Abstract
Activity of the Na+/glucose cotransporter endogenously expressed in LLC-PK1 cells was measured using whole cell recording techniques under three different sodium concentration conditions: 1) externally saturating, zero trans; 2) 40 mM external, zero trans; and 3) externally saturating, 50 mM trans. Activity of the transporter with increasing concentrations of sugar was measured for each set of conditions, from which the maximal current for saturating sugar, Im, was determined. The Im measured shows substantial potential dependence for each set of conditions. The absolute Im and the relative potential dependence of Im compared among the various solute conditions were used to identify which loci in the transport cycle are responsible for potential-dependent changes in function. The experimental data were compared with the predicted Im values calculated from an eight-state, sequential, reversible model of a transport reaction kinetic scheme. Predictions derived from assignment of rate limitation and/or potential dependence to each of the 16 transitions in the transport pathway were derived and compared with the measured data. Most putative models were dismissed because of lack of agreement with the measured data, indicating that several steps along the transport pathway are not rate limiting and/or not potential dependent. Only two models were found that can completely account for the measured data. In one case, translocation of the free carrier must be rate limiting, and both extracellular sodium-binding events as well as translocation of both free and fully loaded carrier forms must be potential-dependent transitions. In the second case, translocation of the free carrier and dissociation of the first sodium to be released intracellularly must be equivalently rate limiting. In this case only the two translocation events are required to be potential dependent. The two external sodium-binding events might still be potential dependent, but this is not required to fit the data. Previous