Abstract
Enzymatic hydrolysis of acetylcholine (ACh) was determined in intact frog sartorius muscles or their homogenates. The Vmax was 29 nmol min-1 in intact muscles and 46 nmol min-1 per muscle in homogenates, and the Km was 6 and 0.2 mM, respectively. The muscle was divided into small segments, which were homogenized; the junctional cholinesterase (ChE) accounted for 60% of total enzyme activity. At low substrate concentrations the rate of hydrolysis was up to 30 times higher in homogenates than in intact muscles. This difference was greatly reduced at very high substrate concentrations. It appears that most of the ChE in intact muscle is 'occluded' to external ACh, mainly because the ChE at the edges of the synaptic cleft prevents the ACh from reaching the enzyme situated further inwards, which consequently does not contribute to its hydrolysis; homogenization makes all synaptic ChE accessible to added ACh. Incubation of sartorius muscles with collagenase caused an 80% decrease in ChE activity (determined in homogenates) of end-plate-containing parts which became similar to that in end-plate-free parts on which collagenase had little effect. Histochemistry showed that the tendon-muscle junction contained folds which were stained intensively for ChE. Diethyldimethylpyrophosphonate , neostigmine, eserine, and di-isopropyl fluorophosphonate inhibited ChE activity in this order of potency. The I50 values (i.e. the