Abstract
The influence of a gramicidin-like channel former on ion free energy barriers is studied using Monte Carlo simulation. The model explicitly describes the ion, the water dipoles, and the peptide carbonyls; the remaining degrees of freedom, bulk electrolyte, non-polar lipid and peptide regions, and electronic (high frequency) permittivity, are treated in continuum terms. Contributions of the channel waters and peptide COs are studied both separately and collectively. We found that if constrained to their original orientations, the COs substantially increase the cationic permeation free energy; with or without water present, CO reorientation is crucial for ion-CO interaction to lower cation free energy barriers; the translocation free energy profiles for potassium-, rubidium-, and cesium-like cations exhibit no broad barriers; the lipid-bound peptide interacts more effectively with anions than cations; anionic translocation free energy profiles exhibit well defined maxima. Using experimental data to estimate transfer free energies of ions and water from bulk electrolyte to a non-polar dielectric (continuum lipid), we found reasonable ion permeation profiles; cations bind and permeate, whereas anions cannot enter the channel. Cation selectivity arises because, for ions of the same size and charge, anions bind hydration water more strongly.
Full text
PDF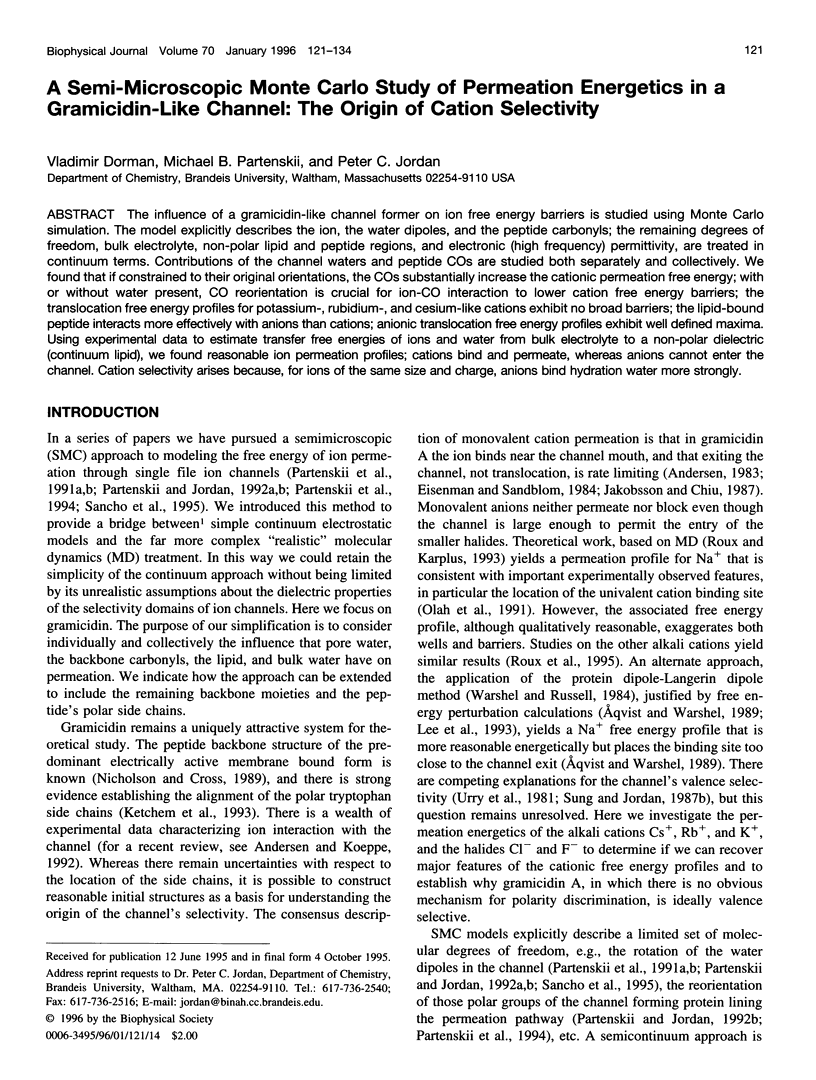
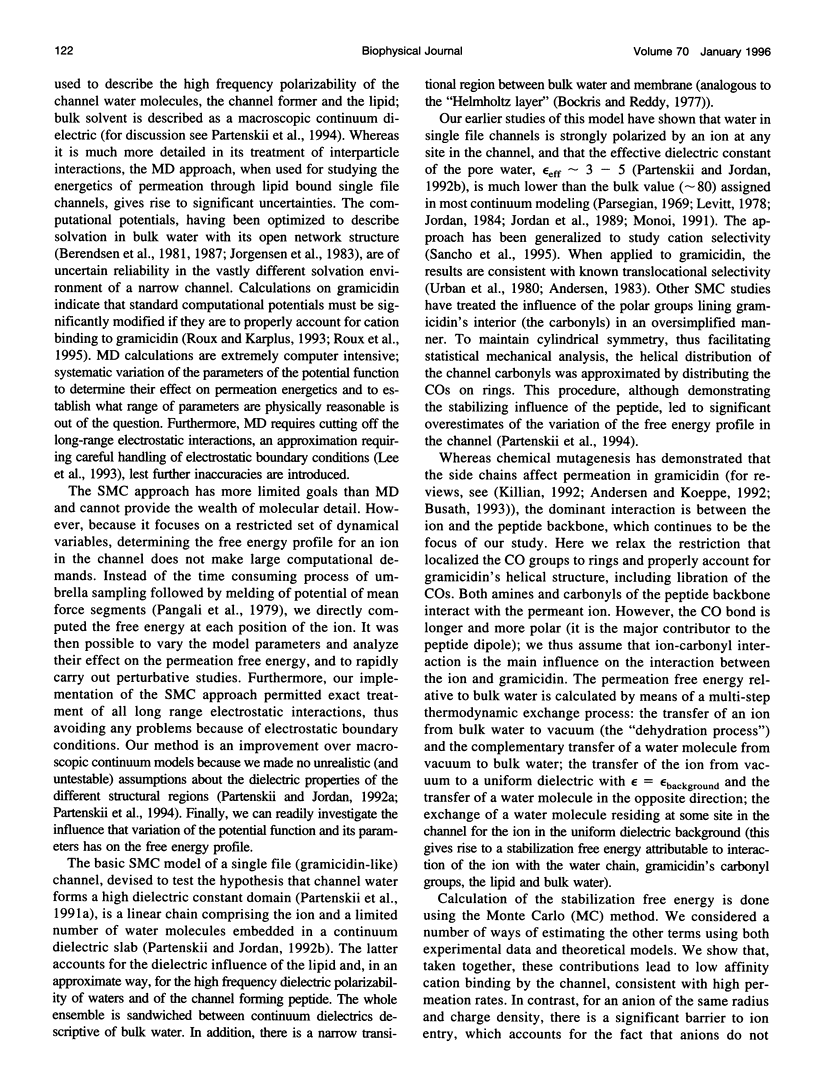
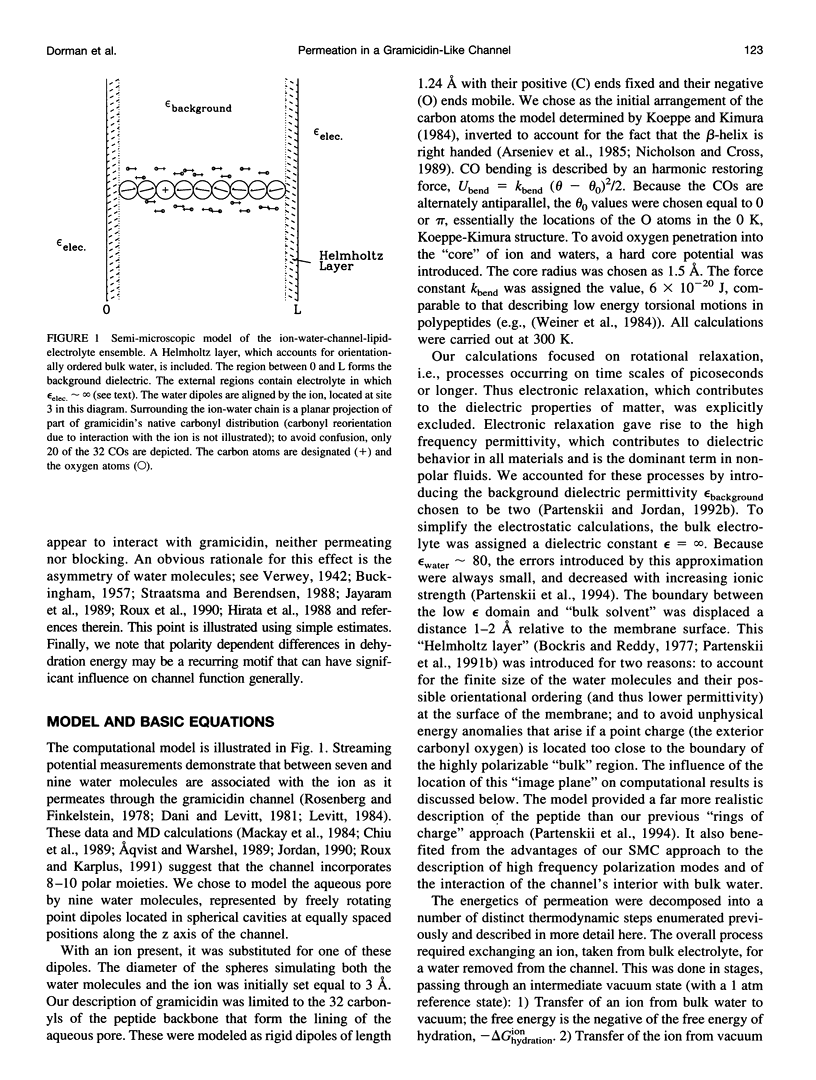
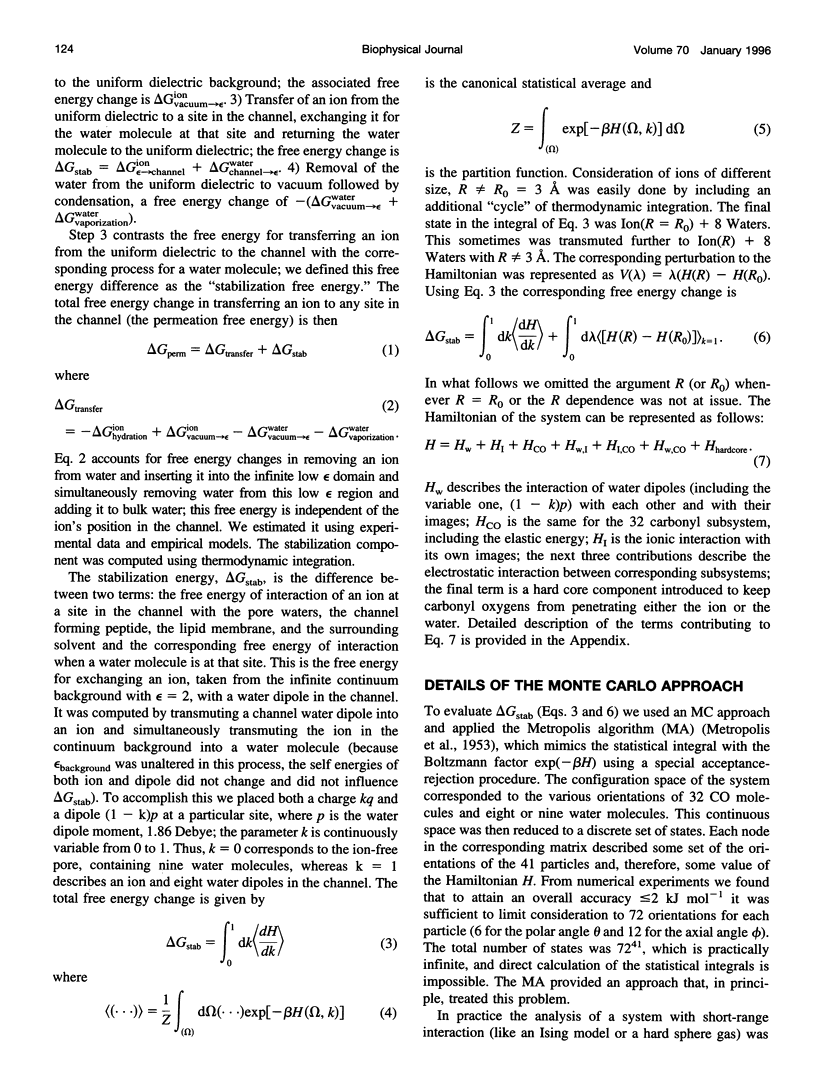
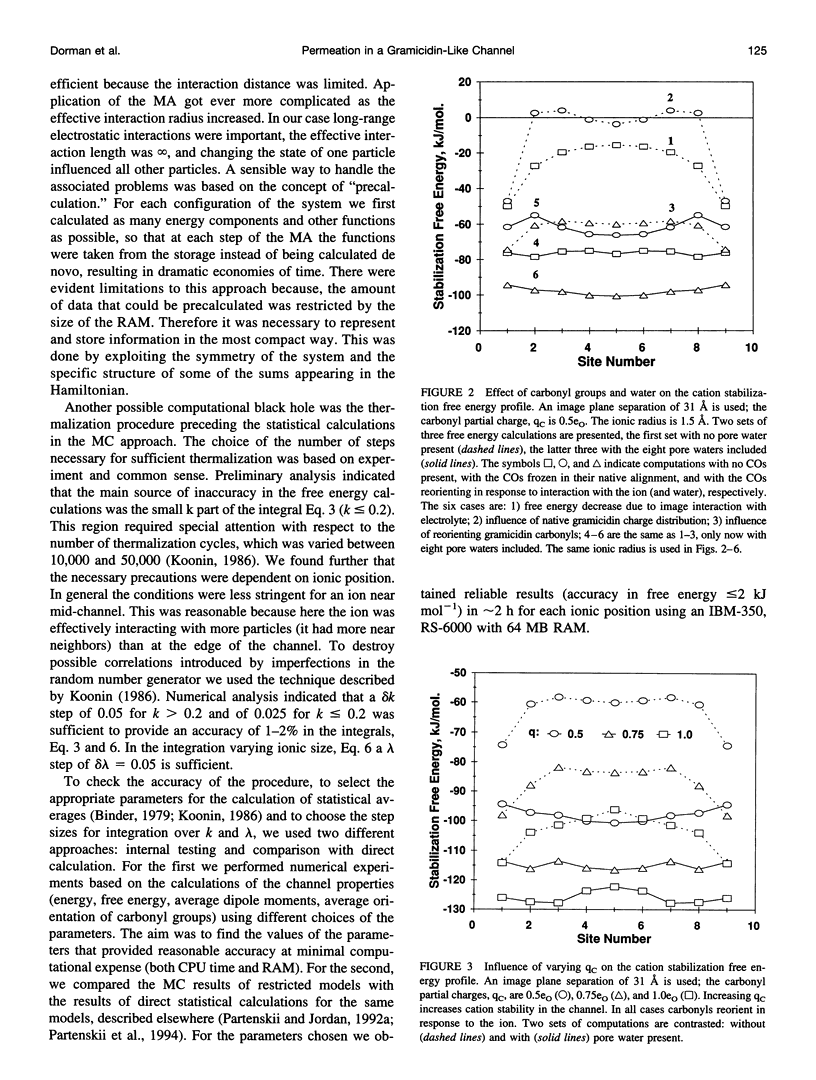
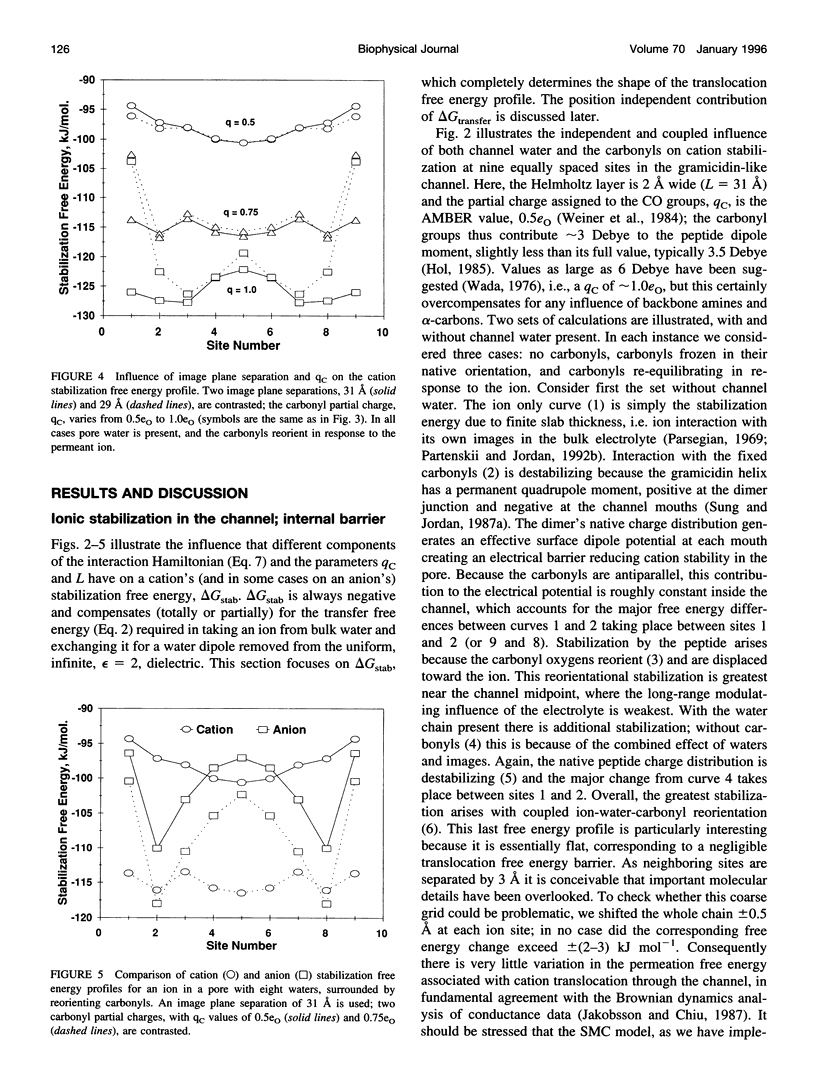
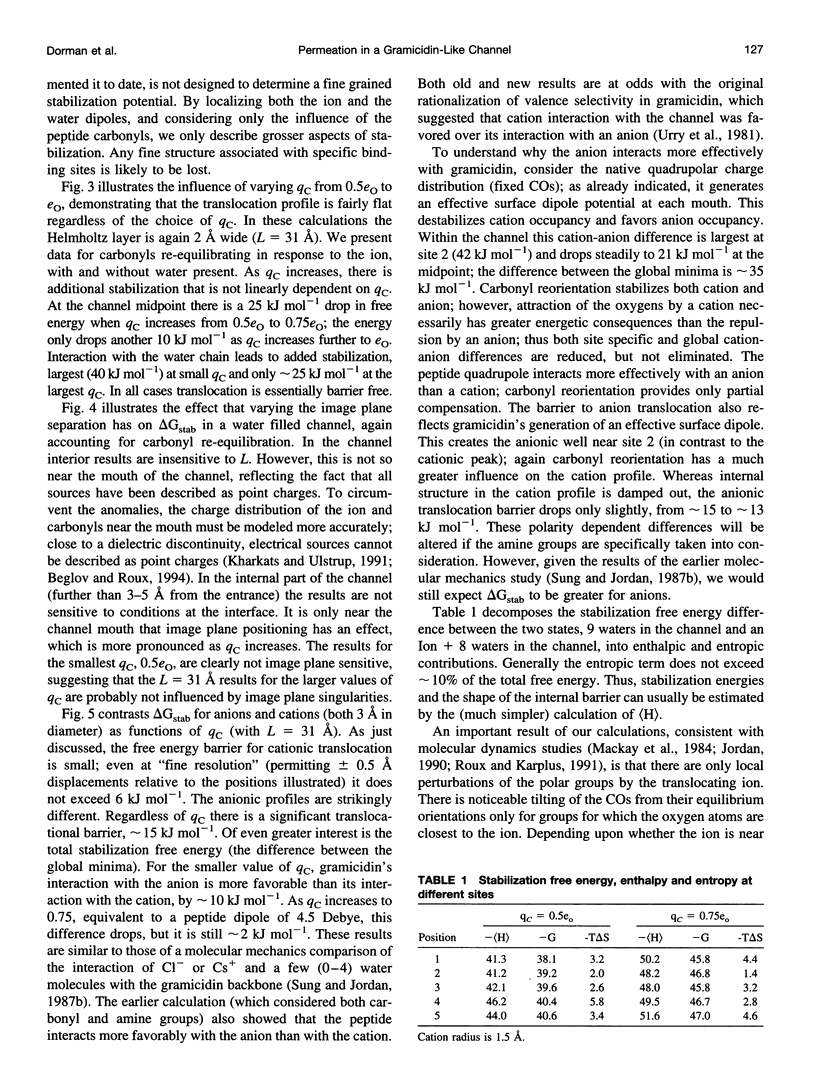
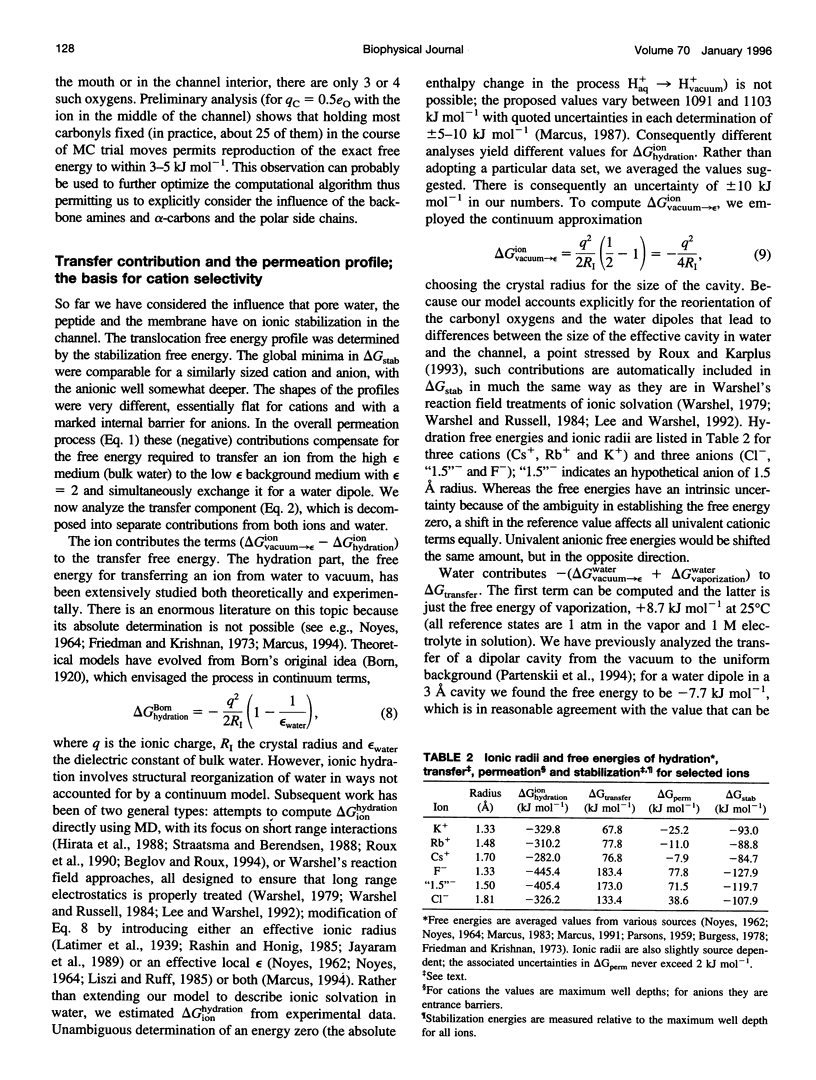
