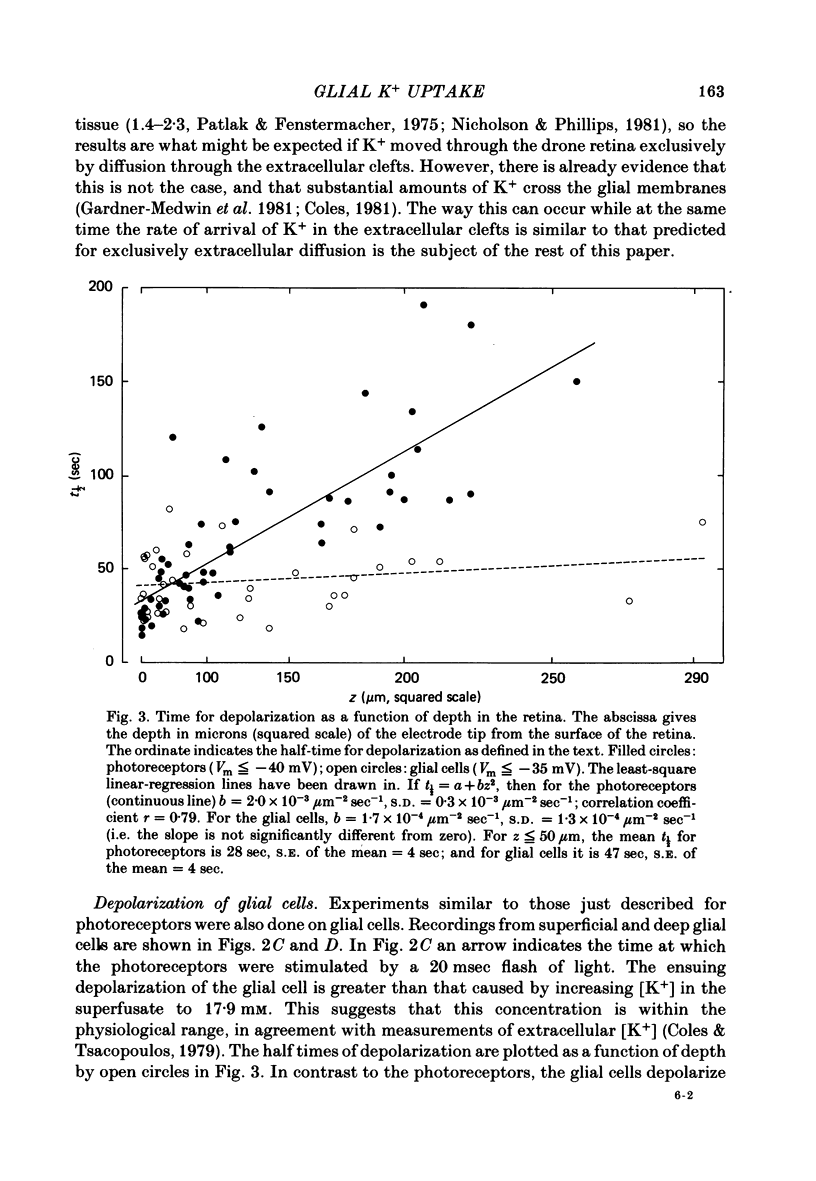
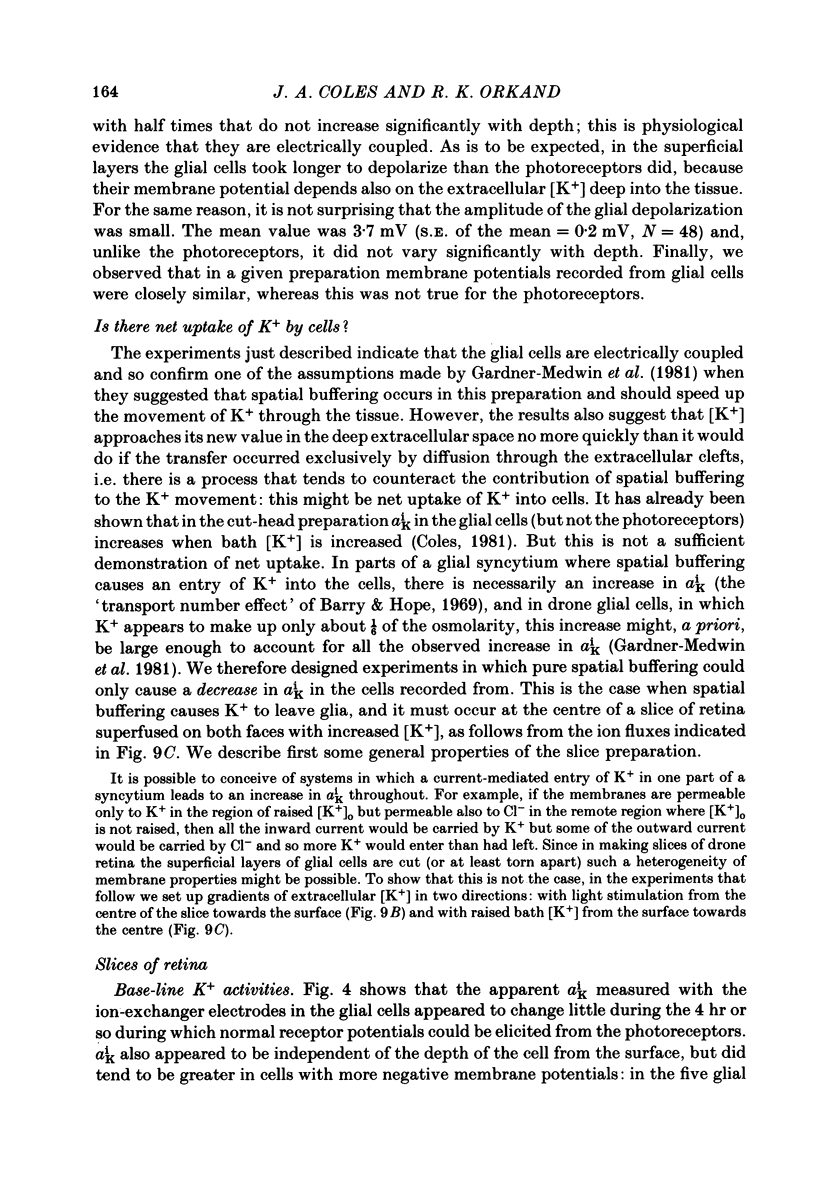
