Abstract
A new algorithm is presented for idealizing single channel data containing any number of conductance levels. The number of levels and their amplitudes do not have to be known a priori. No assumption has to be made about the behavior of the channel, other than that transitions between conductance levels are fast. The algorithm is relatively insensitive to the complexity of the underlying single channel behavior. Idealization may be reliable with signal-to-noise ratios as low as 3.5. The idealization algorithm uses a slope detector to localize transitions between levels and a relative amplitude criterion to remove spurious transitions. After estimating the number of conductances and their amplitudes, conductance states can be assigned to the idealized levels. In addition to improving the quality of the idealization, this "interpretation" allows a statistical analysis of individual (sub)conductance states.
Full text
PDF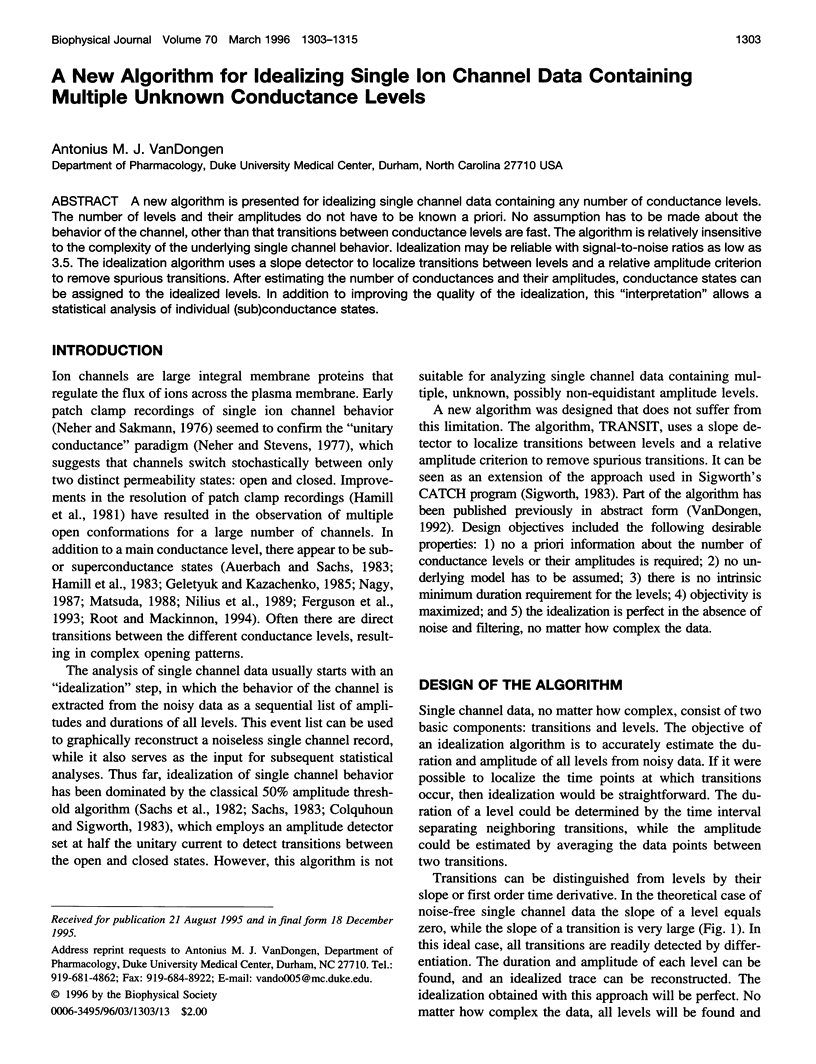
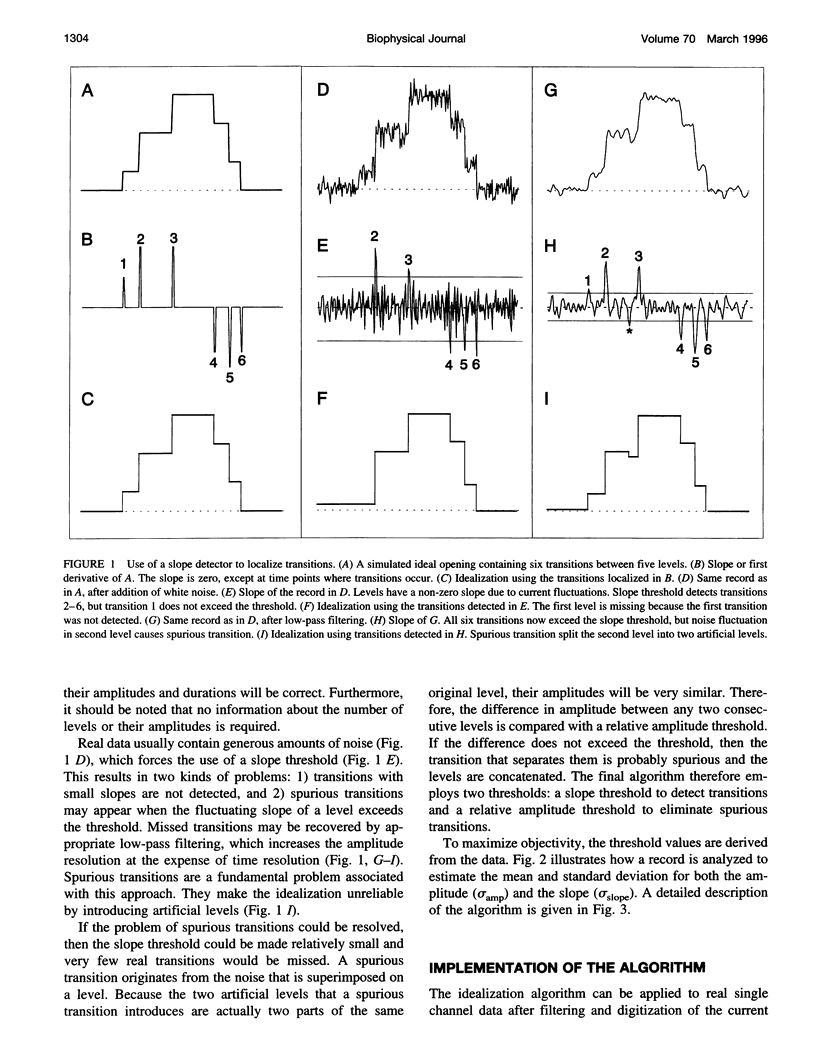
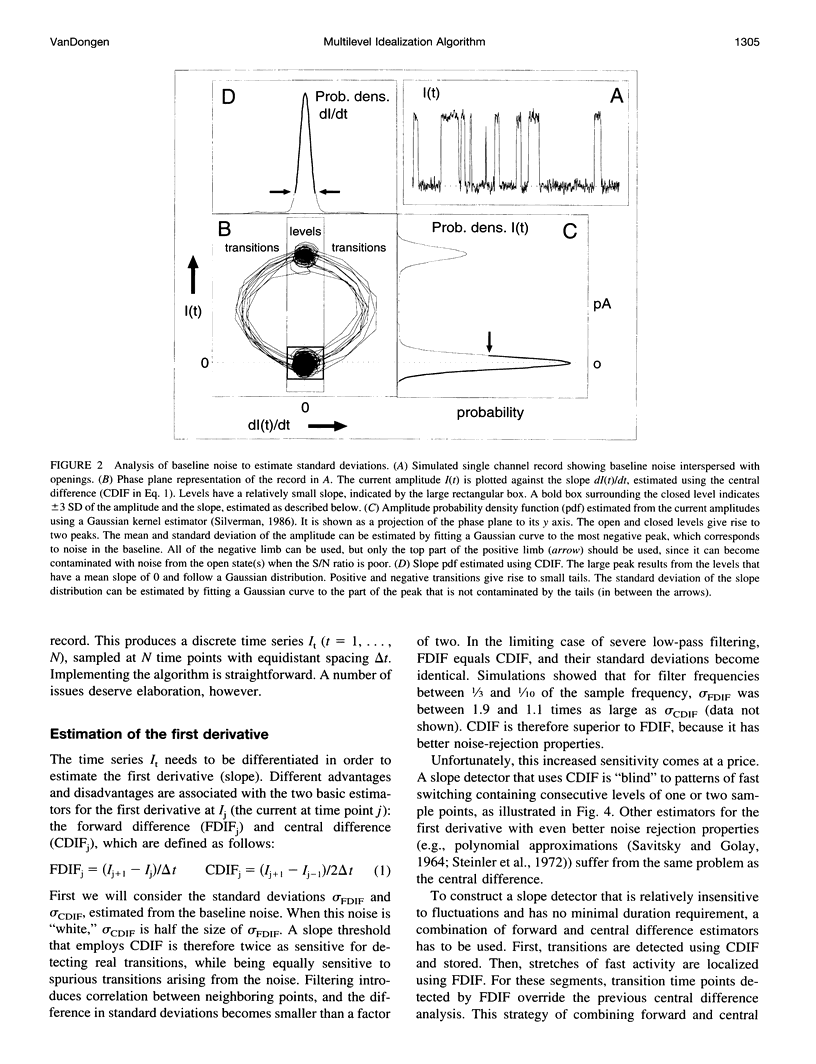
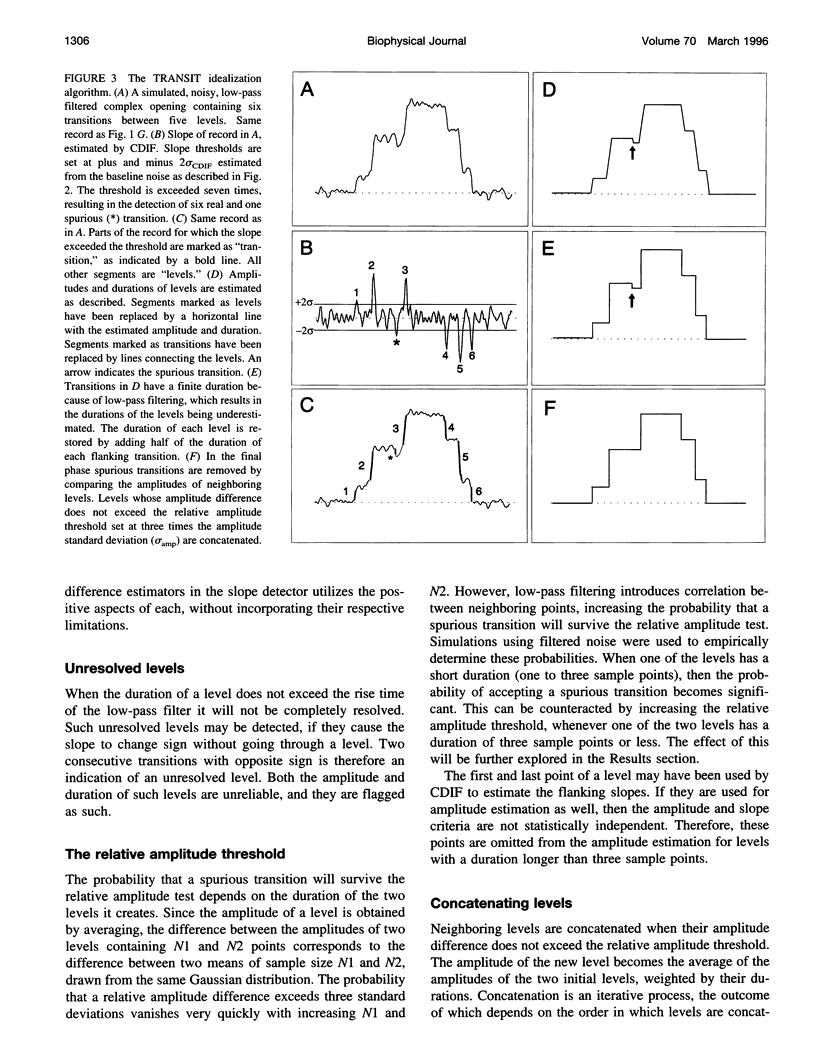
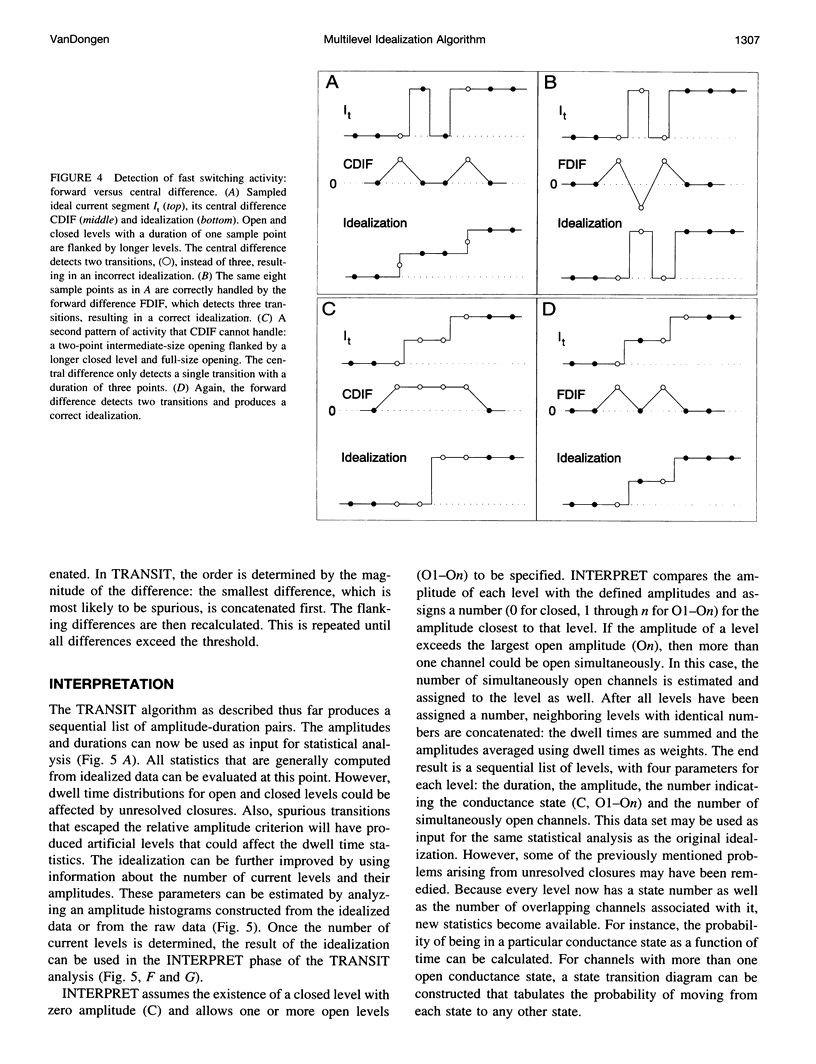