concentrations of the drugs which caused a 50% inhibition) were about 5 times higher in intact than in homogenized tissue. Neostigmine, 0.15 and 0.4 microM, increased the time constant of miniature end-plate currents 1.3- and 1.8-fold, and slowed down ChE activity of muscle homogenates by 1.4 and 2.1 times, respectively, without significantly affecting ACh hydrolysis by intact muscles. This indicates that synaptic ChE is not present in large excess. It is concluded that ChE activity measured in homogenates presents a better picture of in situ ChE activity than that measured in whole muscles especially for evaluating the effect of ChE inhibitors. A mathematical model for ChE-hindered diffusion of ACh is presented in an Appendix.
Full text
PDF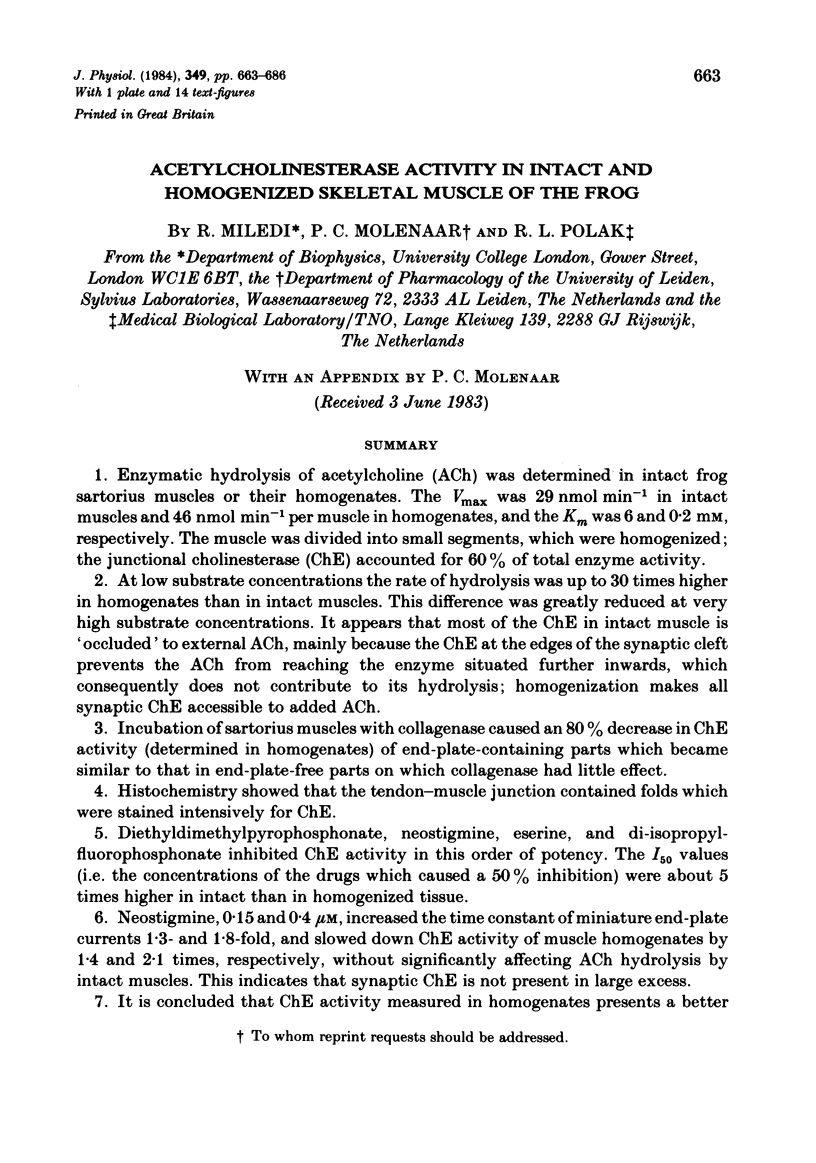
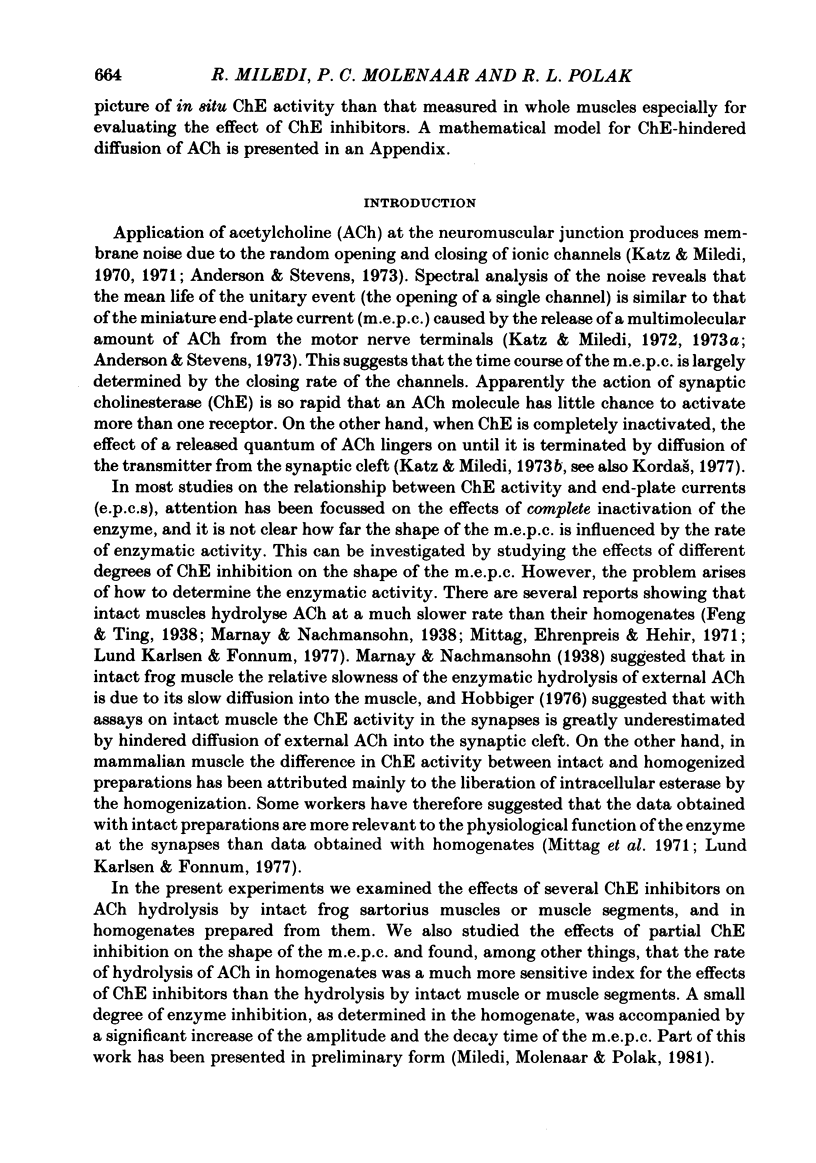
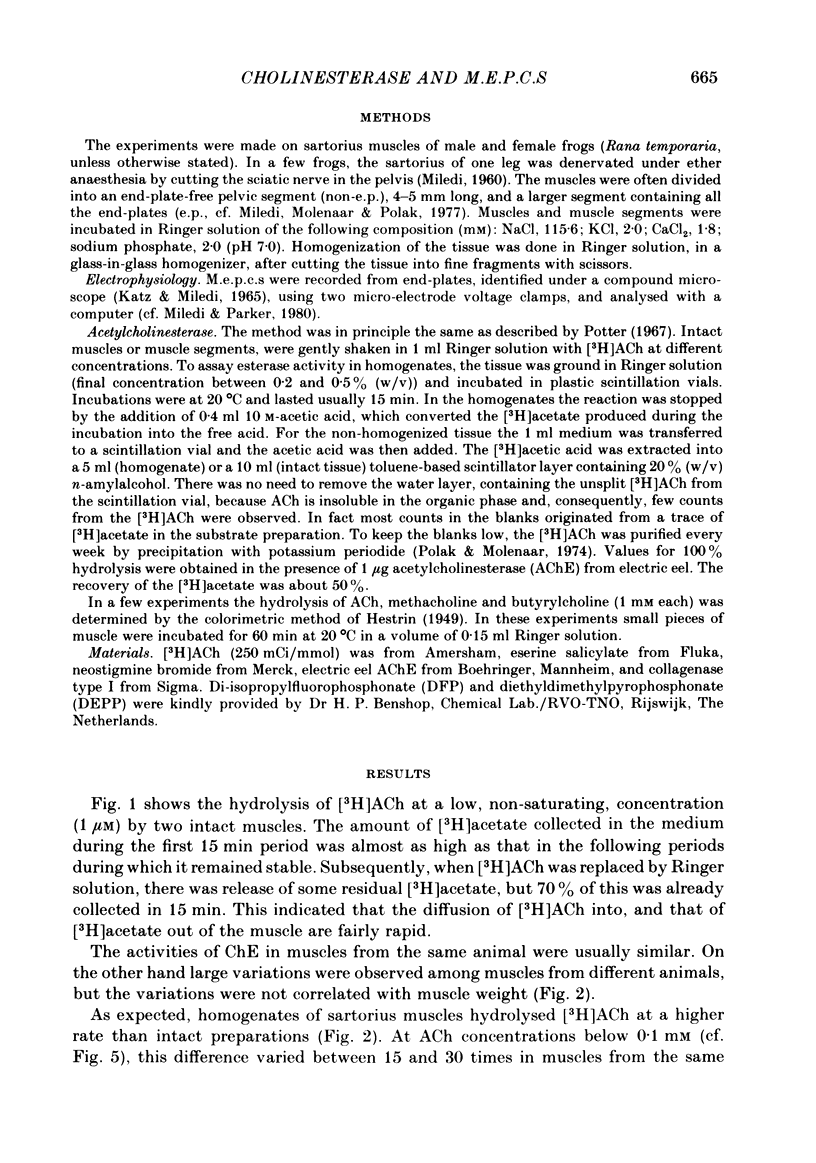
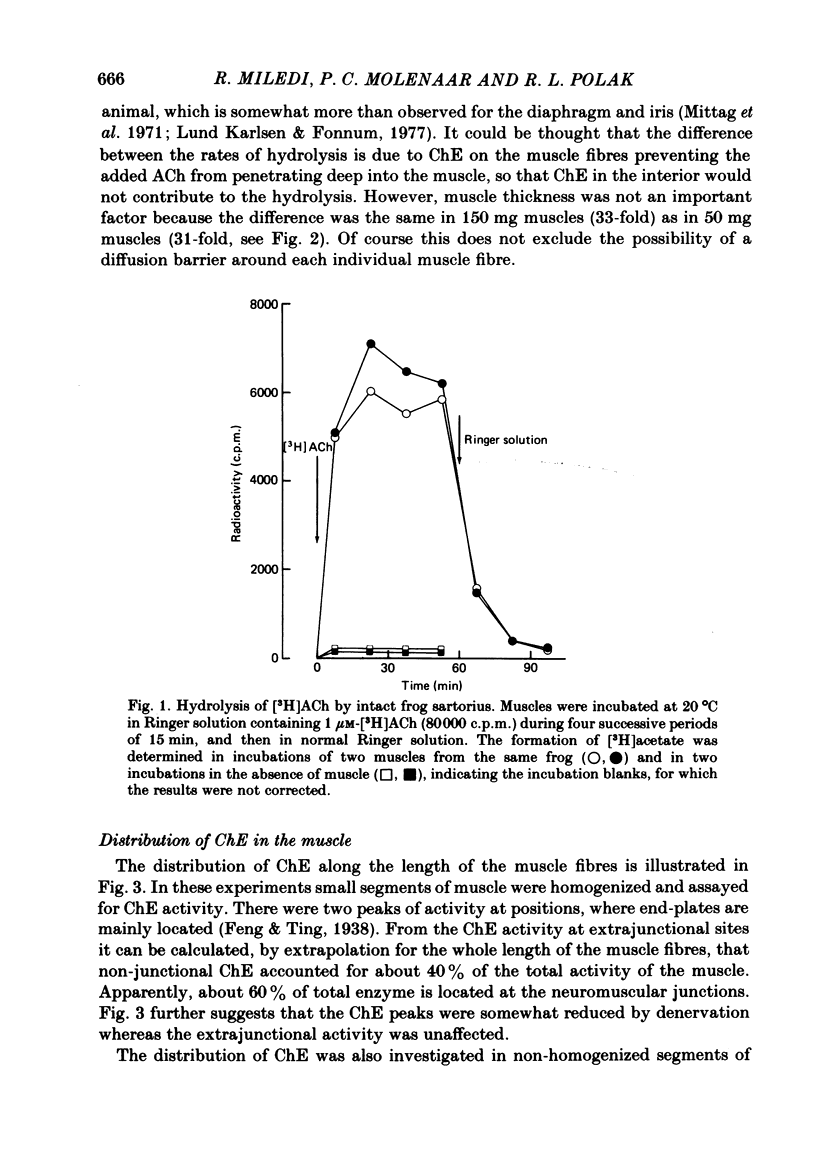
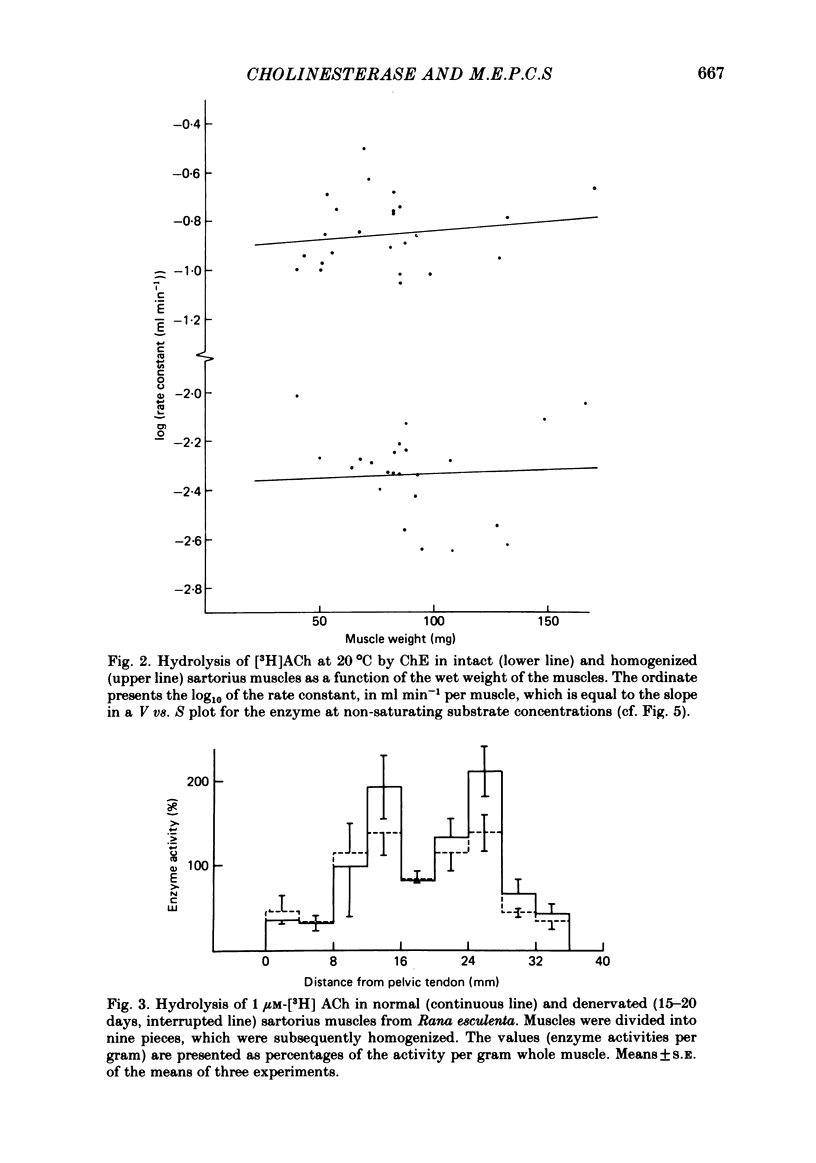