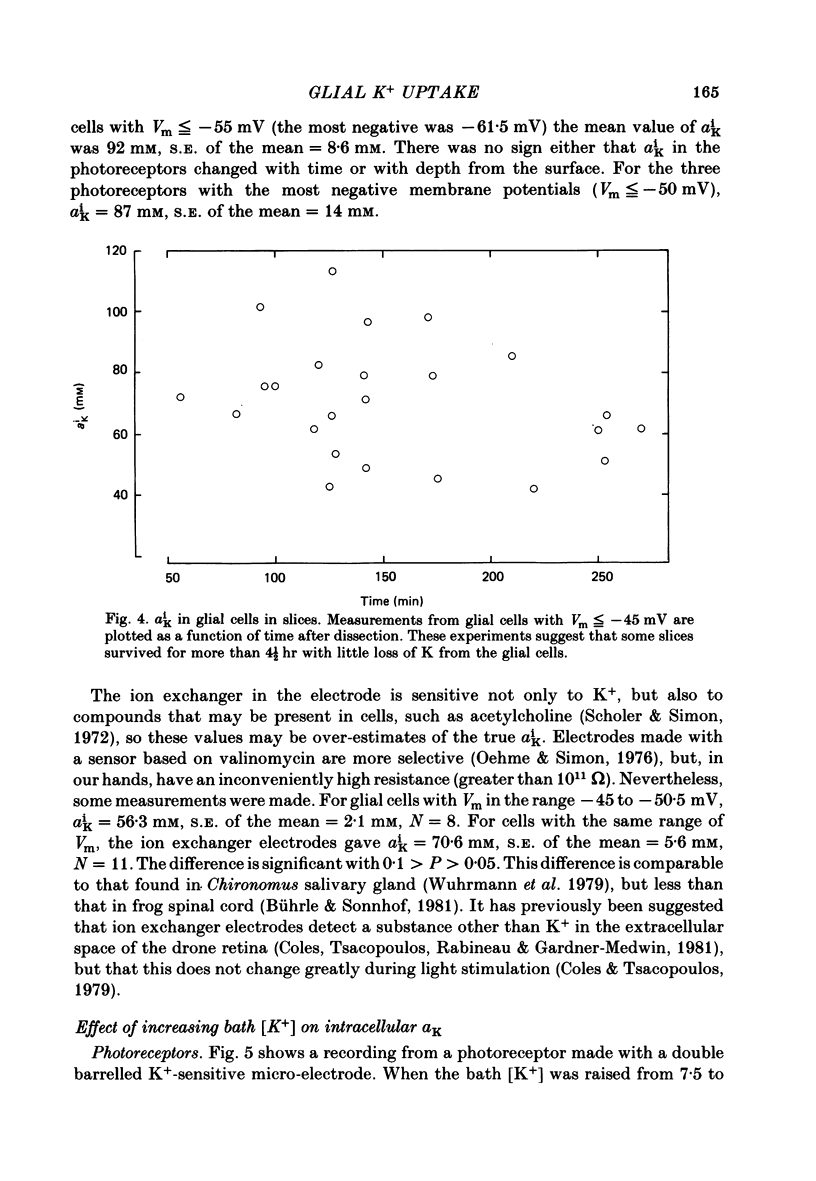
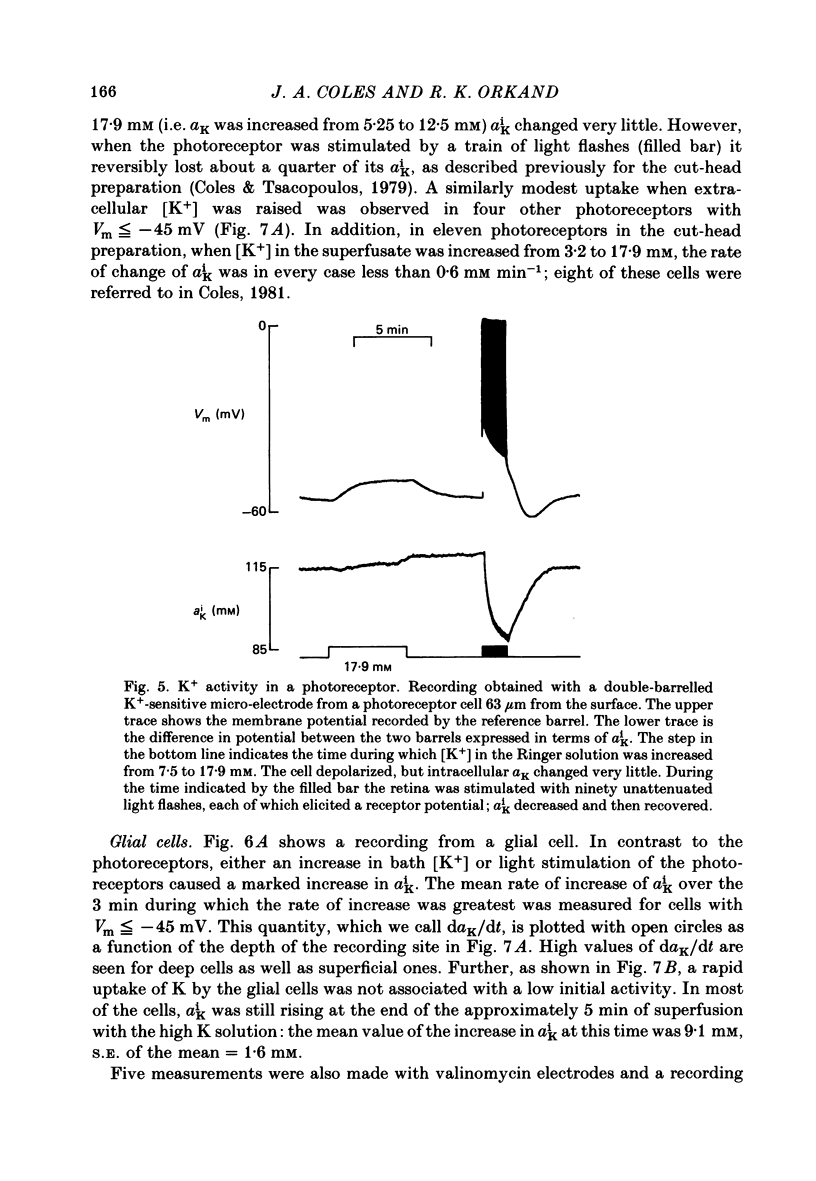
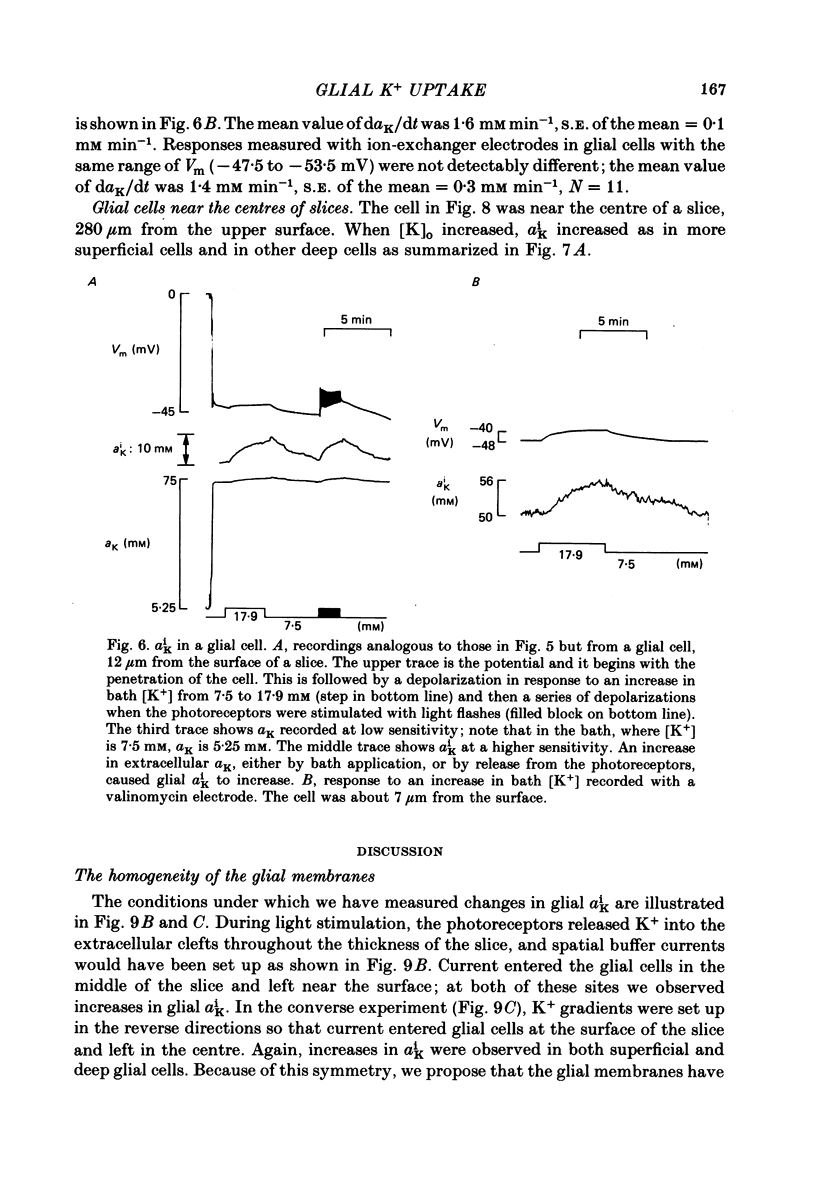