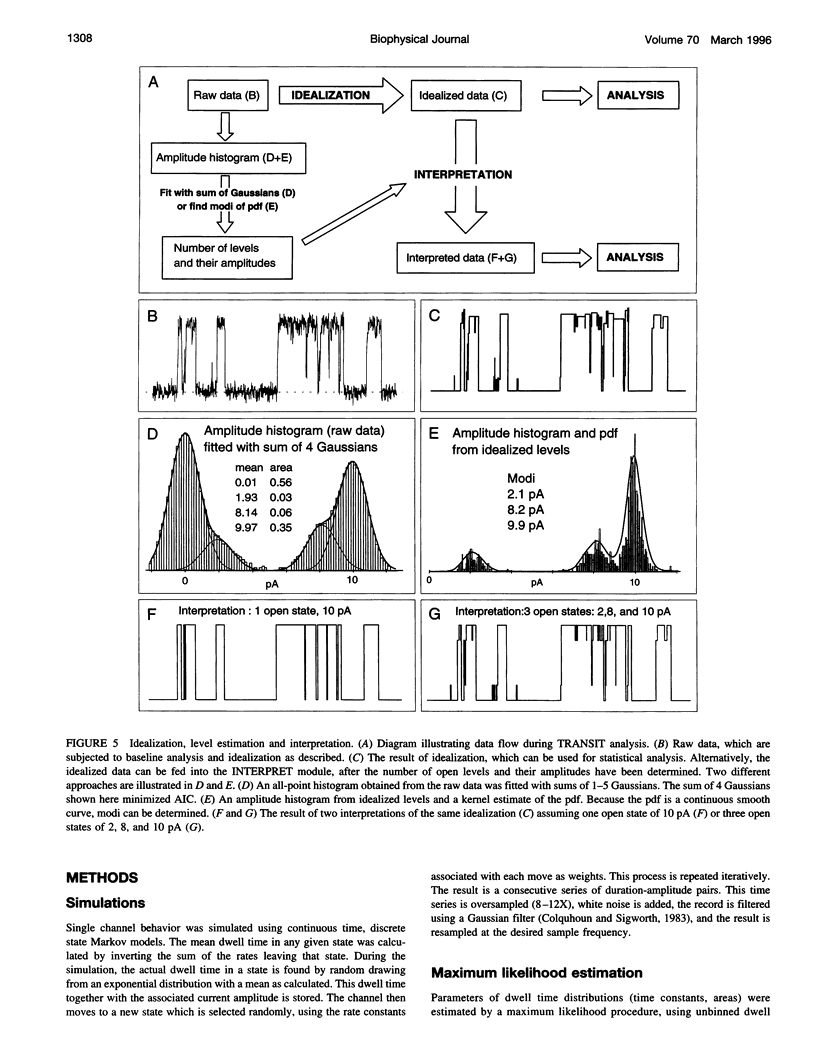
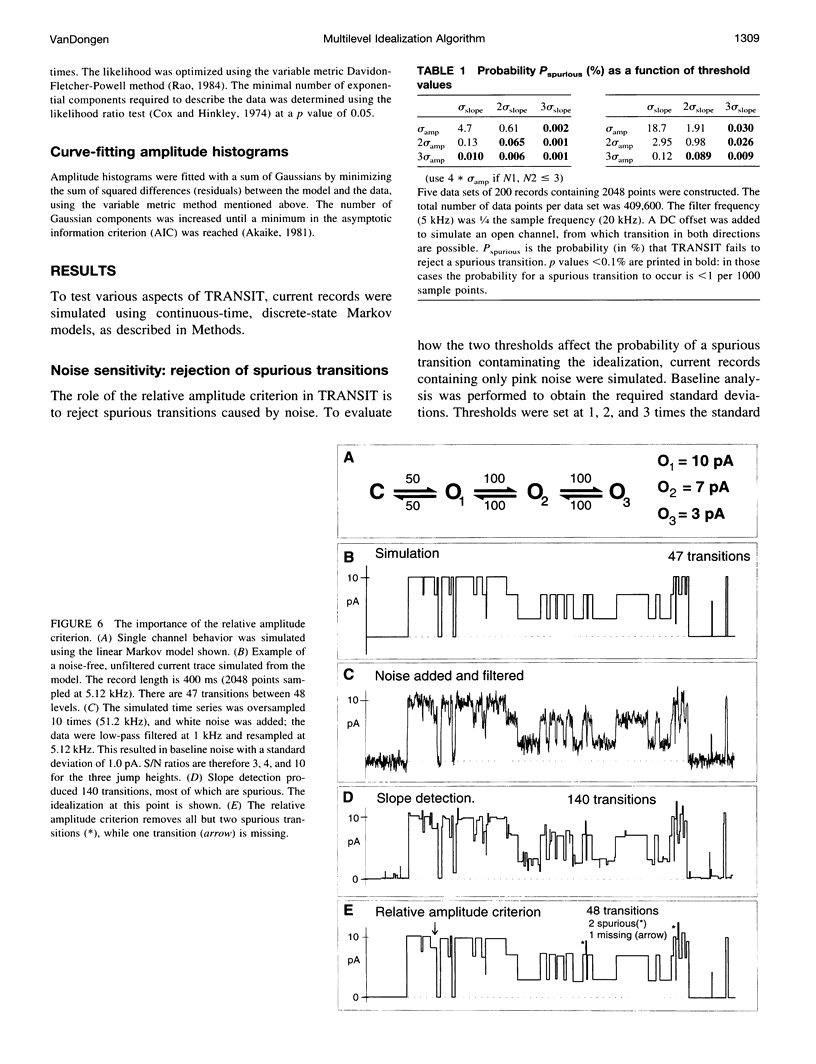
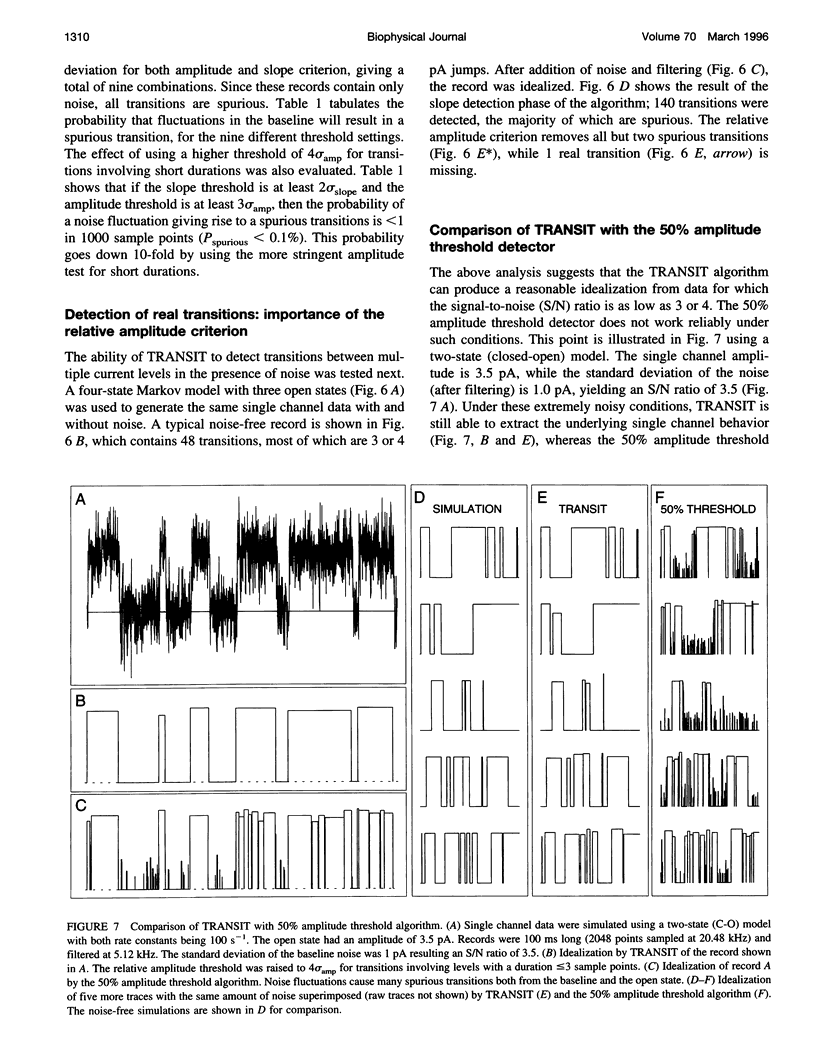
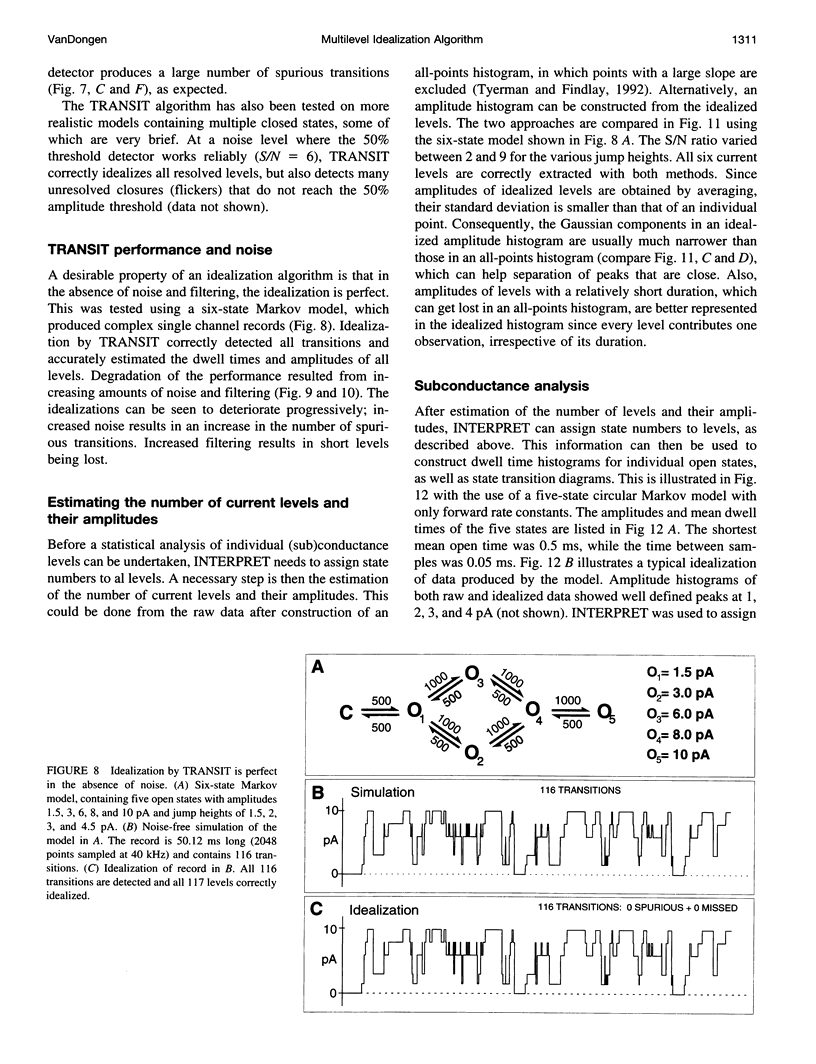
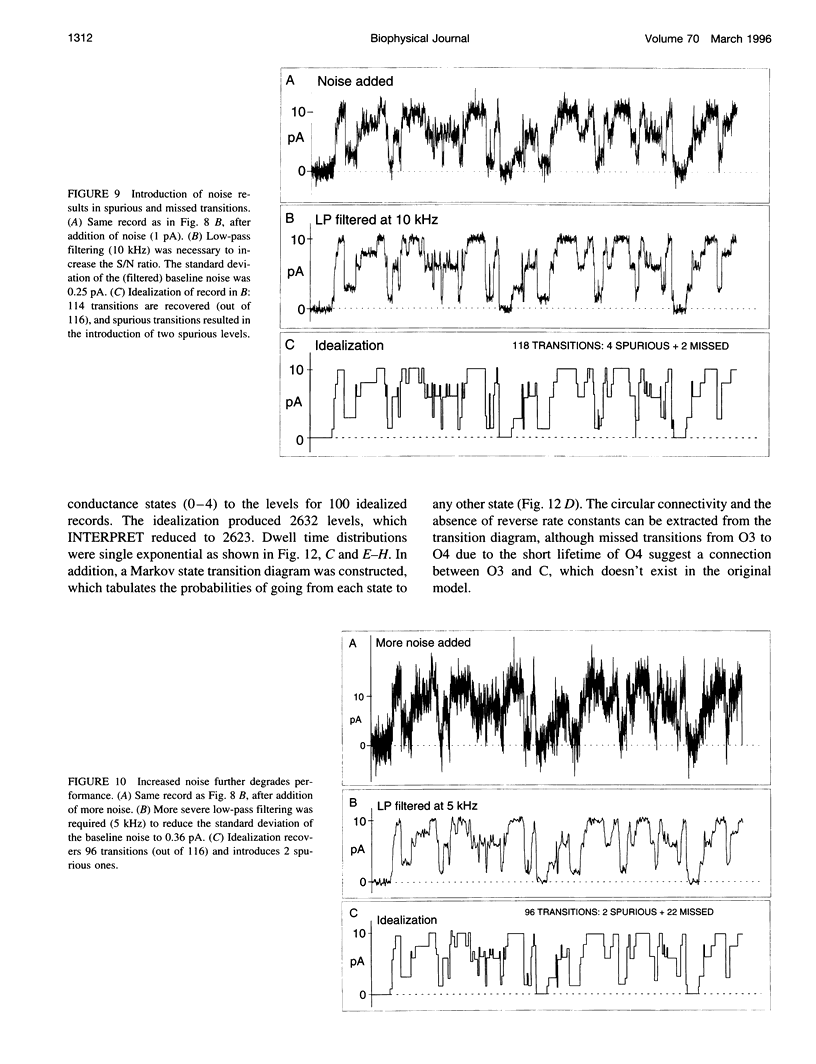