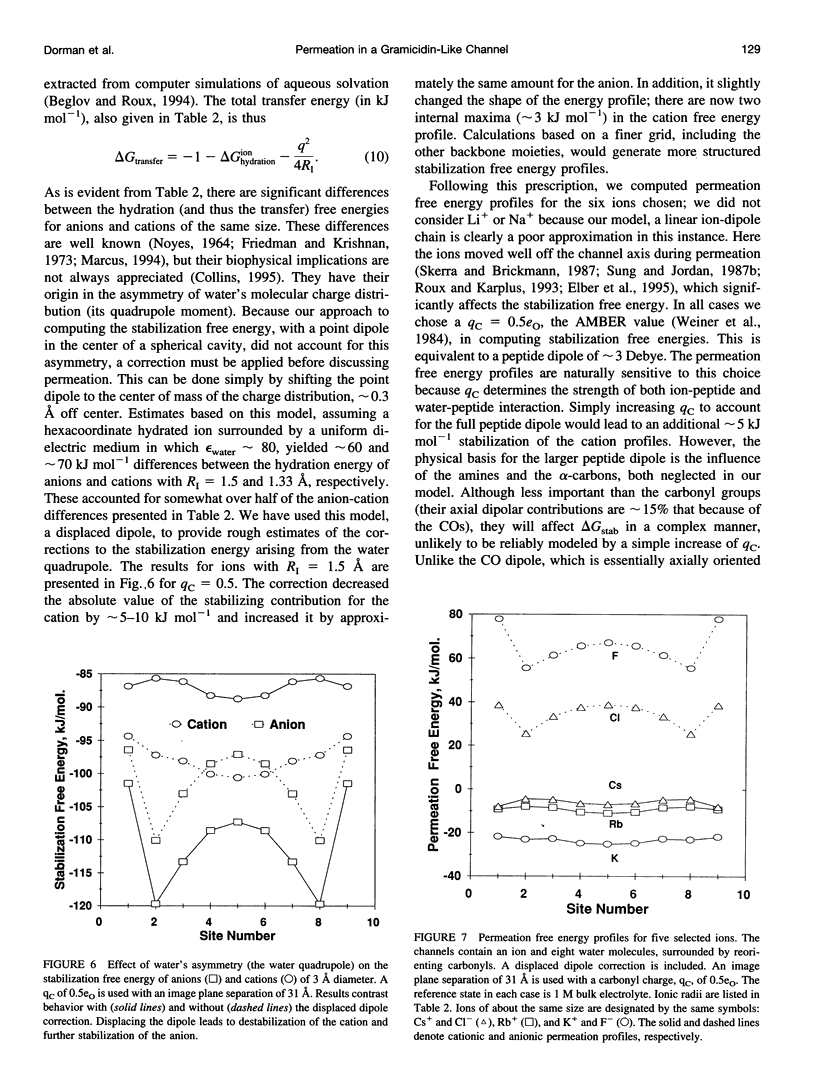
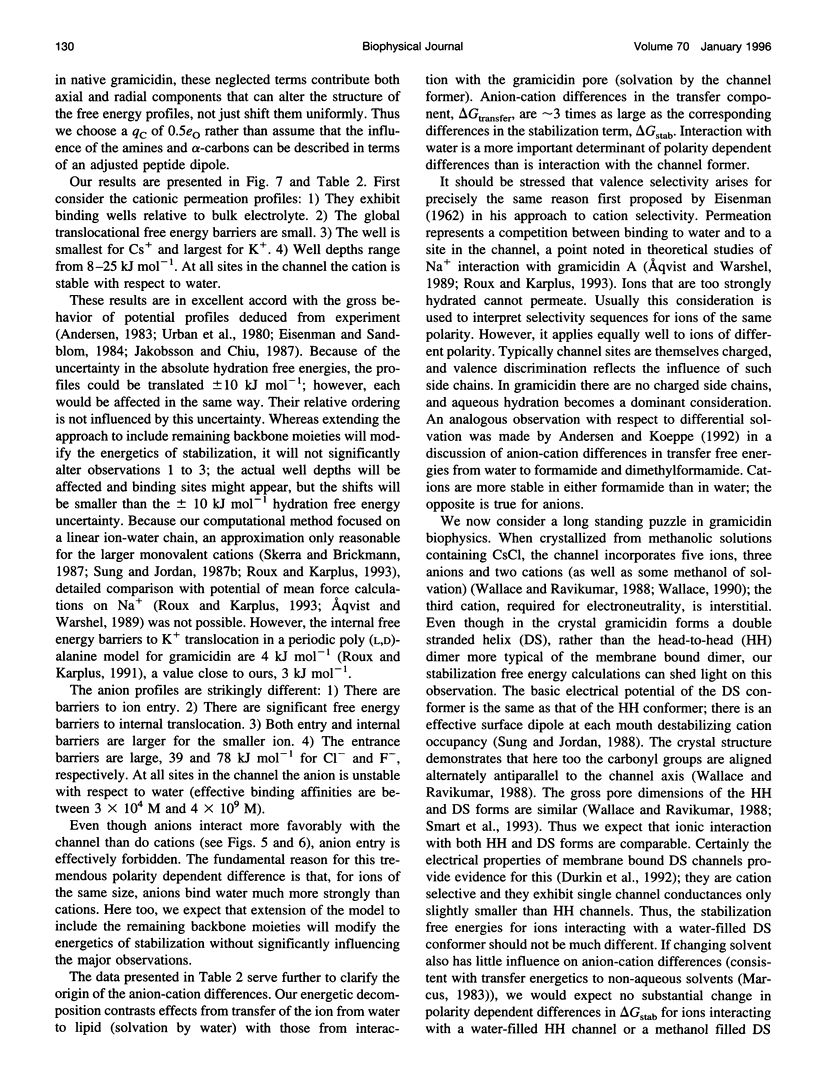
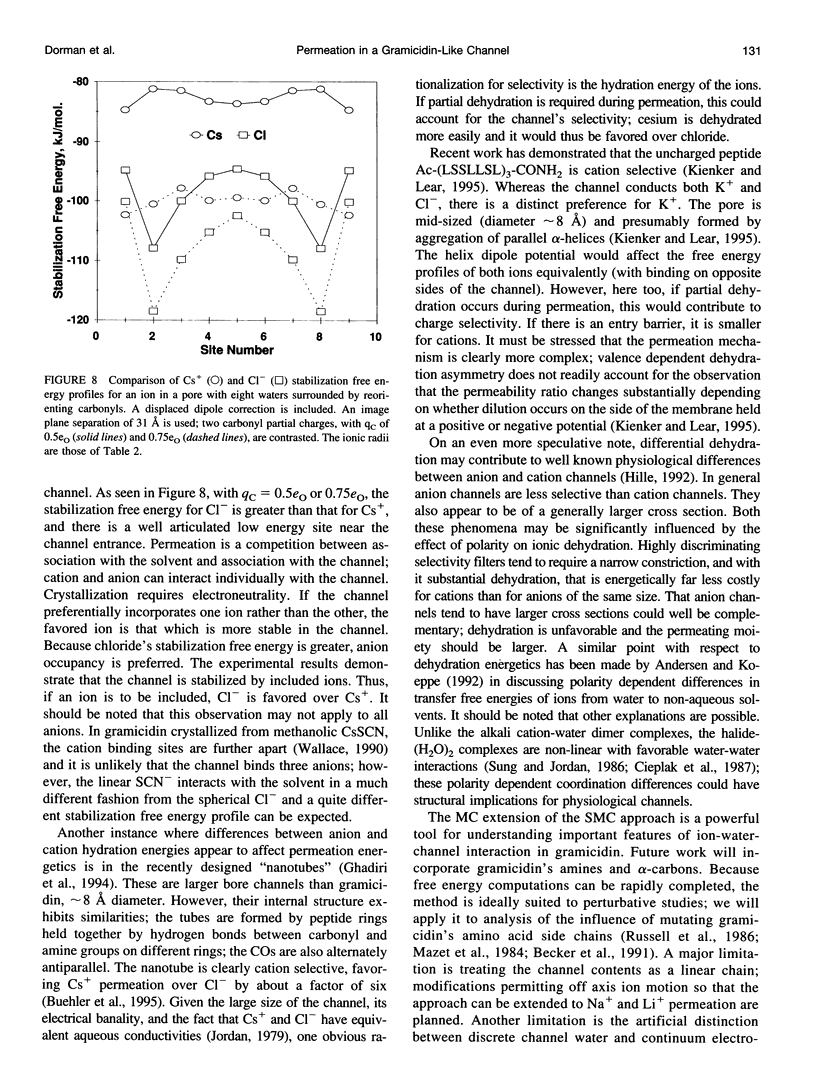
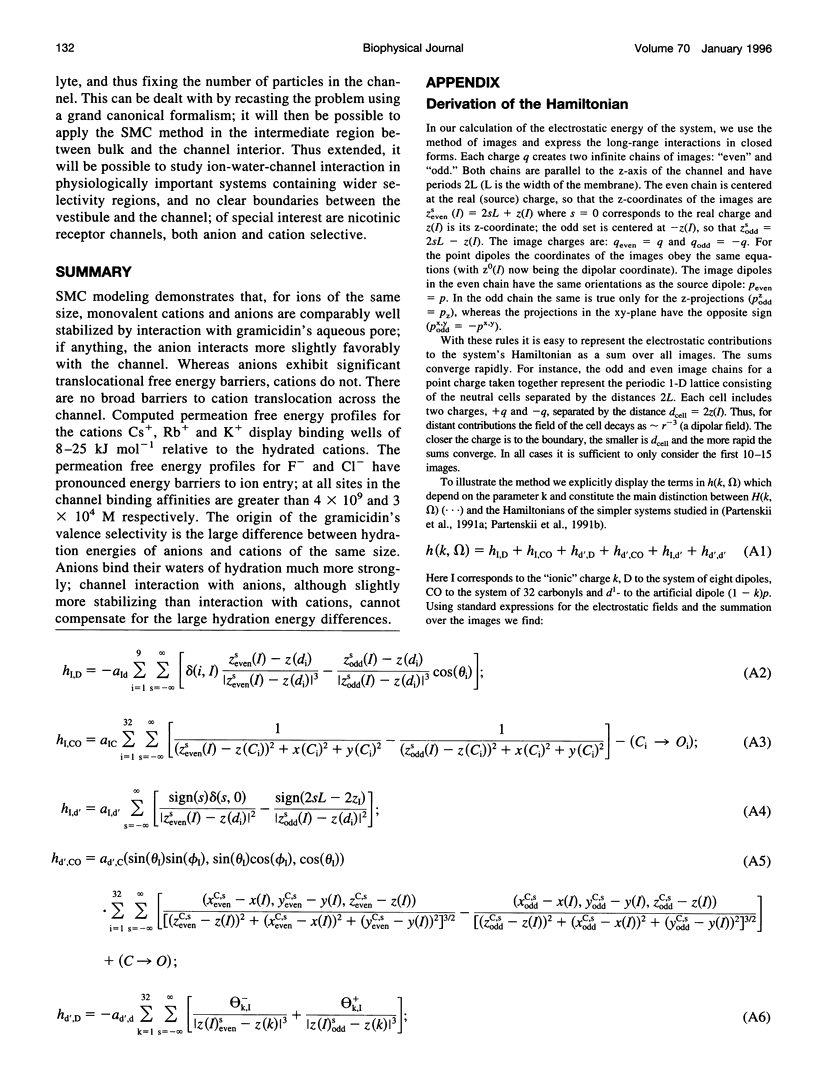
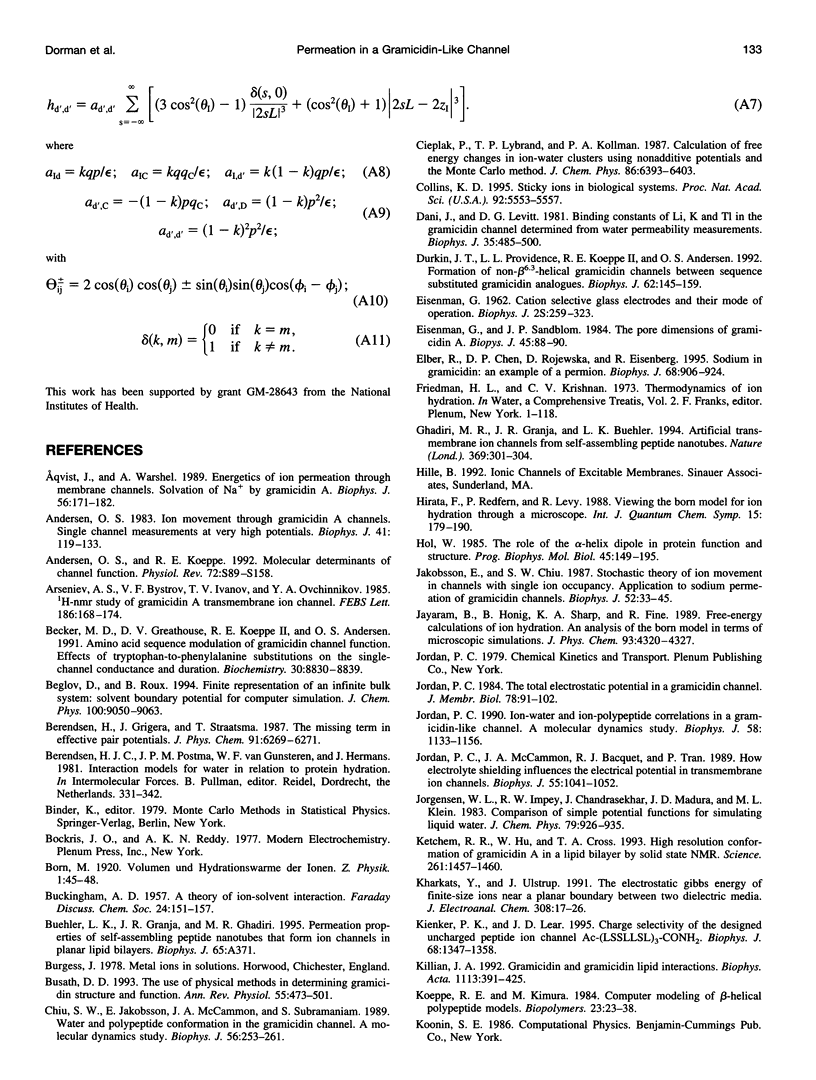
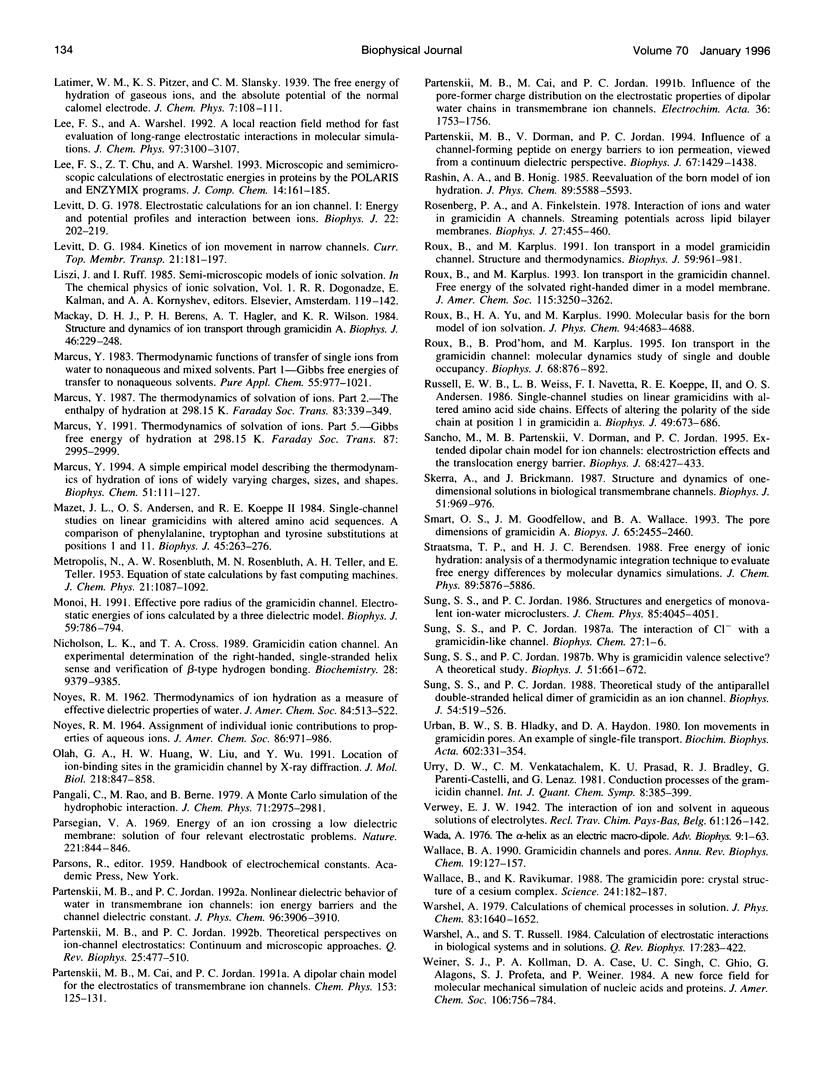
Selected References
These references are in PubMed. This may not be the complete list of references from this article.
