Abstract
1. Intracellular voltage and current responses to short (blue) and long (red) wave-length lights were measured in the distal hyperpolarizing photoreceptor (`off receptor') of the isolated and perfused scallop (Pecten irradians) retina.
2. The early receptor potential (e.r.p.) was isolated by holding membrane potential at the reversal potential for the late receptor potential (l.r.p.) or by working at temperatures (< 5·0 °C) that abolished the l.r.p.
3. The e.r.p., measured using intense flashes of white light, consisted of a positive phase followed by a negative phase, but was converted to a monophasic, negative-going wave following pre-adaptation with red light and to a monophasic, positive-going wave following pre-adaptation with blue light.
4. The spectral sensitivity curve for the negative e.r.p. was maximum at 500 nm, whereas the spectral sensitivity curve for the positive e.r.p. was maximum at 575 nm.
5. The positive or negative e.r.p.s approached their maximum amplitude exponentially when tested with red or blue flashes of increasing intensity. The results suggest that the positive (or negative) e.r.p. is proportional to the number of photopigment molecules photo-isomerized.
6. The photosensitivity maximum of rhodopsin calculated at 500 nm, using the exponential constant and the spectral sensitivity data, was estimated to be 2·1 × 10-16 cm2 photon-1, whereas the photosensitivity maximum of metarhodopsin calculated at 575 nm was estimated to be 2·6 × 10-16 cm2 photon-1.
7. In cells pre-adapted with white light, stimulation with blue light caused a hyperpolarizing l.r.p. which was followed by a prolonged hyperpolarizing after-potential (p.h.a.). Stimulation with red light under similar conditions caused an initial hyperpolarization which was followed by a small depolarization during the stimulus, but no after-potential.
8. The duration of the p.h.a. was increased by pre-adaptation with a red light, which caused the maximum net transfer of metarhodopsin to rhodopsin; however, its decay was always complete in 5 min or less.