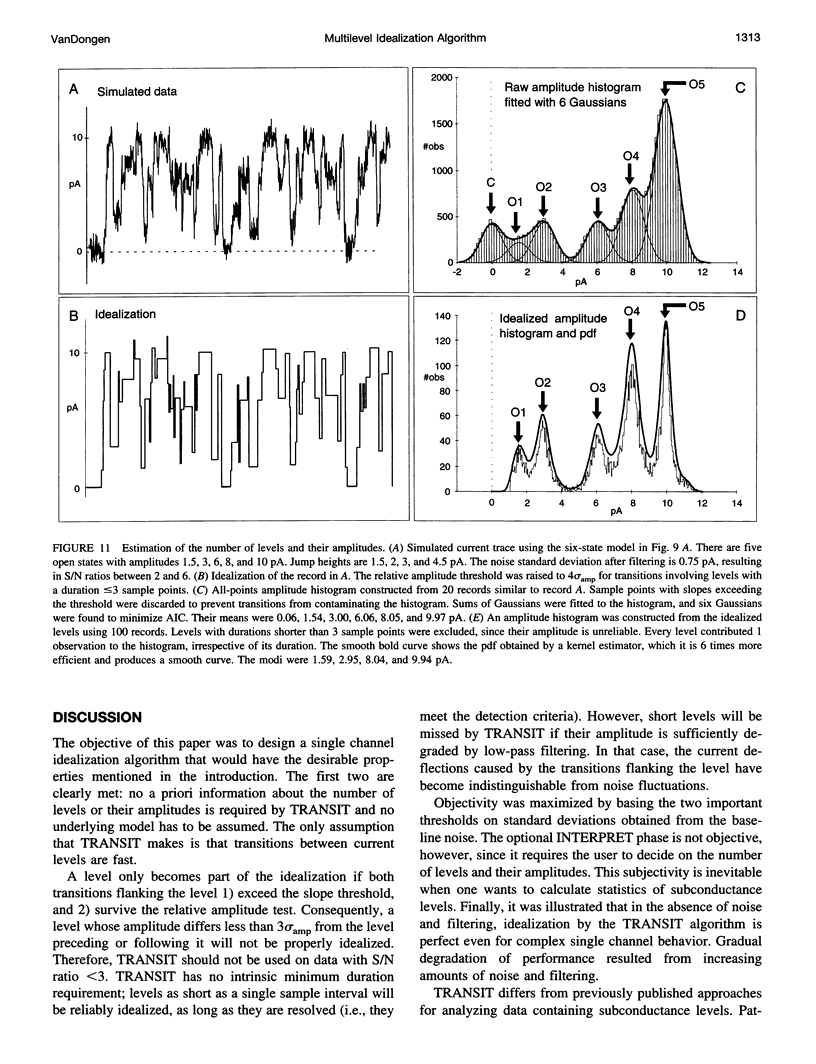
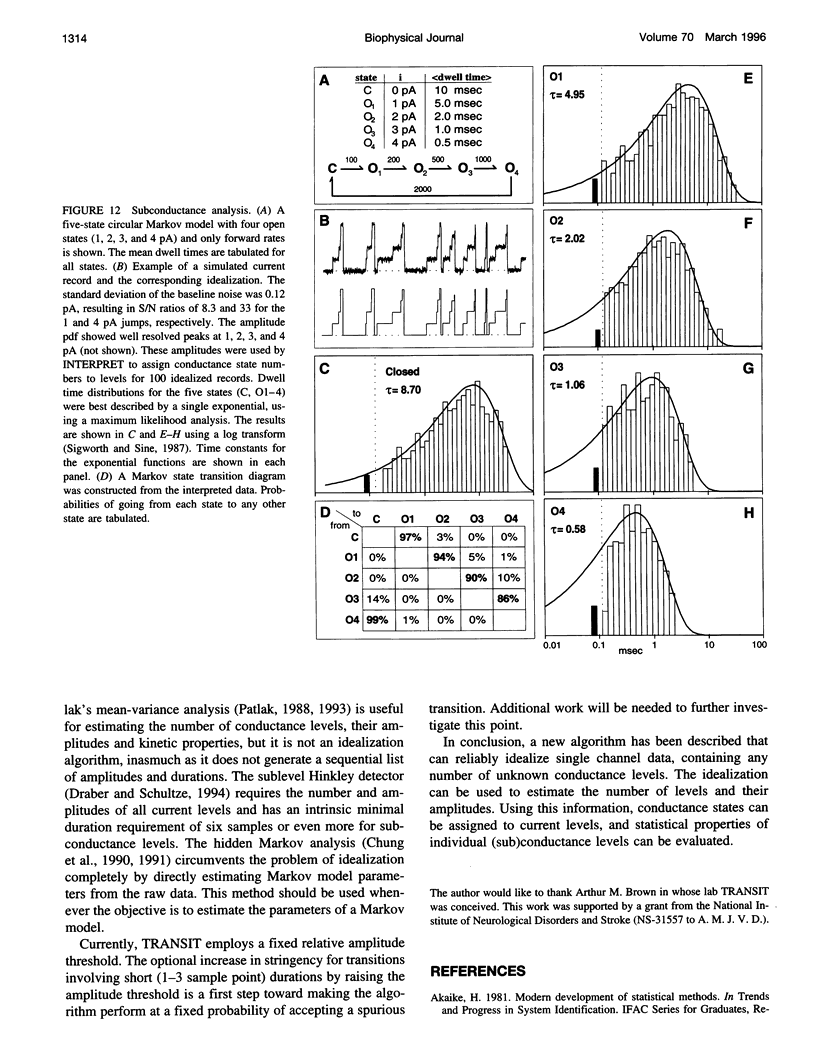
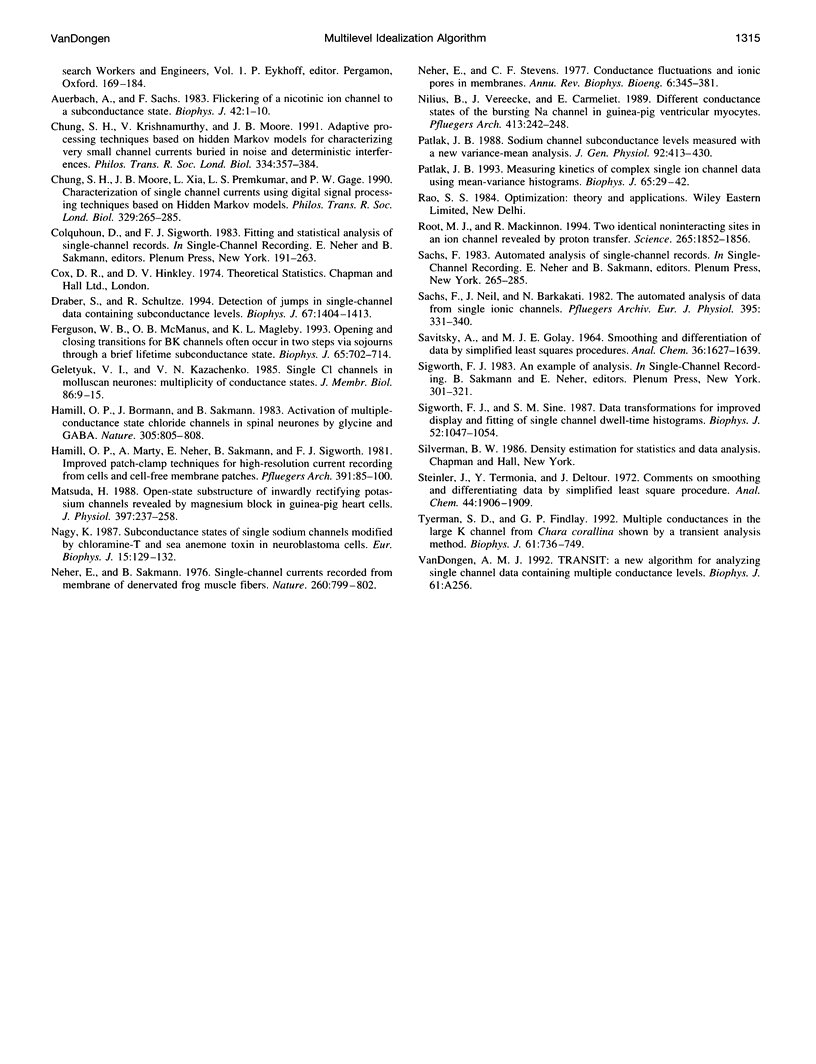
Selected References
These references are in PubMed. This may not be the complete list of references from this article.