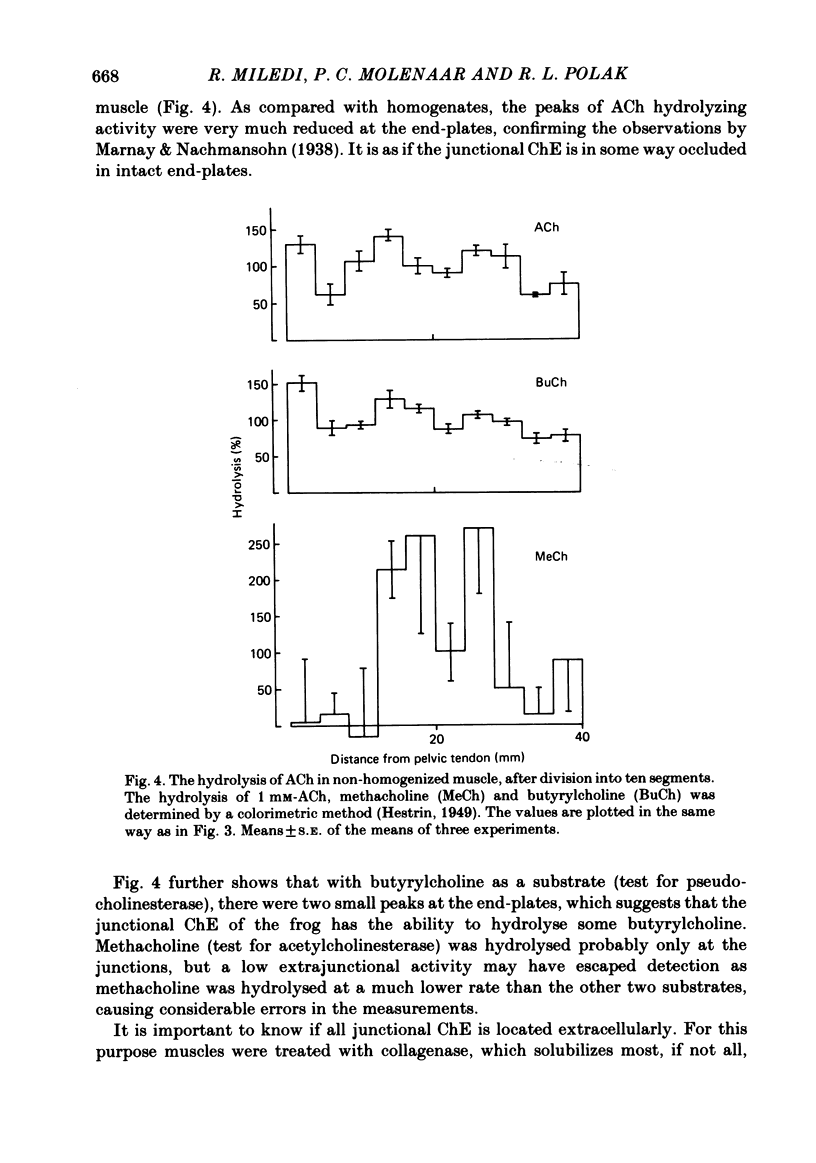
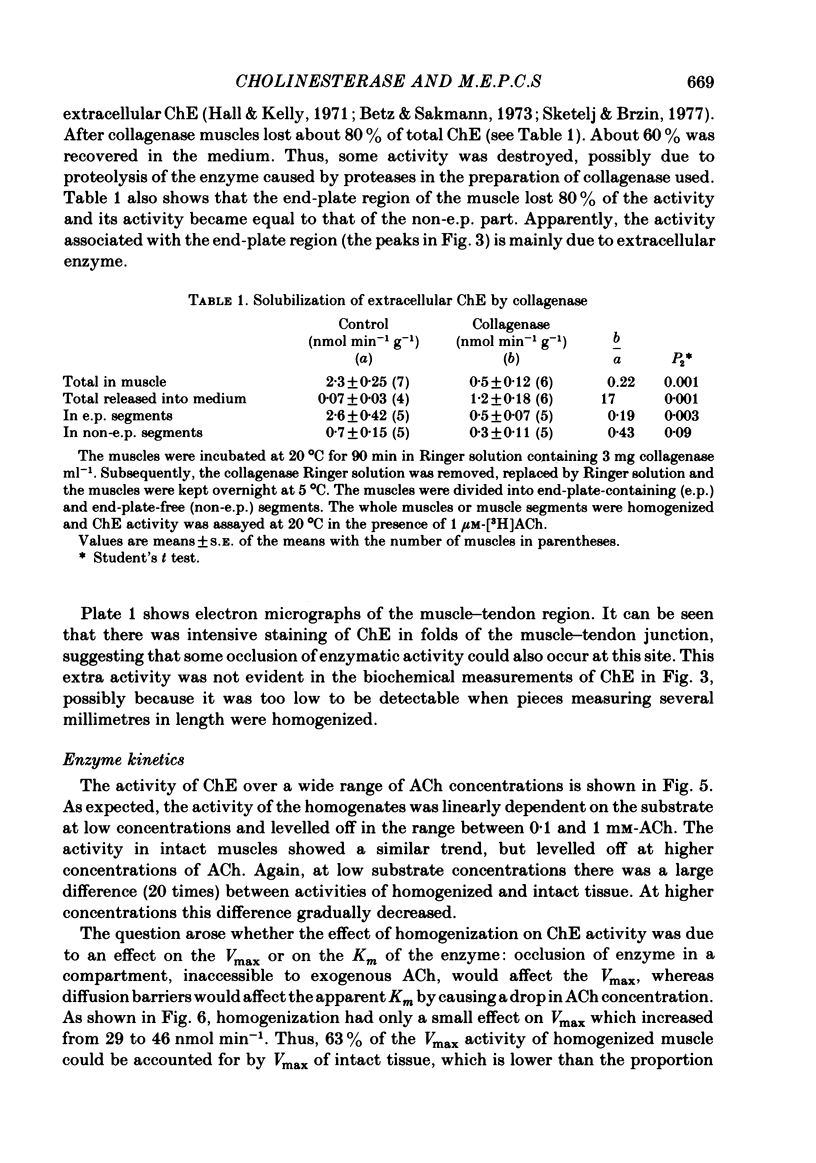
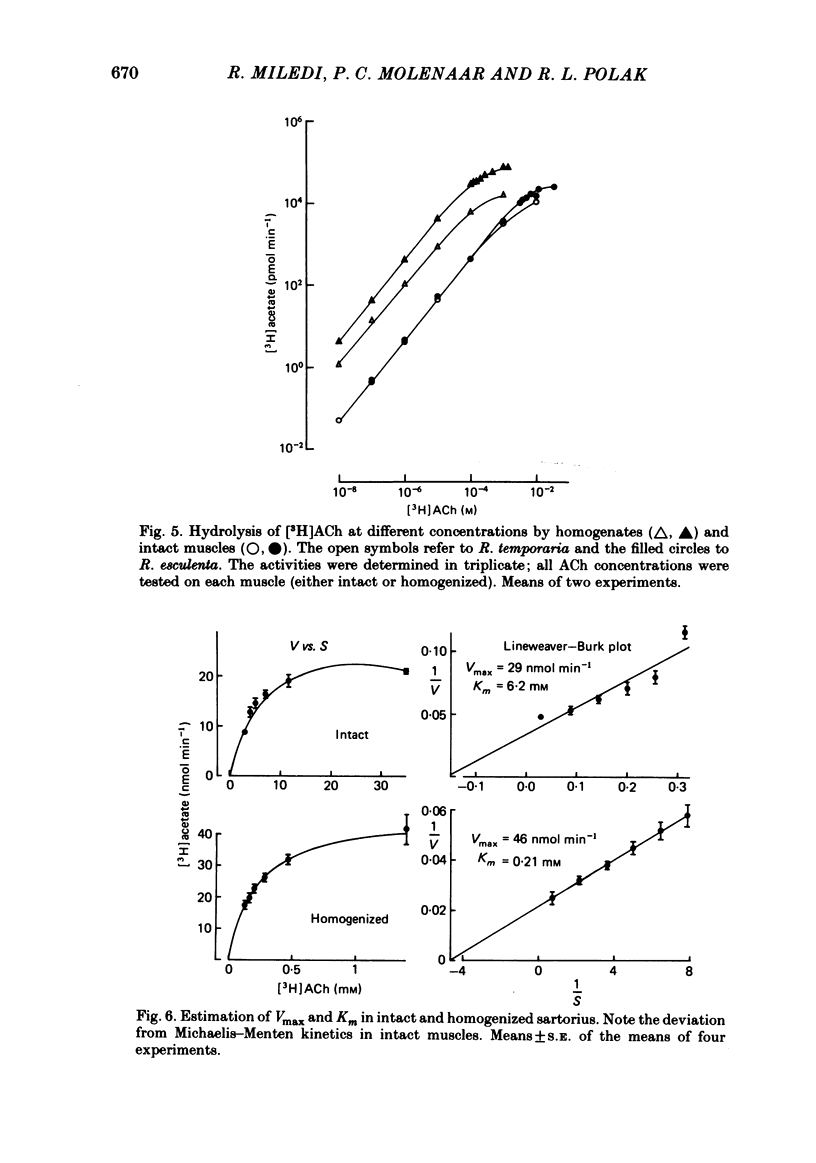
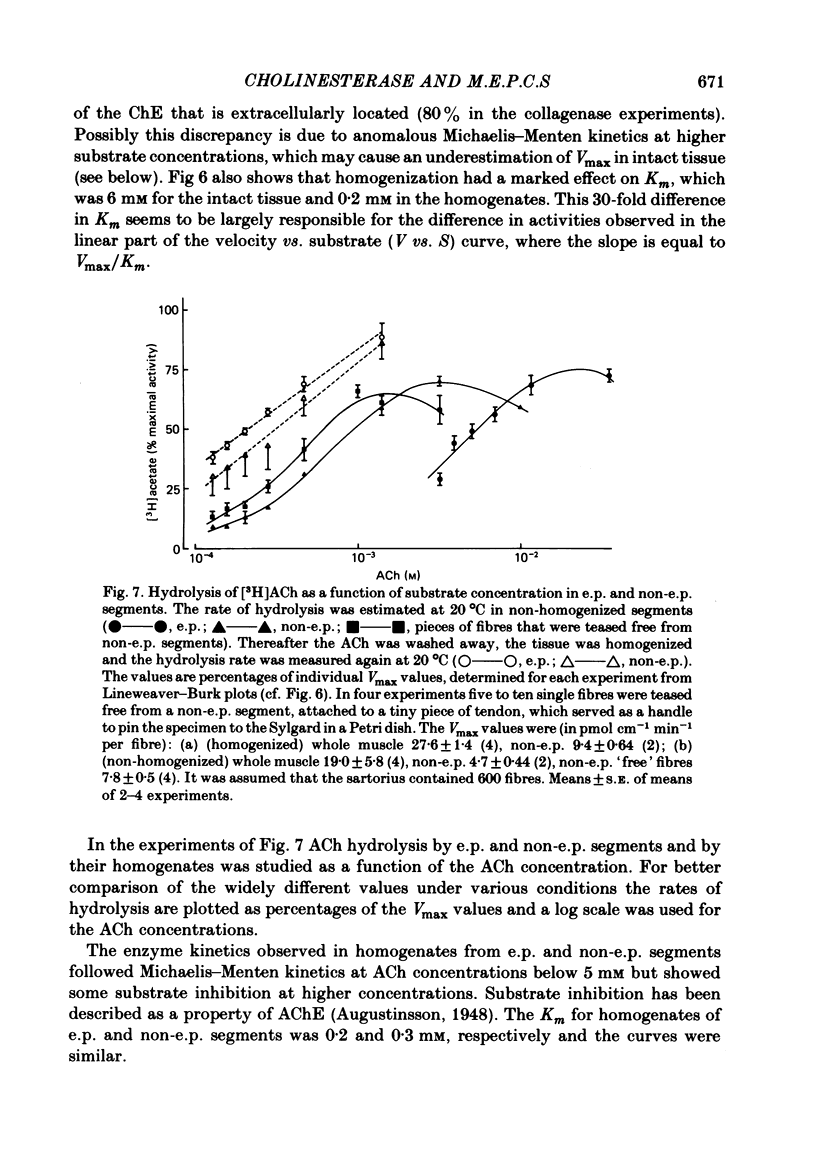
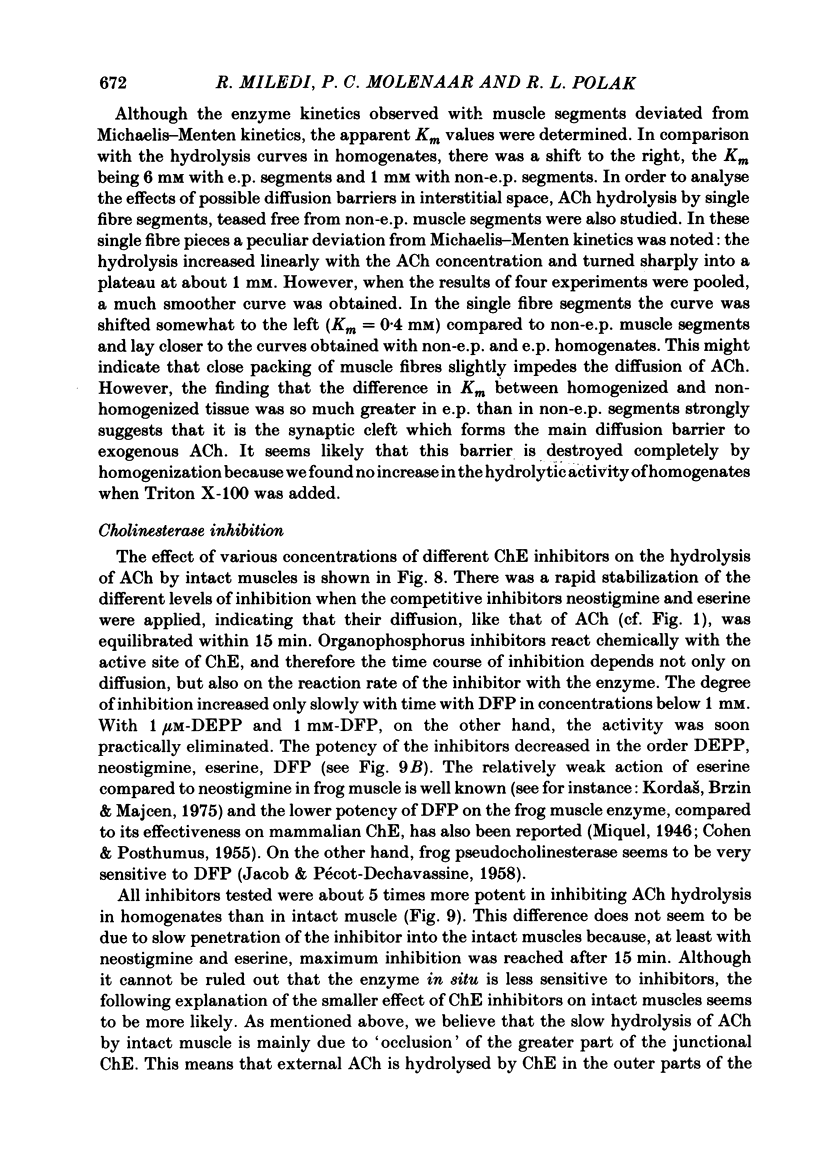
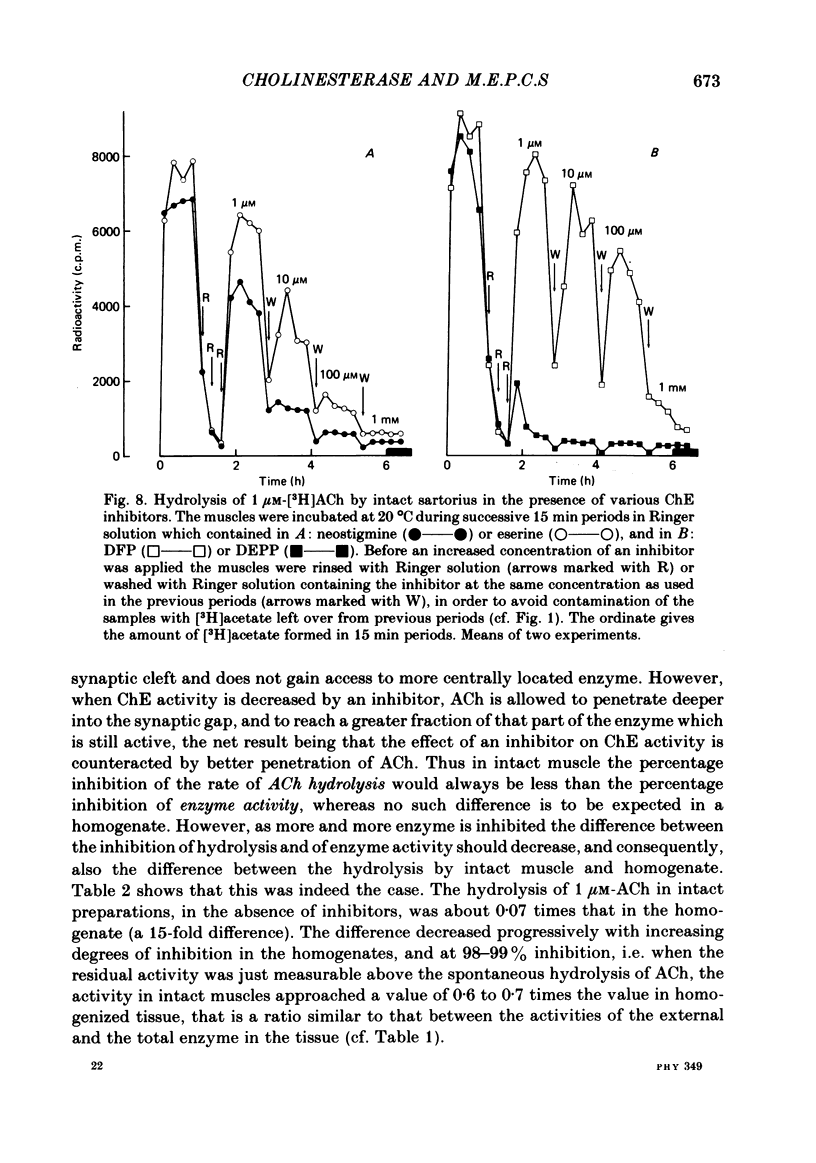
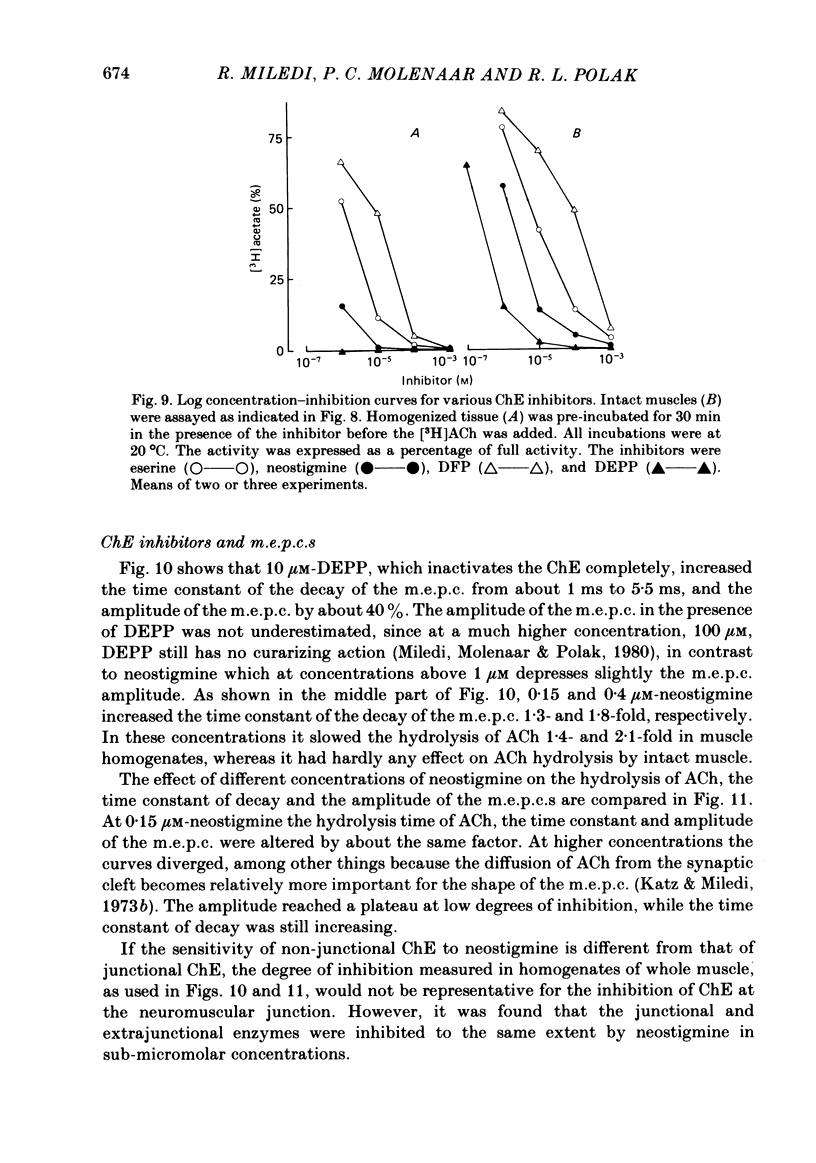
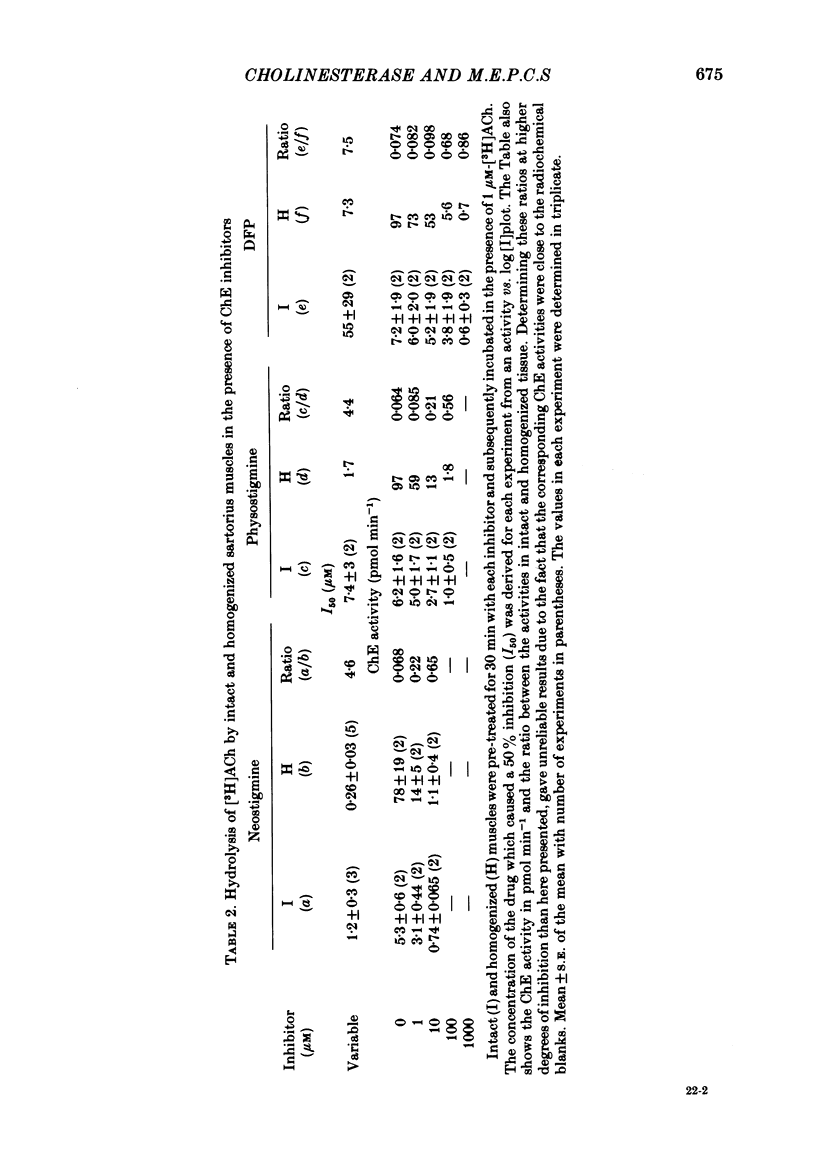
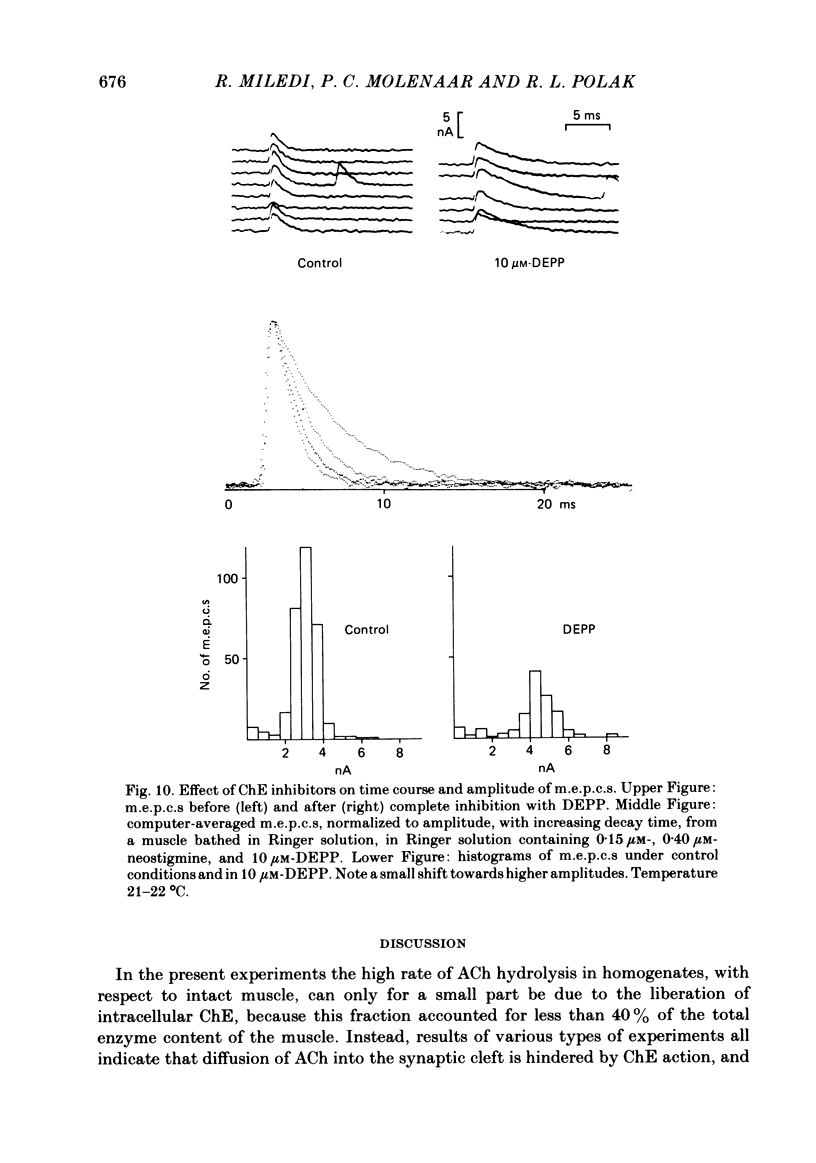
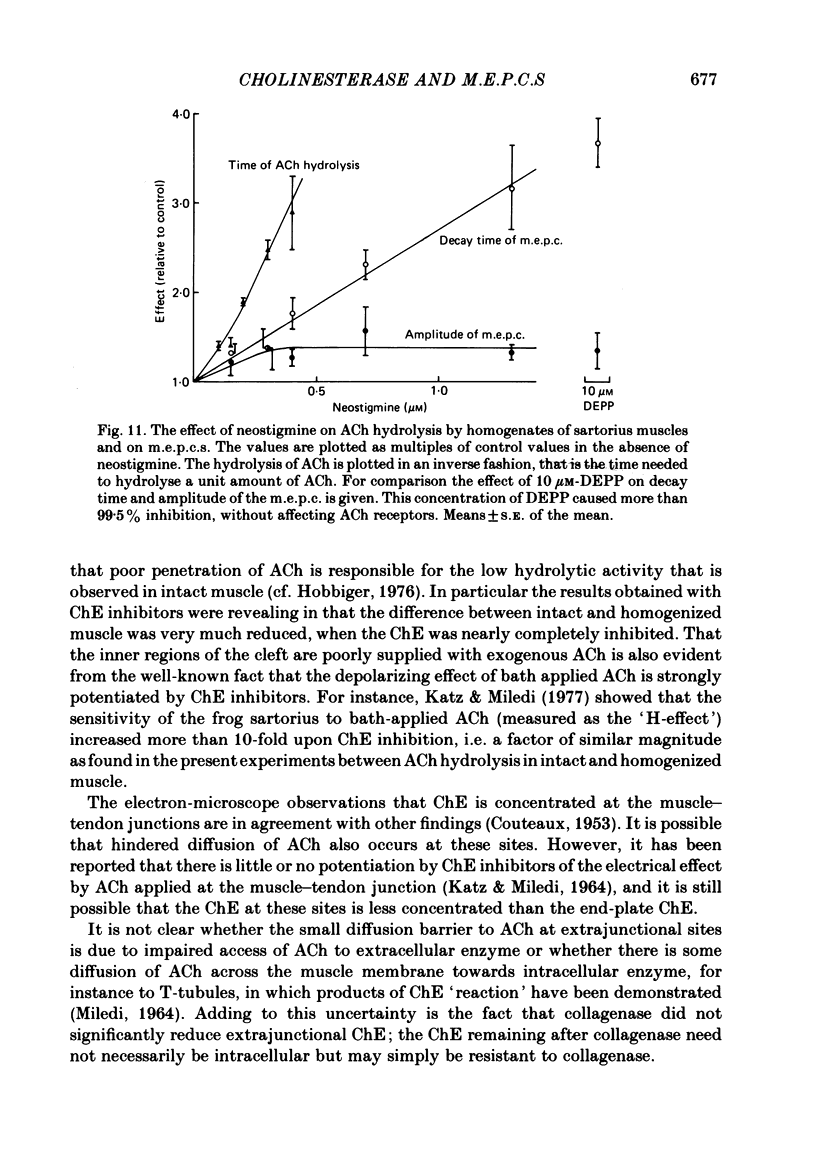
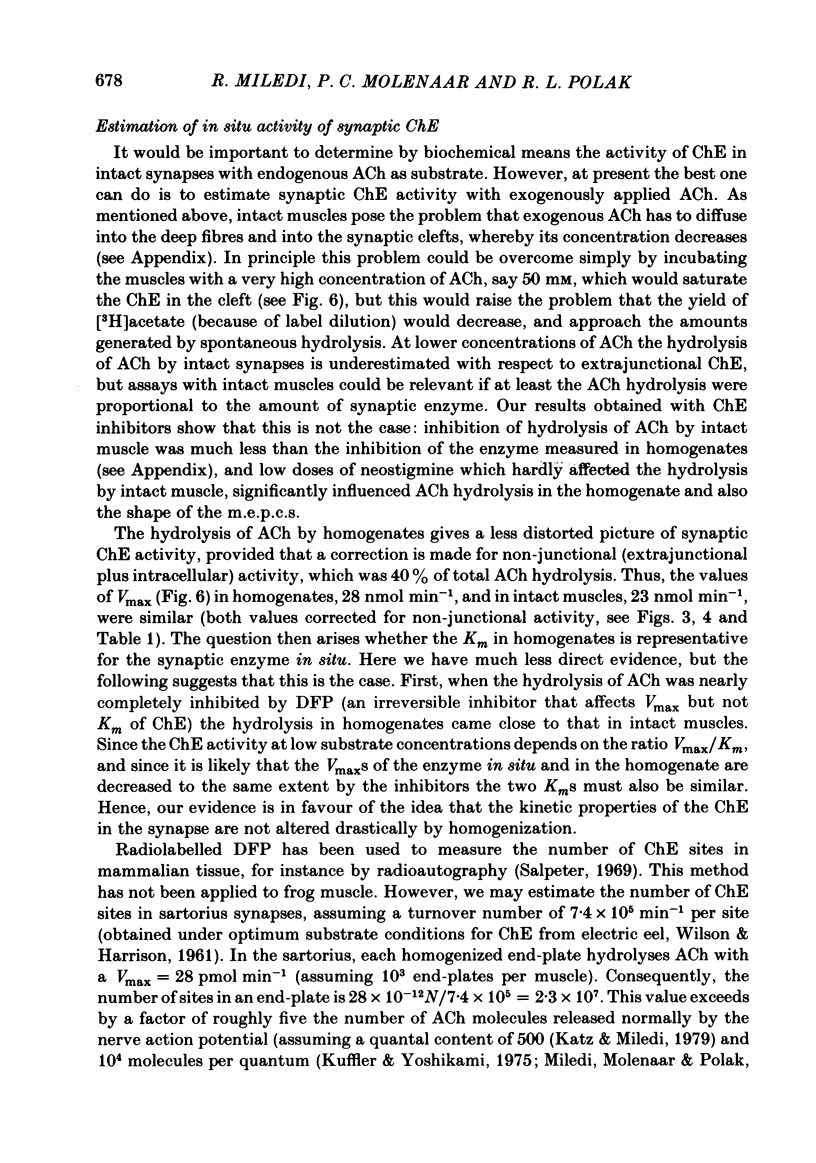
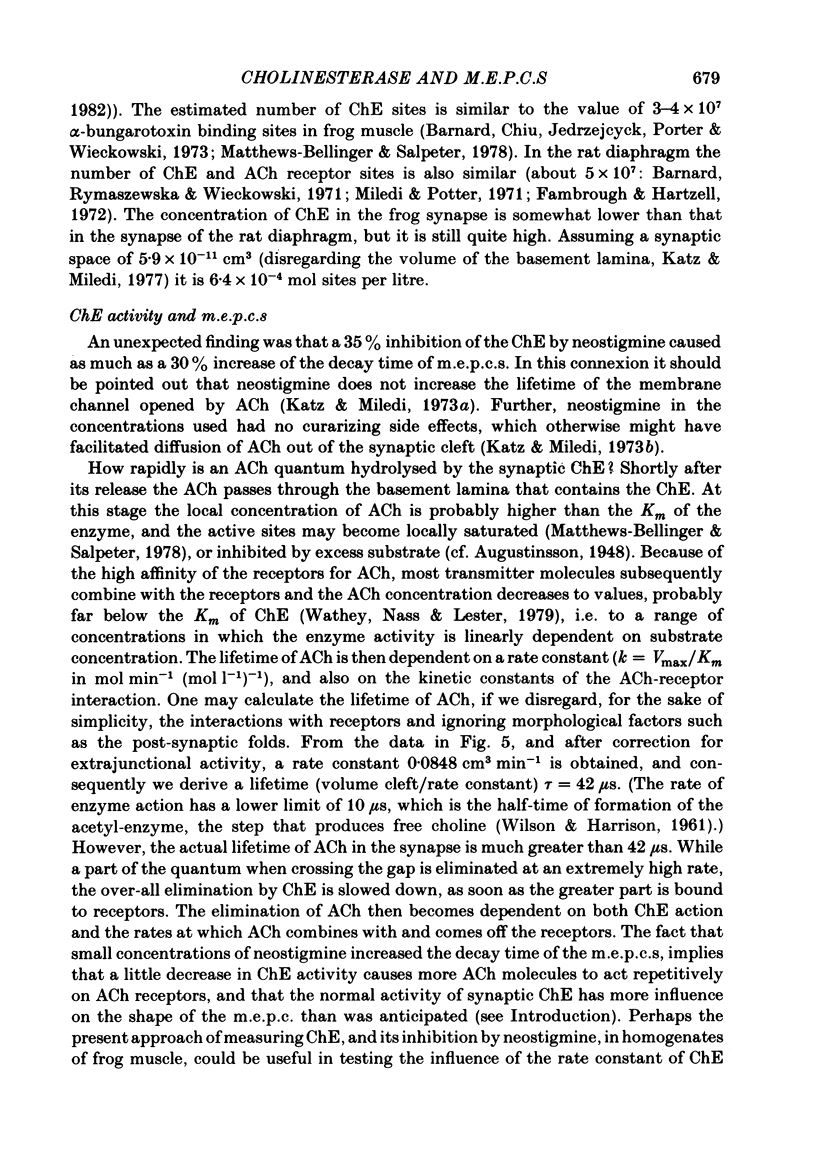