reports suggest that the first model is correct; however, no direct experimental data compel us to dismiss the second option as a feasible model.
Full text
PDF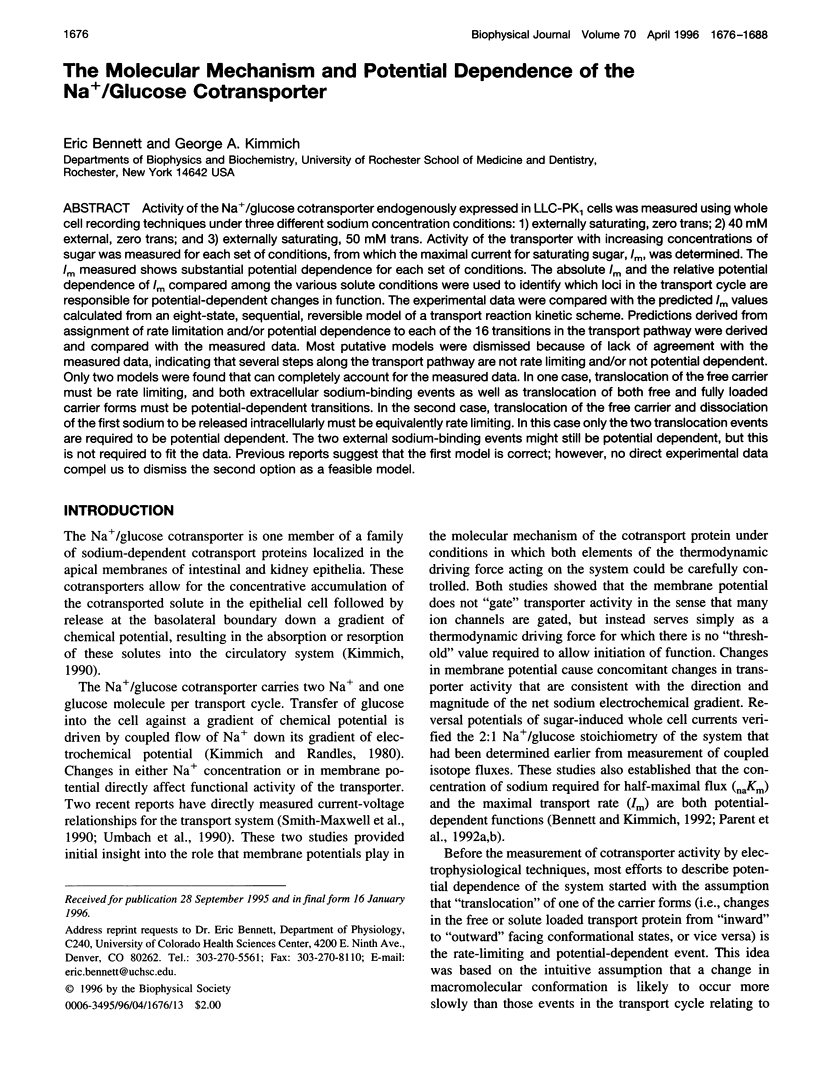
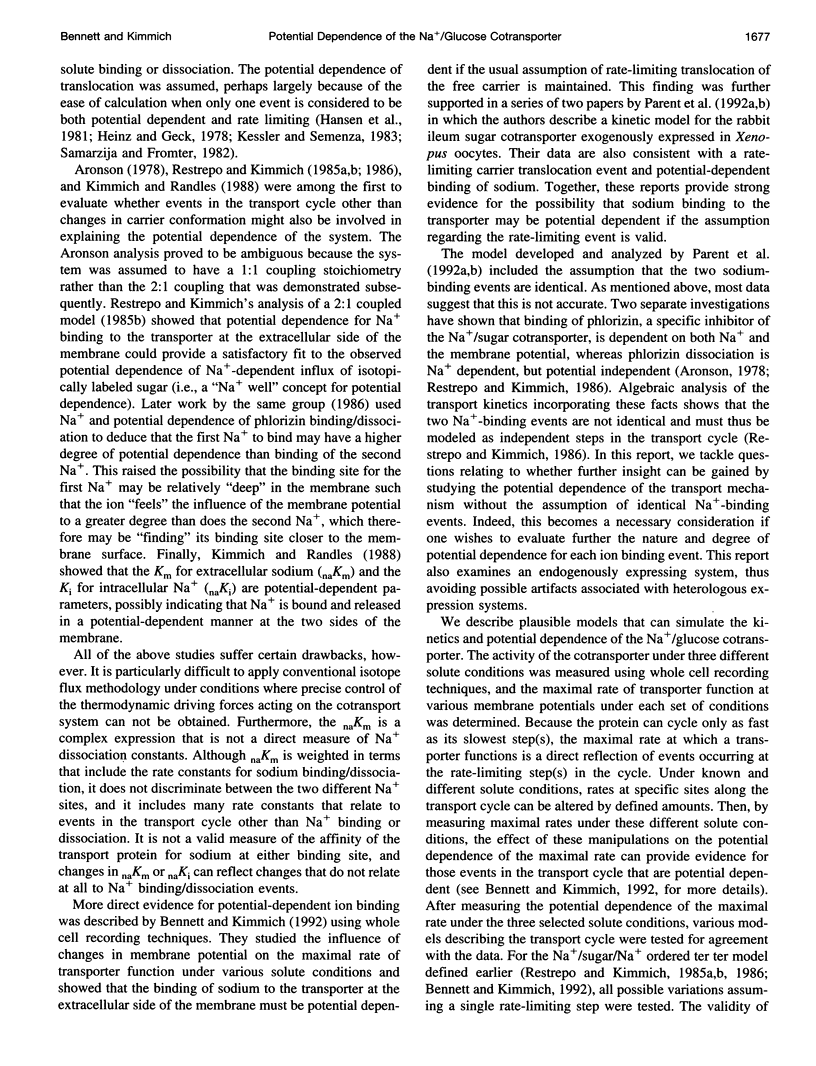
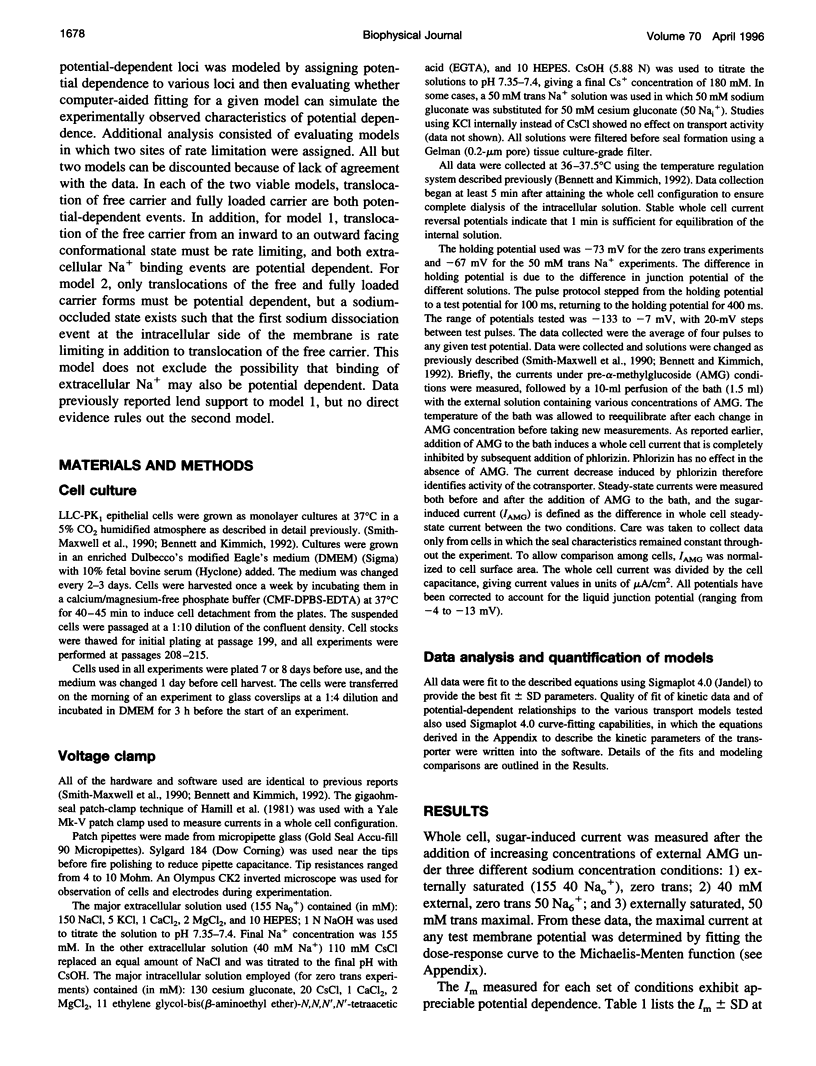
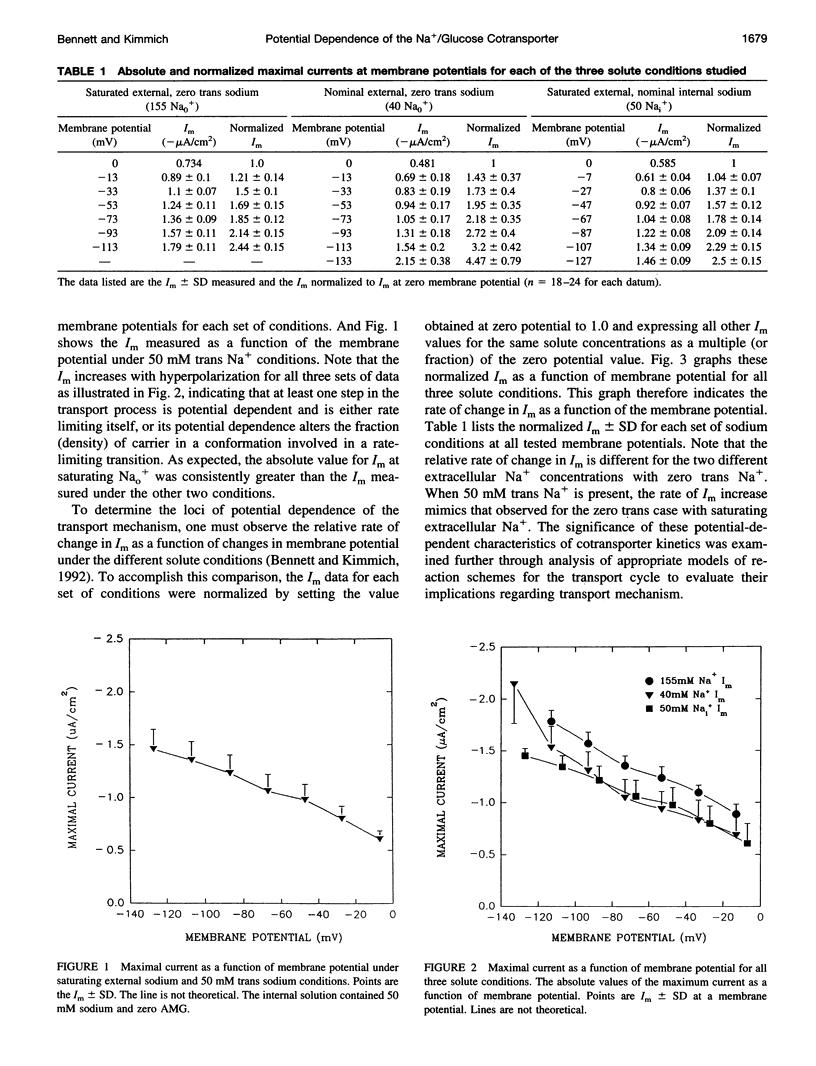
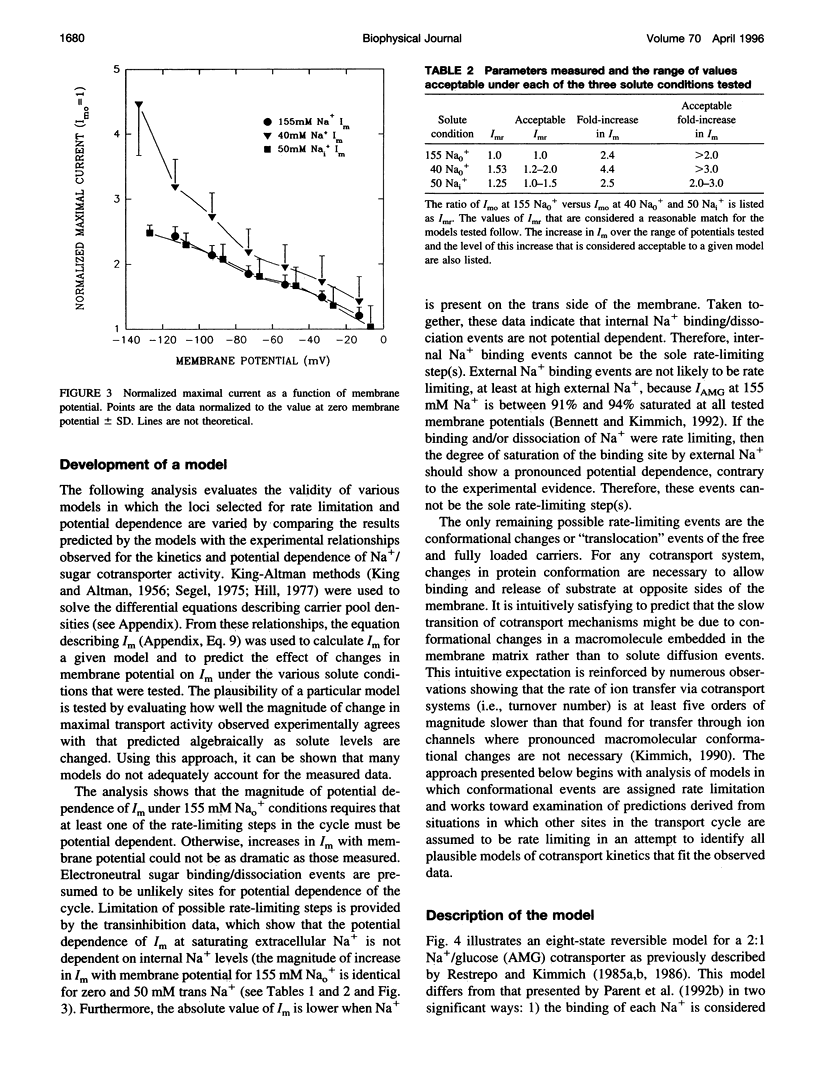
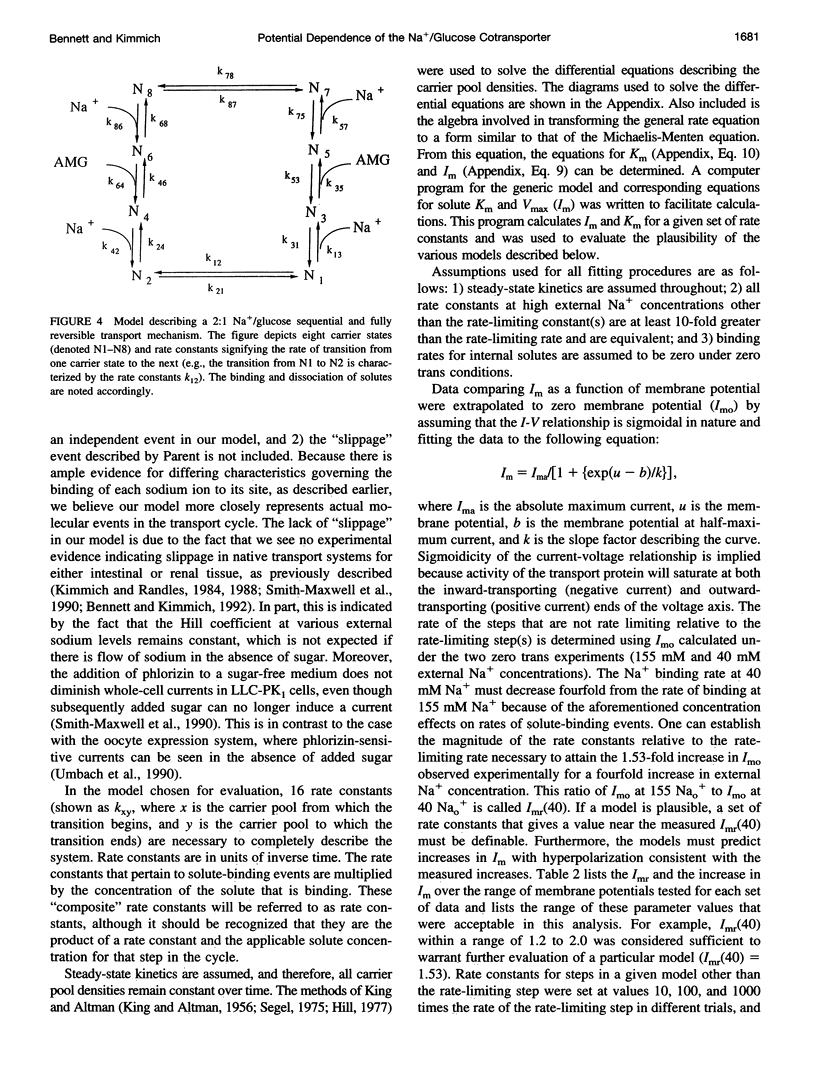