- Auerbach A., Sachs F. Flickering of a nicotinic ion channel to a subconductance state. Biophys J. 1983 Apr;42(1):1–10. doi: 10.1016/S0006-3495(83)84362-8. [DOI] [PMC free article] [PubMed] [Google Scholar]
- Chung S. H., Krishnamurthy V., Moore J. B. Adaptive processing techniques based on hidden Markov models for characterizing very small channel currents buried in noise and deterministic interferences. Philos Trans R Soc Lond B Biol Sci. 1991 Dec 30;334(1271):357–384. doi: 10.1098/rstb.1991.0122. [DOI] [PubMed] [Google Scholar]
- Chung S. H., Moore J. B., Xia L. G., Premkumar L. S., Gage P. W. Characterization of single channel currents using digital signal processing techniques based on Hidden Markov Models. Philos Trans R Soc Lond B Biol Sci. 1990 Sep 29;329(1254):265–285. doi: 10.1098/rstb.1990.0170. [DOI] [PubMed] [Google Scholar]
- Draber S., Schultze R. Detection of jumps in single-channel data containing subconductance levels. Biophys J. 1994 Oct;67(4):1404–1413. doi: 10.1016/S0006-3495(94)80614-9. [DOI] [PMC free article] [PubMed] [Google Scholar]
- Ferguson W. B., McManus O. B., Magleby K. L. Opening and closing transitions for BK channels often occur in two steps via sojourns through a brief lifetime subconductance state. Biophys J. 1993 Aug;65(2):702–714. doi: 10.1016/S0006-3495(93)81097-X. [DOI] [PMC free article] [PubMed] [Google Scholar]
- Geletyuk V. I., Kazachenko V. N. Single Cl- channels in molluscan neurones: multiplicity of the conductance states. J Membr Biol. 1985;86(1):9–15. doi: 10.1007/BF01871605. [DOI] [PubMed] [Google Scholar]
- Hamill O. P., Bormann J., Sakmann B. Activation of multiple-conductance state chloride channels in spinal neurones by glycine and GABA. 1983 Oct 27-Nov 2Nature. 305(5937):805–808. doi: 10.1038/305805a0. [DOI] [PubMed] [Google Scholar]
- Hamill O. P., Marty A., Neher E., Sakmann B., Sigworth F. J. Improved patch-clamp techniques for high-resolution current recording from cells and cell-free membrane patches. Pflugers Arch. 1981 Aug;391(2):85–100. doi: 10.1007/BF00656997. [DOI] [PubMed] [Google Scholar]
- Matsuda H. Open-state substructure of inwardly rectifying potassium channels revealed by magnesium block in guinea-pig heart cells. J Physiol. 1988 Mar;397:237–258. doi: 10.1113/jphysiol.1988.sp016998. [DOI] [PMC free article] [PubMed] [Google Scholar]
- Nagy K. Subconductance states of single sodium channels modified by chloramine-T and sea anemone toxin in neuroblastoma cells. Eur Biophys J. 1987;15(3):129–132. doi: 10.1007/BF00263676. [DOI] [PubMed] [Google Scholar]
- Neher E., Sakmann B. Single-channel currents recorded from membrane of denervated frog muscle fibres. Nature. 1976 Apr 29;260(5554):799–802. doi: 10.1038/260799a0. [DOI] [PubMed] [Google Scholar]
- Neher E., Stevens C. F. Conductance fluctuations and ionic pores in membranes. Annu Rev Biophys Bioeng. 1977;6:345–381. doi: 10.1146/annurev.bb.06.060177.002021. [DOI] [PubMed] [Google Scholar]
- Nilius B., Vereecke J., Carmeliet E. Different conductance states of the bursting Na channel in guinea-pig ventricular myocytes. Pflugers Arch. 1989 Jan;413(3):242–248. doi: 10.1007/BF00583536. [DOI] [PubMed] [Google Scholar]
- Patlak J. B. Measuring kinetics of complex single ion channel data using mean-variance histograms. Biophys J. 1993 Jul;65(1):29–42. doi: 10.1016/S0006-3495(93)81041-5. [DOI] [PMC free article] [PubMed] [Google Scholar]
- Patlak J. B. Sodium channel subconductance levels measured with a new variance-mean analysis. J Gen Physiol. 1988 Oct;92(4):413–430. doi: 10.1085/jgp.92.4.413. [DOI] [PMC free article] [PubMed] [Google Scholar]
- Root M. J., MacKinnon R. Two identical noninteracting sites in an ion channel revealed by proton transfer. Science. 1994 Sep 23;265(5180):1852–1856. doi: 10.1126/science.7522344. [DOI] [PubMed] [Google Scholar]
- Sachs F., Neil J., Barkakati N. The automated analysis of data from single ionic channels. Pflugers Arch. 1982 Dec;395(4):331–340. doi: 10.1007/BF00580798. [DOI] [PubMed] [Google Scholar]
- Sigworth F. J., Sine S. M. Data transformations for improved display and fitting of single-channel dwell time histograms. Biophys J. 1987 Dec;52(6):1047–1054. doi: 10.1016/S0006-3495(87)83298-8. [DOI] [PMC free article] [PubMed] [Google Scholar]
- Tyerman S. D., Terry B. R., Findlay G. P. Multiple conductances in the large K+ channel from Chara corallina shown by a transient analysis method. Biophys J. 1992 Mar;61(3):736–749. doi: 10.1016/S0006-3495(92)81878-7. [DOI] [PMC free article] [PubMed] [Google Scholar]