- Andersen O. S. Ion movement through gramicidin A channels. Single-channel measurements at very high potentials. Biophys J. 1983 Feb;41(2):119–133. doi: 10.1016/S0006-3495(83)84414-2. [DOI] [PMC free article] [PubMed] [Google Scholar]
- Andersen O. S., Koeppe R. E., 2nd Molecular determinants of channel function. Physiol Rev. 1992 Oct;72(4 Suppl):S89–158. doi: 10.1152/physrev.1992.72.suppl_4.S89. [DOI] [PubMed] [Google Scholar]
- Aqvist J., Warshel A. Energetics of ion permeation through membrane channels. Solvation of Na+ by gramicidin A. Biophys J. 1989 Jul;56(1):171–182. doi: 10.1016/S0006-3495(89)82662-1. [DOI] [PMC free article] [PubMed] [Google Scholar]
- Arseniev A. S., Barsukov I. L., Bystrov V. F., Lomize A. L., Ovchinnikov YuA 1H-NMR study of gramicidin A transmembrane ion channel. Head-to-head right-handed, single-stranded helices. FEBS Lett. 1985 Jul 8;186(2):168–174. doi: 10.1016/0014-5793(85)80702-x. [DOI] [PubMed] [Google Scholar]
- Becker M. D., Greathouse D. V., Koeppe R. E., 2nd, Andersen O. S. Amino acid sequence modulation of gramicidin channel function: effects of tryptophan-to-phenylalanine substitutions on the single-channel conductance and duration. Biochemistry. 1991 Sep 10;30(36):8830–8839. doi: 10.1021/bi00100a015. [DOI] [PubMed] [Google Scholar]
- Busath D. D. The use of physical methods in determining gramicidin channel structure and function. Annu Rev Physiol. 1993;55:473–501. doi: 10.1146/annurev.ph.55.030193.002353. [DOI] [PubMed] [Google Scholar]
- Chiu S. W., Subramaniam S., Jakobsson E., McCammon J. A. Water and polypeptide conformations in the gramicidin channel. A molecular dynamics study. Biophys J. 1989 Aug;56(2):253–261. doi: 10.1016/S0006-3495(89)82671-2. [DOI] [PMC free article] [PubMed] [Google Scholar]
- Collins K. D. Sticky ions in biological systems. Proc Natl Acad Sci U S A. 1995 Jun 6;92(12):5553–5557. doi: 10.1073/pnas.92.12.5553. [DOI] [PMC free article] [PubMed] [Google Scholar]
- Dani J. A., Levitt D. G. Binding constants of Li+, K+, and Tl+ in the gramicidin channel determined from water permeability measurements. Biophys J. 1981 Aug;35(2):485–499. doi: 10.1016/S0006-3495(81)84804-7. [DOI] [PMC free article] [PubMed] [Google Scholar]
- Durkin J. T., Providence L. L., Koeppe R. E., 2nd, Andersen O. S. Formation of non-beta 6.3-helical gramicidin channels between sequence-substituted gramicidin analogues. Biophys J. 1992 Apr;62(1):145–159. doi: 10.1016/S0006-3495(92)81801-5. [DOI] [PMC free article] [PubMed] [Google Scholar]
- EISENMAN G. Cation selective glass electrodes and their mode of operation. Biophys J. 1962 Mar;2(2 Pt 2):259–323. doi: 10.1016/s0006-3495(62)86959-8. [DOI] [PMC free article] [PubMed] [Google Scholar]
- Eisenman G., Sandblom J. P. Modeling the gramicidin channel: interpretation of experimental data using rate theory. Biophys J. 1984 Jan;45(1):88–90. doi: 10.1016/S0006-3495(84)84119-3. [DOI] [PMC free article] [PubMed] [Google Scholar]
- Elber R., Chen D. P., Rojewska D., Eisenberg R. Sodium in gramicidin: an example of a permion. Biophys J. 1995 Mar;68(3):906–924. doi: 10.1016/S0006-3495(95)80267-5. [DOI] [PMC free article] [PubMed] [Google Scholar]
- Ghadiri M. R., Granja J. R., Buehler L. K. Artificial transmembrane ion channels from self-assembling peptide nanotubes. Nature. 1994 May 26;369(6478):301–304. doi: 10.1038/369301a0. [DOI] [PubMed] [Google Scholar]
- Hol W. G. The role of the alpha-helix dipole in protein function and structure. Prog Biophys Mol Biol. 1985;45(3):149–195. doi: 10.1016/0079-6107(85)90001-x. [DOI] [PubMed] [Google Scholar]
- Jakobsson E., Chiu S. W. Stochastic theory of ion movement in channels with single-ion occupancy. Application to sodium permeation of gramicidin channels. Biophys J. 1987 Jul;52(1):33–45. doi: 10.1016/S0006-3495(87)83186-7. [DOI] [PMC free article] [PubMed] [Google Scholar]
- Jordan P. C., Bacquet R. J., McCammon J. A., Tran P. How electrolyte shielding influences the electrical potential in transmembrane ion channels. Biophys J. 1989 Jun;55(6):1041–1052. doi: 10.1016/S0006-3495(89)82903-0. [DOI] [PMC free article] [PubMed] [Google Scholar]
- Jordan P. C. Ion-water and ion-polypeptide correlations in a gramicidin-like channel. A molecular dynamics study. Biophys J. 1990 Nov;58(5):1133–1156. doi: 10.1016/S0006-3495(90)82456-5. [DOI] [PMC free article] [PubMed] [Google Scholar]
- Ketchem R. R., Hu W., Cross T. A. High-resolution conformation of gramicidin A in a lipid bilayer by solid-state NMR. Science. 1993 Sep 10;261(5127):1457–1460. doi: 10.1126/science.7690158. [DOI] [PubMed] [Google Scholar]
- Kienker P. K., Lear J. D. Charge selectivity of the designed uncharged peptide ion channel Ac-(LSSLLSL)3-CONH2. Biophys J. 1995 Apr;68(4):1347–1358. doi: 10.1016/S0006-3495(95)80307-3. [DOI] [PMC free article] [PubMed] [Google Scholar]
- Killian J. A. Gramicidin and gramicidin-lipid interactions. Biochim Biophys Acta. 1992 Dec 11;1113(3-4):391–425. doi: 10.1016/0304-4157(92)90008-x. [DOI] [PubMed] [Google Scholar]
- Levitt D. G. Electrostatic calculations for an ion channel. I. Energy and potential profiles and interactions between ions. Biophys J. 1978 May;22(2):209–219. doi: 10.1016/S0006-3495(78)85485-X. [DOI] [PMC free article] [PubMed] [Google Scholar]
