Abstract
The role of the vestibule in influencing the permeation of ions through biological ion channels is investigated. We derive analytical expressions for the electric potential satisfying Poisson's equation with prolate spheroidal boundary conditions. To allow more realistic geometries we devise an iterative method to calculate the electric potential arising from a fixed charge and an arbitrary dielectric boundary, and confirm that the analytical expressions and iterative method give similar potential values. We then investigate the size of the potential barrier presented to an ion by model vestibules of conical and catenary shapes. The height of the potential barrier increases steeply as an ion enters the vestibule and moves toward the constricted region of the channel. We show that the barrier presented by, for example, a 15 degrees conical vestibule can be canceled by placing dipoles with a total moment of about 50 Debyes near the constricted region of the pore. The selectivity of cations and anions can result from the polarity of charge groups or the orientation of dipoles located near the constricted region of the channel.
Full text
PDF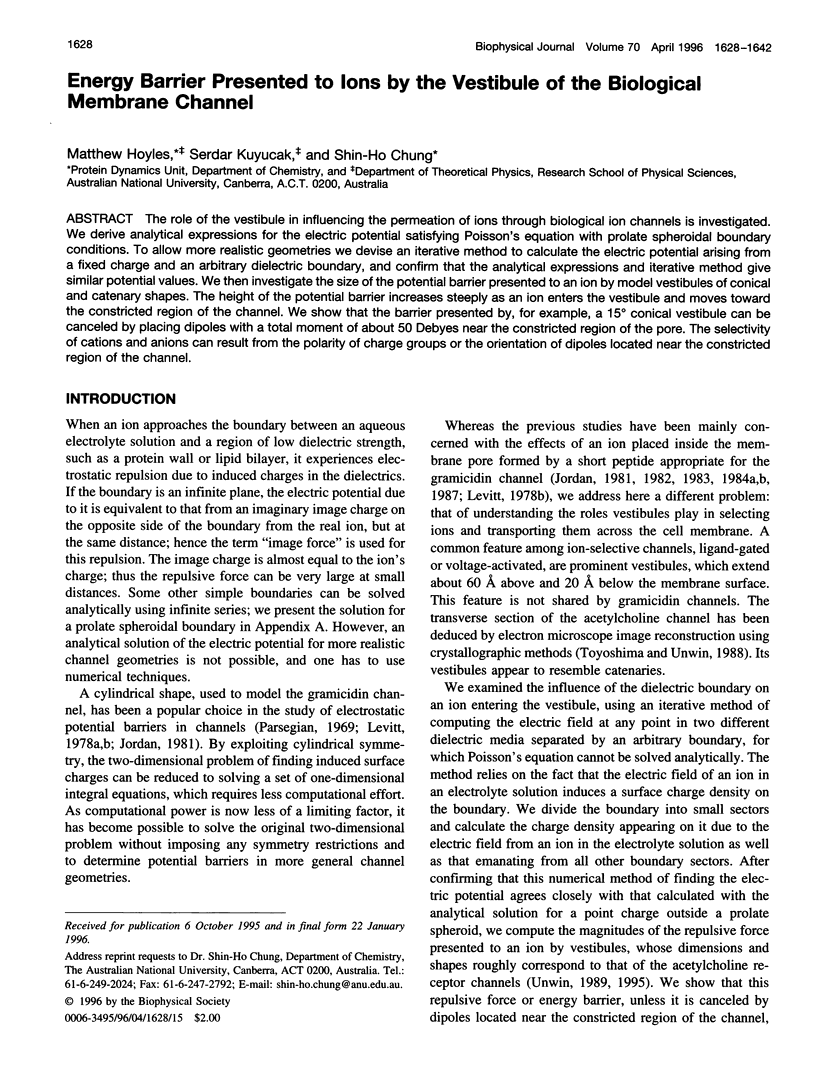
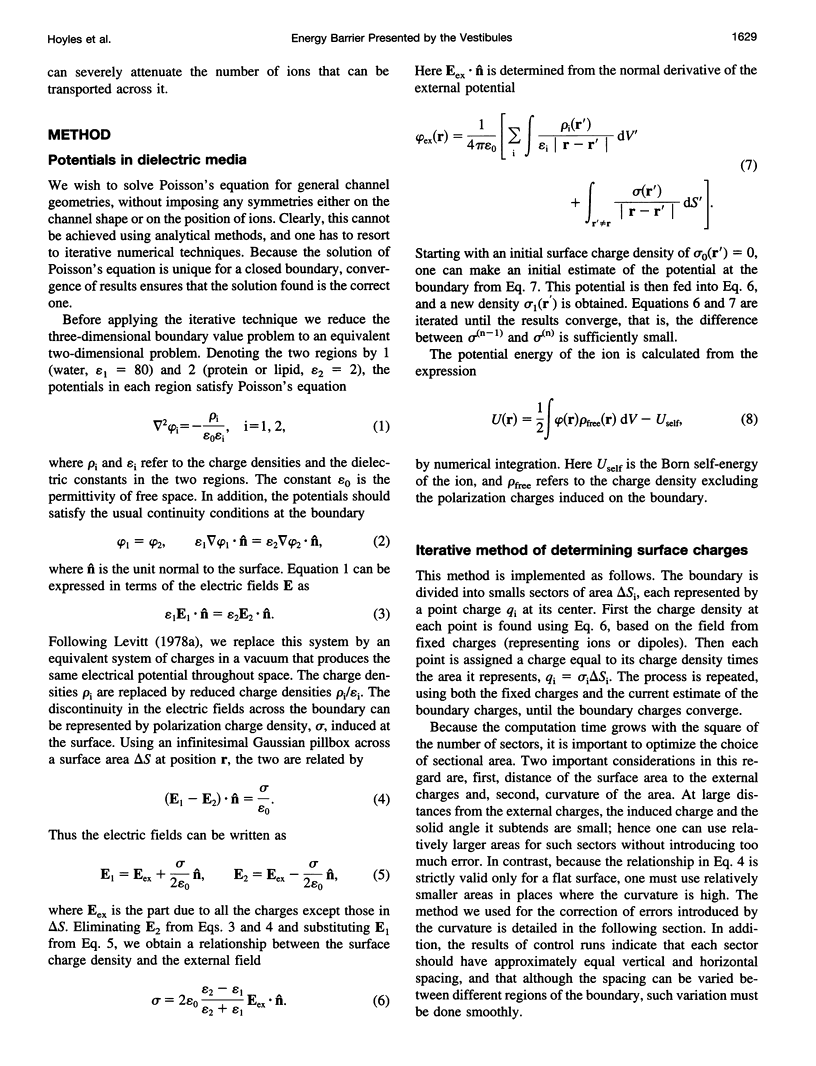
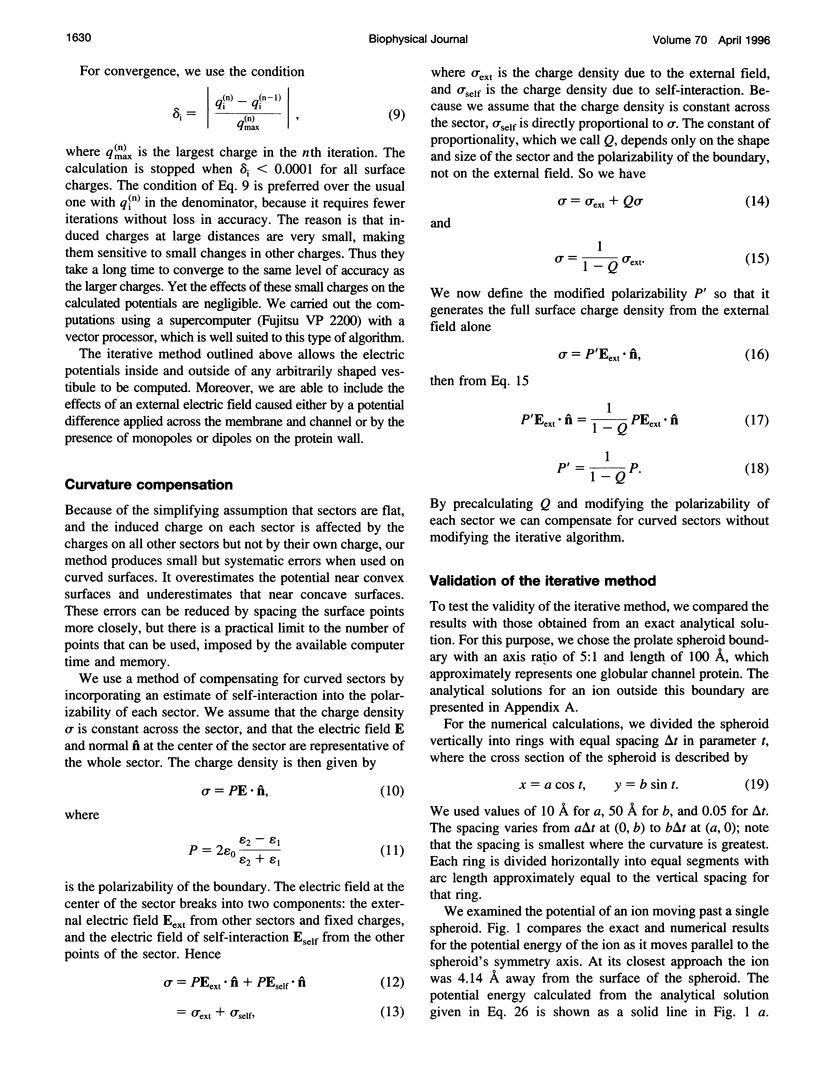
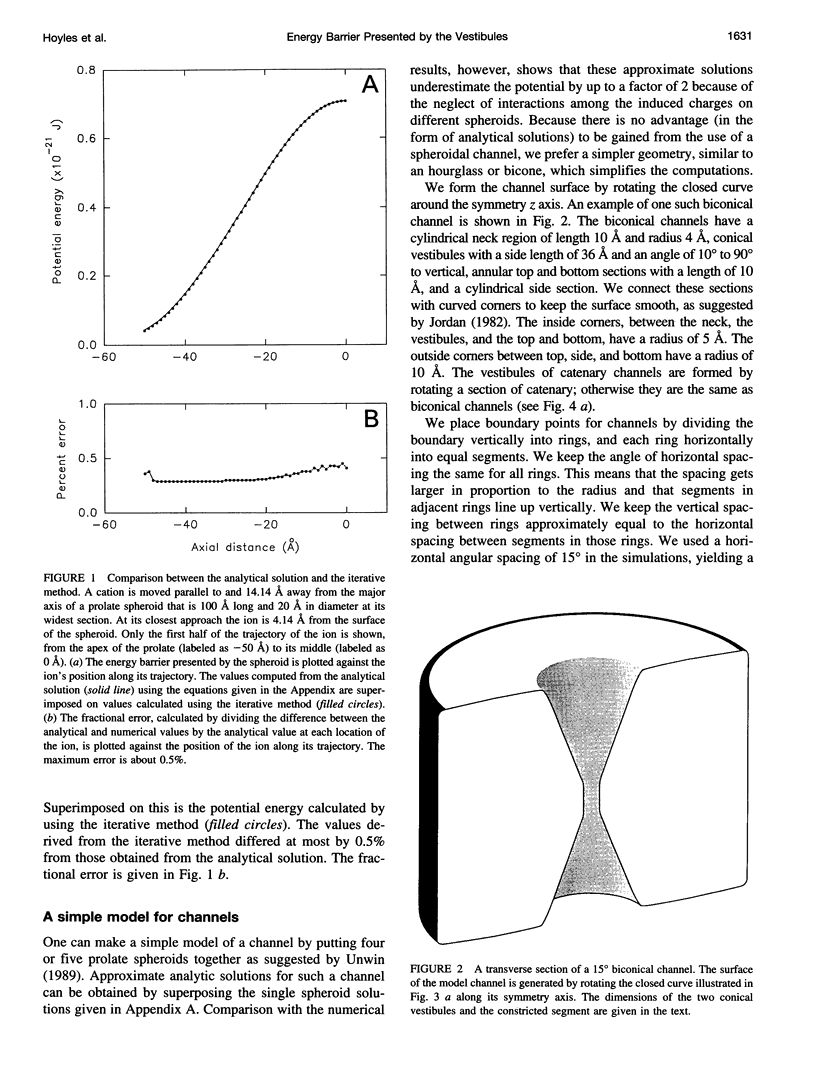
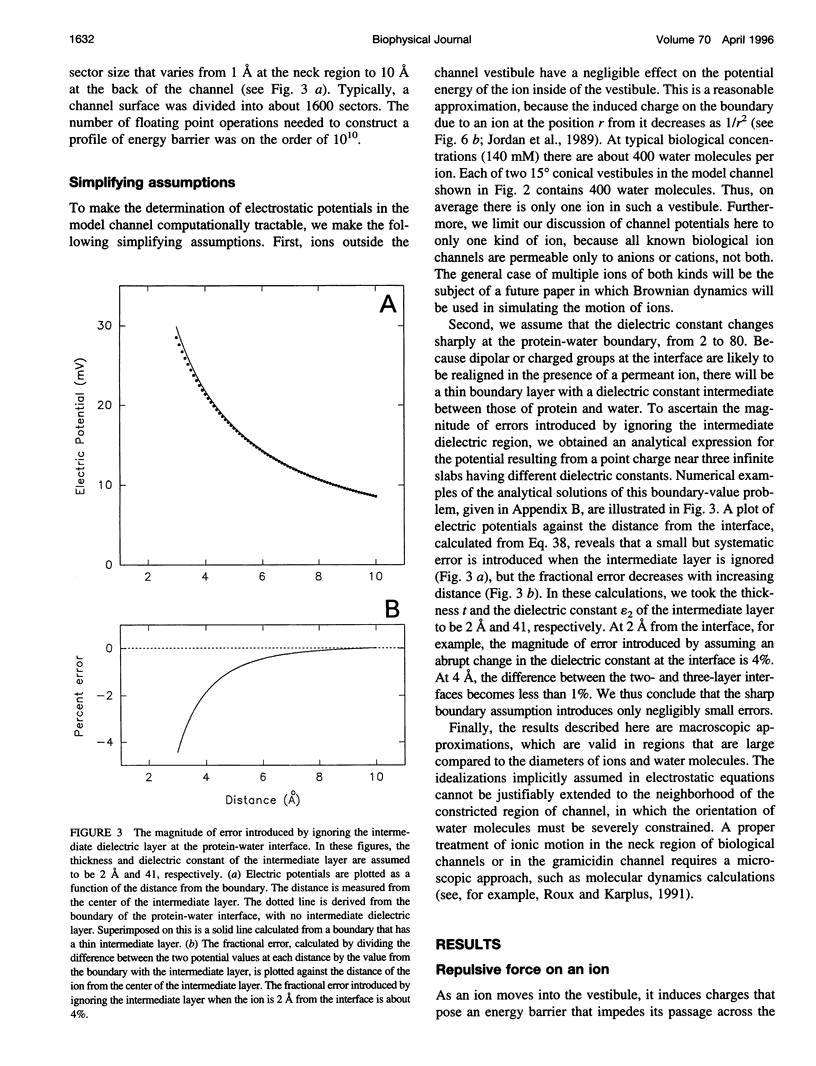