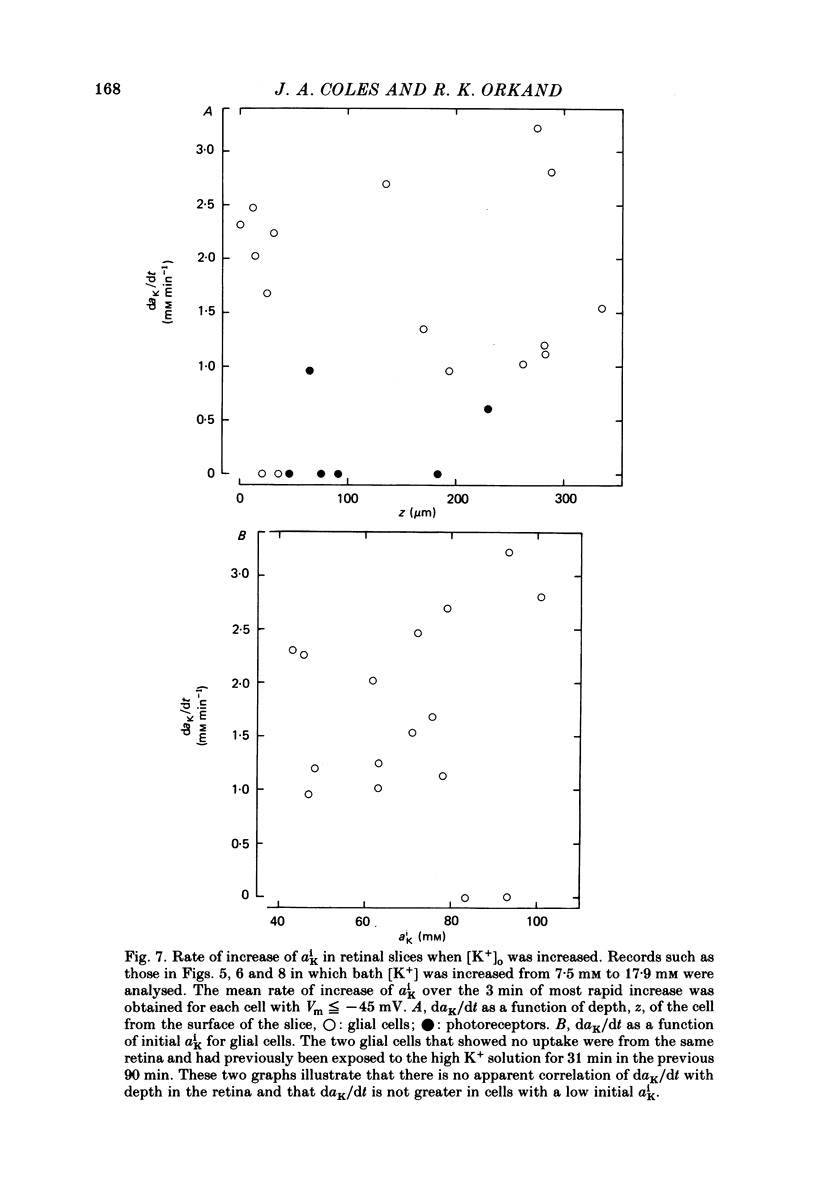
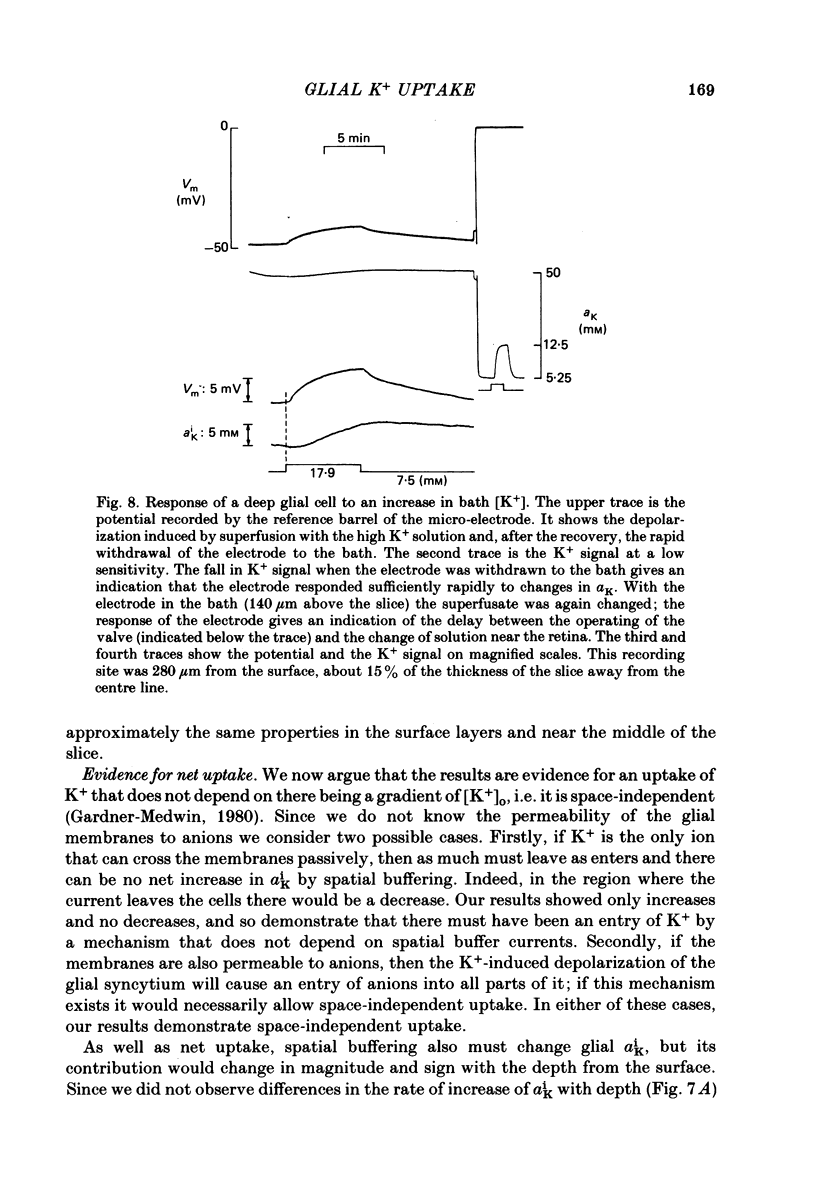