9. The photo-isomerization of metarhodopsin by red light suppressed the p.h.a. and caused an after-depolarizing response that decayed in less than 1 min.
10. The spectral sensitivity curve for the induction of the p.h.a. was maximum at 500 nm and corresponded to the spectral sensitivity for the negative e.r.p. and for the l.r.p. studied in the dark-adapted retina, whereas the spectral sensitivity curve for the suppression of the p.h.a. and for the induction of the after-depolarization was maximum at 575 nm and corresponded to the spectral sensitivity for the positive e.r.p.
11. In photoreceptors clamped to the resting potential in normal ASW, the photo-isomerization of rhodopsin, in the absence of light absorption by metarhodopsin, activated a persistent outward current that had the same time course of decay as the p.h.a. The photo-isomerization of metarhodopsin suppressed the persistent outward current and activated an inward current whose decay took longer than the decay of the after-depolarizing response.
12. In the absence of external Ca2+ and Na+ ions, the persistent outward current produced by light absorption by rhodopsin, and the inward current produced by light absorption by metarhodopsin, both reversed at the K+ equilibrium potential. The results show that the induction of the prolonged hyperpolarizing after-potential and the after-depolarizing response involve only the movement of K+ ions through the same light-dependent K+ channels that determine the hyperpolarizing l.r.p. of the distal cells.
Full text
PDF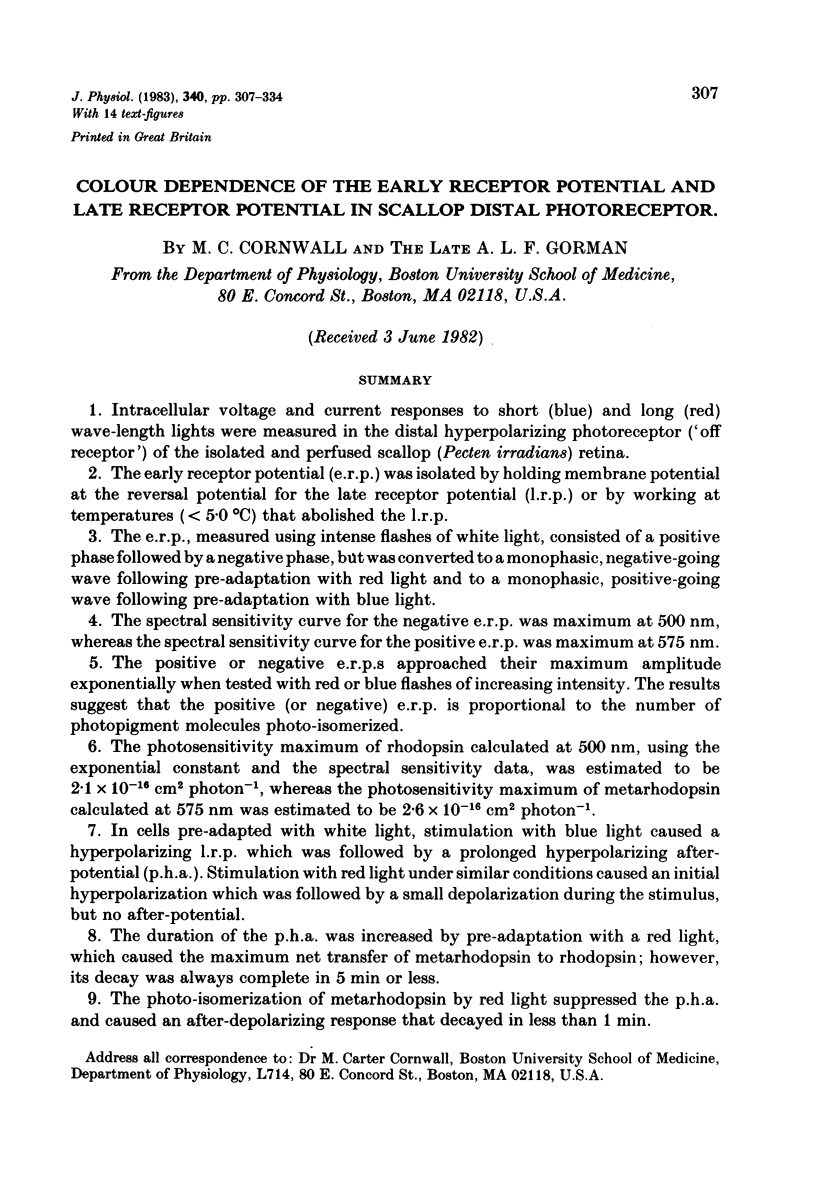
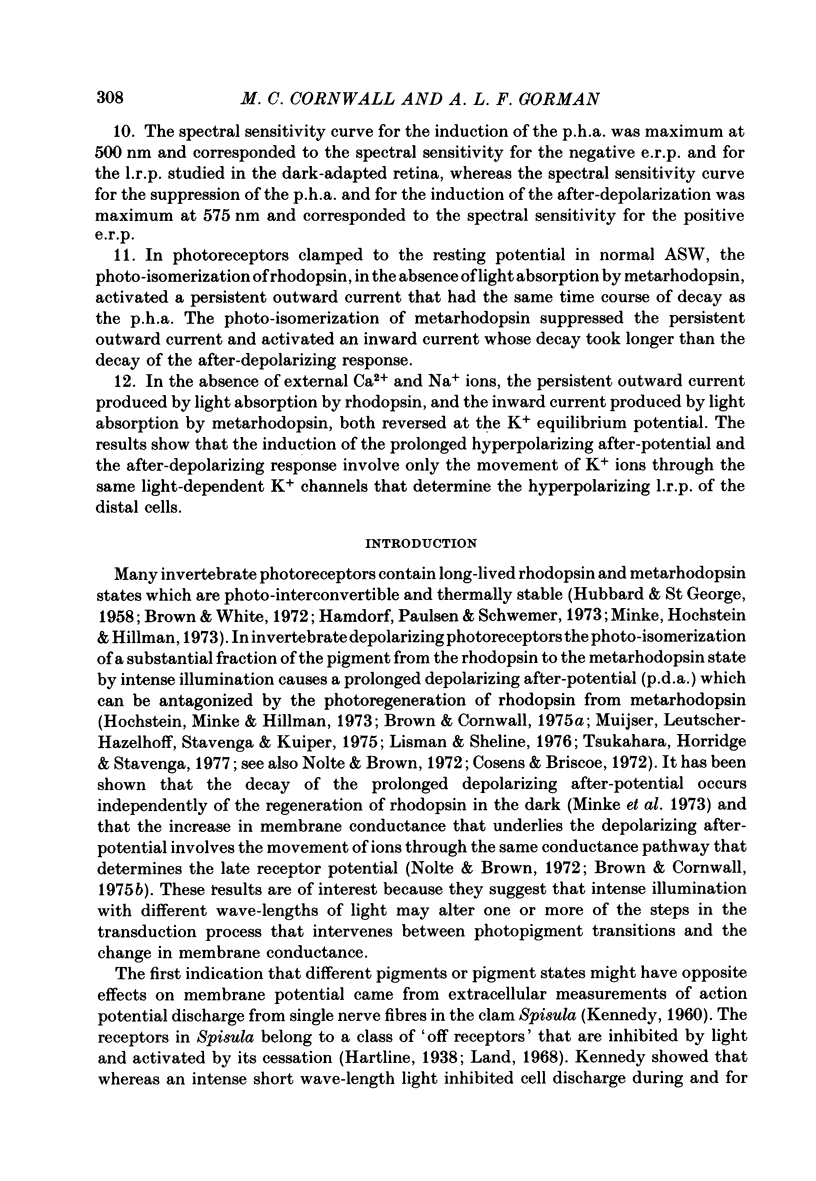
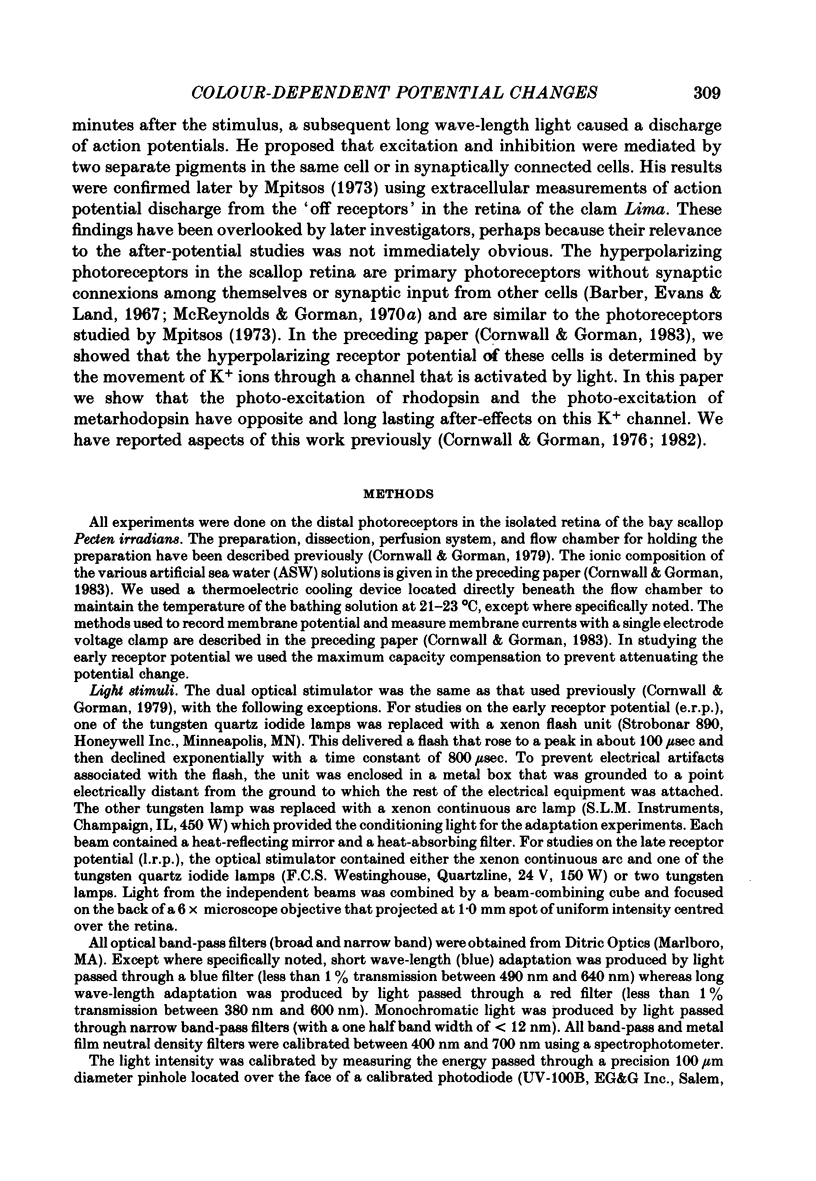
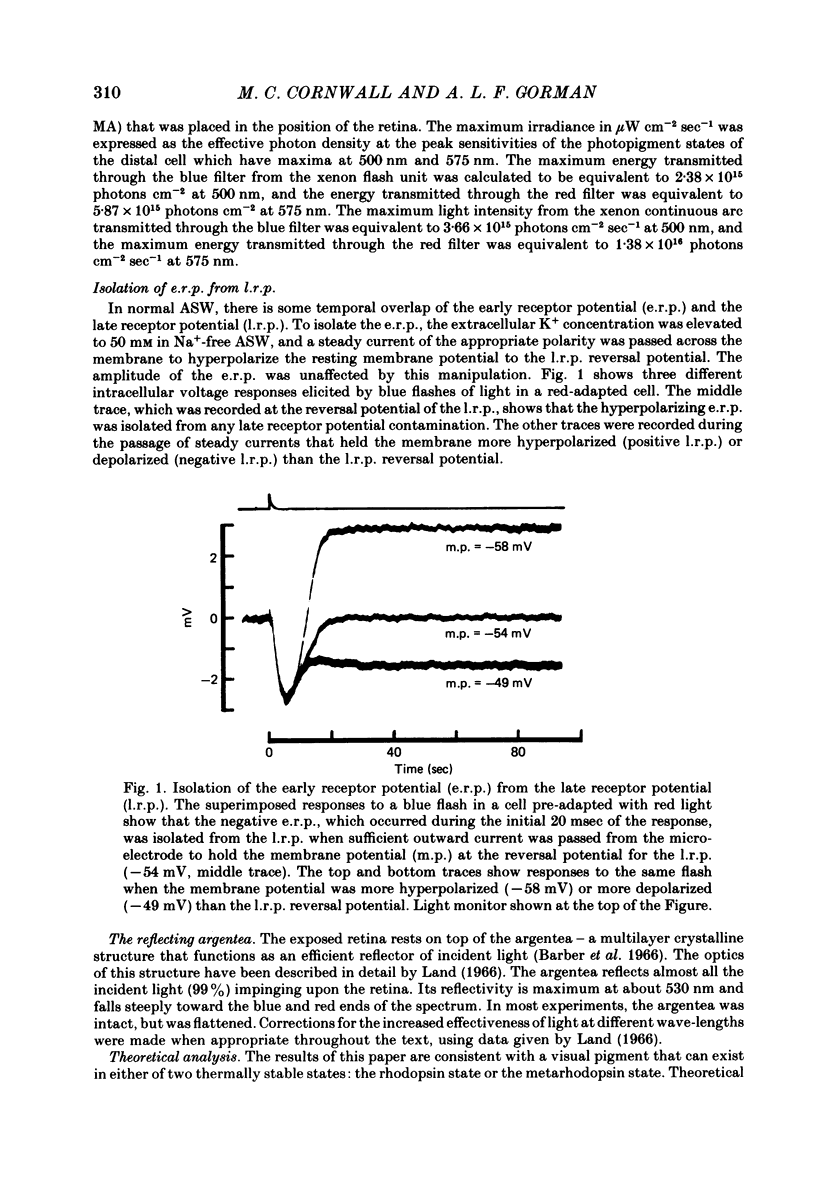
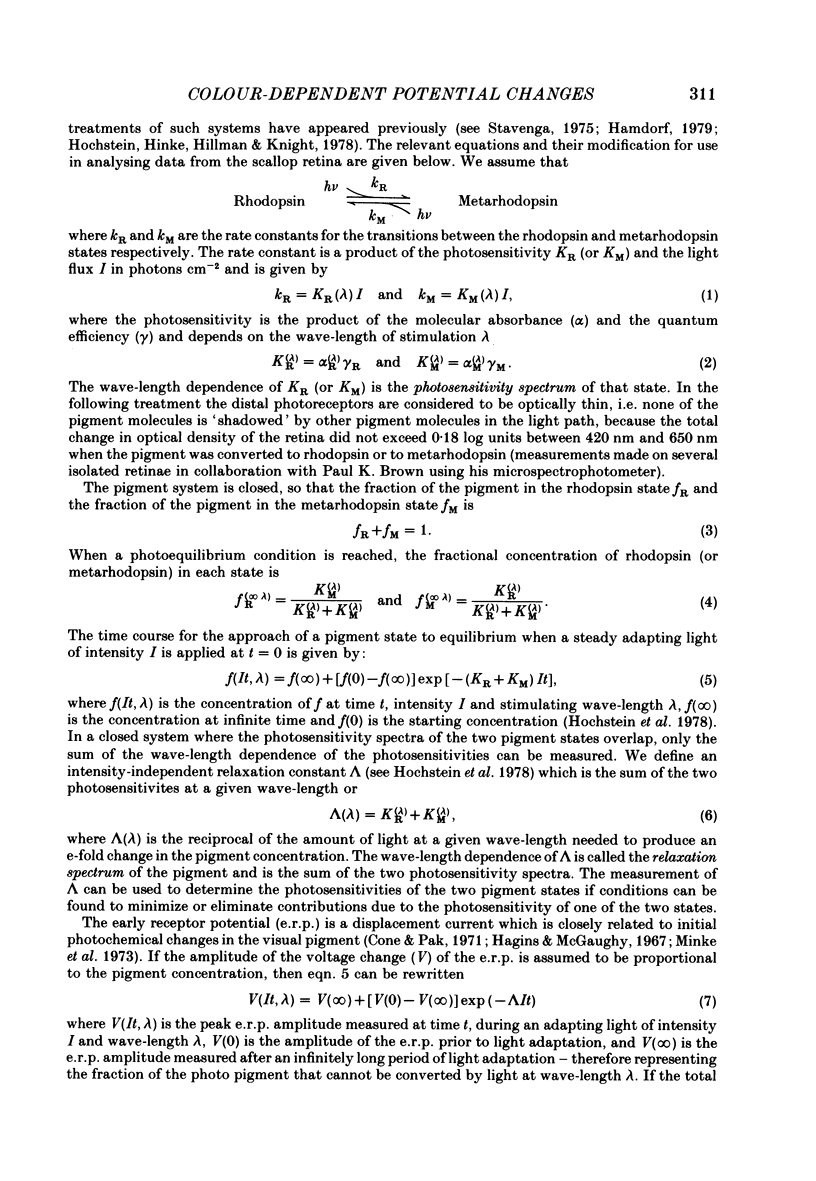
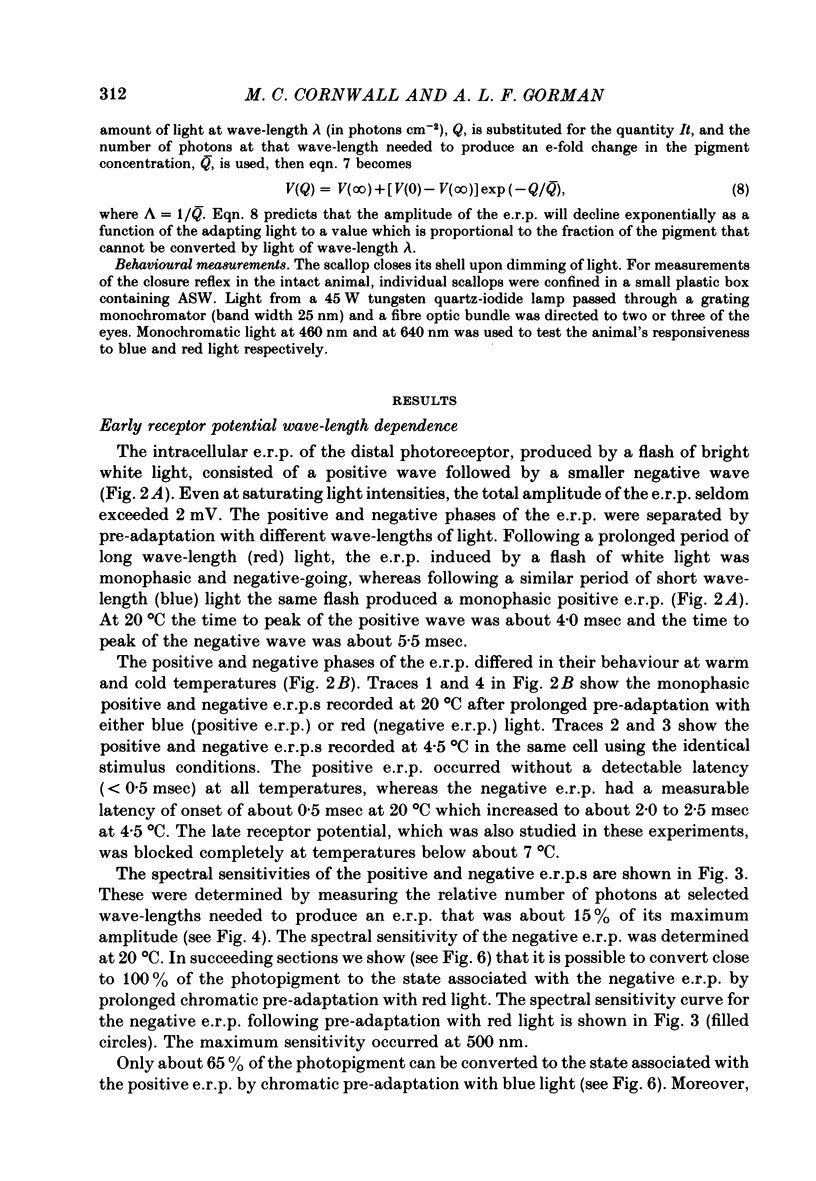
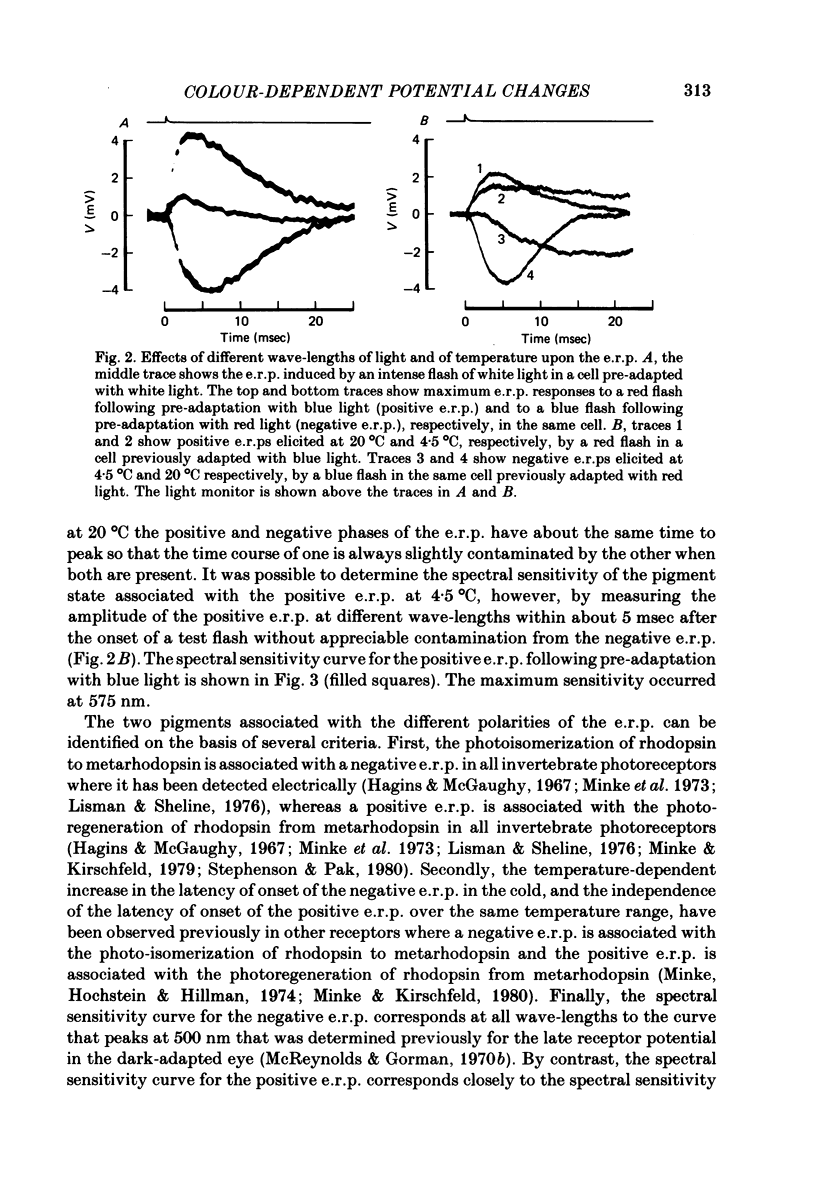
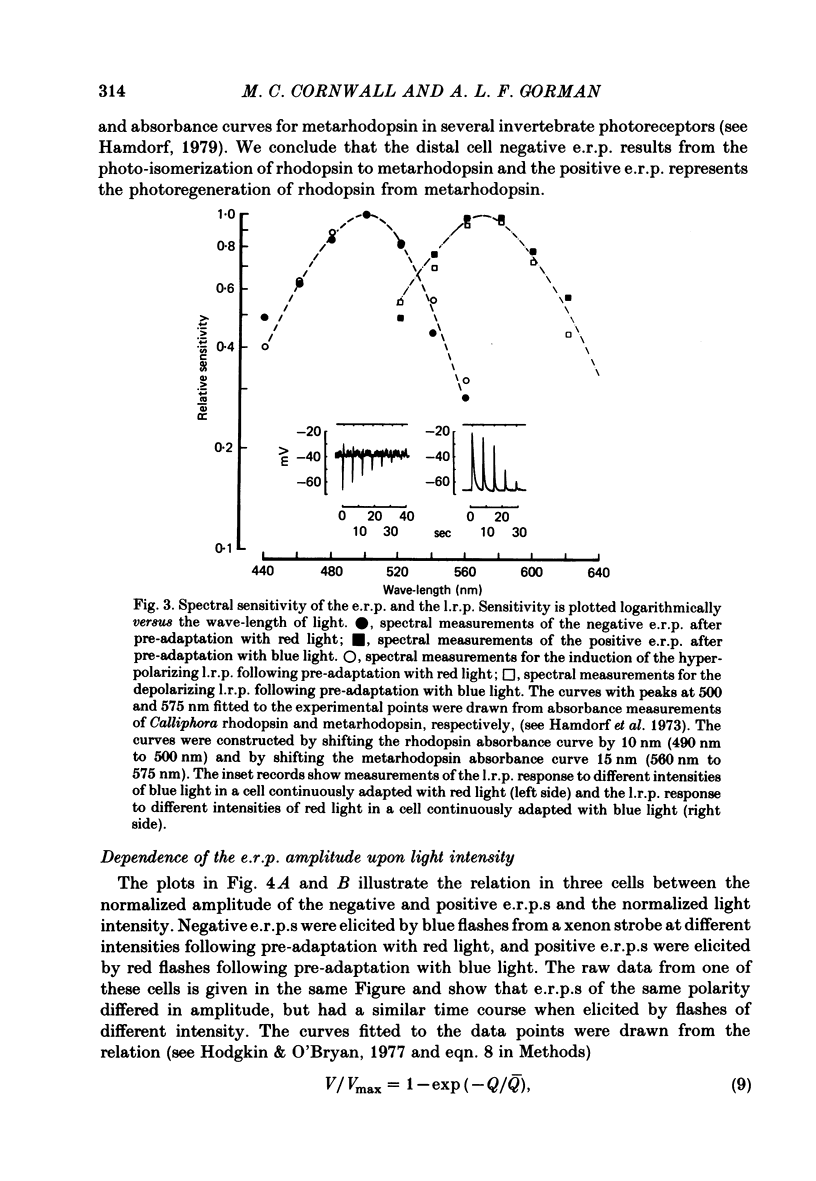
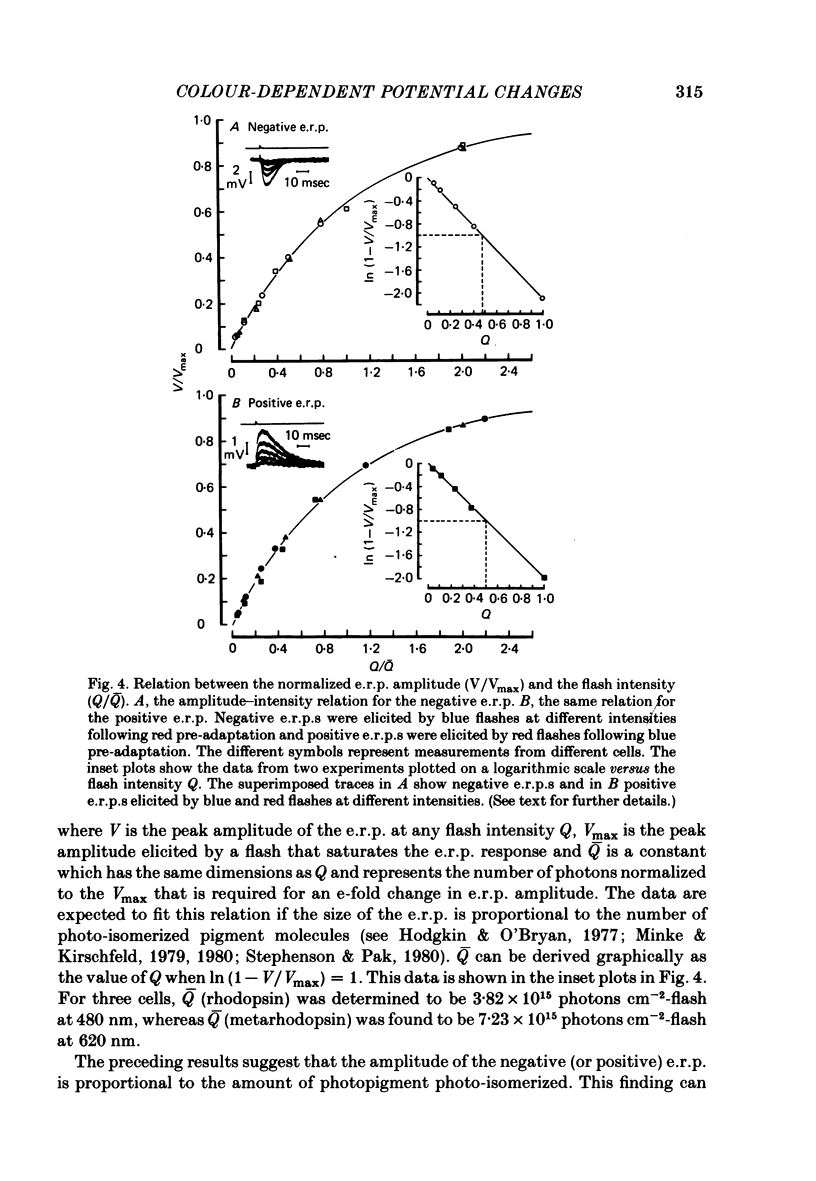
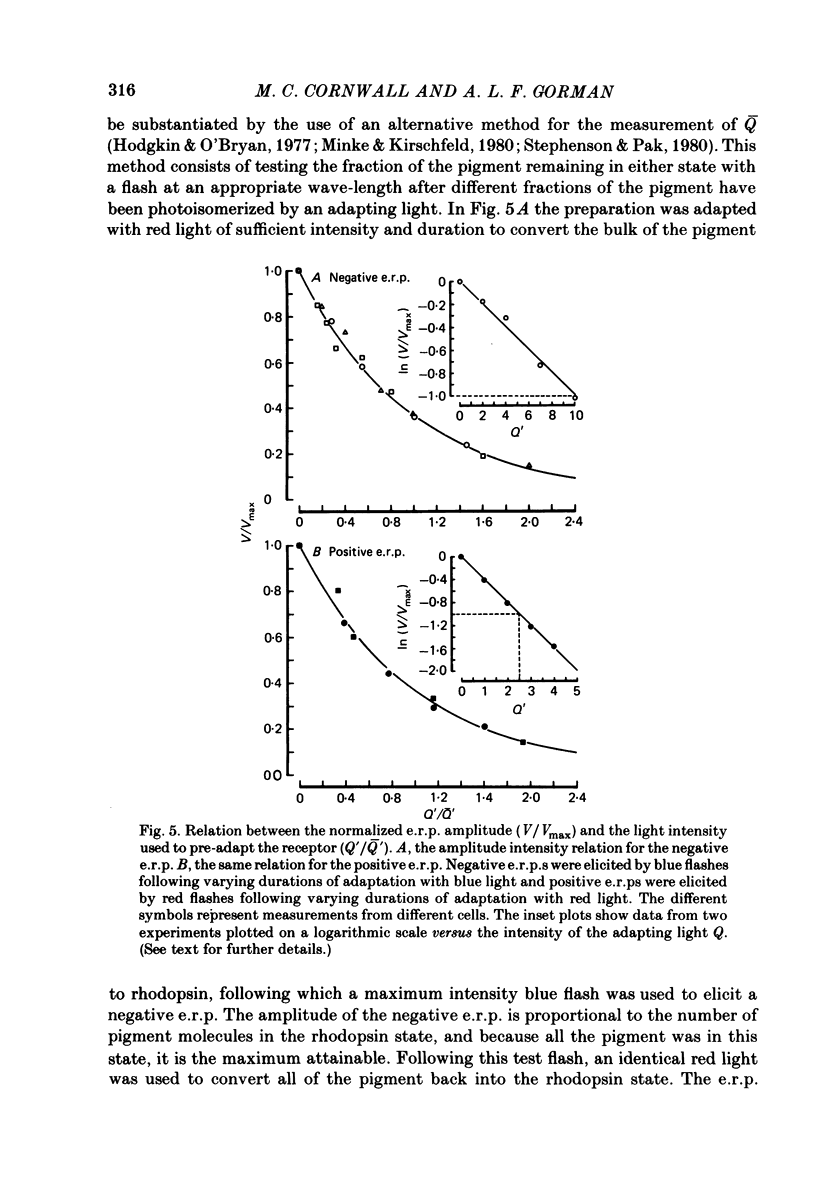
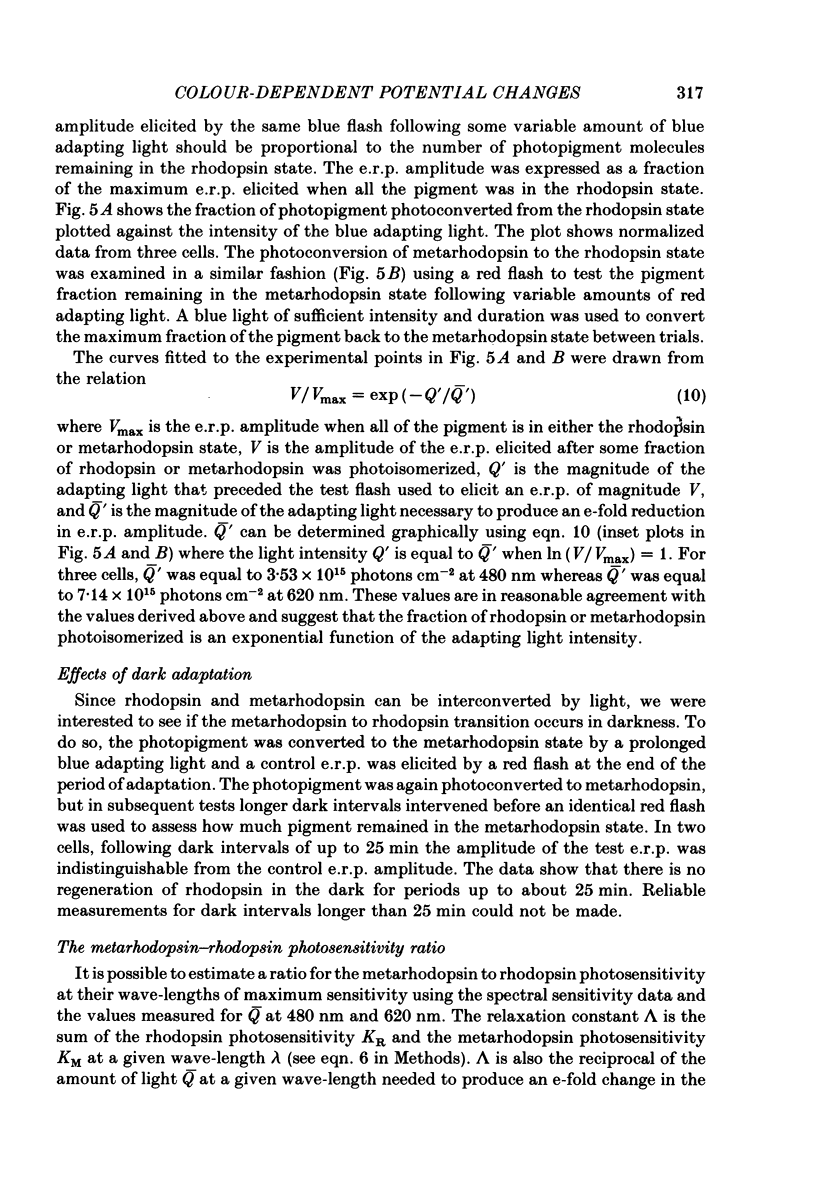
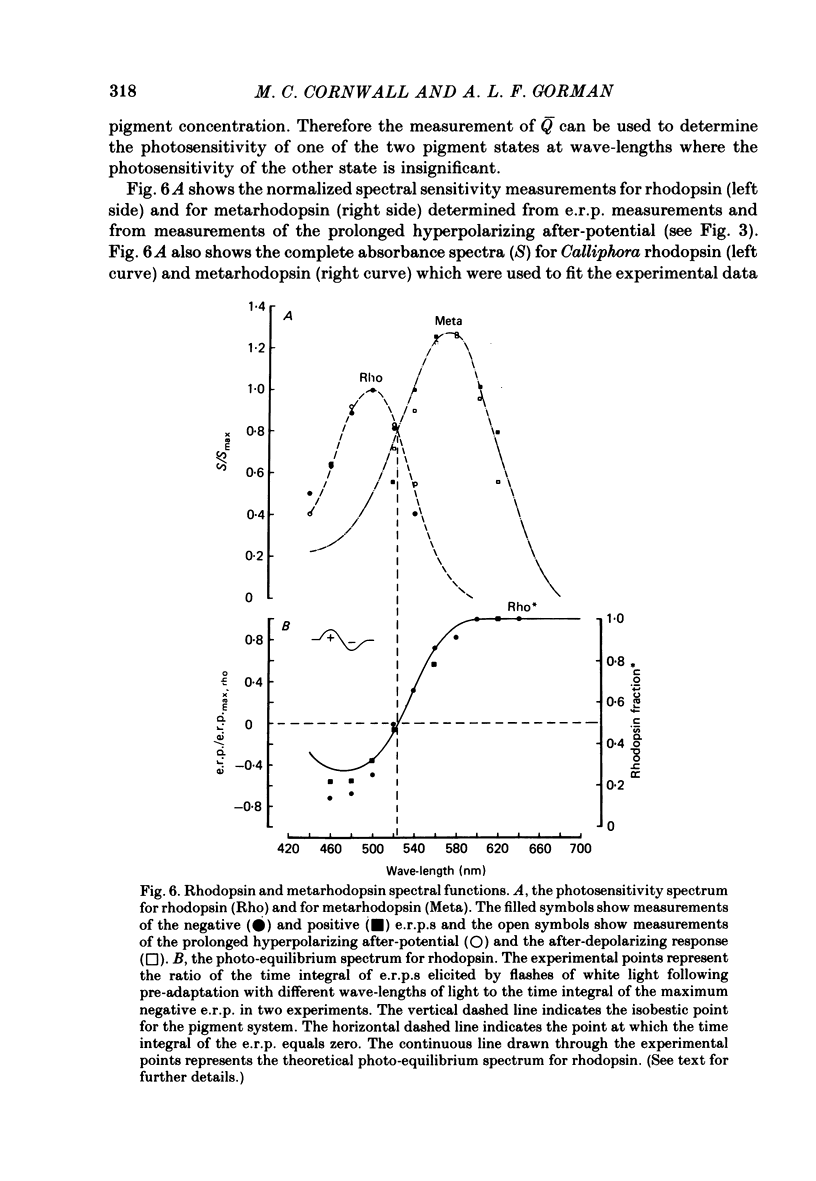
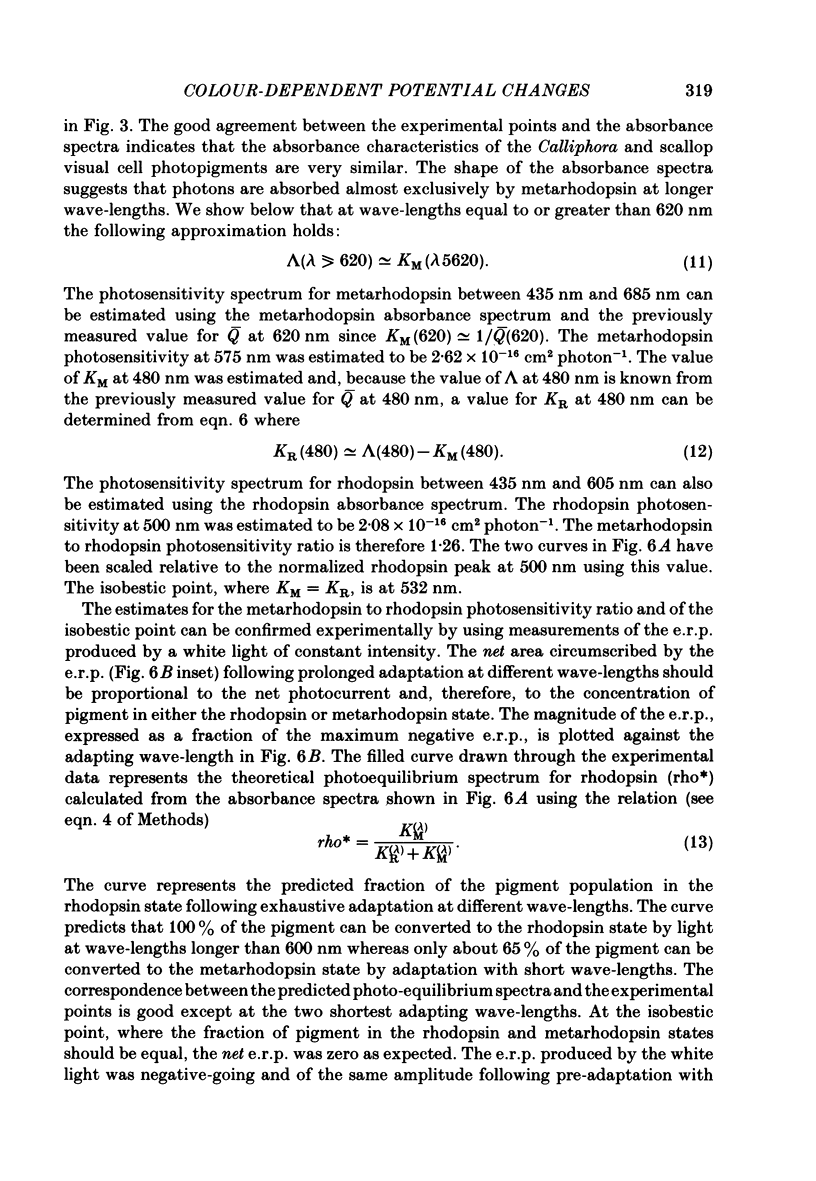
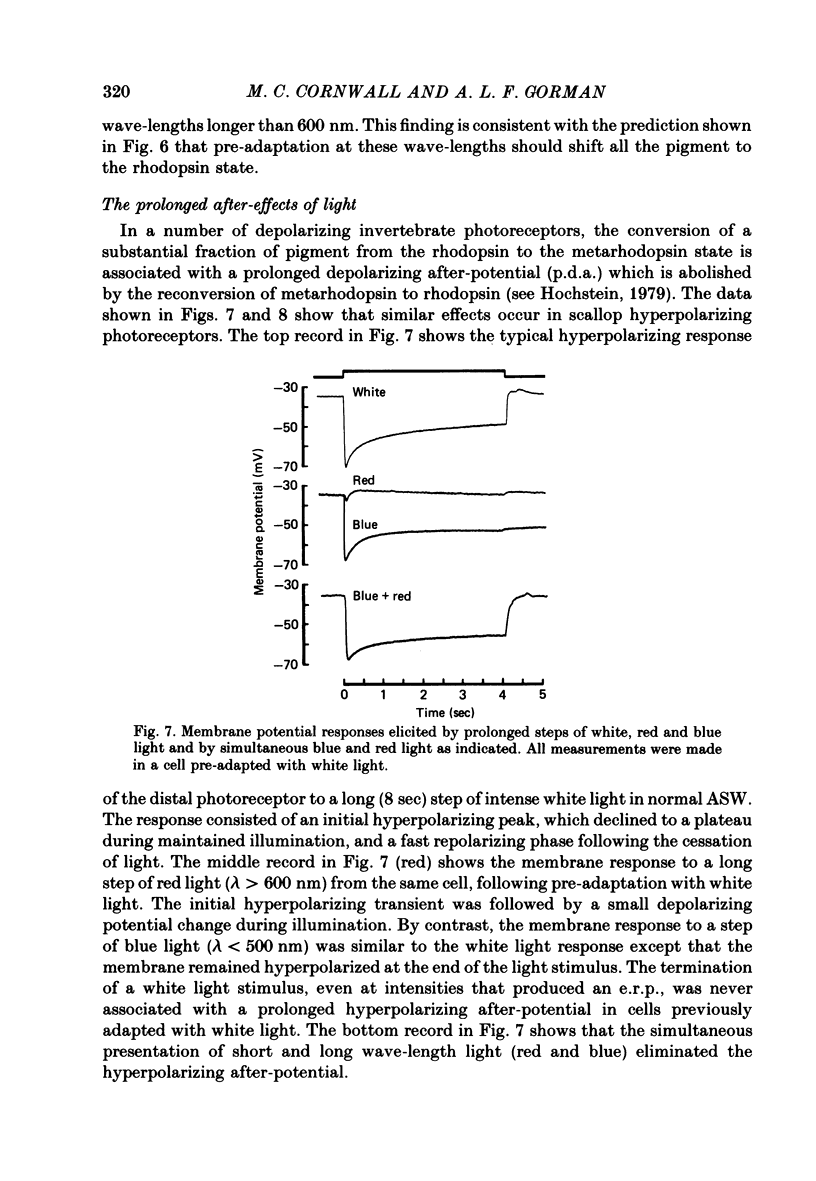