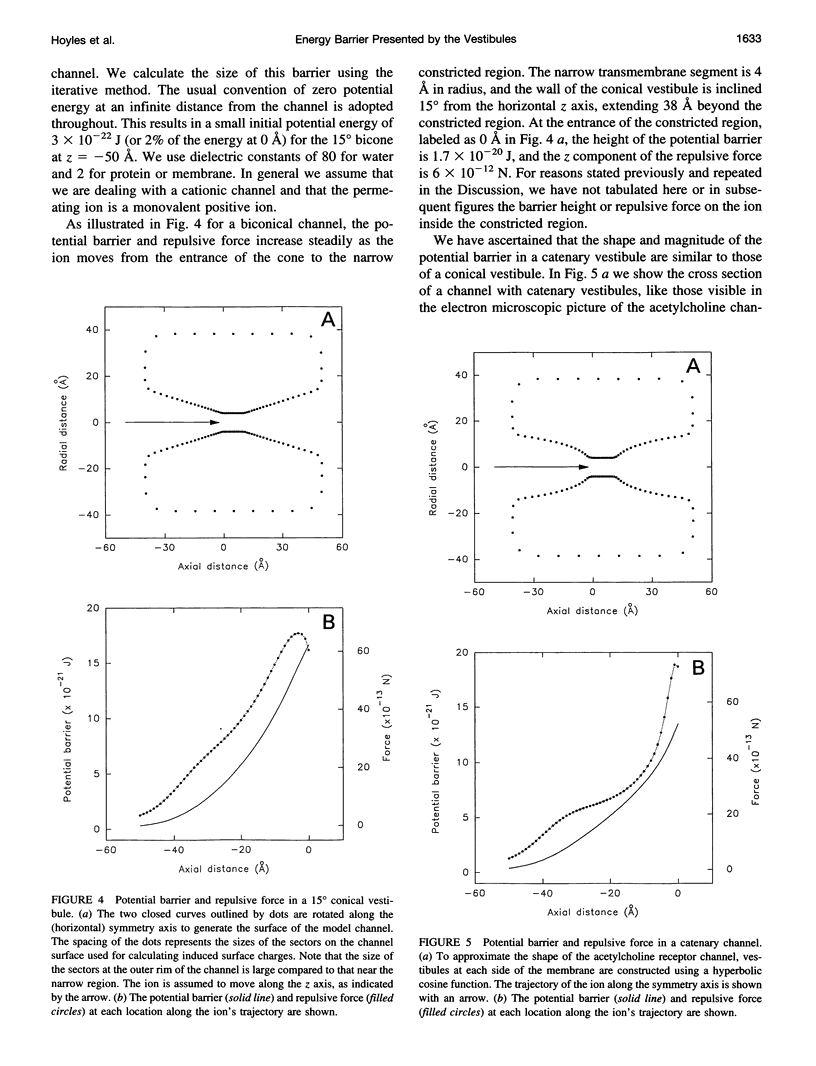
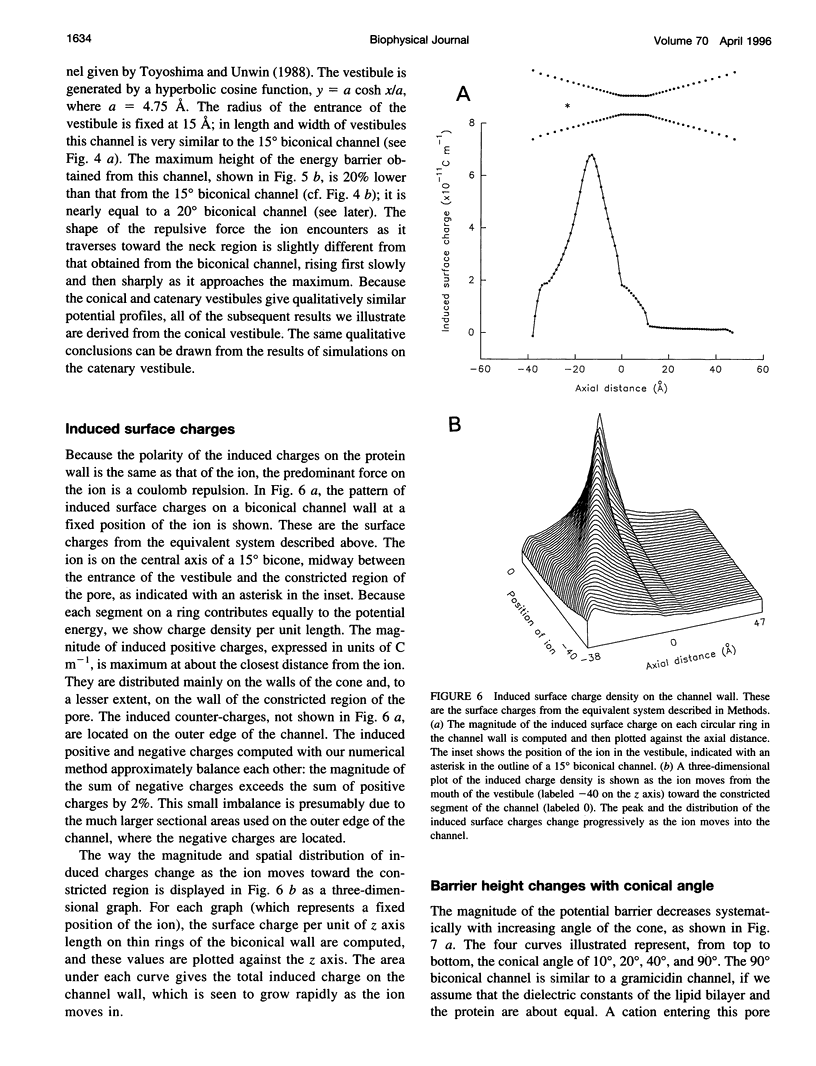
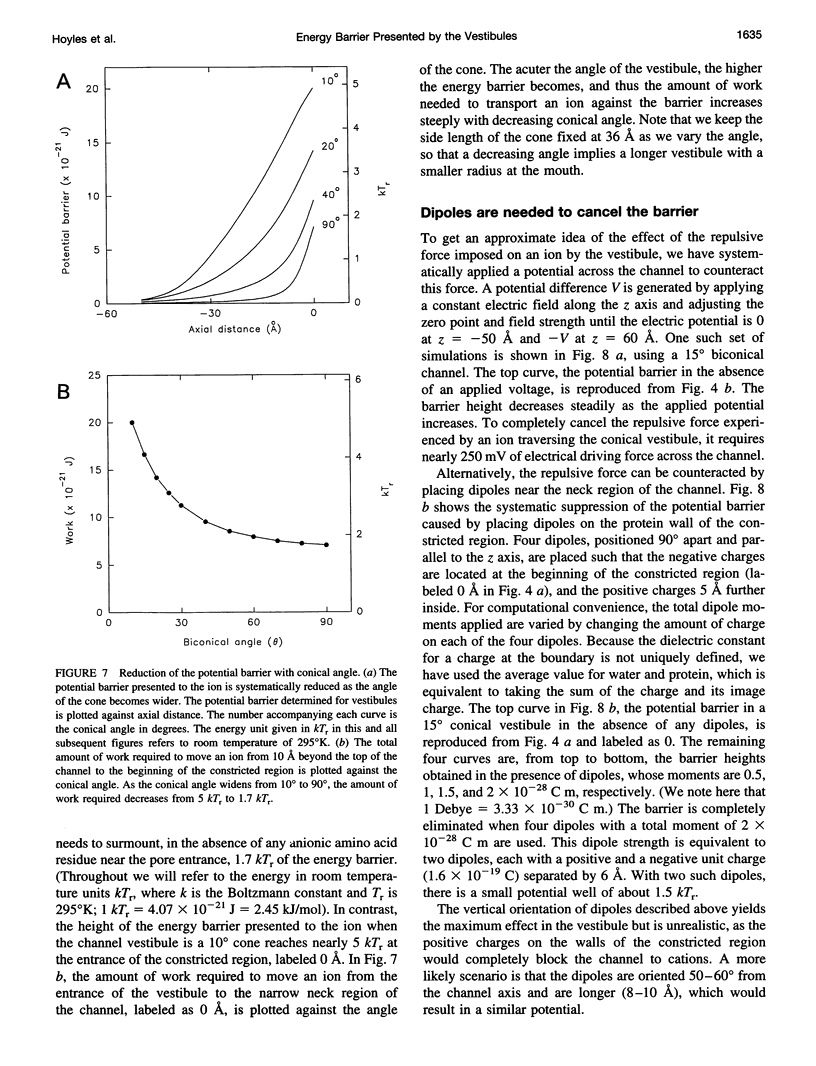
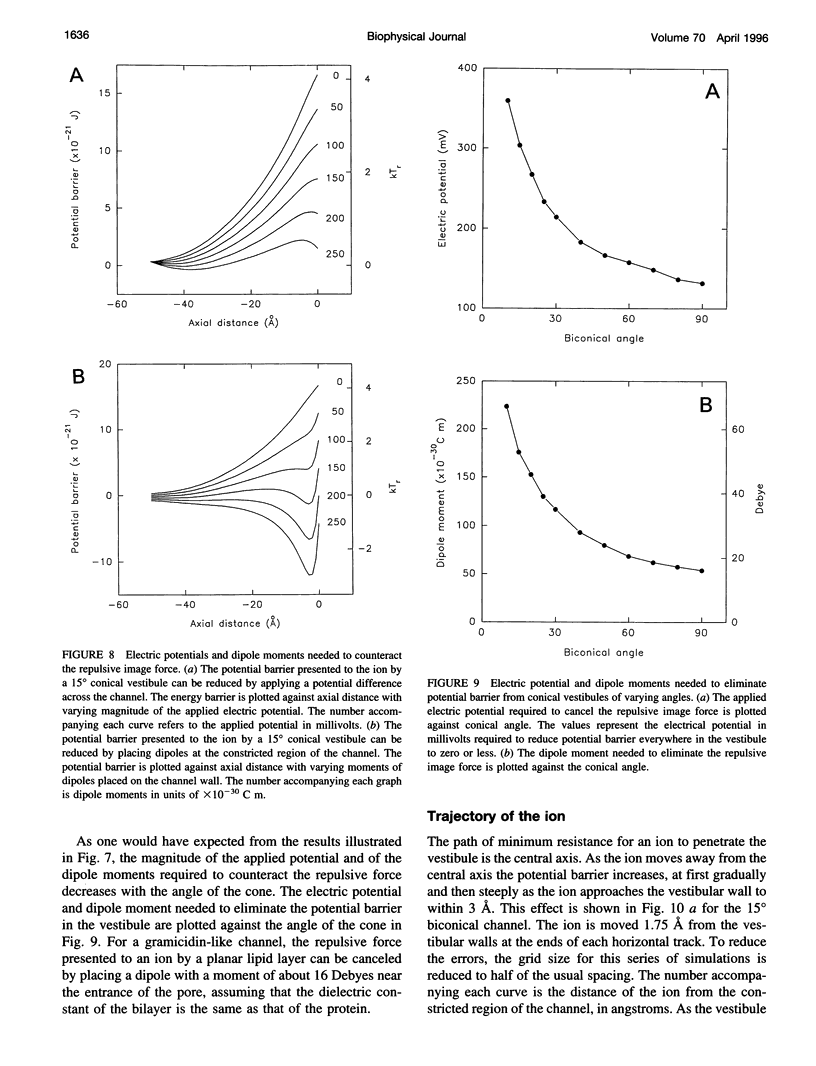
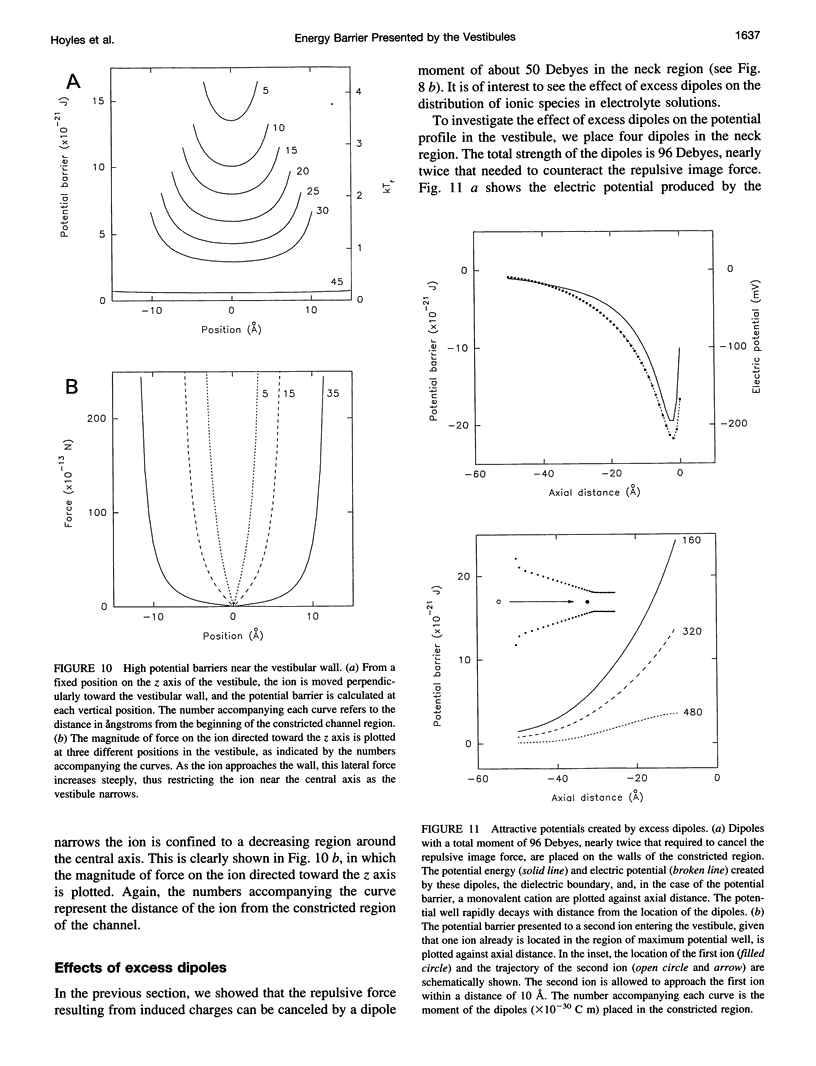
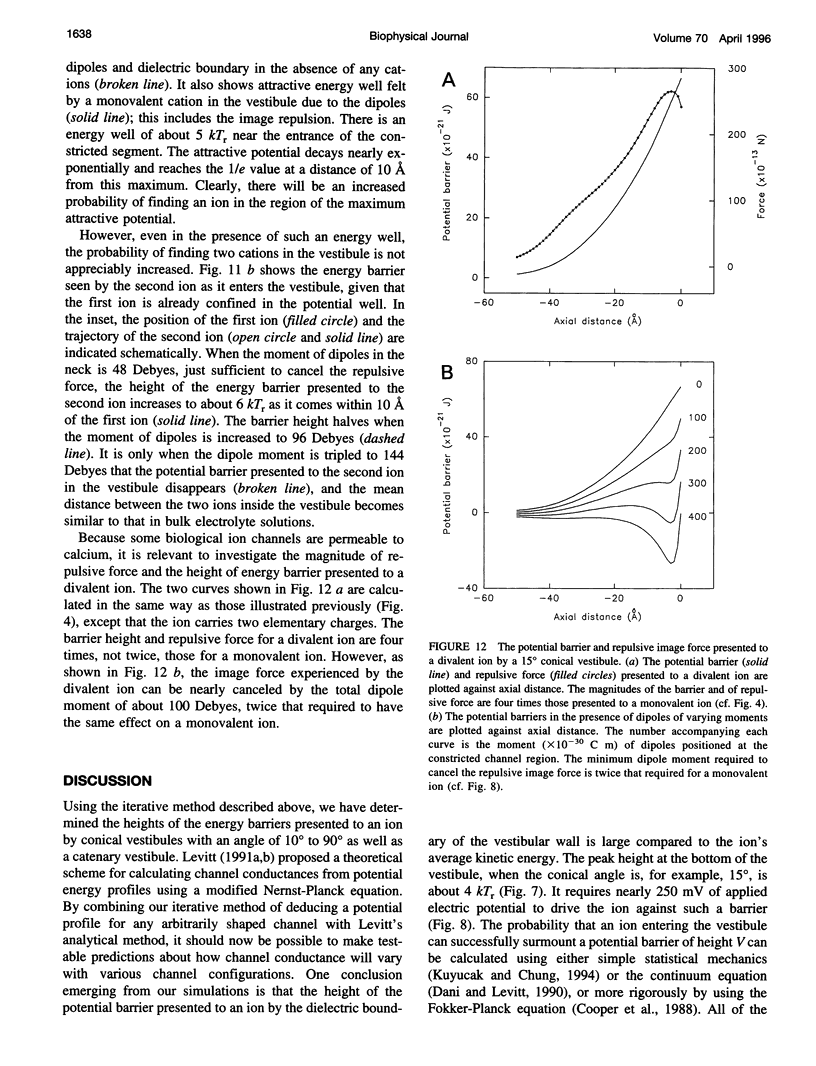
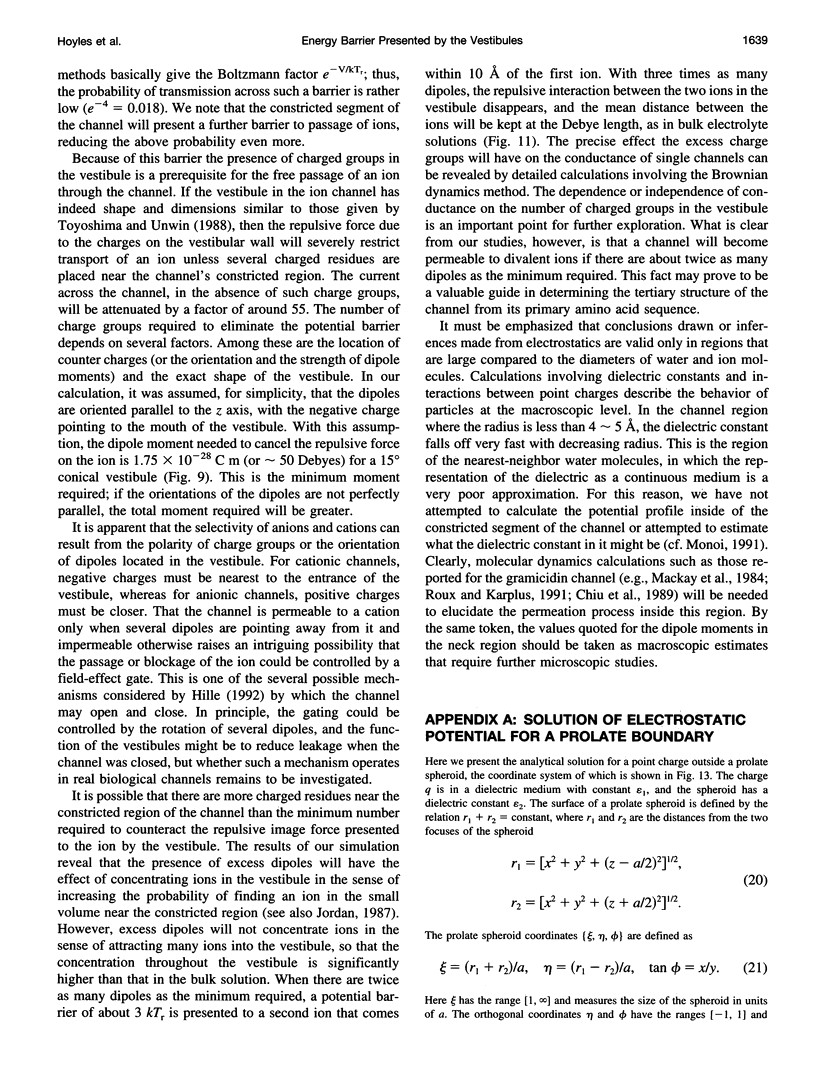