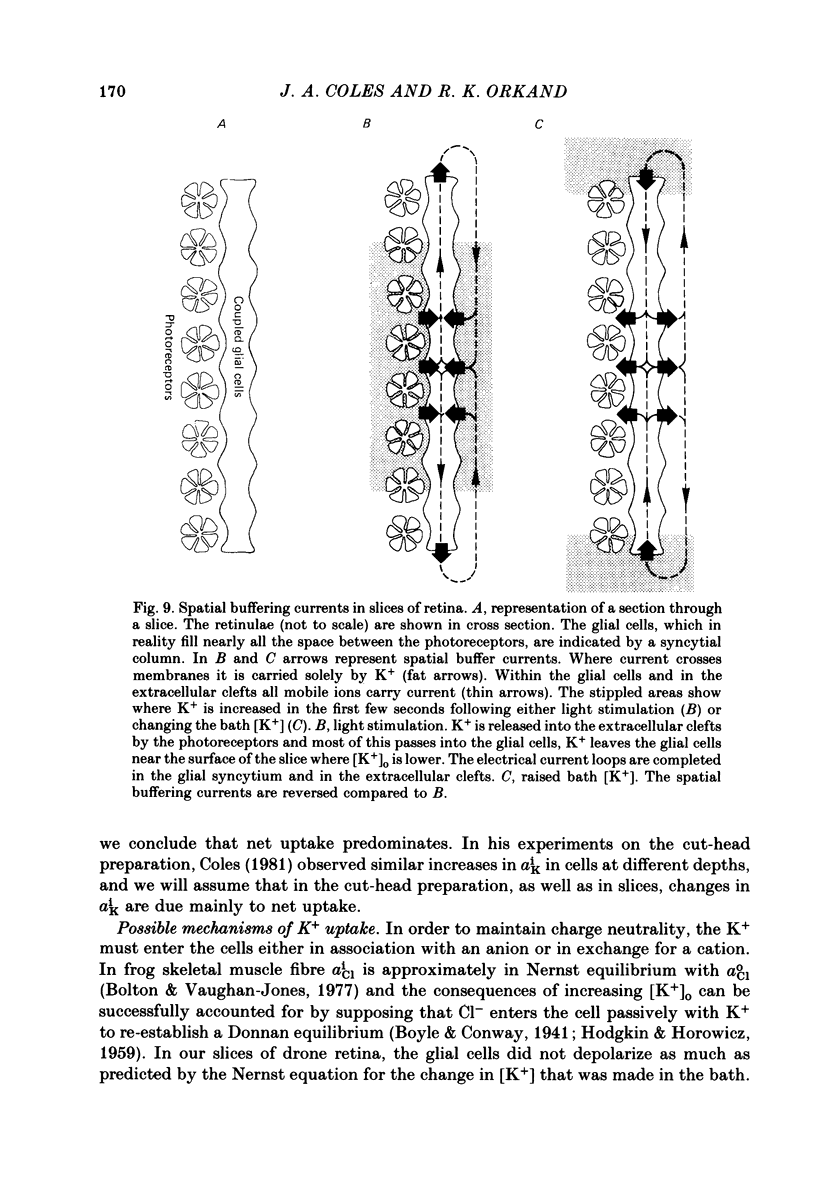
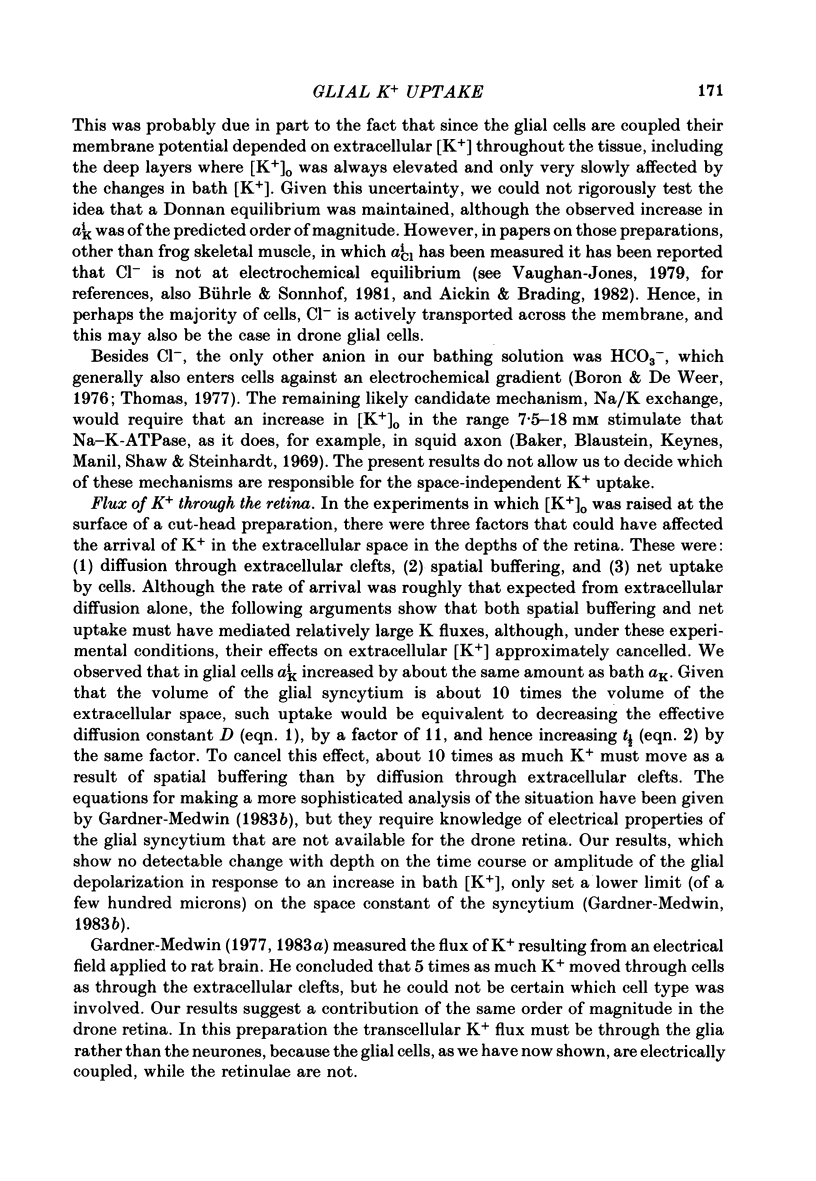
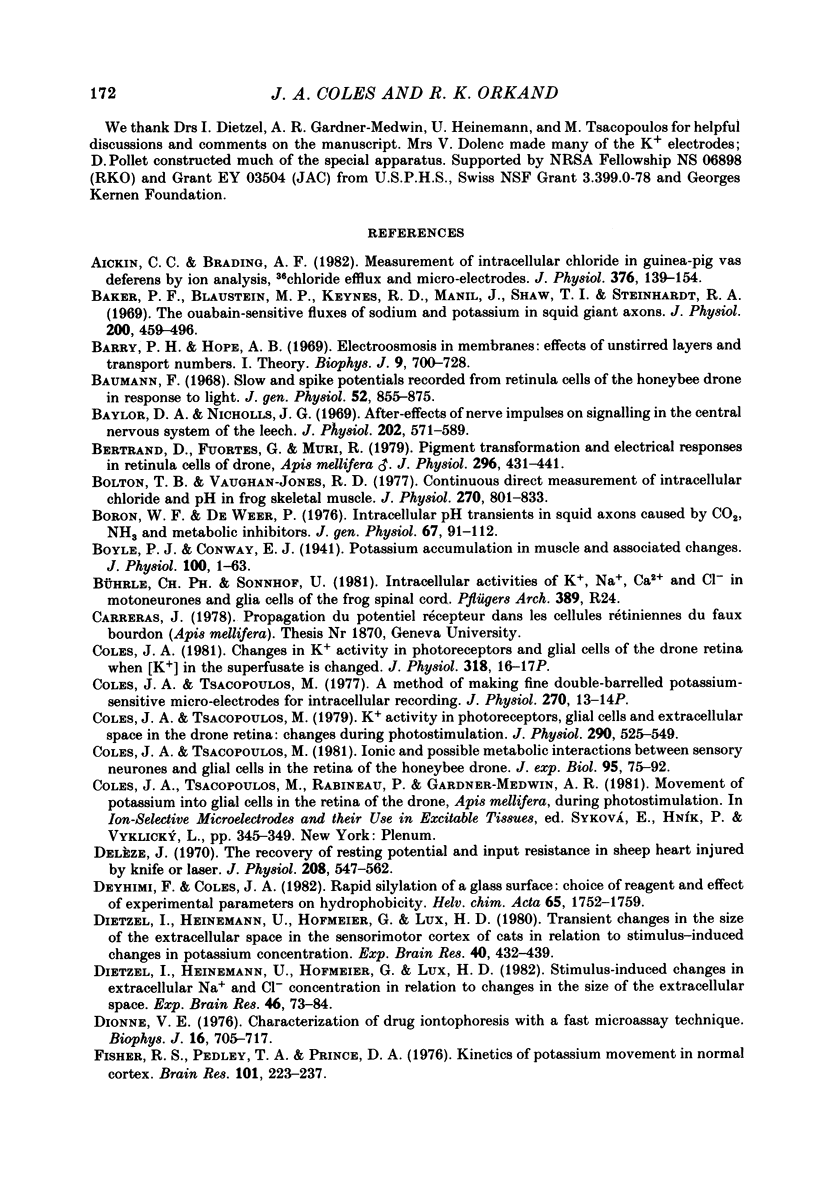