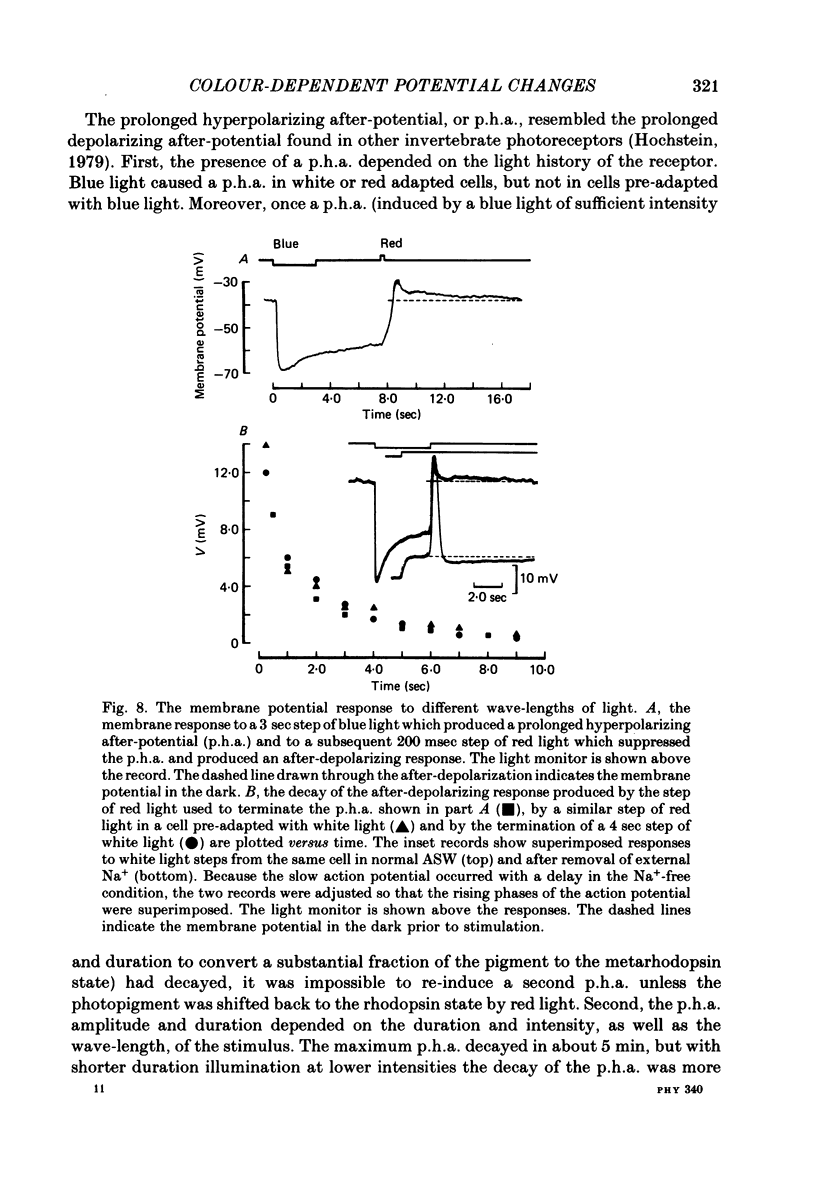
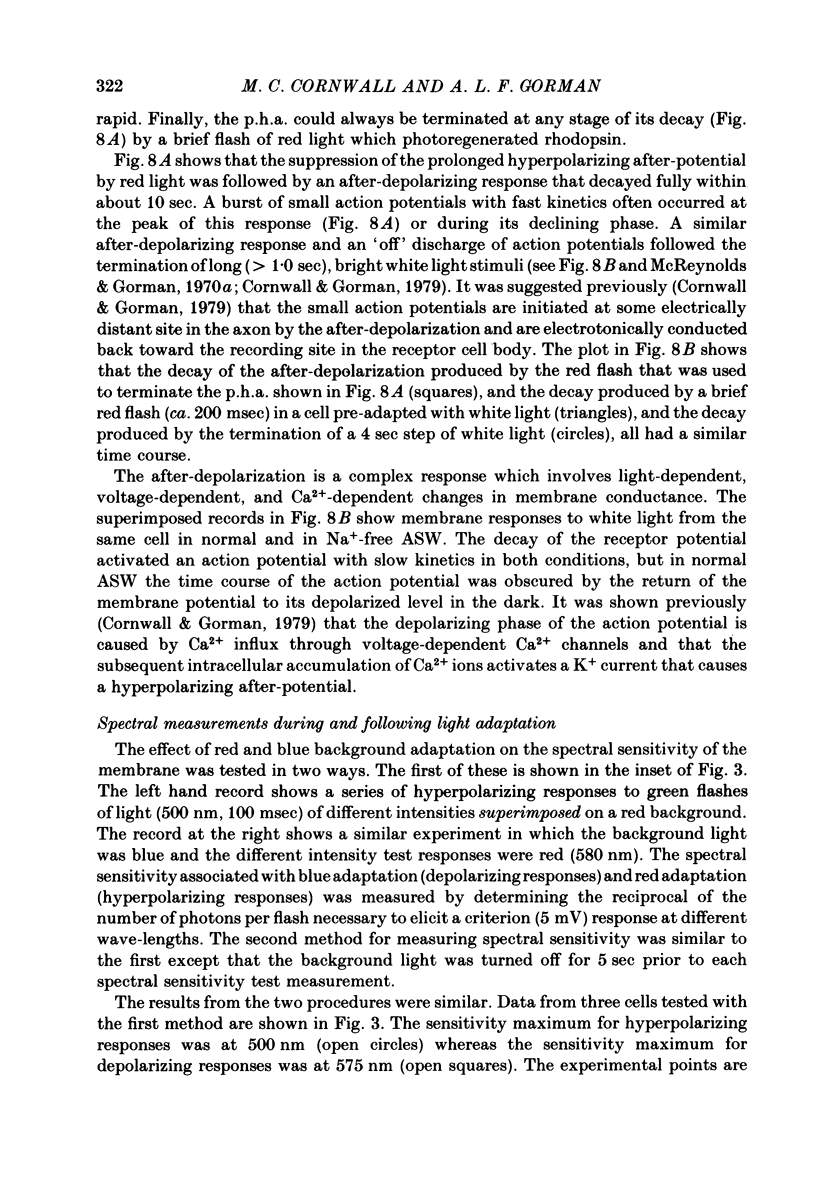
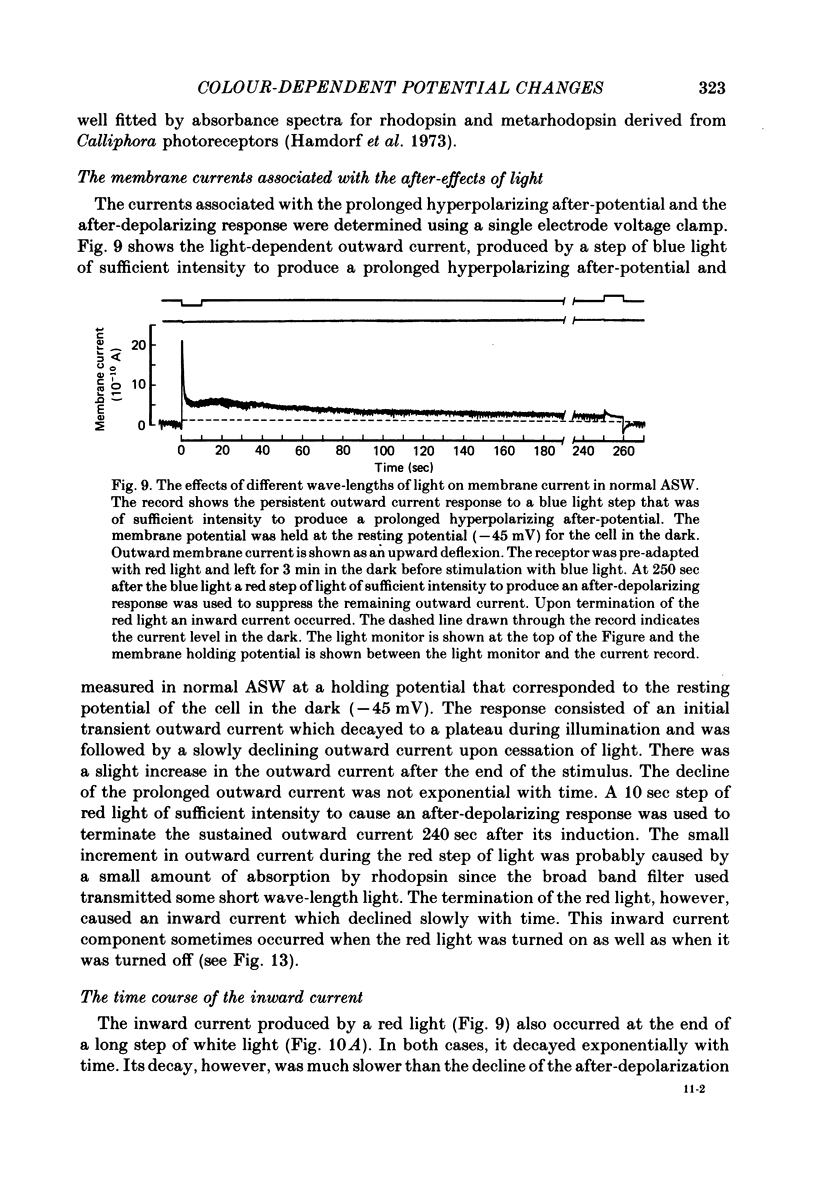
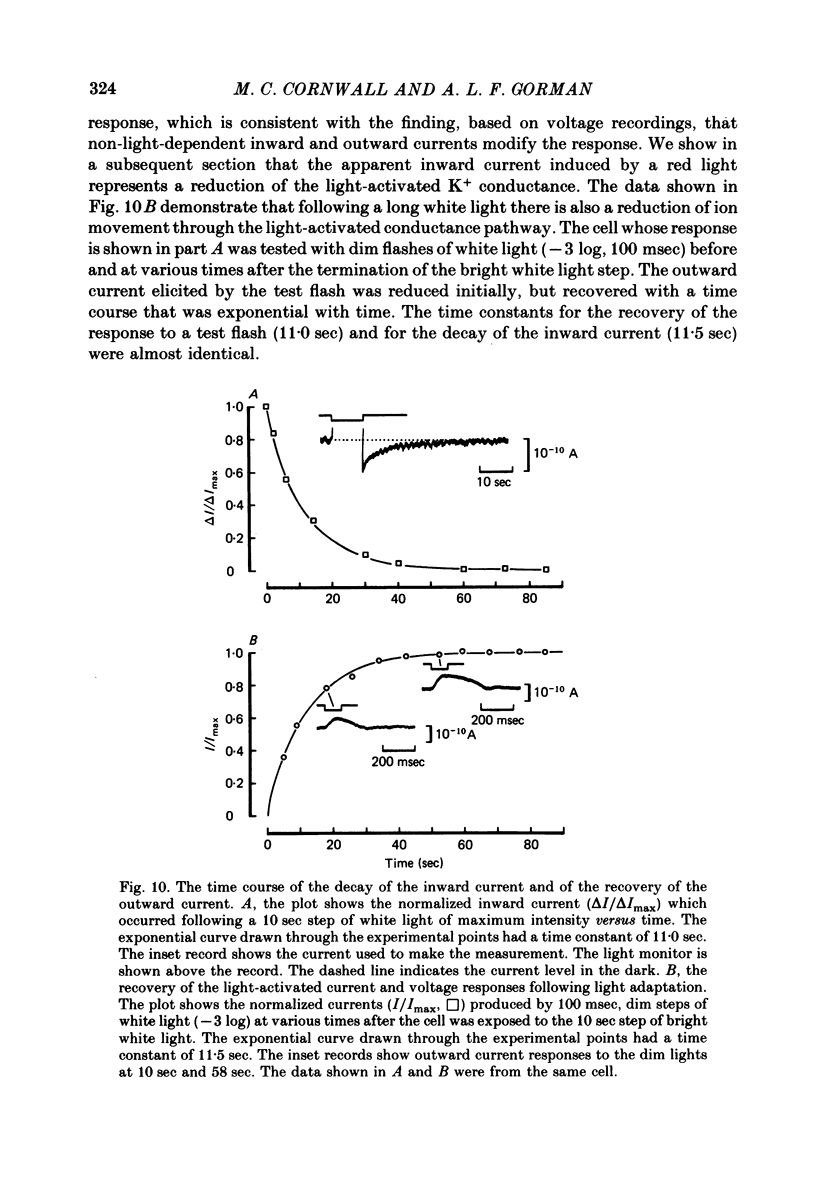
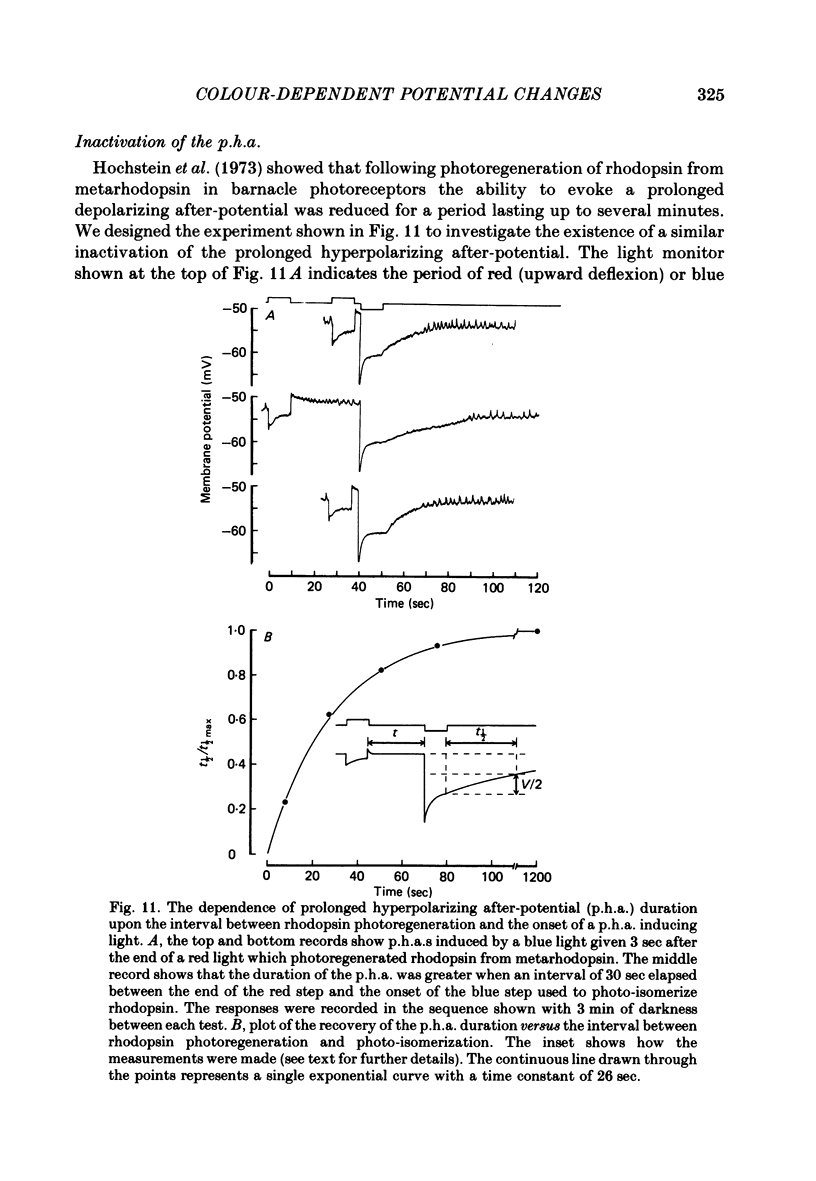
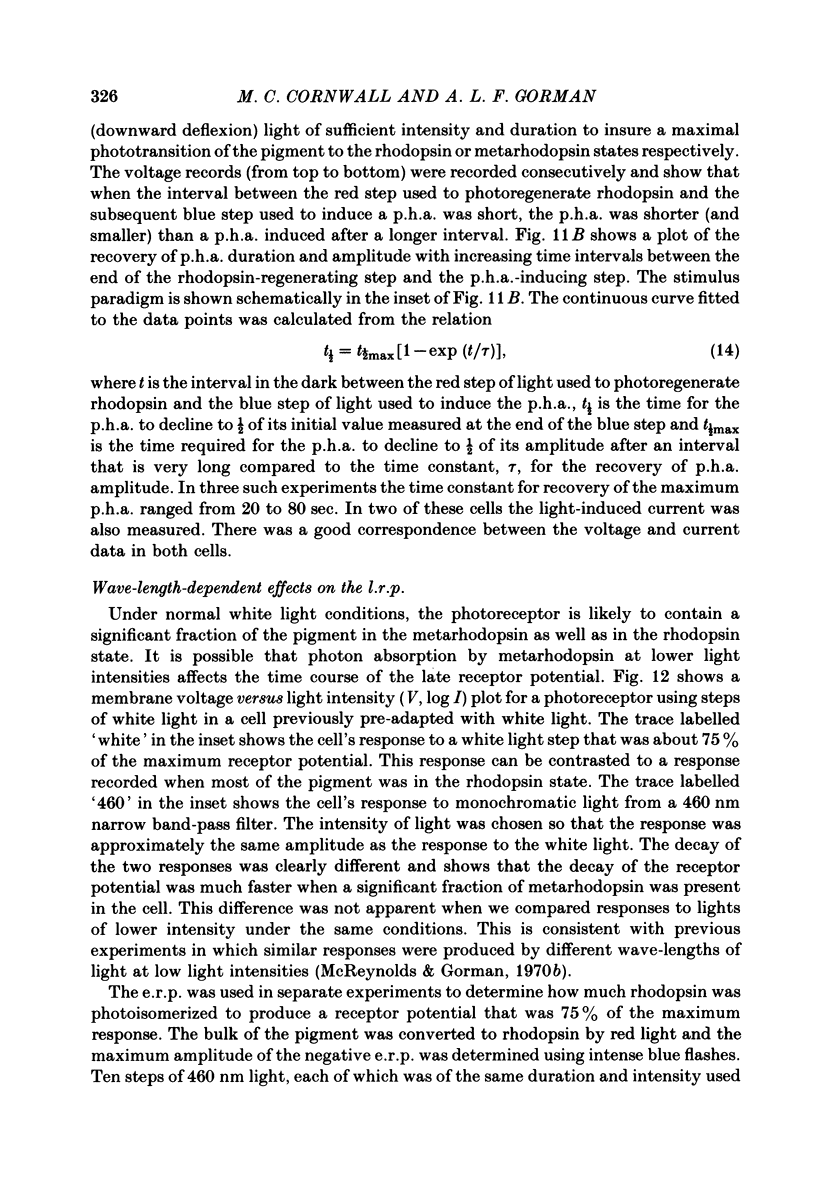
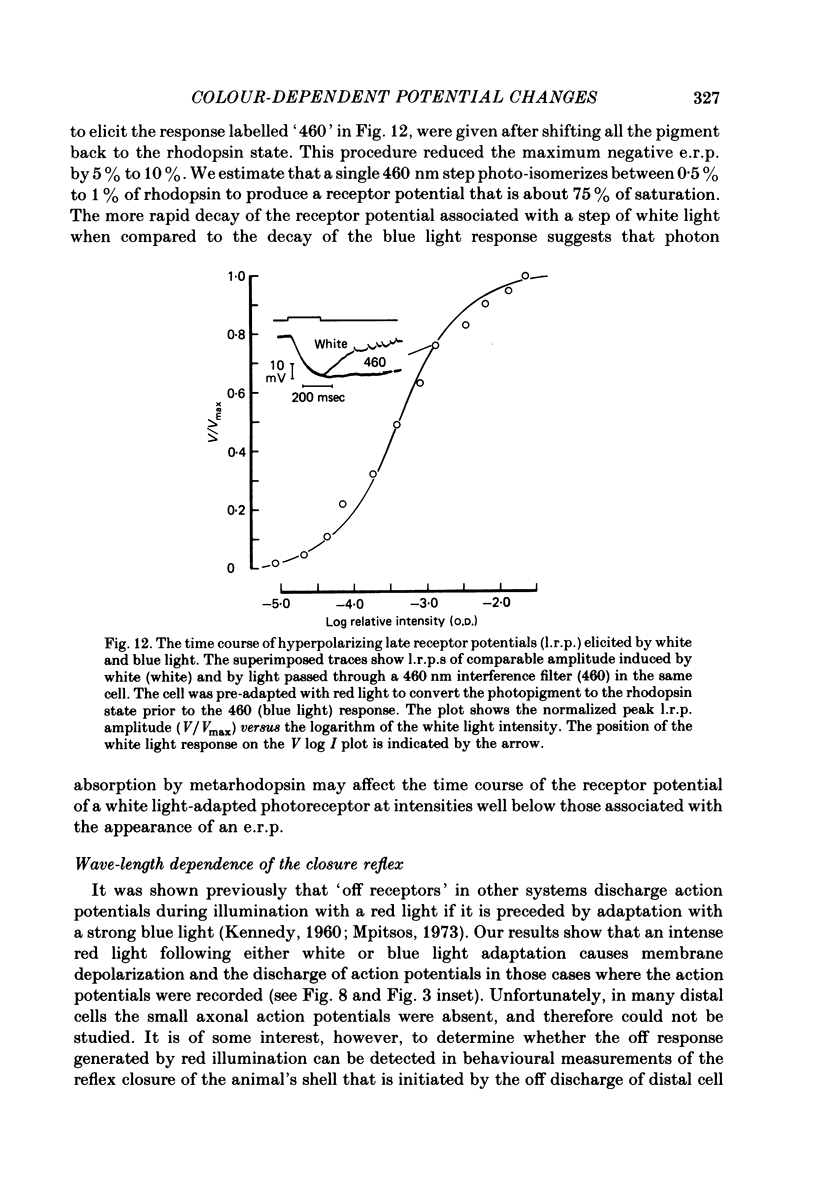
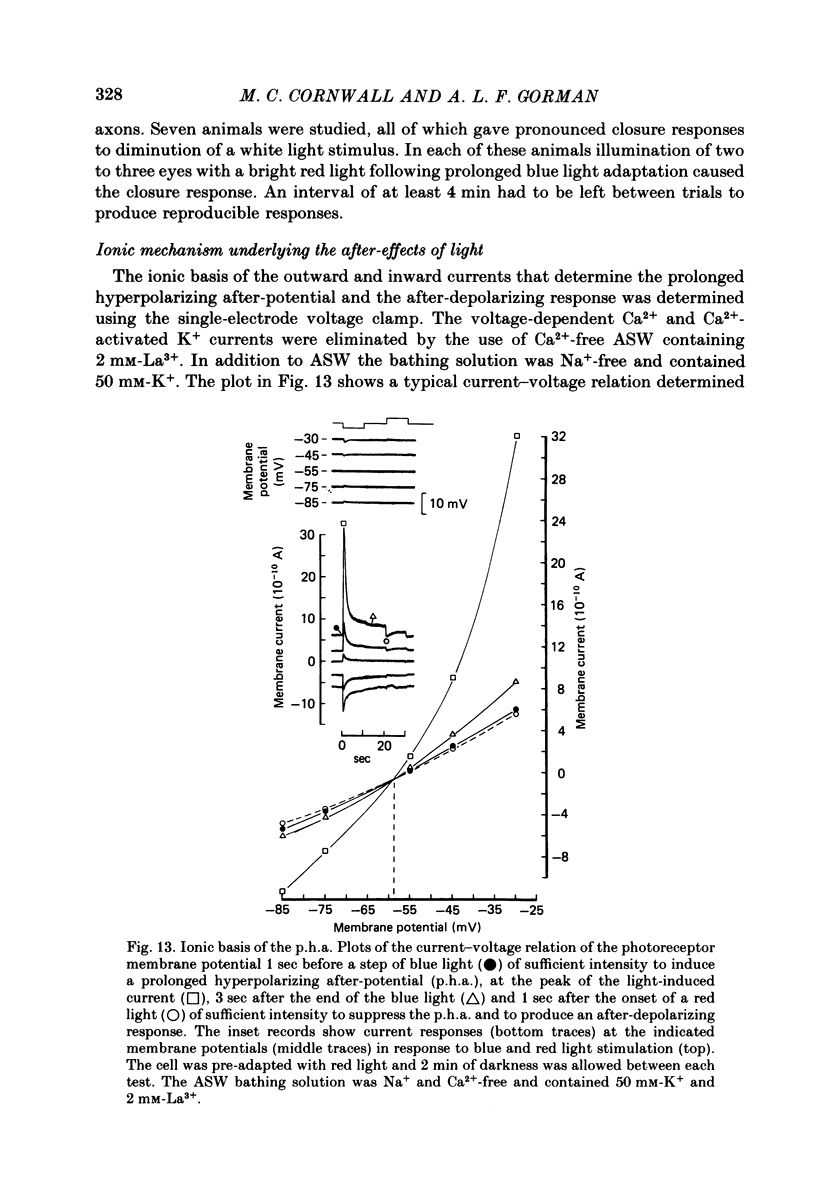
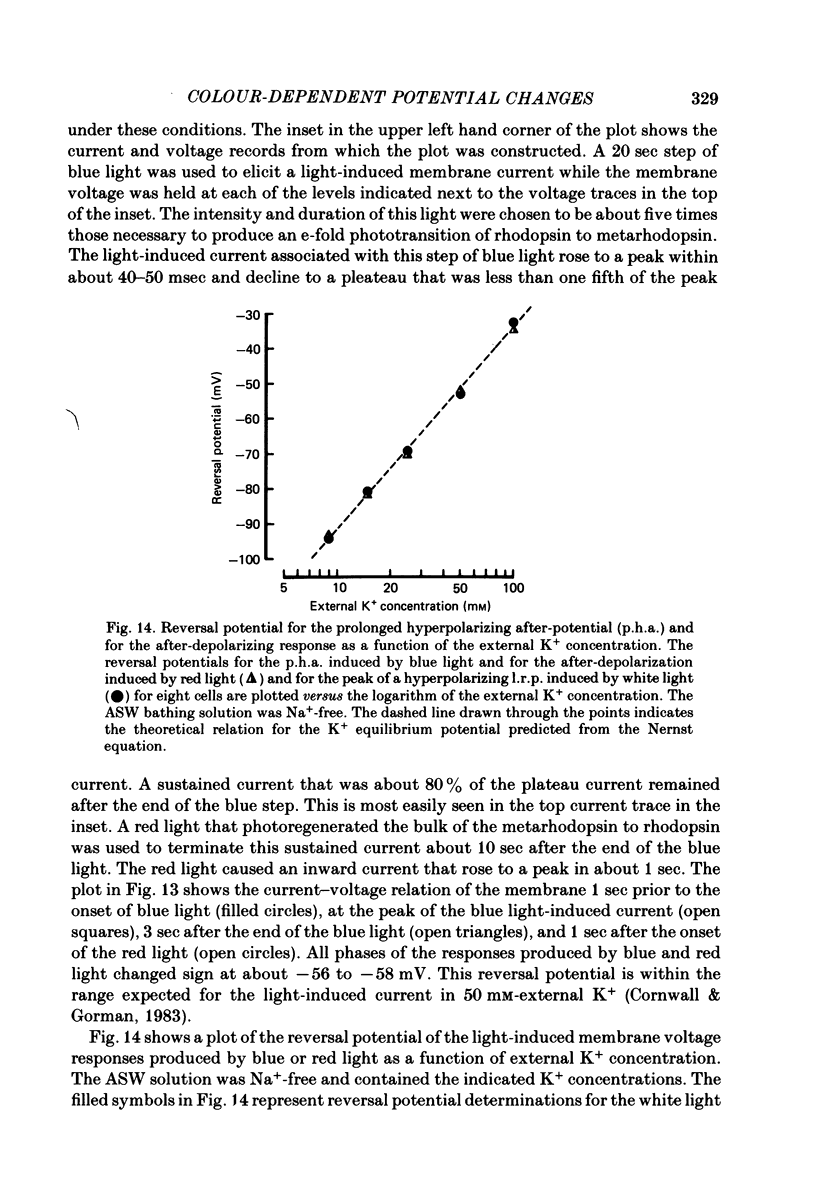
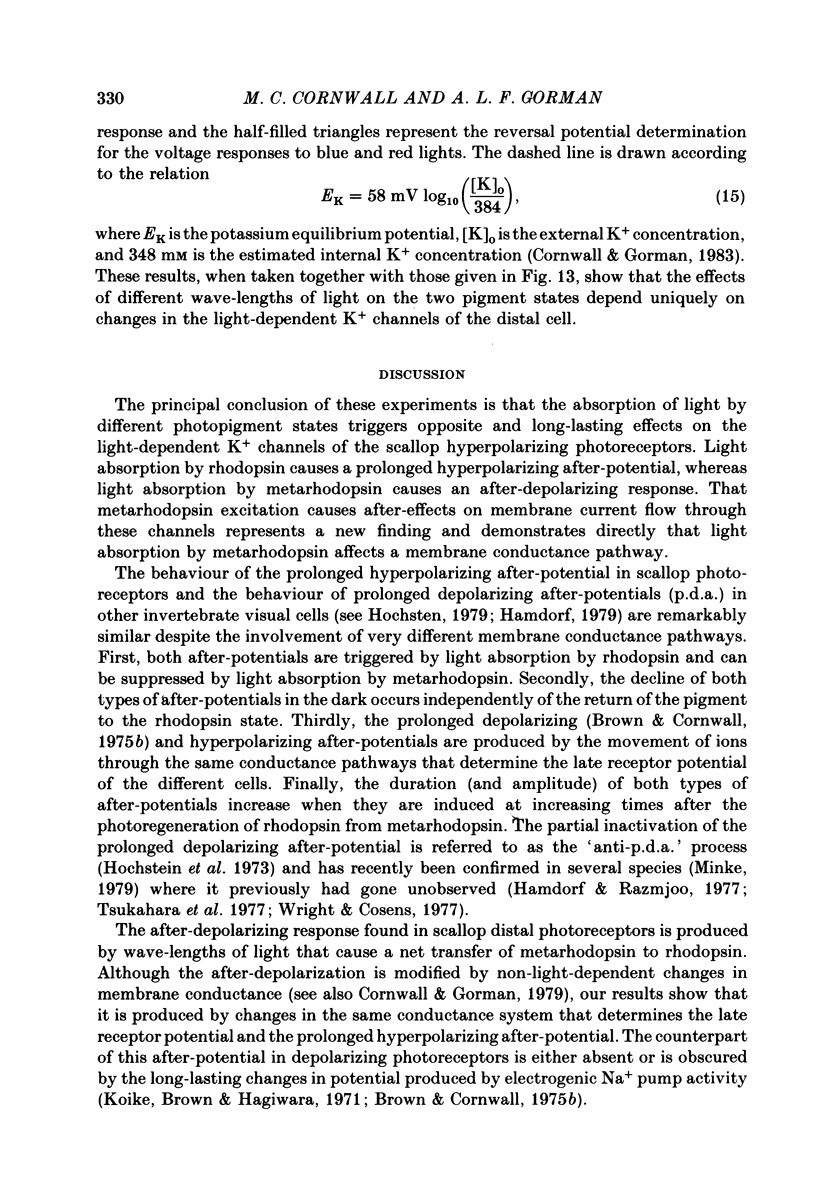
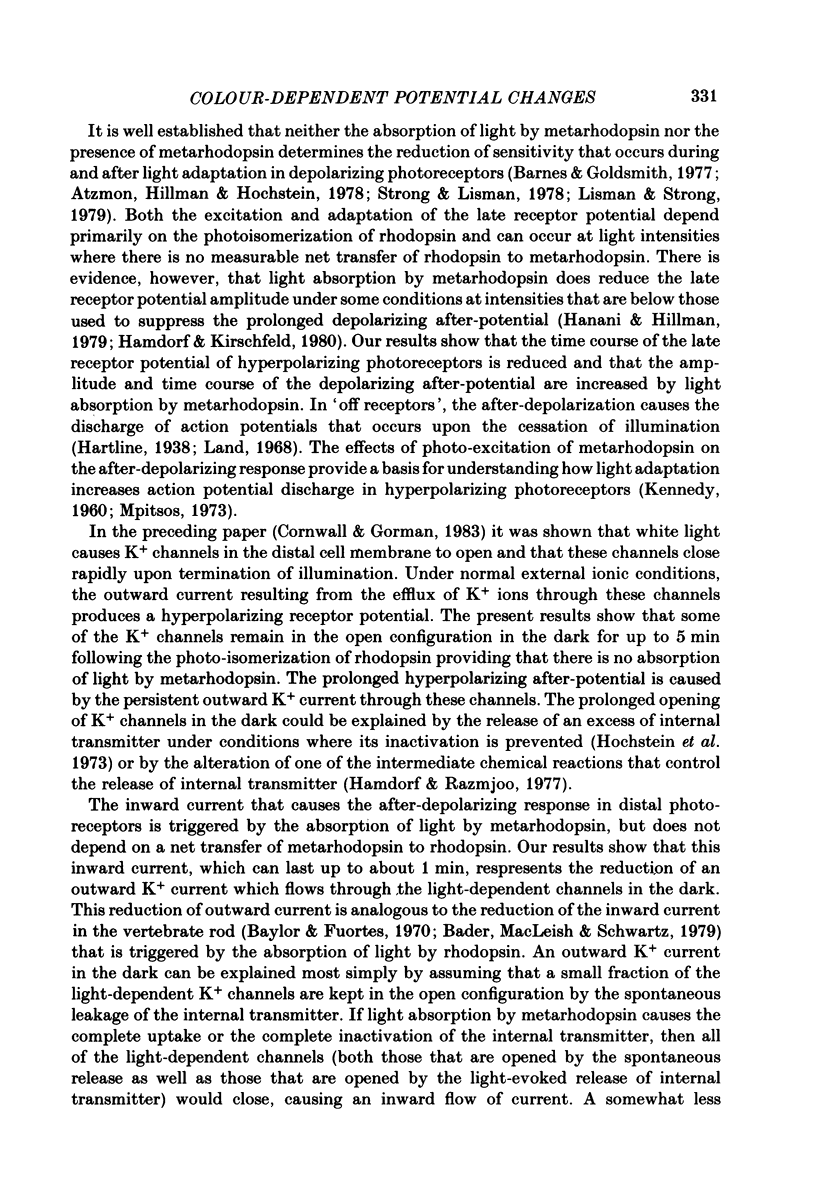
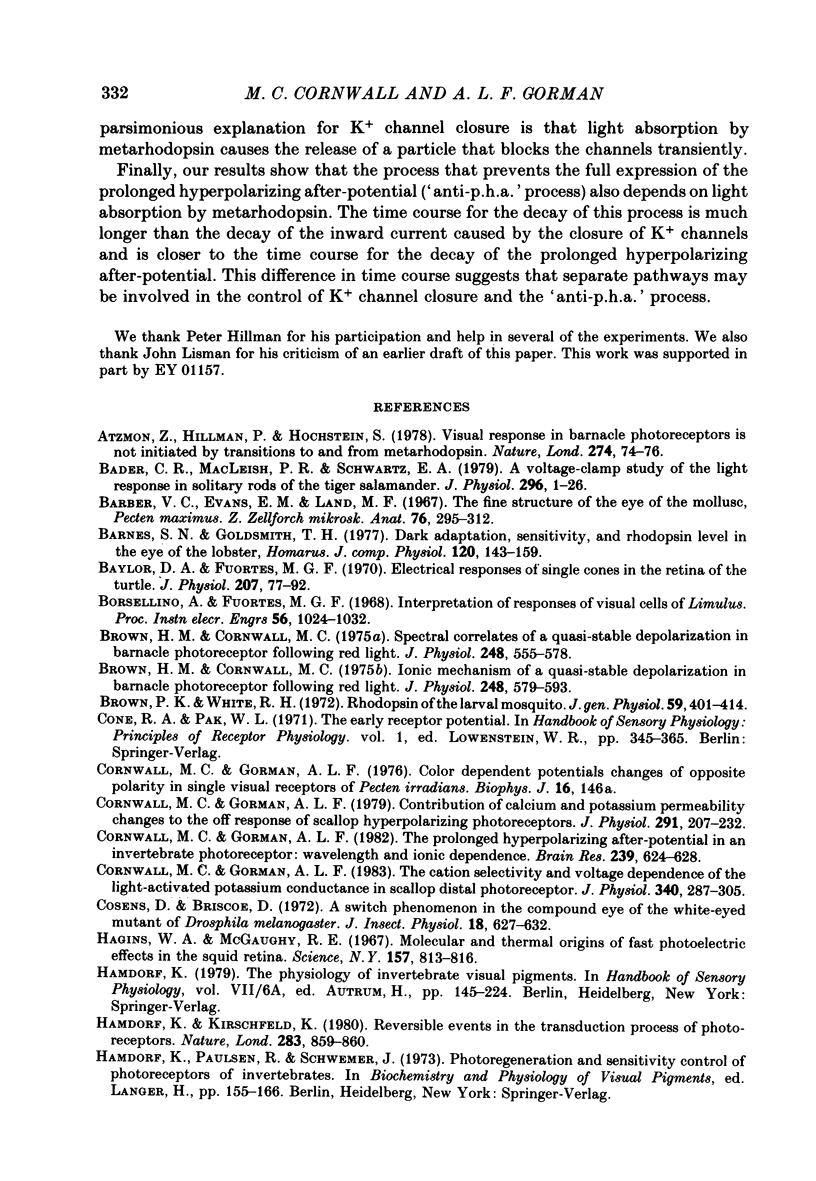
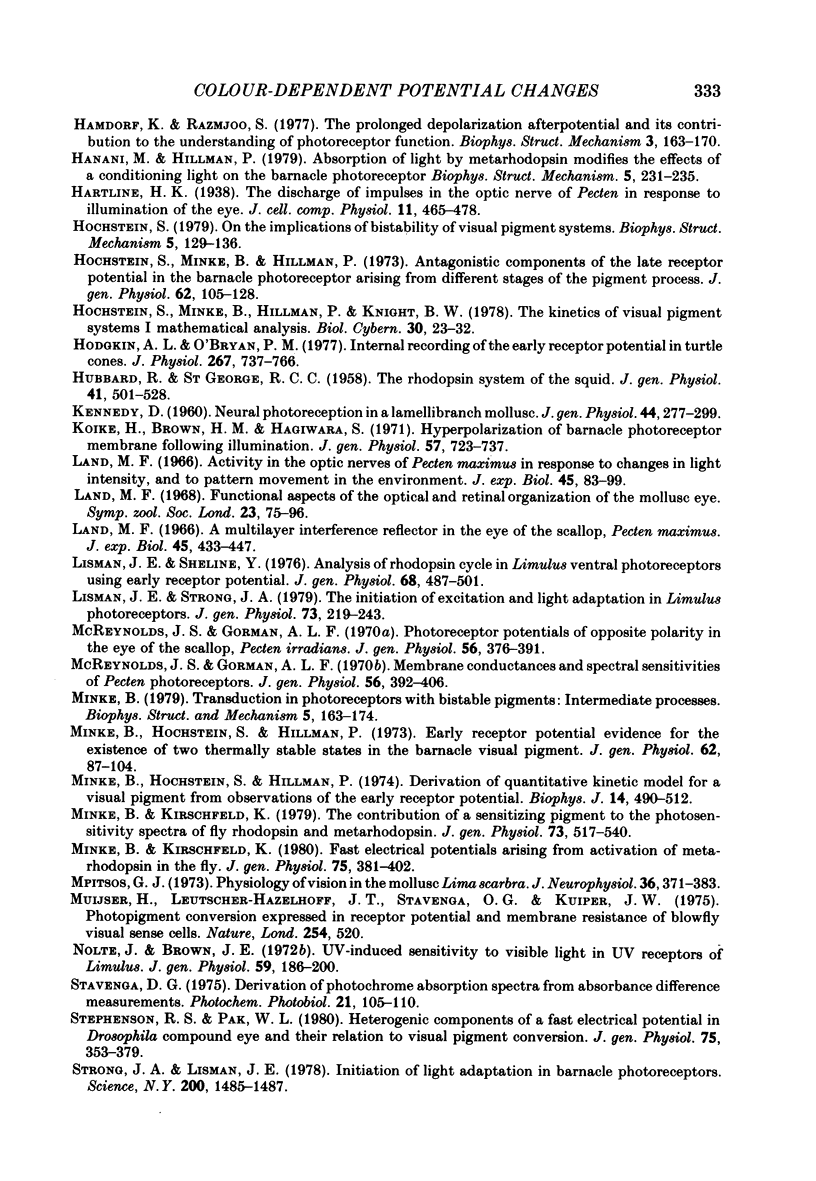
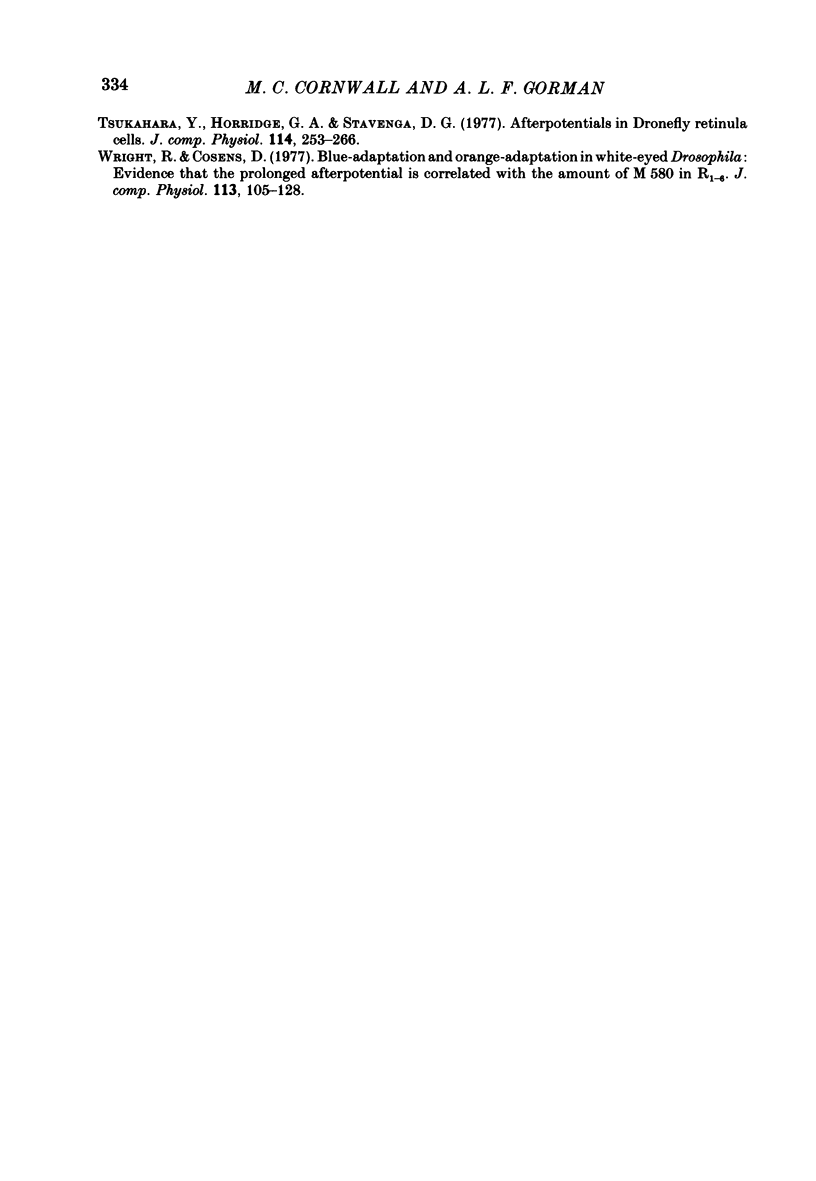
Selected References
These references are in PubMed. This may not be the complete list of references from this article.