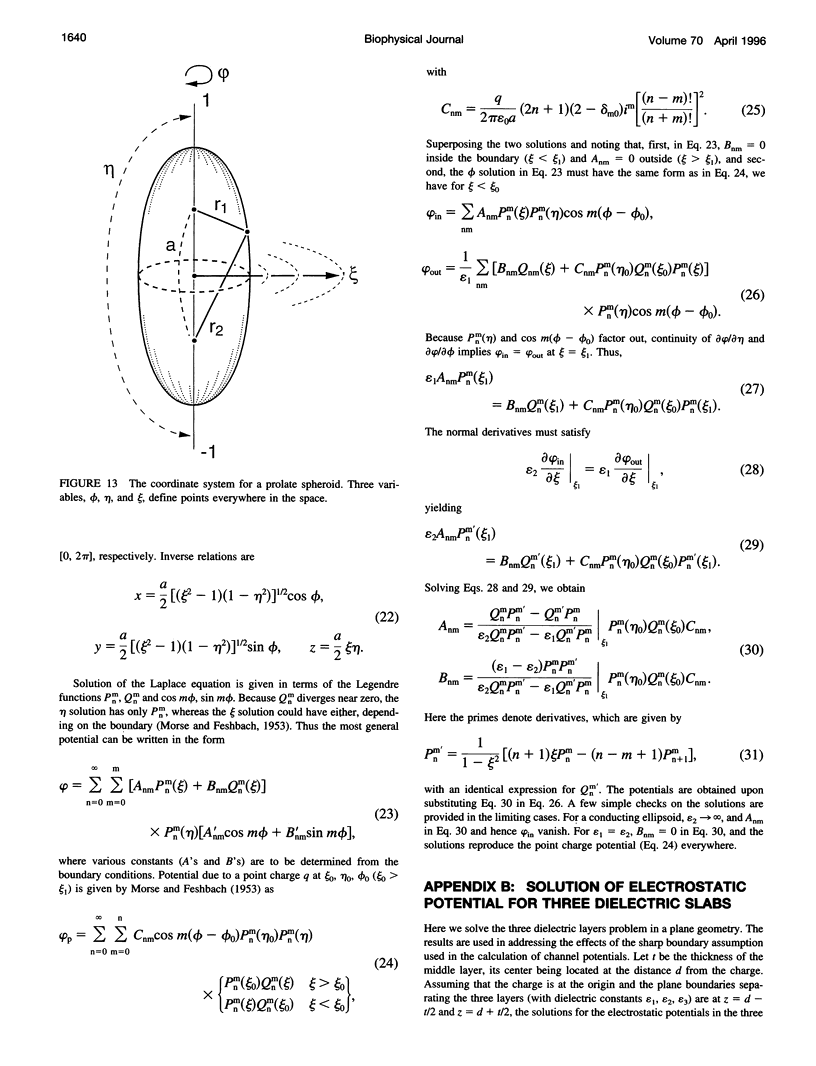
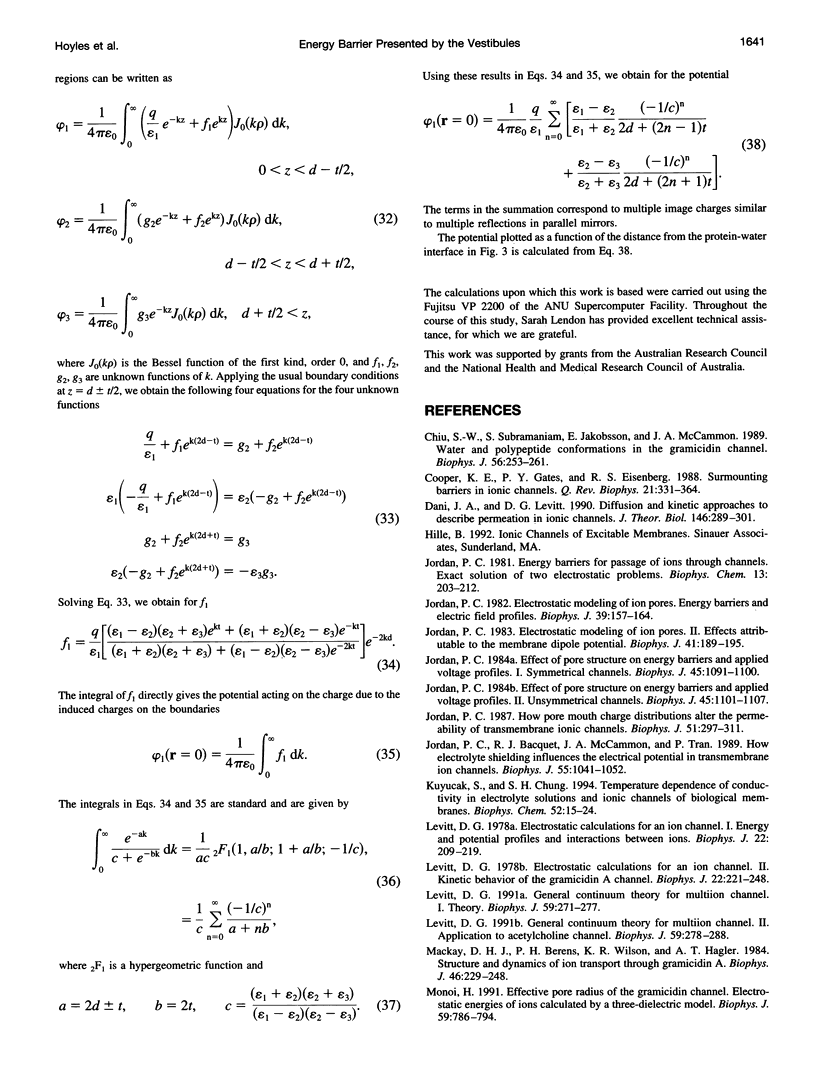
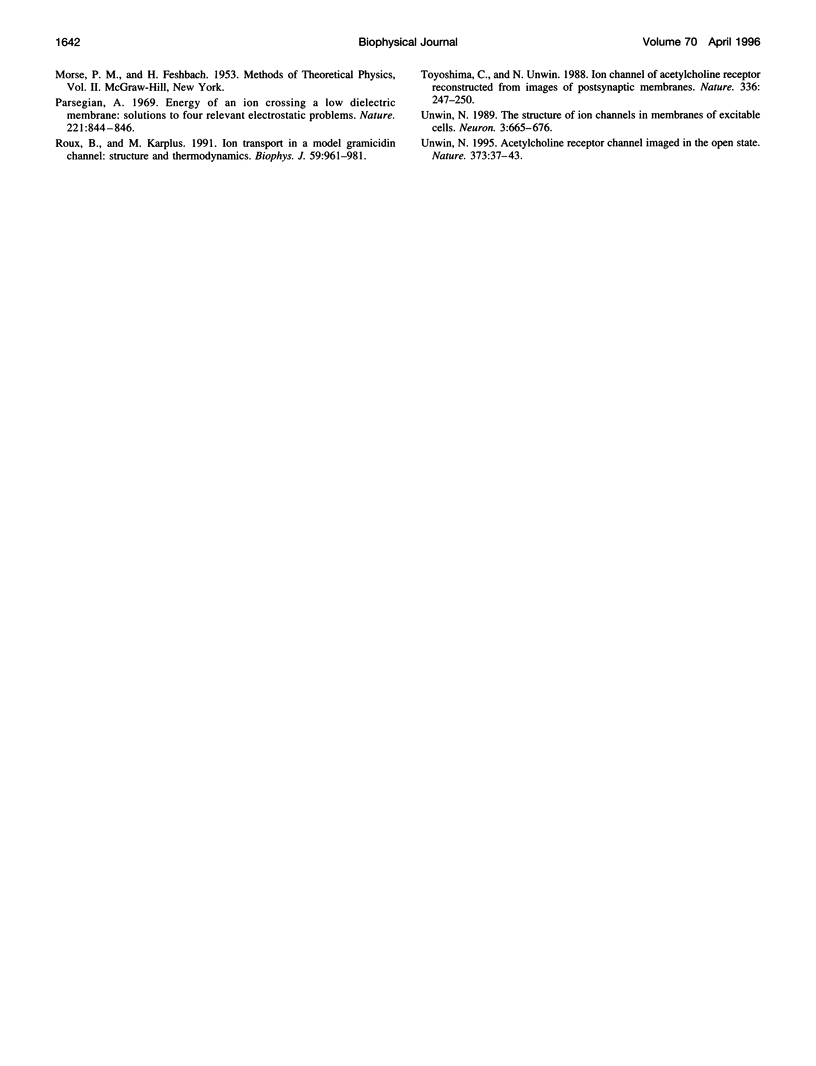
Images in this article
Selected References
These references are in PubMed. This may not be the complete list of references from this article.