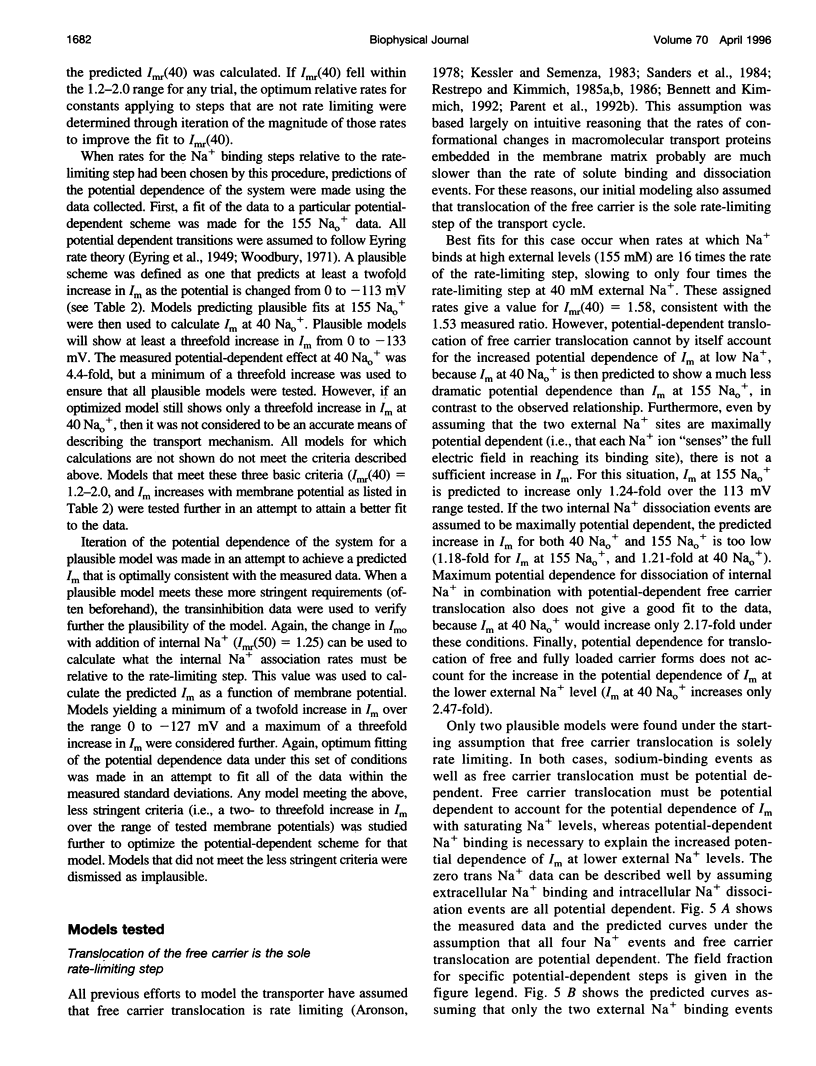
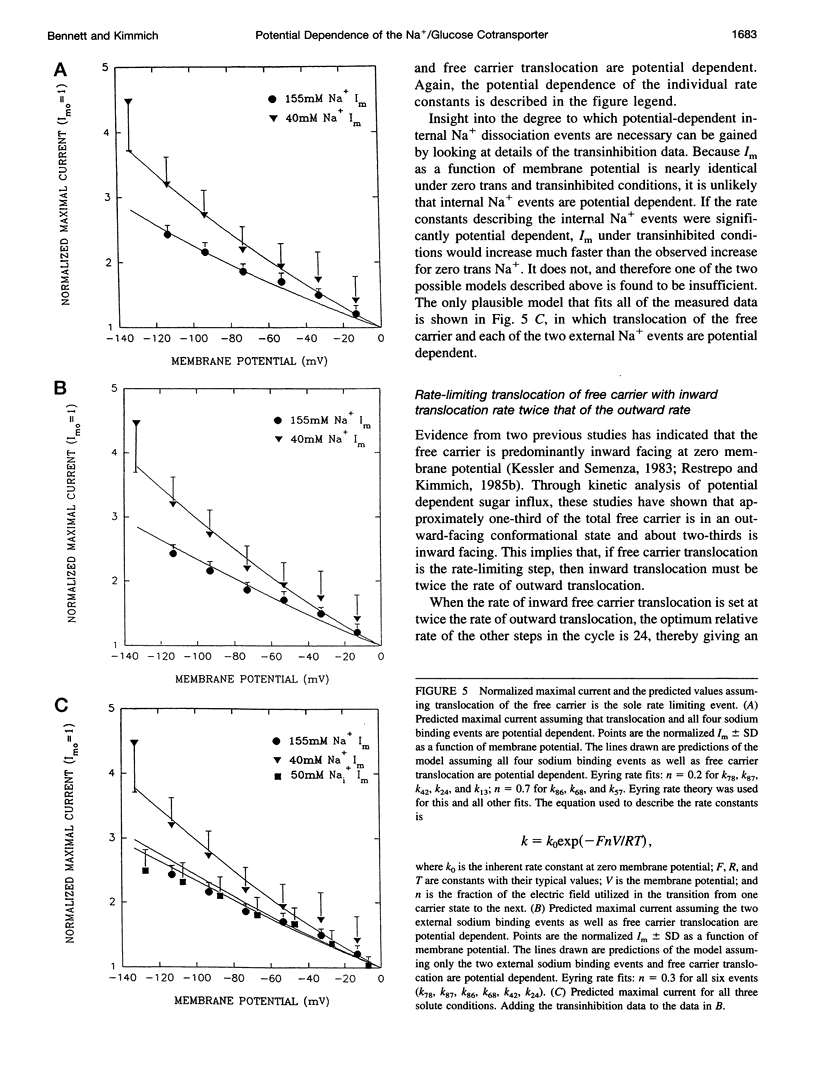
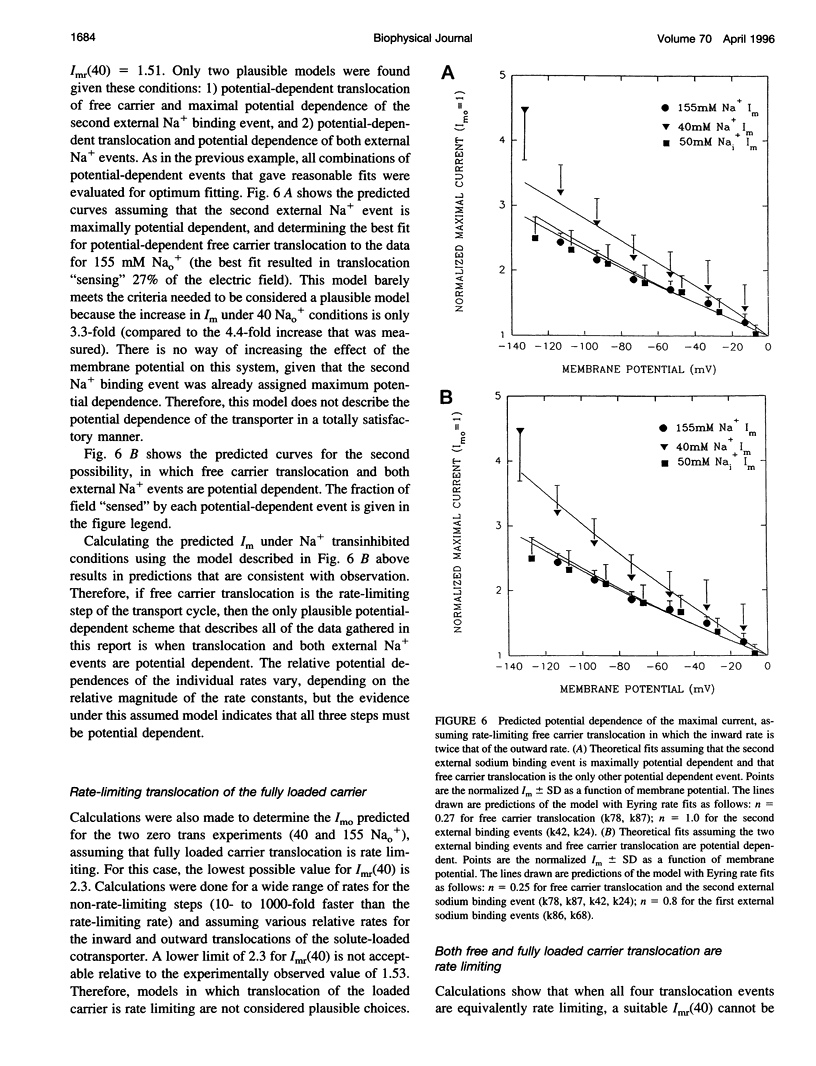
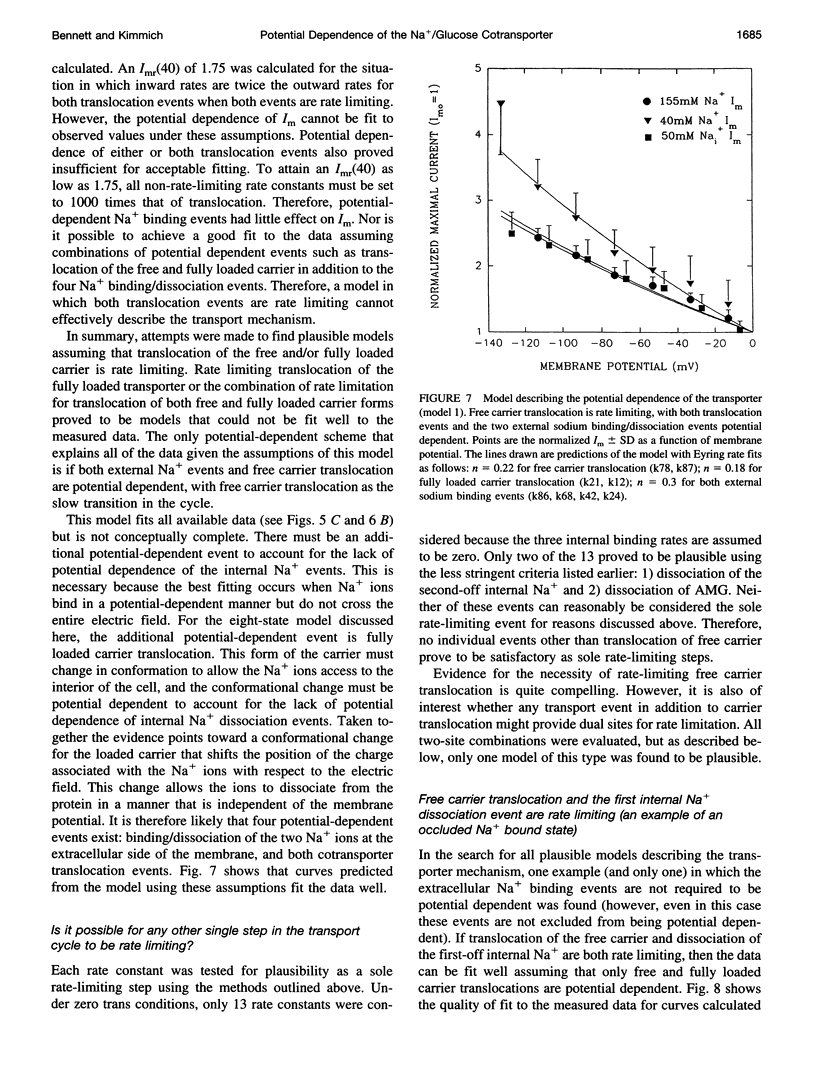
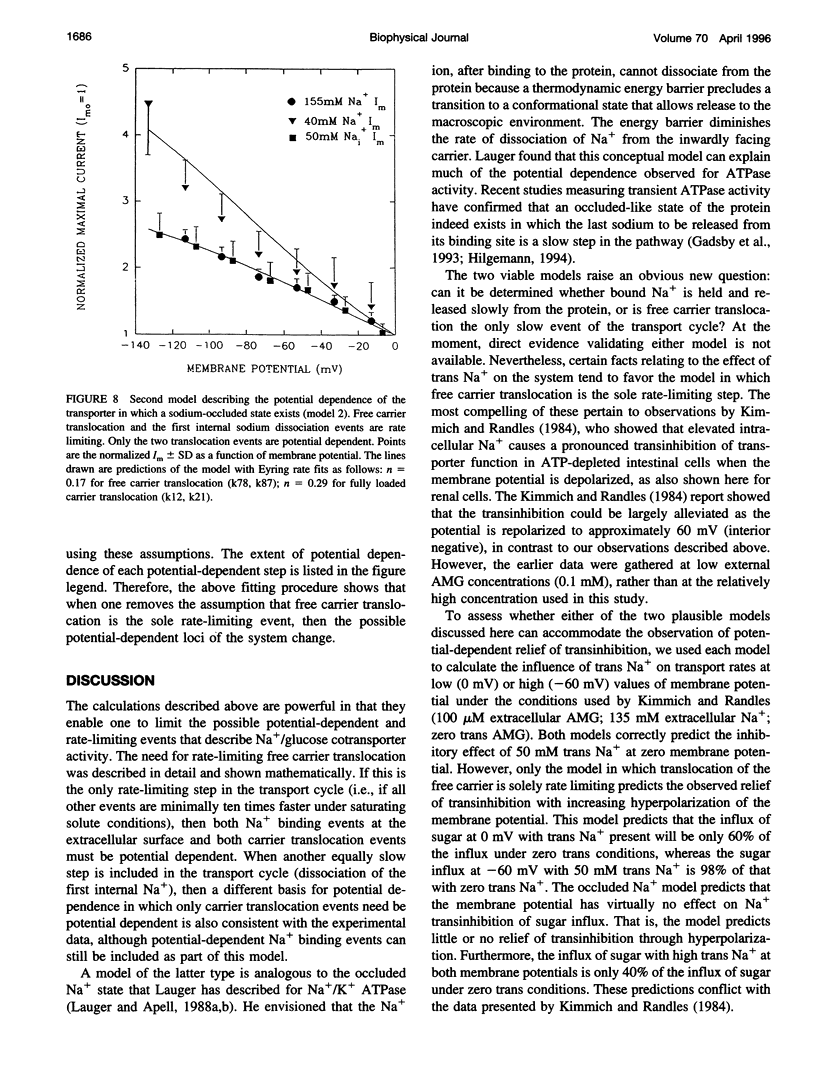
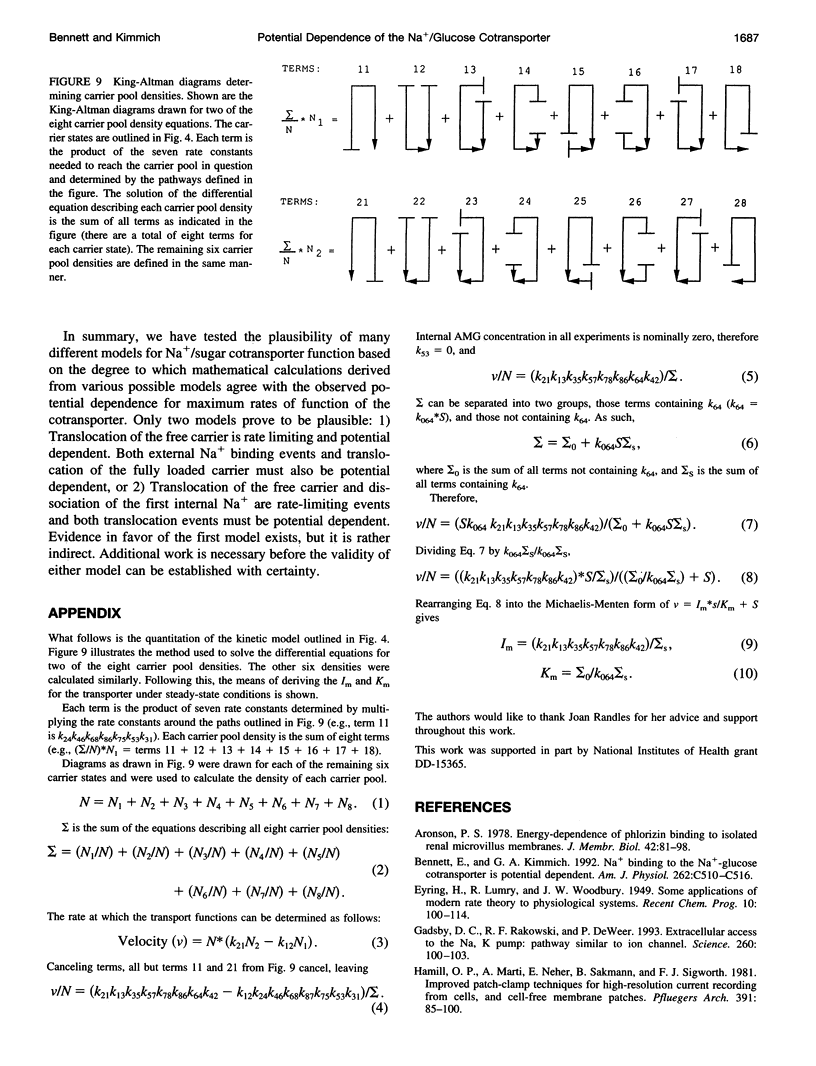
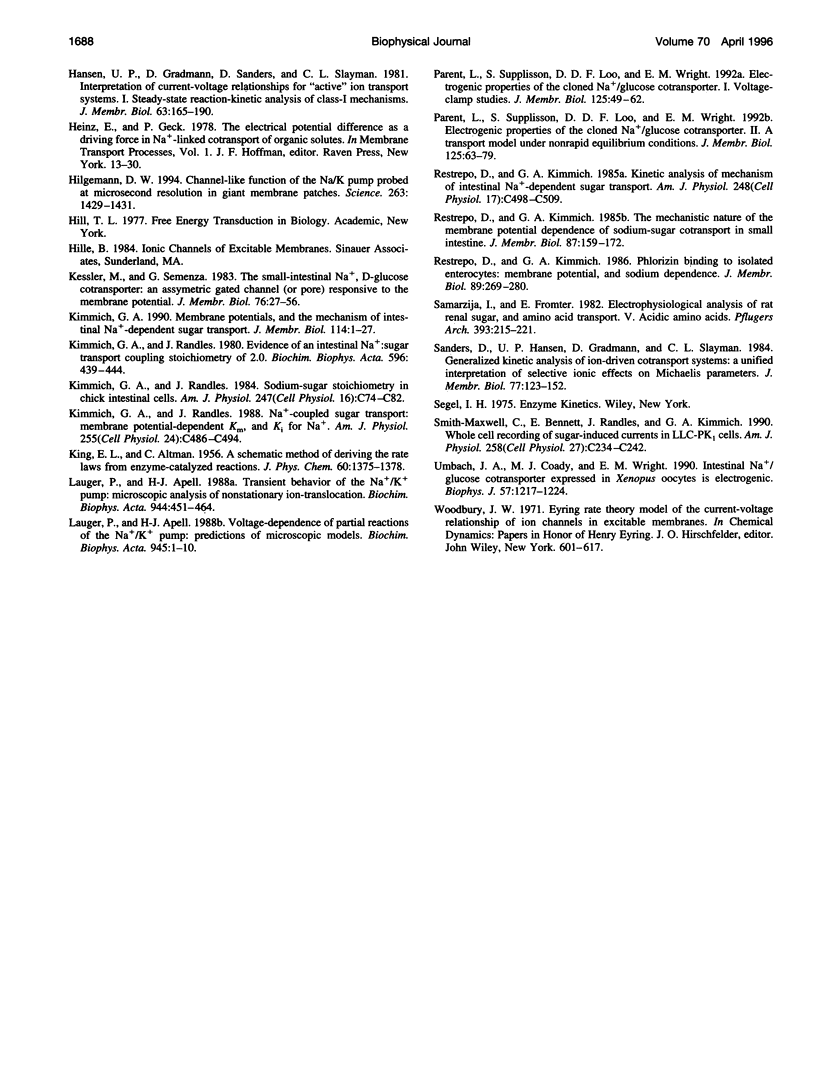
Selected References
These references are in PubMed. This may not be the complete list of references from this article.