- Atzmon Z., Hillman P., Hochstein S. Visual response in barnacle photoreceptors is not initiated by transitions to and from metarhodopsin. Nature. 1978 Jul 6;274(5666):74–76. doi: 10.1038/274074a0. [DOI] [PubMed] [Google Scholar]
- Bader C. R., Macleish P. R., Schwartz E. A. A voltage-clamp study of the light response in solitary rods of the tiger salamander. J Physiol. 1979 Nov;296:1–26. doi: 10.1113/jphysiol.1979.sp012988. [DOI] [PMC free article] [PubMed] [Google Scholar]
- Barber V. C., Evans E. M., Land M. F. The fine structure of the eye of the mollusc Pecten maximus. Z Zellforsch Mikrosk Anat. 1967;76(3):25–312. [PubMed] [Google Scholar]
- Baylor D. A., Fuortes M. G. Electrical responses of single cones in the retina of the turtle. J Physiol. 1970 Mar;207(1):77–92. doi: 10.1113/jphysiol.1970.sp009049. [DOI] [PMC free article] [PubMed] [Google Scholar]
- Brown H. M., Cornwall M. C. Ionic mechanism of a quasi-stable depolarization in barnacle photoreceptor following red light. J Physiol. 1975 Jul;248(3):579–593. doi: 10.1113/jphysiol.1975.sp010989. [DOI] [PMC free article] [PubMed] [Google Scholar]
- Brown H. M., Cornwall M. C. Spectral correlates of a quasi-stable depolarization in barnacle photoreceptor following red light. J Physiol. 1975 Jul;248(3):555–578. doi: 10.1113/jphysiol.1975.sp010988. [DOI] [PMC free article] [PubMed] [Google Scholar]
- Brown P. K., White R. H. Rhodopsin of the larval mosquito. J Gen Physiol. 1972 Apr;59(4):401–414. doi: 10.1085/jgp.59.4.401. [DOI] [PMC free article] [PubMed] [Google Scholar]
- Cornwall M. C., Gorman A. L. Contribution of calcium and potassium permeability changes to the off response of scallop hyperpolarizing photoreceptors. J Physiol. 1979 Jun;291:207–232. doi: 10.1113/jphysiol.1979.sp012808. [DOI] [PMC free article] [PubMed] [Google Scholar]
- Cornwall M. C., Gorman A. L. The cation selectivity and voltage dependence of the light-activated potassium conductance in scallop distal photoreceptor. J Physiol. 1983 Jul;340:287–305. doi: 10.1113/jphysiol.1983.sp014763. [DOI] [PMC free article] [PubMed] [Google Scholar]
- Cornwall M. C., Gorman A. L. The prolonged hyperpolarizing afterpotential in an invertebrate photoreceptor: wavelength and ionic dependence. Brain Res. 1982 May 13;239(2):624–628. doi: 10.1016/0006-8993(82)90539-x. [DOI] [PubMed] [Google Scholar]
- HUBBARD R., ST GEORGE R. C. The rhodopsin system of the squid. J Gen Physiol. 1958 Jan 20;41(3):501–528. doi: 10.1085/jgp.41.3.501. [DOI] [PMC free article] [PubMed] [Google Scholar]
- Hagins W. A., McGaughy R. E. Molecular and thermal origins of fast photoelectric effects in the squid retina. Science. 1967 Aug 18;157(3790):813–816. doi: 10.1126/science.157.3790.813. [DOI] [PubMed] [Google Scholar]
- Hamdorf K., Kirschfeld K. Reversible events in the transduction process of photoreceptors. Nature. 1980 Feb 28;283(5750):859–860. doi: 10.1038/283859a0. [DOI] [PubMed] [Google Scholar]
- Hamdorf K., Razmjoo S. The prolonged depolarizing afterpotential and its contribution to the understanding of photoreceptor function. Biophys Struct Mech. 1977 Jun 29;3(2):163–170. doi: 10.1007/BF00535813. [DOI] [PubMed] [Google Scholar]
- Hochstein S., Minke B., Hillman P. Antagonistic components of the late receptor potential in the barnacle photoreceptor arising from different stages of the pigment process. J Gen Physiol. 1973 Jul;62(1):105–128. doi: 10.1085/jgp.62.1.105. [DOI] [PMC free article] [PubMed] [Google Scholar]
- Hochstein S., Minke B., Hillman P., Knight B. W. The kinetics of visual pigment systems. I. Mathematical analysis. Biol Cybern. 1978 Jul 14;30(1):23–32. doi: 10.1007/BF00365480. [DOI] [PubMed] [Google Scholar]
- Hodgkin A. L., Obryan P. M. Internal recording of the early receptor potential in turtle cones. J Physiol. 1977 Jun;267(3):737–766. doi: 10.1113/jphysiol.1977.sp011836. [DOI] [PMC free article] [PubMed] [Google Scholar]
- KENNEDY D. Neural photoreception in a lamellibranch mollusc. J Gen Physiol. 1960 Nov;44:277–299. doi: 10.1085/jgp.44.2.277. [DOI] [PMC free article] [PubMed] [Google Scholar]
- Koike H., Brown H. M., Hagiwara S. Hyperpolarization of a barnacle photoreceptor membrane following illumination. J Gen Physiol. 1971 Jun;57(6):723–737. doi: 10.1085/jgp.57.6.723. [DOI] [PMC free article] [PubMed] [Google Scholar]
- Land M. F. Activity in the optic nerve of Pecten maximus in response to changes in light intensity, and to pattern and movement in the optical environment. J Exp Biol. 1966 Aug;45(1):83–99. doi: 10.1242/jeb.45.1.83. [DOI] [PubMed] [Google Scholar]
- Lisman J. E., Sheline Y. Analysis of the rhodopsin cycle in limulus ventral photoreceptors using the early receptor potential. J Gen Physiol. 1976 Nov;68(5):487–501. doi: 10.1085/jgp.68.5.487. [DOI] [PMC free article] [PubMed] [Google Scholar]
- Lisman J. E., Strong J. A. The initiation of excitation and light adaptation in Limulus ventral photoreceptors. J Gen Physiol. 1979 Feb;73(2):219–243. doi: 10.1085/jgp.73.2.219. [DOI] [PMC free article] [PubMed] [Google Scholar]
- McReynolds J. S., Gorman A. L. Membrane conductances and spectral sensitivities of Pecten photoreceptors. J Gen Physiol. 1970 Sep;56(3):392–406. doi: 10.1085/jgp.56.3.392. [DOI] [PMC free article] [PubMed] [Google Scholar]
- McReynolds J. S., Gorman A. L. Photoreceptor potentials of opposite polarity in the eye of the scallop, Pecten irradians. J Gen Physiol. 1970 Sep;56(3):376–391. doi: 10.1085/jgp.56.3.376. [DOI] [PMC free article] [PubMed] [Google Scholar]
- Minke B., Hochstein S., Hillman P. Derivation of a quantitative kinetic model for a visual pigment from observations of early receptor potential. Biophys J. 1974 Jun;14(6):490–512. doi: 10.1016/S0006-3495(74)85929-1. [DOI] [PMC free article] [PubMed] [Google Scholar]
- Minke B., Hochstein S., Hillman P. Early receptor potential evidence for the existence of two thermally stable states in the barnacle visual pigment. J Gen Physiol. 1973 Jul;62(1):87–104. doi: 10.1085/jgp.62.1.87. [DOI] [PMC free article] [PubMed] [Google Scholar]
- Minke B., Kirschfeld K. Fast electrical potentials arising from activation of metarhodopsin in the fly. J Gen Physiol. 1980 Apr;75(4):381–402. doi: 10.1085/jgp.75.4.381. [DOI] [PMC free article] [PubMed] [Google Scholar]
- Minke B., Kirschfeld K. The contribution of a sensitizing pigment to the photosensitivity spectra of fly rhodopsin and metarhodopsin. J Gen Physiol. 1979 May;73(5):517–540. doi: 10.1085/jgp.73.5.517. [DOI] [PMC free article] [PubMed] [Google Scholar]
- Mpitosos G. J. Physiology of vision in the mollusk Lima scabra. J Neurophysiol. 1973 Mar;36(2):371–383. doi: 10.1152/jn.1973.36.2.371. [DOI] [PubMed] [Google Scholar]
- Muijser H., Leutscher-Hazelhoff J. T., Stavenga D. G., Kuiper J. W. Photopigment conversions expressed in receptor potential and membrane resistance of blowfly visual sense cells. Nature. 1975 Apr 10;254(5500):520–522. doi: 10.1038/254520a0. [DOI] [PubMed] [Google Scholar]
- Nolte J., Brown J. E. Ultraviolet-induced sensitivity to visible light in ultraviolet receptors of Limulus. J Gen Physiol. 1972 Feb;59(2):186–200. doi: 10.1085/jgp.59.2.186. [DOI] [PMC free article] [PubMed] [Google Scholar]
- Stavenga D. G. Derivation of photochrome absorption spectra from absorbance difference measurements. Photochem Photobiol. 1975 Feb;21(2):105–110. doi: 10.1111/j.1751-1097.1975.tb06636.x. [DOI] [PubMed] [Google Scholar]
- Stephenson R. S., Pak W. L. Heterogenic components of a fast electrical potential in Drosophila compound eye and their relation to visual pigment photoconversion. J Gen Physiol. 1980 Apr;75(4):353–379. doi: 10.1085/jgp.75.4.353. [DOI] [PMC free article] [PubMed] [Google Scholar]
- Strong J., Lisman J. Initiation of light adaptation in barnacle photoreceptors. Science. 1978 Jun 30;200(4349):1485–1487. doi: 10.1126/science.663629. [DOI] [PubMed] [Google Scholar]