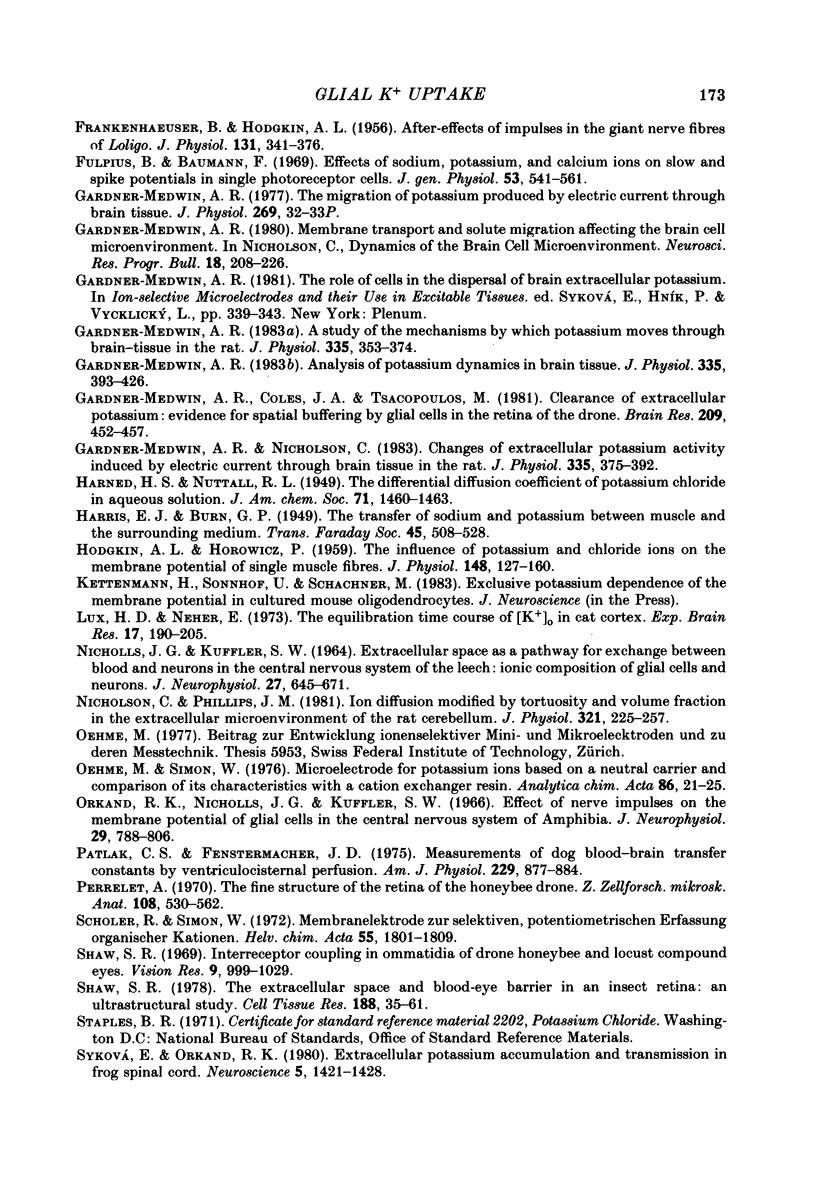
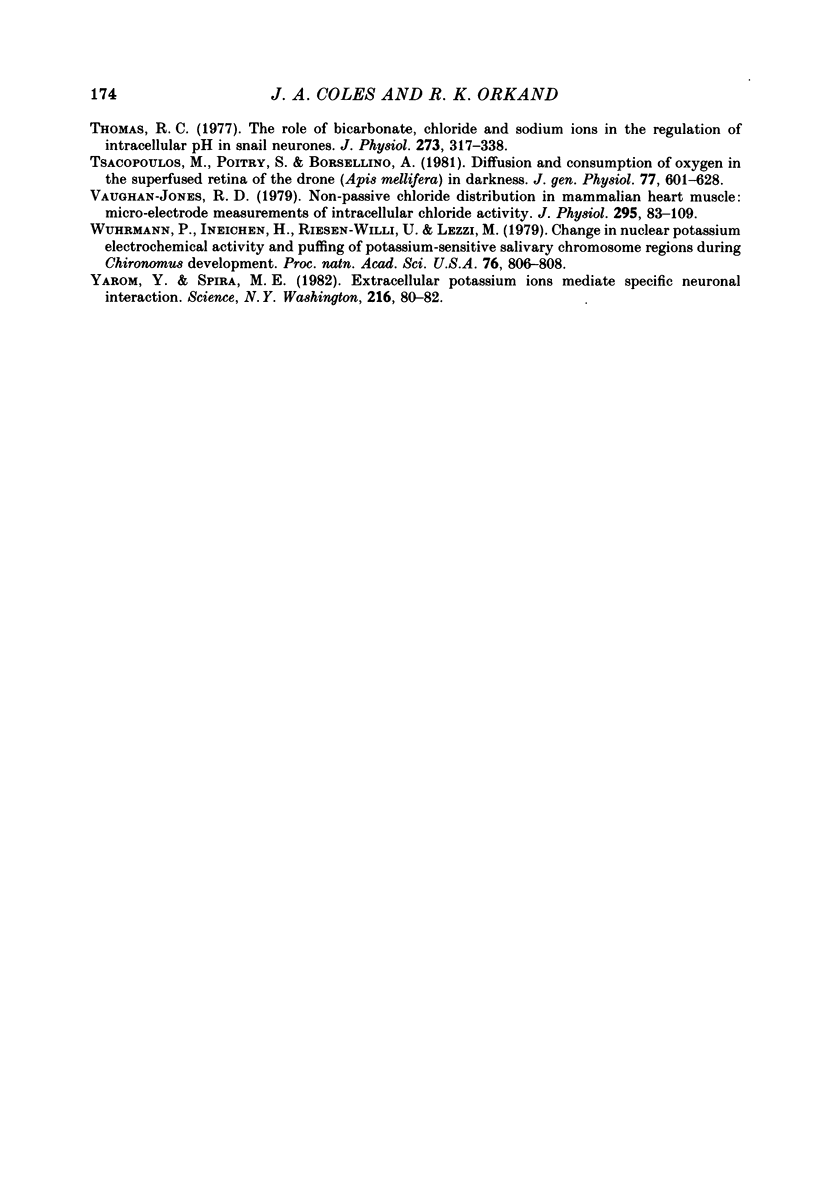
Selected References
These references are in PubMed. This may not be the complete list of references from this article.