- Aronson P. S. Energy-dependence of phlorizin binding to isolated renal microvillus membranes. Evidence concerning the mechanism of coupling between the electrochemical Na+ gradient the sugar transport. J Membr Biol. 1978 Jul 21;42(1):81–98. doi: 10.1007/BF01870395. [DOI] [PubMed] [Google Scholar]
- Bennett E., Kimmich G. A. Na+ binding to the Na(+)-glucose cotransporter is potential dependent. Am J Physiol. 1992 Feb;262(2 Pt 1):C510–C516. doi: 10.1152/ajpcell.1992.262.2.C510. [DOI] [PubMed] [Google Scholar]
- Gadsby D. C., Rakowski R. F., De Weer P. Extracellular access to the Na,K pump: pathway similar to ion channel. Science. 1993 Apr 2;260(5104):100–103. doi: 10.1126/science.7682009. [DOI] [PubMed] [Google Scholar]
- Hamill O. P., Marty A., Neher E., Sakmann B., Sigworth F. J. Improved patch-clamp techniques for high-resolution current recording from cells and cell-free membrane patches. Pflugers Arch. 1981 Aug;391(2):85–100. doi: 10.1007/BF00656997. [DOI] [PubMed] [Google Scholar]
- Hansen U. P., Gradmann D., Sanders D., Slayman C. L. Interpretation of current-voltage relationships for "active" ion transport systems: I. Steady-state reaction-kinetic analysis of class-I mechanisms. J Membr Biol. 1981;63(3):165–190. doi: 10.1007/BF01870979. [DOI] [PubMed] [Google Scholar]
- Hilgemann D. W. Channel-like function of the Na,K pump probed at microsecond resolution in giant membrane patches. Science. 1994 Mar 11;263(5152):1429–1432. doi: 10.1126/science.8128223. [DOI] [PubMed] [Google Scholar]
- Kessler M., Semenza G. The small-intestinal Na+, D-glucose cotransporter: an asymmetric gated channel (or pore) responsive to delta psi. J Membr Biol. 1983;76(1):27–56. doi: 10.1007/BF01871452. [DOI] [PubMed] [Google Scholar]
- Kimmich G. A. Membrane potentials and the mechanism of intestinal Na(+)-dependent sugar transport. J Membr Biol. 1990 Mar;114(1):1–27. doi: 10.1007/BF01869381. [DOI] [PubMed] [Google Scholar]
- Kimmich G. A., Randles J. Evidence for an intestinal Na+:sugar transport coupling stoichiometry of 2.0. Biochim Biophys Acta. 1980 Mar 13;596(3):439–444. doi: 10.1016/0005-2736(80)90131-5. [DOI] [PubMed] [Google Scholar]
- Kimmich G. A., Randles J. Na+-coupled sugar transport: membrane potential-dependent Km and Ki for Na+. Am J Physiol. 1988 Oct;255(4 Pt 1):C486–C494. doi: 10.1152/ajpcell.1988.255.4.C486. [DOI] [PubMed] [Google Scholar]
- Kimmich G. A., Randles J. Sodium-sugar coupling stoichiometry in chick intestinal cells. Am J Physiol. 1984 Jul;247(1 Pt 1):C74–C82. doi: 10.1152/ajpcell.1984.247.1.C74. [DOI] [PubMed] [Google Scholar]
- Läuger P., Apell H. J. Transient behaviour of the Na+/K+-pump: microscopic analysis of nonstationary ion-translocation. Biochim Biophys Acta. 1988 Oct 20;944(3):451–464. doi: 10.1016/0005-2736(88)90516-0. [DOI] [PubMed] [Google Scholar]
- Läuger P., Apell H. J. Voltage dependence of partial reactions of the Na+/K+ pump: predictions from microscopic models. Biochim Biophys Acta. 1988 Nov 3;945(1):1–10. doi: 10.1016/0005-2736(88)90355-0. [DOI] [PubMed] [Google Scholar]
- Parent L., Supplisson S., Loo D. D., Wright E. M. Electrogenic properties of the cloned Na+/glucose cotransporter: I. Voltage-clamp studies. J Membr Biol. 1992 Jan;125(1):49–62. doi: 10.1007/BF00235797. [DOI] [PubMed] [Google Scholar]
- Parent L., Supplisson S., Loo D. D., Wright E. M. Electrogenic properties of the cloned Na+/glucose cotransporter: II. A transport model under nonrapid equilibrium conditions. J Membr Biol. 1992 Jan;125(1):63–79. doi: 10.1007/BF00235798. [DOI] [PubMed] [Google Scholar]
- Restrepo D., Kimmich G. A. Kinetic analysis of mechanism of intestinal Na+-dependent sugar transport. Am J Physiol. 1985 May;248(5 Pt 1):C498–C509. doi: 10.1152/ajpcell.1985.248.5.C498. [DOI] [PubMed] [Google Scholar]
- Restrepo D., Kimmich G. A. Phlorizin binding to isolated enterocytes: membrane potential and sodium dependence. J Membr Biol. 1986;89(3):269–280. doi: 10.1007/BF01870669. [DOI] [PubMed] [Google Scholar]
- Restrepo D., Kimmich G. A. The mechanistic nature of the membrane potential dependence of sodium-sugar cotransport in small intestine. J Membr Biol. 1985;87(2):159–172. doi: 10.1007/BF01870662. [DOI] [PubMed] [Google Scholar]
- Samarzija I., Frömter E. Electrophysiological analysis of rat renal sugar and amino acid transport. V. Acidic amino acids. Pflugers Arch. 1982 May;393(3):215–221. doi: 10.1007/BF00584072. [DOI] [PubMed] [Google Scholar]
- Sanders D., Hansen U. P., Gradmann D., Slayman C. L. Generalized kinetic analysis of ion-driven cotransport systems: a unified interpretation of selective ionic effects on Michaelis parameters. J Membr Biol. 1984;77(2):123–152. doi: 10.1007/BF01925862. [DOI] [PubMed] [Google Scholar]
- Smith-Maxwell C., Bennett E., Randles J., Kimmich G. A. Whole cell recording of sugar-induced currents in LLC-PK1 cells. Am J Physiol. 1990 Feb;258(2 Pt 1):C234–C242. doi: 10.1152/ajpcell.1990.258.2.C234. [DOI] [PubMed] [Google Scholar]
- Umbach J. A., Coady M. J., Wright E. M. Intestinal Na+/glucose cotransporter expressed in Xenopus oocytes is electrogenic. Biophys J. 1990 Jun;57(6):1217–1224. doi: 10.1016/S0006-3495(90)82640-0. [DOI] [PMC free article] [PubMed] [Google Scholar]