- Chiu S. W., Subramaniam S., Jakobsson E., McCammon J. A. Water and polypeptide conformations in the gramicidin channel. A molecular dynamics study. Biophys J. 1989 Aug;56(2):253–261. doi: 10.1016/S0006-3495(89)82671-2. [DOI] [PMC free article] [PubMed] [Google Scholar]
- Cooper K. E., Gates P. Y., Eisenberg R. S. Surmounting barriers in ionic channels. Q Rev Biophys. 1988 Aug;21(3):331–364. doi: 10.1017/s0033583500004480. [DOI] [PubMed] [Google Scholar]
- Dani J. A., Levitt D. G. Diffusion and kinetic approaches to describe permeation in ionic channels. J Theor Biol. 1990 Oct 7;146(3):289–301. doi: 10.1016/s0022-5193(05)80740-4. [DOI] [PubMed] [Google Scholar]
- Jordan P. C., Bacquet R. J., McCammon J. A., Tran P. How electrolyte shielding influences the electrical potential in transmembrane ion channels. Biophys J. 1989 Jun;55(6):1041–1052. doi: 10.1016/S0006-3495(89)82903-0. [DOI] [PMC free article] [PubMed] [Google Scholar]
- Jordan P. C. Effect of pore structure on energy barriers and applied voltage profiles. I. Symmetrical channels. Biophys J. 1984 Jun;45(6):1091–1100. doi: 10.1016/S0006-3495(84)84257-5. [DOI] [PMC free article] [PubMed] [Google Scholar]
- Jordan P. C. Effect of pore structure on energy barriers and applied voltage profiles. II. Unsymmetrical channels. Biophys J. 1984 Jun;45(6):1101–1107. doi: 10.1016/S0006-3495(84)84258-7. [DOI] [PMC free article] [PubMed] [Google Scholar]
- Jordan P. C. Electrostatic modeling of ion pores. Energy barriers and electric field profiles. Biophys J. 1982 Aug;39(2):157–164. doi: 10.1016/S0006-3495(82)84503-7. [DOI] [PMC free article] [PubMed] [Google Scholar]
- Jordan P. C. Electrostatic modeling of ion pores. II. Effects attributable to the membrane dipole potential. Biophys J. 1983 Feb;41(2):189–195. doi: 10.1016/S0006-3495(83)84419-1. [DOI] [PMC free article] [PubMed] [Google Scholar]
- Jordan P. C. Energy barriers for passage of ions through channels. Exact solution of two electrostatic problems. Biophys Chem. 1981 Jun;13(3):203–212. doi: 10.1016/0301-4622(81)80002-6. [DOI] [PubMed] [Google Scholar]
- Jordan P. C. How pore mouth charge distributions alter the permeability of transmembrane ionic channels. Biophys J. 1987 Feb;51(2):297–311. doi: 10.1016/S0006-3495(87)83336-2. [DOI] [PMC free article] [PubMed] [Google Scholar]
- Kuyucak S., Chung S. H. Temperature dependence of conductivity in electrolyte solutions and ionic channels of biological membranes. Biophys Chem. 1994 Sep;52(1):15–24. doi: 10.1016/0301-4622(94)00034-4. [DOI] [PubMed] [Google Scholar]
- Levitt D. G. Electrostatic calculations for an ion channel. I. Energy and potential profiles and interactions between ions. Biophys J. 1978 May;22(2):209–219. doi: 10.1016/S0006-3495(78)85485-X. [DOI] [PMC free article] [PubMed] [Google Scholar]
- Levitt D. G. Electrostatic calculations for an ion channel. II. Kinetic behavior of the gramicidin A channel. Biophys J. 1978 May;22(2):221–248. doi: 10.1016/S0006-3495(78)85486-1. [DOI] [PMC free article] [PubMed] [Google Scholar]
- Levitt D. G. General continuum theory for multiion channel. I. Theory. Biophys J. 1991 Feb;59(2):271–277. doi: 10.1016/S0006-3495(91)82220-2. [DOI] [PMC free article] [PubMed] [Google Scholar]
- Levitt D. G. General continuum theory for multiion channel. II. Application to acetylcholine channel. Biophys J. 1991 Feb;59(2):278–288. doi: 10.1016/S0006-3495(91)82221-4. [DOI] [PMC free article] [PubMed] [Google Scholar]
- Mackay D. H., Berens P. H., Wilson K. R., Hagler A. T. Structure and dynamics of ion transport through gramicidin A. Biophys J. 1984 Aug;46(2):229–248. doi: 10.1016/S0006-3495(84)84016-3. [DOI] [PMC free article] [PubMed] [Google Scholar]
- Monoi H. Effective pore radius of the gramicidin channel. Electrostatic energies of ions calculated by a three-dielectric model. Biophys J. 1991 Apr;59(4):786–794. doi: 10.1016/S0006-3495(91)82291-3. [DOI] [PMC free article] [PubMed] [Google Scholar]
- Parsegian A. Energy of an ion crossing a low dielectric membrane: solutions to four relevant electrostatic problems. Nature. 1969 Mar 1;221(5183):844–846. doi: 10.1038/221844a0. [DOI] [PubMed] [Google Scholar]
- Roux B., Karplus M. Ion transport in a model gramicidin channel. Structure and thermodynamics. Biophys J. 1991 May;59(5):961–981. doi: 10.1016/S0006-3495(91)82311-6. [DOI] [PMC free article] [PubMed] [Google Scholar]
- Toyoshima C., Unwin N. Ion channel of acetylcholine receptor reconstructed from images of postsynaptic membranes. Nature. 1988 Nov 17;336(6196):247–250. doi: 10.1038/336247a0. [DOI] [PubMed] [Google Scholar]
- Unwin N. Acetylcholine receptor channel imaged in the open state. Nature. 1995 Jan 5;373(6509):37–43. doi: 10.1038/373037a0. [DOI] [PubMed] [Google Scholar]
- Unwin N. The structure of ion channels in membranes of excitable cells. Neuron. 1989 Dec;3(6):665–676. doi: 10.1016/0896-6273(89)90235-3. [DOI] [PubMed] [Google Scholar]