- Aickin C. C., Brading A. F. Measurement of intracellular chloride in guinea-pig vas deferens by ion analysis, 36chloride efflux and micro-electrodes. J Physiol. 1982 May;326:139–154. doi: 10.1113/jphysiol.1982.sp014182. [DOI] [PMC free article] [PubMed] [Google Scholar]
- Baker P. F., Blaustein M. P., Keynes R. D., Manil J., Shaw T. I., Steinhardt R. A. The ouabain-sensitive fluxes of sodium and potassium in squid giant axons. J Physiol. 1969 Feb;200(2):459–496. doi: 10.1113/jphysiol.1969.sp008703. [DOI] [PMC free article] [PubMed] [Google Scholar]
- Barry P. H., Hope A. B. Electroosmosis in membranes: effects of unstirred layers and transport numbers. I. Theory. Biophys J. 1969 May;9(5):700–728. doi: 10.1016/S0006-3495(69)86413-1. [DOI] [PMC free article] [PubMed] [Google Scholar]
- Baumann F. Slow and spike potentials recorded from retinula cells of the honeybee drone in response to light. J Gen Physiol. 1968 Dec;52(6):855–875. doi: 10.1085/jgp.52.6.855. [DOI] [PMC free article] [PubMed] [Google Scholar]
- Baylor D. A., Nicholls J. G. After-effects of nerve impulses on signalling in the central nervous system of the leech. J Physiol. 1969 Aug;203(3):571–589. doi: 10.1113/jphysiol.1969.sp008880. [DOI] [PMC free article] [PubMed] [Google Scholar]
- Bertrand D., Fuortes G., Muri R. Pigment transformation and electrical responses in retinula cells of drone, Apis mellifera male. J Physiol. 1979 Nov;296:431–441. doi: 10.1113/jphysiol.1979.sp013014. [DOI] [PMC free article] [PubMed] [Google Scholar]
- Bolton T. B., Vaughan-Jones R. D. Continuous direct measurement of intracellular chloride and pH in frog skeletal muscle. J Physiol. 1977 Sep;270(3):801–833. doi: 10.1113/jphysiol.1977.sp011983. [DOI] [PMC free article] [PubMed] [Google Scholar]
- Boron W. F., De Weer P. Intracellular pH transients in squid giant axons caused by CO2, NH3, and metabolic inhibitors. J Gen Physiol. 1976 Jan;67(1):91–112. doi: 10.1085/jgp.67.1.91. [DOI] [PMC free article] [PubMed] [Google Scholar]
- Boyle P. J., Conway E. J. Potassium accumulation in muscle and associated changes. J Physiol. 1941 Aug 11;100(1):1–63. doi: 10.1113/jphysiol.1941.sp003922. [DOI] [PMC free article] [PubMed] [Google Scholar]
- Coles J. A., Tsacopoulos M. A method of making fine double-barrelled potassium-sensitive micro-electrodes for intracellular recording [proceedings]. J Physiol. 1977 Aug;270(1):12P–14P. [PubMed] [Google Scholar]
- Coles J. A., Tsacopoulos M. Ionic and possible metabolic interactions between sensory neurones and glial cells in the retina of the honeybee drone. J Exp Biol. 1981 Dec;95:75–92. doi: 10.1242/jeb.95.1.75. [DOI] [PubMed] [Google Scholar]
- Coles J. A., Tsacopoulos M. Potassium activity in photoreceptors, glial cells and extracellular space in the drone retina: changes during photostimulation. J Physiol. 1979 May;290(2):525–549. doi: 10.1113/jphysiol.1979.sp012788. [DOI] [PMC free article] [PubMed] [Google Scholar]
- Dietzel I., Heinemann U., Hofmeier G., Lux H. D. Stimulus-induced changes in extracellular Na+ and Cl- concentration in relation to changes in the size of the extracellular space. Exp Brain Res. 1982;46(1):73–84. doi: 10.1007/BF00238100. [DOI] [PubMed] [Google Scholar]
- Dietzel I., Heinemann U., Hofmeier G., Lux H. D. Transient changes in the size of the extracellular space in the sensorimotor cortex of cats in relation to stimulus-induced changes in potassium concentration. Exp Brain Res. 1980;40(4):432–439. doi: 10.1007/BF00236151. [DOI] [PubMed] [Google Scholar]
- Dionne V. E. Characterization of drug iontophoresis with a fast microassay technique. Biophys J. 1976 Jul;16(7):705–717. doi: 10.1016/S0006-3495(76)85723-2. [DOI] [PMC free article] [PubMed] [Google Scholar]
- Délèze J. The recovery of resting potential and input resistance in sheep heart injured by knife or laser. J Physiol. 1970 Jul;208(3):547–562. doi: 10.1113/jphysiol.1970.sp009136. [DOI] [PMC free article] [PubMed] [Google Scholar]
- FRANKENHAEUSER B., HODGKIN A. L. The after-effects of impulses in the giant nerve fibres of Loligo. J Physiol. 1956 Feb 28;131(2):341–376. doi: 10.1113/jphysiol.1956.sp005467. [DOI] [PMC free article] [PubMed] [Google Scholar]
- Fisher R. S., Pedley T. A., Prince D. A. Kinetics of potassium movement in norman cortex. Brain Res. 1976 Jan 16;101(2):223–237. doi: 10.1016/0006-8993(76)90265-1. [DOI] [PubMed] [Google Scholar]
- Fulpius B., Baumann F. Effects of sodium, potassium, and calcium ions on slow and spike potentials in single photoreceptor cells. J Gen Physiol. 1969 May;53(5):541–561. doi: 10.1085/jgp.53.5.541. [DOI] [PMC free article] [PubMed] [Google Scholar]