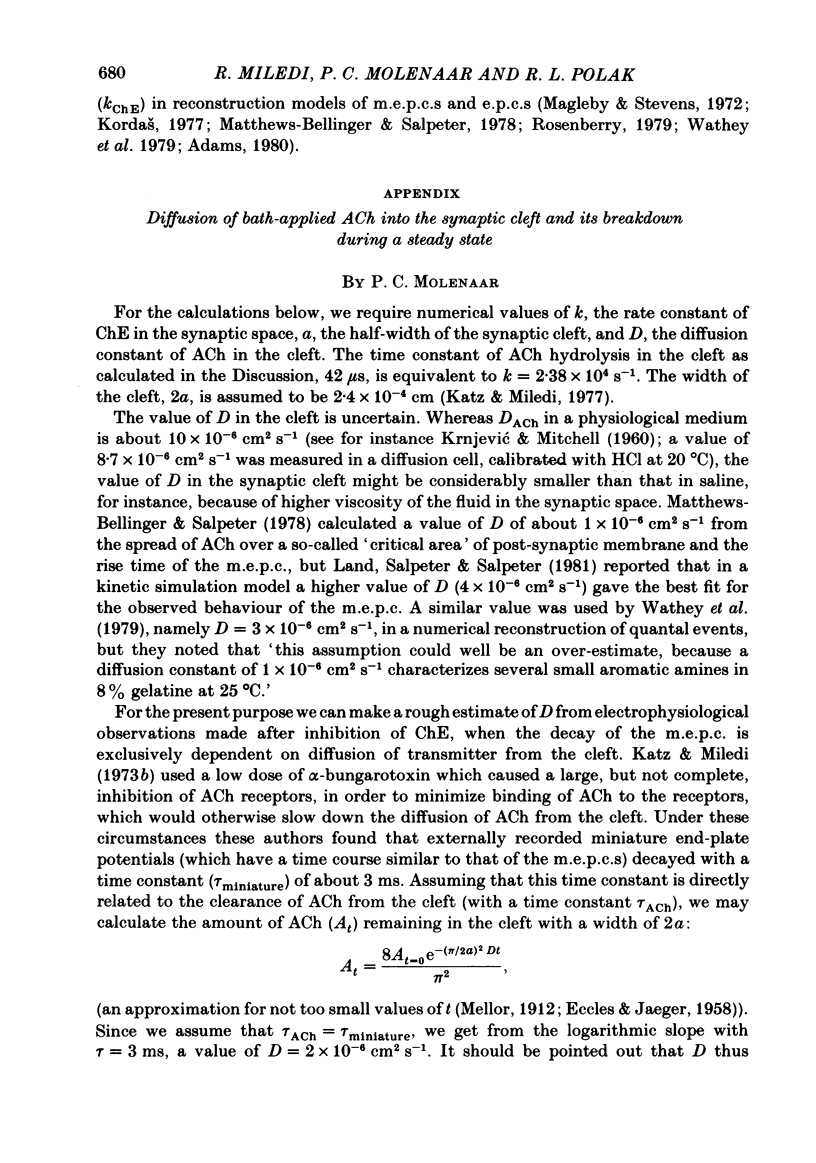
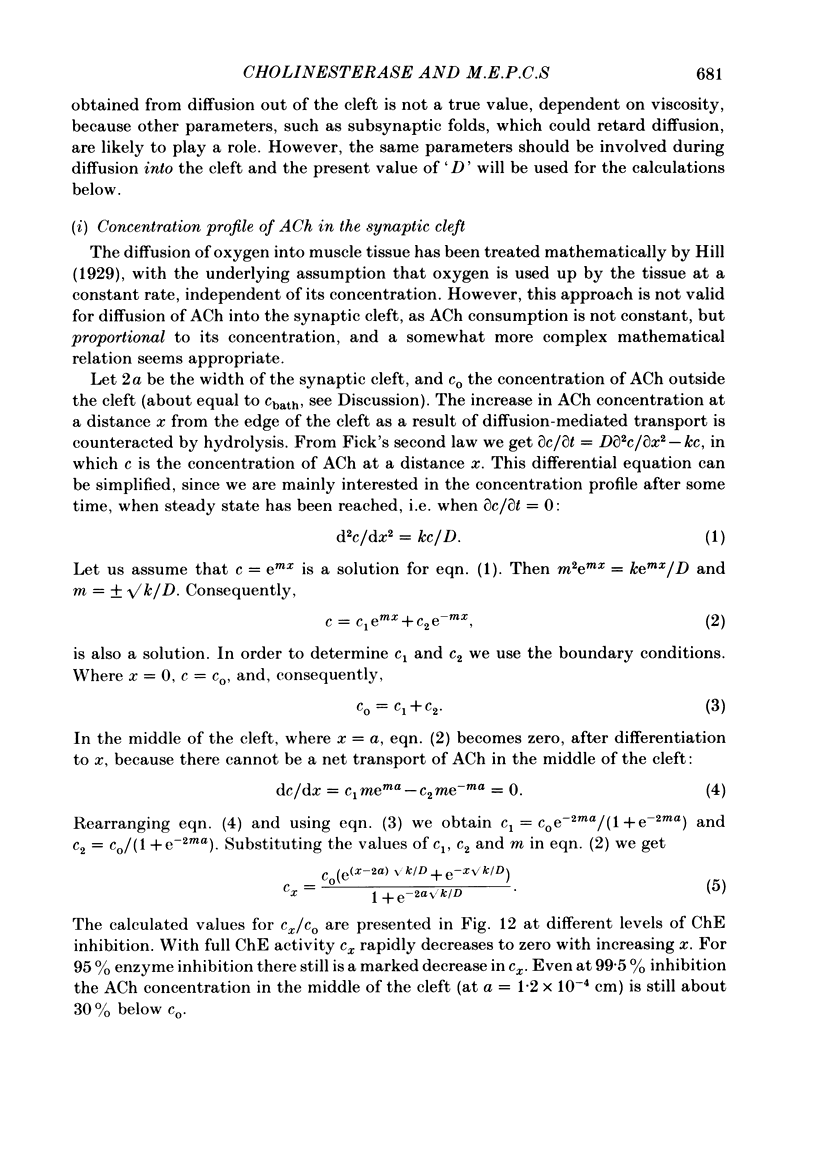
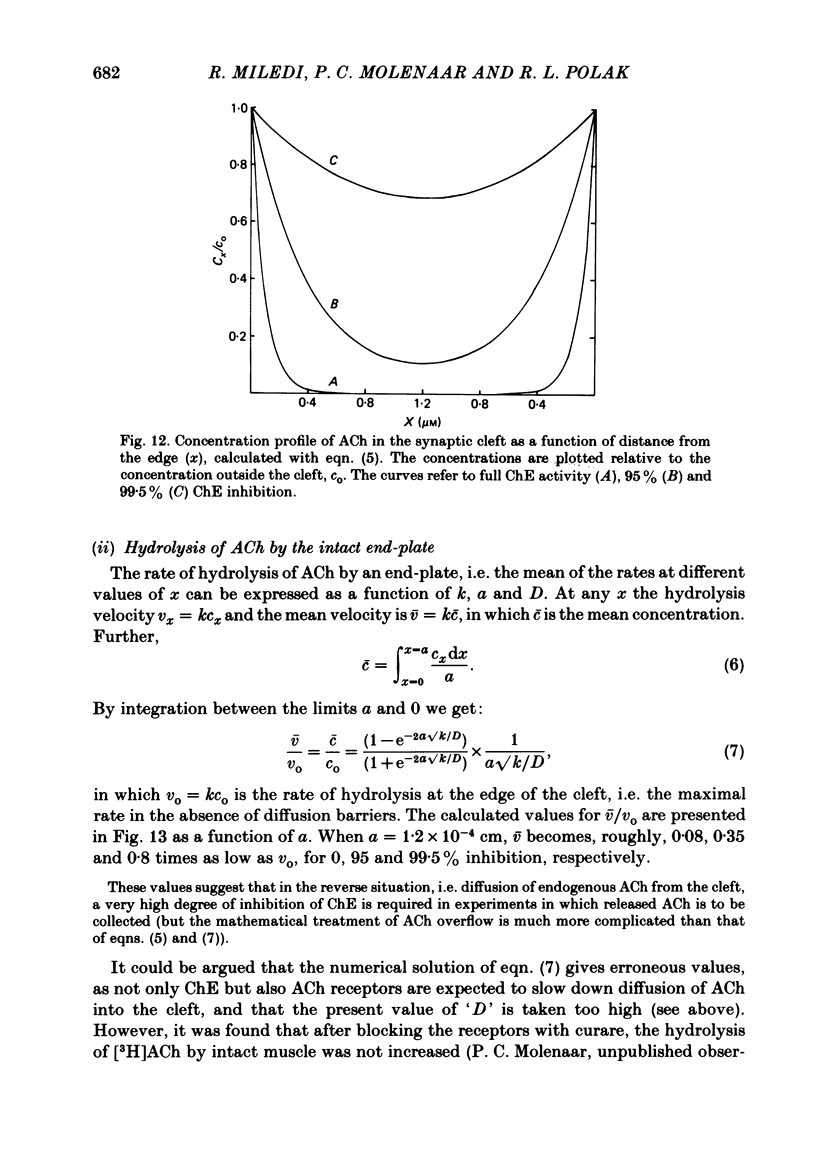
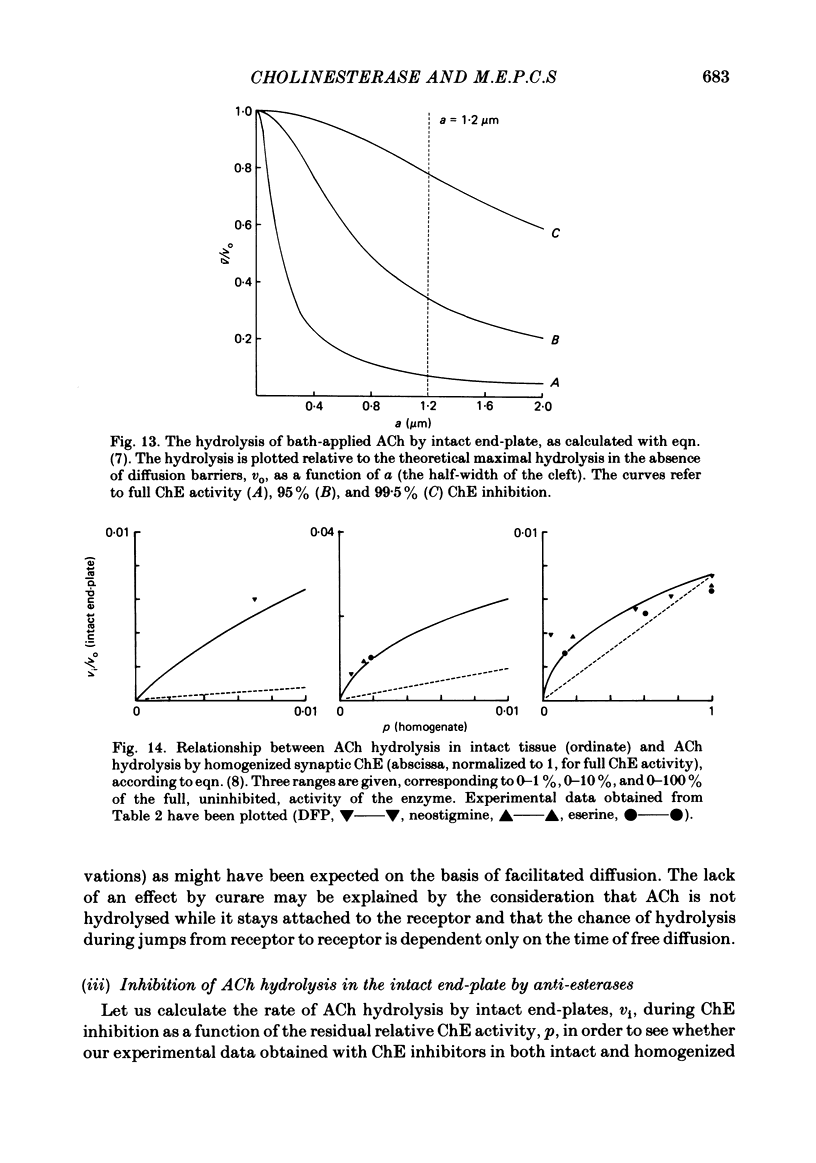
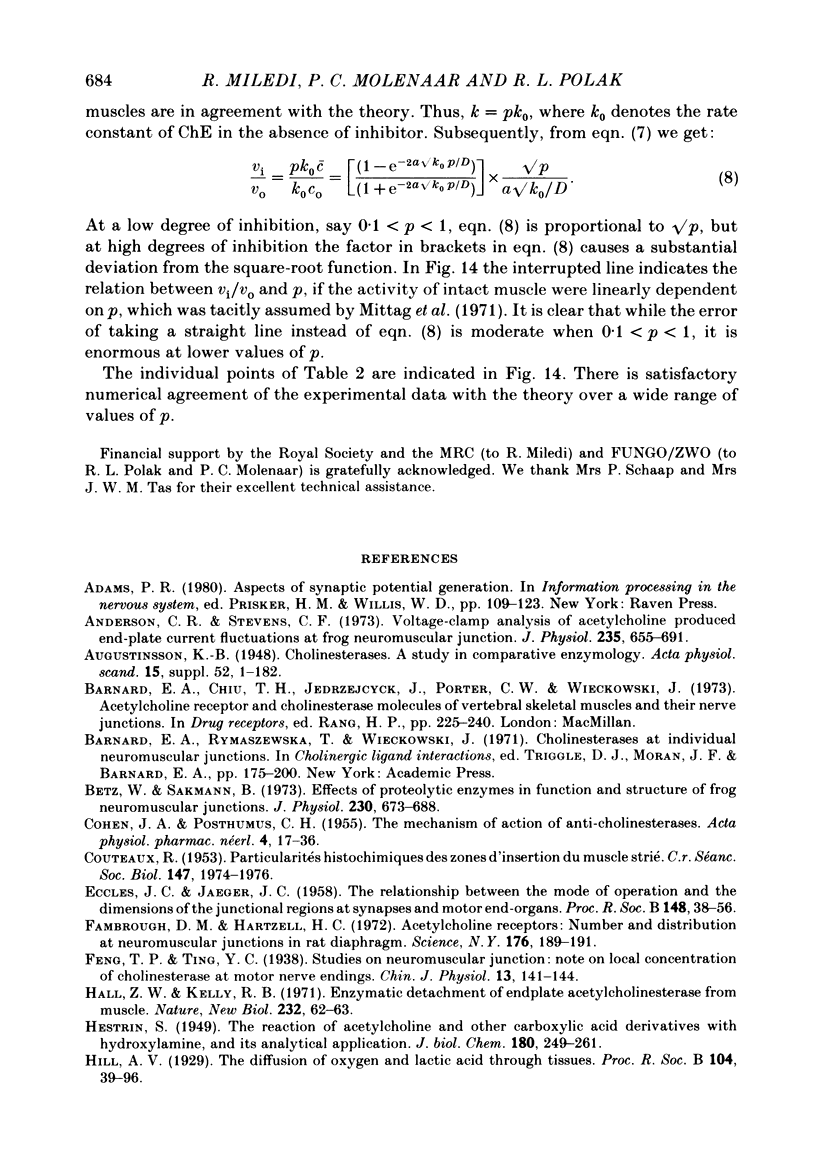
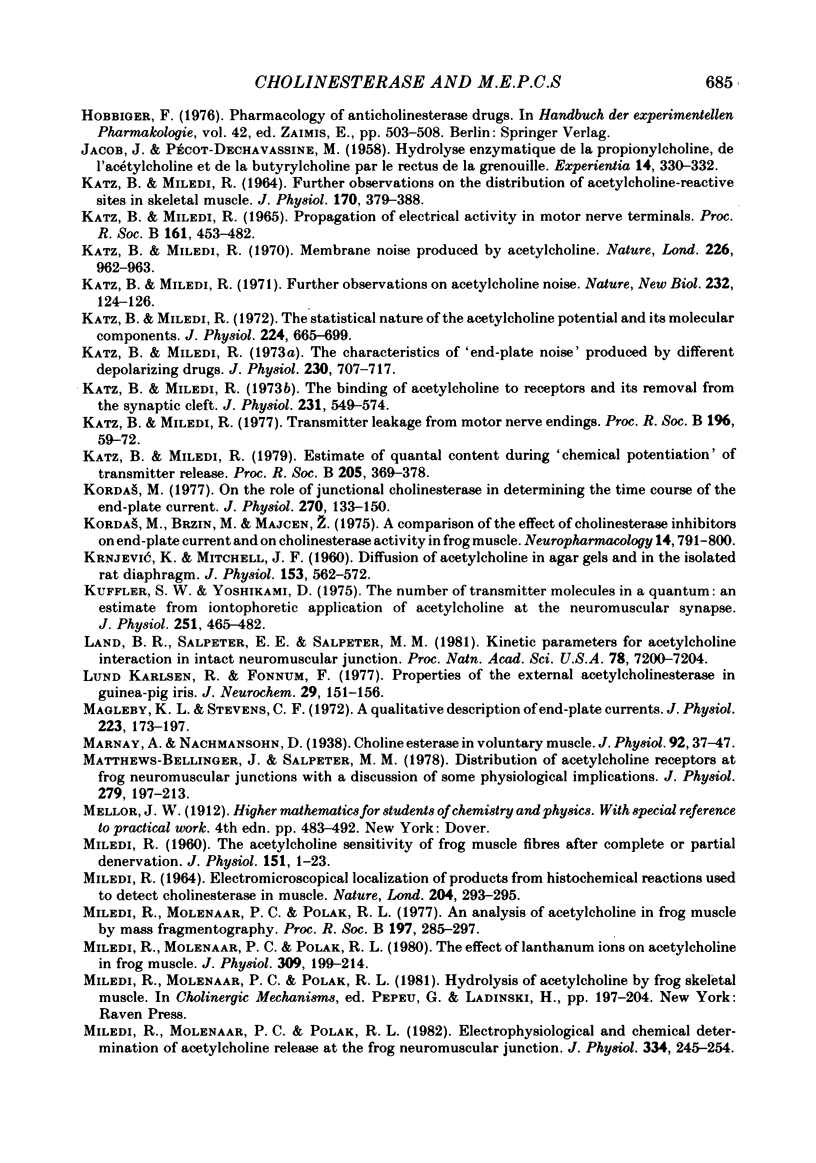
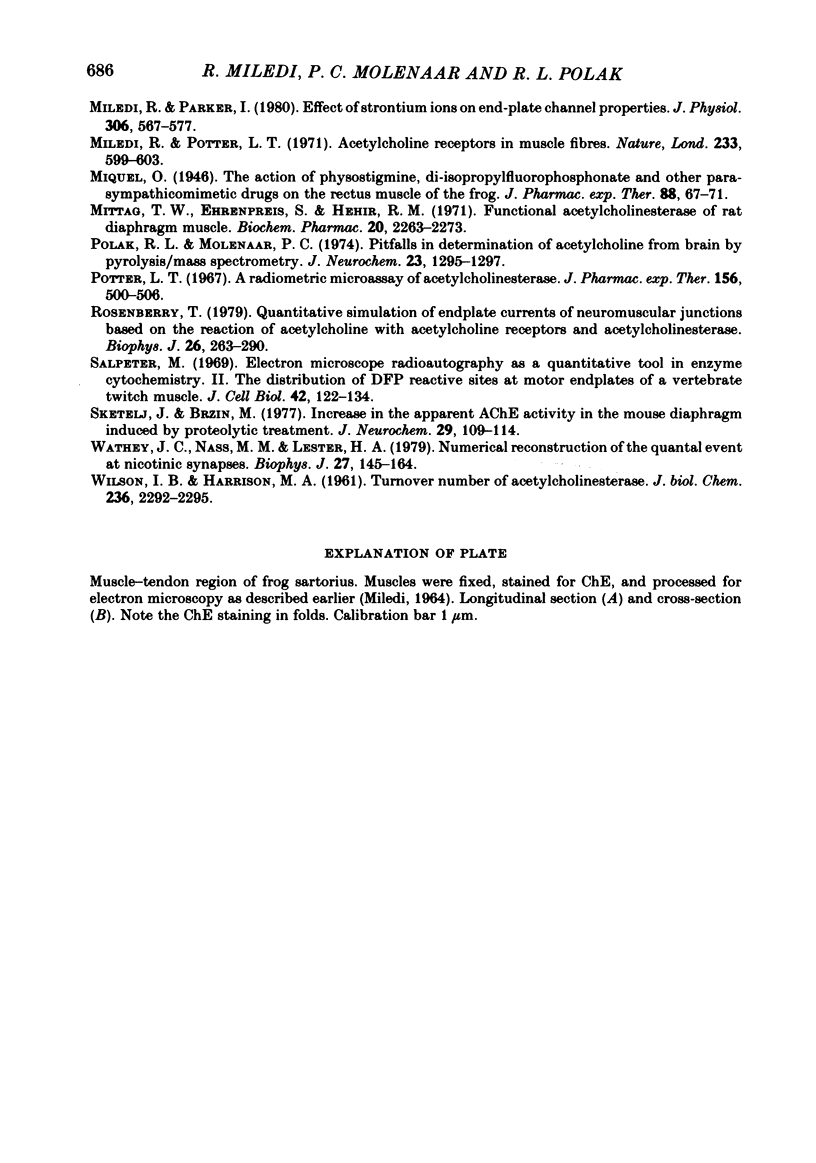
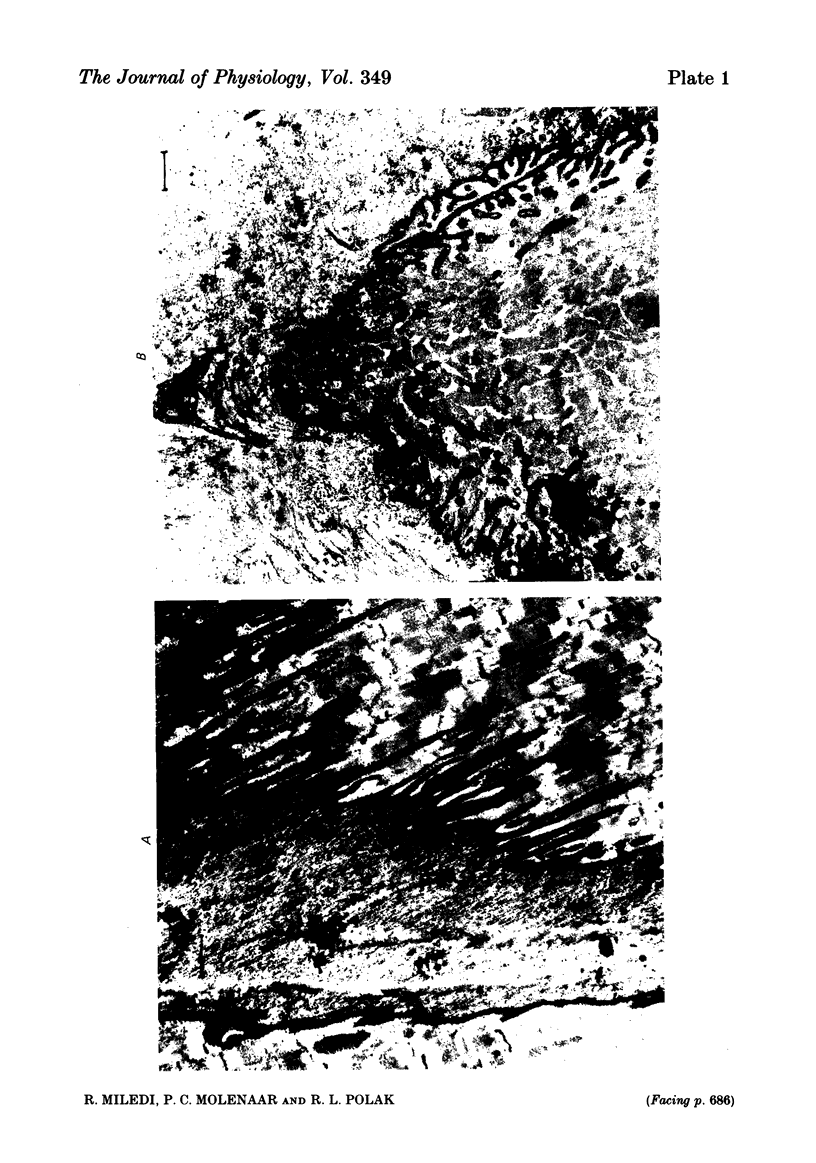
Images in this article
Selected References
These references are in PubMed. This may not be the complete list of references from this article.