- Mackay D. H., Berens P. H., Wilson K. R., Hagler A. T. Structure and dynamics of ion transport through gramicidin A. Biophys J. 1984 Aug;46(2):229–248. doi: 10.1016/S0006-3495(84)84016-3. [DOI] [PMC free article] [PubMed] [Google Scholar]
- Mazet J. L., Andersen O. S., Koeppe R. E., 2nd Single-channel studies on linear gramicidins with altered amino acid sequences. A comparison of phenylalanine, tryptophane, and tyrosine substitutions at positions 1 and 11. Biophys J. 1984 Jan;45(1):263–276. doi: 10.1016/S0006-3495(84)84153-3. [DOI] [PMC free article] [PubMed] [Google Scholar]
- Monoi H. Effective pore radius of the gramicidin channel. Electrostatic energies of ions calculated by a three-dielectric model. Biophys J. 1991 Apr;59(4):786–794. doi: 10.1016/S0006-3495(91)82291-3. [DOI] [PMC free article] [PubMed] [Google Scholar]
- Nicholson L. K., Cross T. A. Gramicidin cation channel: an experimental determination of the right-handed helix sense and verification of beta-type hydrogen bonding. Biochemistry. 1989 Nov 28;28(24):9379–9385. doi: 10.1021/bi00450a019. [DOI] [PubMed] [Google Scholar]
- Olah G. A., Huang H. W., Liu W. H., Wu Y. L. Location of ion-binding sites in the gramicidin channel by X-ray diffraction. J Mol Biol. 1991 Apr 20;218(4):847–858. doi: 10.1016/0022-2836(91)90272-8. [DOI] [PubMed] [Google Scholar]
- Parsegian A. Energy of an ion crossing a low dielectric membrane: solutions to four relevant electrostatic problems. Nature. 1969 Mar 1;221(5183):844–846. doi: 10.1038/221844a0. [DOI] [PubMed] [Google Scholar]
- Partenskii M. B., Dorman V., Jordan P. C. Influence of a channel-forming peptide on energy barriers to ion permeation, viewed from a continuum dielectric perspective. Biophys J. 1994 Oct;67(4):1429–1438. doi: 10.1016/S0006-3495(94)80616-2. [DOI] [PMC free article] [PubMed] [Google Scholar]
- Partenskii M. B., Jordan P. C. Theoretical perspectives on ion-channel electrostatics: continuum and microscopic approaches. Q Rev Biophys. 1992 Nov;25(4):477–510. doi: 10.1017/s0033583500004388. [DOI] [PubMed] [Google Scholar]
- Roux B., Karplus M. Ion transport in a model gramicidin channel. Structure and thermodynamics. Biophys J. 1991 May;59(5):961–981. doi: 10.1016/S0006-3495(91)82311-6. [DOI] [PMC free article] [PubMed] [Google Scholar]
- Roux B., Prod'hom B., Karplus M. Ion transport in the gramicidin channel: molecular dynamics study of single and double occupancy. Biophys J. 1995 Mar;68(3):876–892. doi: 10.1016/S0006-3495(95)80264-X. [DOI] [PMC free article] [PubMed] [Google Scholar]
- Russell E. W., Weiss L. B., Navetta F. I., Koeppe R. E., 2nd, Andersen O. S. Single-channel studies on linear gramicidins with altered amino acid side chains. Effects of altering the polarity of the side chain at position 1 in gramicidin A. Biophys J. 1986 Mar;49(3):673–686. doi: 10.1016/S0006-3495(86)83694-3. [DOI] [PMC free article] [PubMed] [Google Scholar]
- Sancho M., Partenskii M. B., Dorman V., Jordan P. C. Extended dipolar chain model for ion channels: electrostriction effects and the translocational energy barrier. Biophys J. 1995 Feb;68(2):427–433. doi: 10.1016/S0006-3495(95)80204-3. [DOI] [PMC free article] [PubMed] [Google Scholar]
- Skerra A., Brickmann J. Structure and dynamics of one-dimensional ionic solutions in biological transmembrane channels. Biophys J. 1987 Jun;51(6):969–976. doi: 10.1016/S0006-3495(87)83424-0. [DOI] [PMC free article] [PubMed] [Google Scholar]
- Smart O. S., Goodfellow J. M., Wallace B. A. The pore dimensions of gramicidin A. Biophys J. 1993 Dec;65(6):2455–2460. doi: 10.1016/S0006-3495(93)81293-1. [DOI] [PMC free article] [PubMed] [Google Scholar]
- Sung S. S., Jordan P. C. The interaction of Cl- with a gramicidin-like channel. Biophys Chem. 1987 Jul;27(1):1–6. doi: 10.1016/0301-4622(87)80041-8. [DOI] [PubMed] [Google Scholar]
- Sung S. S., Jordan P. C. Theoretical study of the antiparallel double-stranded helical dimer of gramicidin as an ion channel. Biophys J. 1988 Sep;54(3):519–526. doi: 10.1016/S0006-3495(88)82984-9. [DOI] [PMC free article] [PubMed] [Google Scholar]
- Sung S. S., Jordan P. C. Why is gramicidin valence selective? A theoretical study. Biophys J. 1987 Apr;51(4):661–672. doi: 10.1016/S0006-3495(87)83391-X. [DOI] [PMC free article] [PubMed] [Google Scholar]
- Urban B. W., Hladky S. B., Haydon D. A. Ion movements in gramicidin pores. An example of single-file transport. Biochim Biophys Acta. 1980 Nov 4;602(2):331–354. doi: 10.1016/0005-2736(80)90316-8. [DOI] [PubMed] [Google Scholar]
- Wada A. The alpha-helix as an electric macro-dipole. Adv Biophys. 1976:1–63. [PubMed] [Google Scholar]
- Wallace B. A. Gramicidin channels and pores. Annu Rev Biophys Biophys Chem. 1990;19:127–157. doi: 10.1146/annurev.bb.19.060190.001015. [DOI] [PubMed] [Google Scholar]
- Wallace B. A., Ravikumar K. The gramicidin pore: crystal structure of a cesium complex. Science. 1988 Jul 8;241(4862):182–187. doi: 10.1126/science.2455344. [DOI] [PubMed] [Google Scholar]
- Warshel A., Russell S. T. Calculations of electrostatic interactions in biological systems and in solutions. Q Rev Biophys. 1984 Aug;17(3):283–422. doi: 10.1017/s0033583500005333. [DOI] [PubMed] [Google Scholar]