- Gardner-Medwin A. R. A study of the mechanisms by which potassium moves through brain tissue in the rat. J Physiol. 1983 Feb;335:353–374. doi: 10.1113/jphysiol.1983.sp014539. [DOI] [PMC free article] [PubMed] [Google Scholar]
- Gardner-Medwin A. R. Analysis of potassium dynamics in mammalian brain tissue. J Physiol. 1983 Feb;335:393–426. doi: 10.1113/jphysiol.1983.sp014541. [DOI] [PMC free article] [PubMed] [Google Scholar]
- Gardner-Medwin A. R., Coles J. A., Tsacopoulos M. Clearance of extracellular potassium: evidence for spatial buffering by glial cells in the retina of the drone. Brain Res. 1981 Mar 30;209(2):452–457. doi: 10.1016/0006-8993(81)90169-4. [DOI] [PubMed] [Google Scholar]
- Gardner-Medwin A. R., Nicholson C. Changes of extracellular potassium activity induced by electric current through brain tissue in the rat. J Physiol. 1983 Feb;335:375–392. doi: 10.1113/jphysiol.1983.sp014540. [DOI] [PMC free article] [PubMed] [Google Scholar]
- Gardner-Medwin A. R. The migration of potassium produced by electric current through brain tissue [proceedings]. J Physiol. 1977 Jul;269(1):32P–33P. [PubMed] [Google Scholar]
- HODGKIN A. L., HOROWICZ P. The influence of potassium and chloride ions on the membrane potential of single muscle fibres. J Physiol. 1959 Oct;148:127–160. doi: 10.1113/jphysiol.1959.sp006278. [DOI] [PMC free article] [PubMed] [Google Scholar]
- Lux H. D., Neher E. The equilibration time course of (K + ) 0 in cat cortex. Exp Brain Res. 1973 Apr 30;17(2):190–205. doi: 10.1007/BF00235028. [DOI] [PubMed] [Google Scholar]
- NICHOLLS J. G., KUFFLER S. W. EXTRACELLULAR SPACE AS A PATHWAY FOR EXCHANGE BETWEEN BLOOD AND NEURONS IN THE CENTRAL NERVOUS SYSTEM OF THE LEECH: IONIC COMPOSITION OF GLIAL CELLS AND NEURONS. J Neurophysiol. 1964 Jul;27:645–671. doi: 10.1152/jn.1964.27.4.645. [DOI] [PubMed] [Google Scholar]
- Nicholson C., Phillips J. M. Ion diffusion modified by tortuosity and volume fraction in the extracellular microenvironment of the rat cerebellum. J Physiol. 1981 Dec;321:225–257. doi: 10.1113/jphysiol.1981.sp013981. [DOI] [PMC free article] [PubMed] [Google Scholar]
- Orkand R. K., Nicholls J. G., Kuffler S. W. Effect of nerve impulses on the membrane potential of glial cells in the central nervous system of amphibia. J Neurophysiol. 1966 Jul;29(4):788–806. doi: 10.1152/jn.1966.29.4.788. [DOI] [PubMed] [Google Scholar]
- Patlak C. S., Fenstermacher J. D. Measurements of dog blood-brain transfer constants by ventriculocisternal perfusion. Am J Physiol. 1975 Oct;229(4):877–884. doi: 10.1152/ajplegacy.1975.229.4.877. [DOI] [PubMed] [Google Scholar]
- Perrelet A. The fine structure of the retina of the honey bee drone. An electron microscopical study. Z Zellforsch Mikrosk Anat. 1970;108(4):530–562. doi: 10.1007/BF00339658. [DOI] [PubMed] [Google Scholar]
- Shaw S. R. Interreceptor coupling in ommatidia of drone honeybee and locust compound eyes. Vision Res. 1969 Sep;9(9):999–1029. doi: 10.1016/0042-6989(69)90044-3. [DOI] [PubMed] [Google Scholar]
- Shaw S. R. The extracellular space and blood-eye barrier in an insect retina: an ultrastructural study. Cell Tissue Res. 1978 Mar 31;188(1):35–61. doi: 10.1007/BF00220513. [DOI] [PubMed] [Google Scholar]
- Syková E., Orkand R. K. Extracellular potassium accumulation and transmission in frog spinal cord. Neuroscience. 1980;5(8):1421–1428. doi: 10.1016/0306-4522(80)90003-2. [DOI] [PubMed] [Google Scholar]
- Thomas R. C. The role of bicarbonate, chloride and sodium ions in the regulation of intracellular pH in snail neurones. J Physiol. 1977 Dec;273(1):317–338. doi: 10.1113/jphysiol.1977.sp012096. [DOI] [PMC free article] [PubMed] [Google Scholar]
- Tsacopoulos M., Poitry S., Borsellino A. Diffusion and consumption of oxygen in the superfused retina of the drone (Apis mellifera) in darkness. J Gen Physiol. 1981 Jun;77(6):601–628. doi: 10.1085/jgp.77.6.601. [DOI] [PMC free article] [PubMed] [Google Scholar]
- Vaughan-Jones R. D. Non-passive chloride distribution in mammalian heart muscle: micro-electrode measurement of the intracellular chloride activity. J Physiol. 1979 Oct;295:83–109. doi: 10.1113/jphysiol.1979.sp012956. [DOI] [PMC free article] [PubMed] [Google Scholar]
- Wuhrmann P., Ineichen H., Riesen-Willi U., Lezzi M. Change in nuclear potassium electrochemical activity and puffing of potassium-sensitive salivary chromosome regions during Chironomus development. Proc Natl Acad Sci U S A. 1979 Feb;76(2):806–808. doi: 10.1073/pnas.76.2.806. [DOI] [PMC free article] [PubMed] [Google Scholar]
- Yarom Y., Spira M. E. Extracellular potassium ions mediate specific neuronal interaction. Science. 1982 Apr 2;216(4541):80–82. doi: 10.1126/science.6278595. [DOI] [PubMed] [Google Scholar]