- Anderson C. R., Stevens C. F. Voltage clamp analysis of acetylcholine produced end-plate current fluctuations at frog neuromuscular junction. J Physiol. 1973 Dec;235(3):655–691. doi: 10.1113/jphysiol.1973.sp010410. [DOI] [PMC free article] [PubMed] [Google Scholar]
- Betz W., Sakmann B. Effects of proteolytic enzymes on function and structure of frog neuromuscular junctions. J Physiol. 1973 May;230(3):673–688. doi: 10.1113/jphysiol.1973.sp010211. [DOI] [PMC free article] [PubMed] [Google Scholar]
- COHEN J. A., POSTHUMUS C. H. The mechanism of action of anti-cholinesterases. Acta Physiol Pharmacol Neerl. 1955;4(1):17–36. [PubMed] [Google Scholar]
- COUTEAUX R. Particularités histochimiques des zones d'insertion du muscle strié. C R Seances Soc Biol Fil. 1953 Dec;147(23-24):1974–1976. [PubMed] [Google Scholar]
- ECCLES J. C., JAEGER J. C. The relationship between the mode of operation and the dimensions of the junctional regions at synapses and motor end-organs. Proc R Soc Lond B Biol Sci. 1958 Jan 1;148(930):38–56. doi: 10.1098/rspb.1958.0003. [DOI] [PubMed] [Google Scholar]
- Fambrough D. M., Hartzell H. C. Acetylcholine receptors: number and distribution at neuromuscular junctions in rat diaphragm. Science. 1972 Apr 14;176(4031):189–191. doi: 10.1126/science.176.4031.189. [DOI] [PubMed] [Google Scholar]
- Hall Z. W., Kelly R. B. Enzymatic detachment of endplate acetylcholinesterase from muscle. Nat New Biol. 1971 Jul 14;232(28):62–63. doi: 10.1038/newbio232062a0. [DOI] [PubMed] [Google Scholar]
- JACOB J., PECOT-DECHAVASSINE M. Hydrolyse enzymatique de la propionylcholine, de l'acétylthiocholine et de la butyrylthiocholine par le rectus de grenouille. Experientia. 1958 Sep 15;14(9):330–332. doi: 10.1007/BF02160391. [DOI] [PubMed] [Google Scholar]
- KATZ B., MILEDI R. FURTHER OBSERVATIONS ON THE DISTRIBUTION OF ACTYLCHOLINE-REACTIVE SITES IN SKELETAL MUSCLE. J Physiol. 1964 Mar;170:379–388. doi: 10.1113/jphysiol.1964.sp007338. [DOI] [PMC free article] [PubMed] [Google Scholar]
- KATZ B., MILEDI R. PROPAGATION OF ELECTRIC ACTIVITY IN MOTOR NERVE TERMINALS. Proc R Soc Lond B Biol Sci. 1965 Feb 16;161:453–482. doi: 10.1098/rspb.1965.0015. [DOI] [PubMed] [Google Scholar]
- KRNJEVIC K., MITCHELL J. F. Diffusion of acetylcholine in agar gels and in the isolated rat diaphragm. J Physiol. 1960 Oct;153:562–572. doi: 10.1113/jphysiol.1960.sp006555. [DOI] [PMC free article] [PubMed] [Google Scholar]
- Karlsen R. L., Fonnum F. Properties of the external acetylcholinesterase in guinea-pig iris. J Neurochem. 1977 Jul;29(1):151–156. doi: 10.1111/j.1471-4159.1977.tb03937.x. [DOI] [PubMed] [Google Scholar]
- Katz B., Miledi R. Estimates of quantal content during 'chemical potentiation' of transmitter release. Proc R Soc Lond B Biol Sci. 1979 Aug 31;205(1160):369–378. doi: 10.1098/rspb.1979.0070. [DOI] [PubMed] [Google Scholar]
- Katz B., Miledi R. Further observations on acetylcholine noise. Nat New Biol. 1971 Jul 28;232(30):124–126. doi: 10.1038/newbio232124b0. [DOI] [PubMed] [Google Scholar]
- Katz B., Miledi R. Membrane noise produced by acetylcholine. Nature. 1970 Jun 6;226(5249):962–963. doi: 10.1038/226962a0. [DOI] [PubMed] [Google Scholar]
- Katz B., Miledi R. The binding of acetylcholine to receptors and its removal from the synaptic cleft. J Physiol. 1973 Jun;231(3):549–574. doi: 10.1113/jphysiol.1973.sp010248. [DOI] [PMC free article] [PubMed] [Google Scholar]
- Katz B., Miledi R. The characteristics of 'end-plate noise' produced by different depolarizing drugs. J Physiol. 1973 May;230(3):707–717. doi: 10.1113/jphysiol.1973.sp010213. [DOI] [PMC free article] [PubMed] [Google Scholar]
- Katz B., Miledi R. The statistical nature of the acetycholine potential and its molecular components. J Physiol. 1972 Aug;224(3):665–699. doi: 10.1113/jphysiol.1972.sp009918. [DOI] [PMC free article] [PubMed] [Google Scholar]
- Katz B., Miledi R. Transmitter leakage from motor nerve endings. Proc R Soc Lond B Biol Sci. 1977 Feb 11;196(1122):59–72. doi: 10.1098/rspb.1977.0029. [DOI] [PubMed] [Google Scholar]
- Kordás M., Brzin M., Majcen Z. A comparison of the effect of cholinesterase inhibitors on end-plate current and on cholinesterase activity in frog muscle. Neuropharmacology. 1975 Nov;14(11):791–800. doi: 10.1016/0028-3908(75)90106-9. [DOI] [PubMed] [Google Scholar]
- Kuffler S. W., Yoshikami D. The number of transmitter molecules in a quantum: an estimate from iontophoretic application of acetylcholine at the neuromuscular synapse. J Physiol. 1975 Oct;251(2):465–482. doi: 10.1113/jphysiol.1975.sp011103. [DOI] [PMC free article] [PubMed] [Google Scholar]
- Land B. R., Salpeter E. E., Salpeter M. M. Kinetic parameters for acetylcholine interaction in intact neuromuscular junction. Proc Natl Acad Sci U S A. 1981 Nov;78(11):7200–7204. doi: 10.1073/pnas.78.11.7200. [DOI] [PMC free article] [PubMed] [Google Scholar]
- MILEDI R. ELECTRON-MICROSCOPICAL LOCALIZATION OF PRODUCTS FROM HISTOCHEMICAL REACTIONS USED TO DETECT CHOLINESTERASE IN MUSCLE. Nature. 1964 Oct 17;204:293–295. doi: 10.1038/204293b0. [DOI] [PubMed] [Google Scholar]
- MILEDI R. The acetylcholine sensitivity of frog muscle fibres after complete or partial devervation. J Physiol. 1960 Apr;151:1–23. [PMC free article] [PubMed] [Google Scholar]
- Magleby K. L., Stevens C. F. A quantitative description of end-plate currents. J Physiol. 1972 May;223(1):173–197. doi: 10.1113/jphysiol.1972.sp009840. [DOI] [PMC free article] [PubMed] [Google Scholar]
- Matthews-Bellinger J., Salpeter M. M. Distribution of acetylcholine receptors at frog neuromuscular junctions with a discussion of some physiological implications. J Physiol. 1978 Jun;279:197–213. doi: 10.1113/jphysiol.1978.sp012340. [DOI] [PMC free article] [PubMed] [Google Scholar]
- Miledi R., Molenaar P. C., Polak R. L. An analysis of acetylcholine in frog muscle by mass fragmentography. Proc R Soc Lond B Biol Sci. 1977 Jun 15;197(1128):285–297. doi: 10.1098/rspb.1977.0071. [DOI] [PubMed] [Google Scholar]
- Miledi R., Molenaar P. C., Polak R. L. Electrophysiological and chemical determination of acetylcholine release at the frog neuromuscular junction. J Physiol. 1983 Jan;334:245–254. doi: 10.1113/jphysiol.1983.sp014492. [DOI] [PMC free article] [PubMed] [Google Scholar]
- Miledi R., Molenaar P. C., Polak R. L. The effect of lanthanum ions on acetylcholine in frog muscle. J Physiol. 1980 Dec;309:199–214. doi: 10.1113/jphysiol.1980.sp013504. [DOI] [PMC free article] [PubMed] [Google Scholar]
- Miledi R., Parker I. Effects of strontium ions on end-plate channel properties. J Physiol. 1980 Sep;306:567–577. doi: 10.1113/jphysiol.1980.sp013415. [DOI] [PMC free article] [PubMed] [Google Scholar]
- Miledi R., Potter L. T. Acetylcholine receptors in muscle fibres. Nature. 1971 Oct 29;233(5322):599–603. doi: 10.1038/233599a0. [DOI] [PubMed] [Google Scholar]
- Mittag T. W., Ehrenpreis S., Hehir R. M. Functional acetylcholinesterase of rat diaphragm muscle. Biochem Pharmacol. 1971 Sep;20(9):2263–2273. doi: 10.1016/0006-2952(71)90226-7. [DOI] [PubMed] [Google Scholar]
- PLATE W. P. Wigvormige resectie van de ovaria. Ned Tijdschr Geneeskd. 1956 Jul 14;100(28):1958–1960. [PubMed] [Google Scholar]
- Polak R. L., Molenaar P. C. Pitfalls in determination of acetylcholine from brain by pyrolysis-gas chromatography/mass spectrometry. J Neurochem. 1974 Dec;23(6):1295–1297. doi: 10.1111/j.1471-4159.1974.tb12230.x. [DOI] [PubMed] [Google Scholar]
- Potter L. T. A radiometric microassay of acetylcholinesterase. J Pharmacol Exp Ther. 1967 Jun;156(3):500–506. [PubMed] [Google Scholar]
- Rosenberry T. L. Quantitative simulation of endplate currents at neuromuscular junctions based on the reaction of acetylcholine with acetylcholine receptor and acetylcholinesterase. Biophys J. 1979 May;26(2):263–289. doi: 10.1016/S0006-3495(79)85249-2. [DOI] [PMC free article] [PubMed] [Google Scholar]
- Salpeter M. M. Electron microscope radioautography as a quantitative tool in enzyme cytochemistry. II. The distribution of DFP-reactive sties at motor endplates of a vertebrate twitch muscle. J Cell Biol. 1969 Jul;42(1):122–134. doi: 10.1083/jcb.42.1.122. [DOI] [PMC free article] [PubMed] [Google Scholar]
- Sketelj J., Brzin M. Increase in the apparent AChE activity in the mouse diaphragm induced by proteolytic treatment. J Neurochem. 1977 Jul;29(1):109–114. doi: 10.1111/j.1471-4159.1977.tb03931.x. [DOI] [PubMed] [Google Scholar]
- WILSON I. B., HARRISON M. A. Turnover number of acetyl-cholinesterase. J Biol Chem. 1961 Aug;236:2292–2295. [PubMed] [Google Scholar]
- Wathey J. C., Nass M. M., Lester H. A. Numerical reconstruction of the quantal event at nicotinic synapses. Biophys J. 1979 Jul;27(1):145–164. doi: 10.1016/S0006-3495(79)85208-X. [DOI] [PMC free article] [PubMed] [Google Scholar]