Abstract
The simplest model for a neutrophil in its "passive" state views the cell as consisting of a liquid-like cytoplasmic region surrounded by a membrane. The cell surface is in a state of isotropic contraction, which causes the cell to assume a spherical shape. This contraction is characterized by the cortical tension. The cortical tension shows a weak area dilation dependence, and it determines the elastic properties of the cell for small curvature deformations. At high curvature deformations in small pipets (with internal radii less than 1 micron), the measured critical suction pressure for cell flow into the pipet is larger than its estimate from the law of Laplace. A model is proposed where the region consisting of the cytoplasm membrane and the underlying cortex (having a finite thickness) is introduced at the cell surface. The mechanical properties of this region are characterized by the apparent cortical tension (defined as a free contraction energy per unit area) and the apparent bending modulus (introduced as a bending free energy per unit area) of its middle plane. The model predicts that for small curvature deformations (in pipets having radii larger than 1.2 microns) the role of the cortical thickness and the resistance for bending of the membrane-cortex complex is negligible. For high curvature deformations, they lead to elevated suction pressures above the values predicted from the law of Laplace. The existence of elevated suction pressures for pipets with radii from 1 micron down to 0.24 micron is found experimentally. The measured excess suction pressures cannot be explained only by the modified law of Laplace (for a cortex with finite thickness and negligible bending resistance), because it predicts unacceptable high cortical thicknesses (from 0.3 to 0.7 micron). It is concluded that the membrane-cortex complex has an apparent bending modulus from 1 x 10(-18) to 2 x 10(-18) J for a cortex with a thickness from 0.1 micron down to values much smaller than the radius of the smallest pipet (0.24 micron) used in this study.
Full text
PDF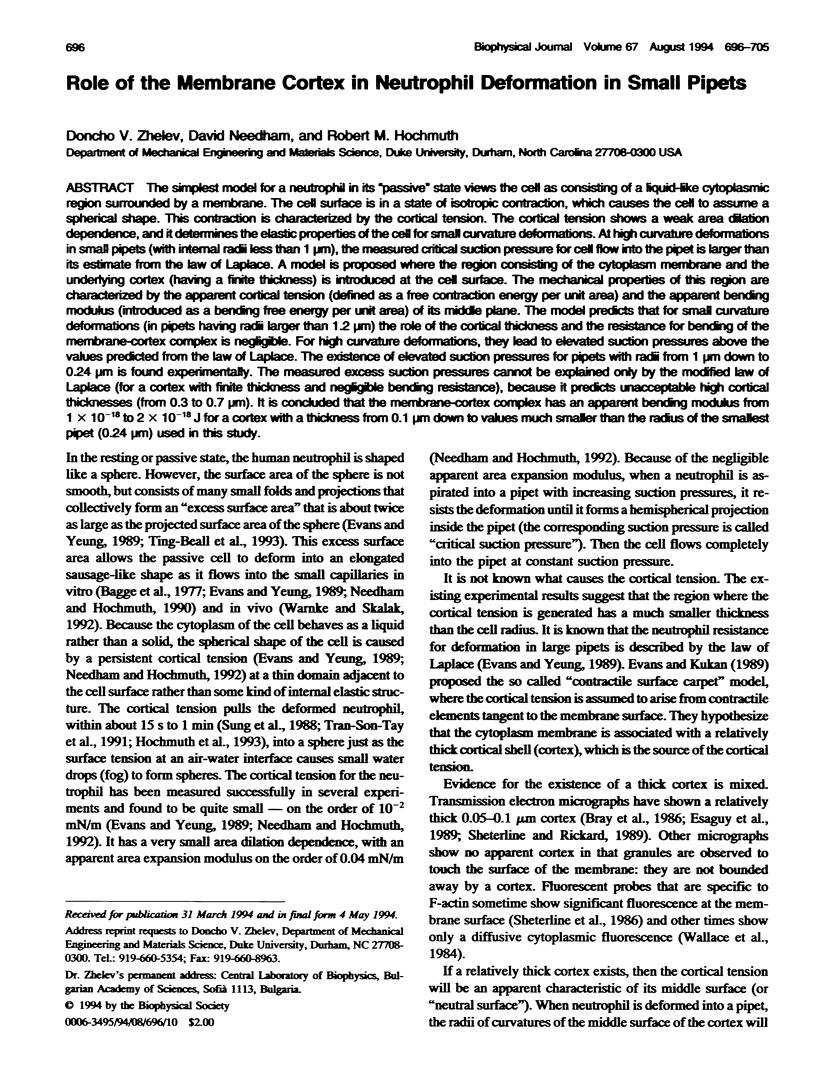
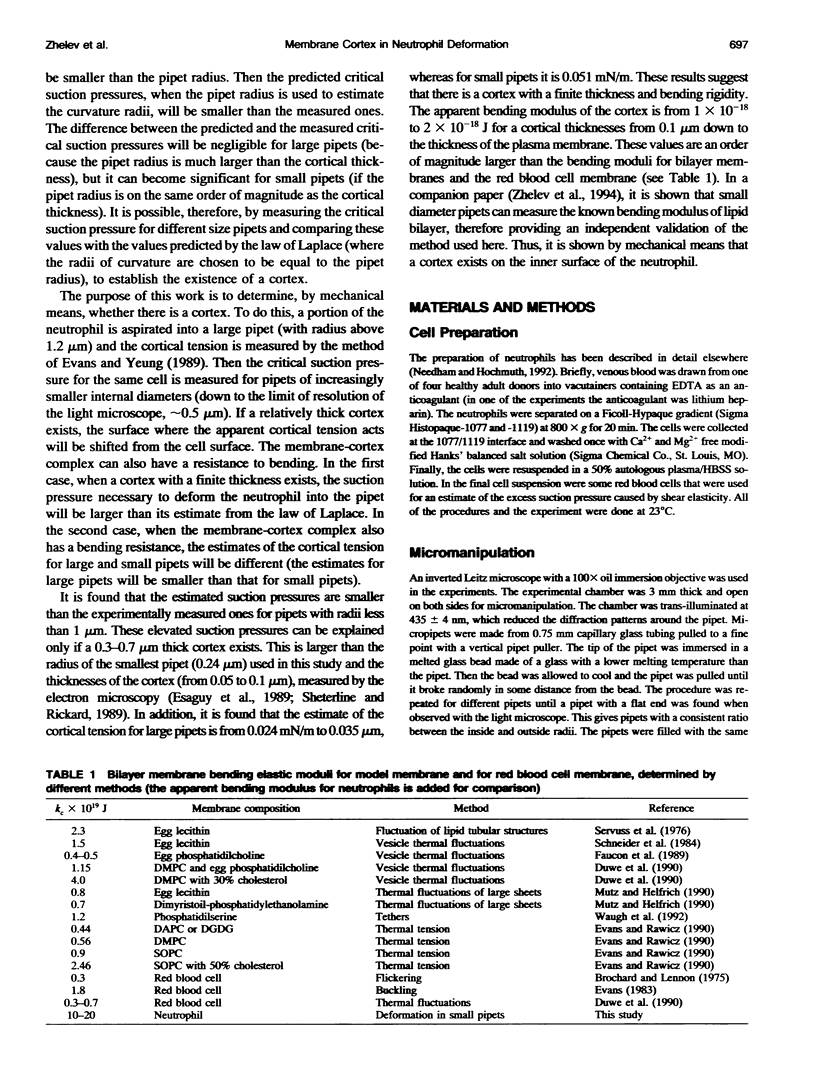
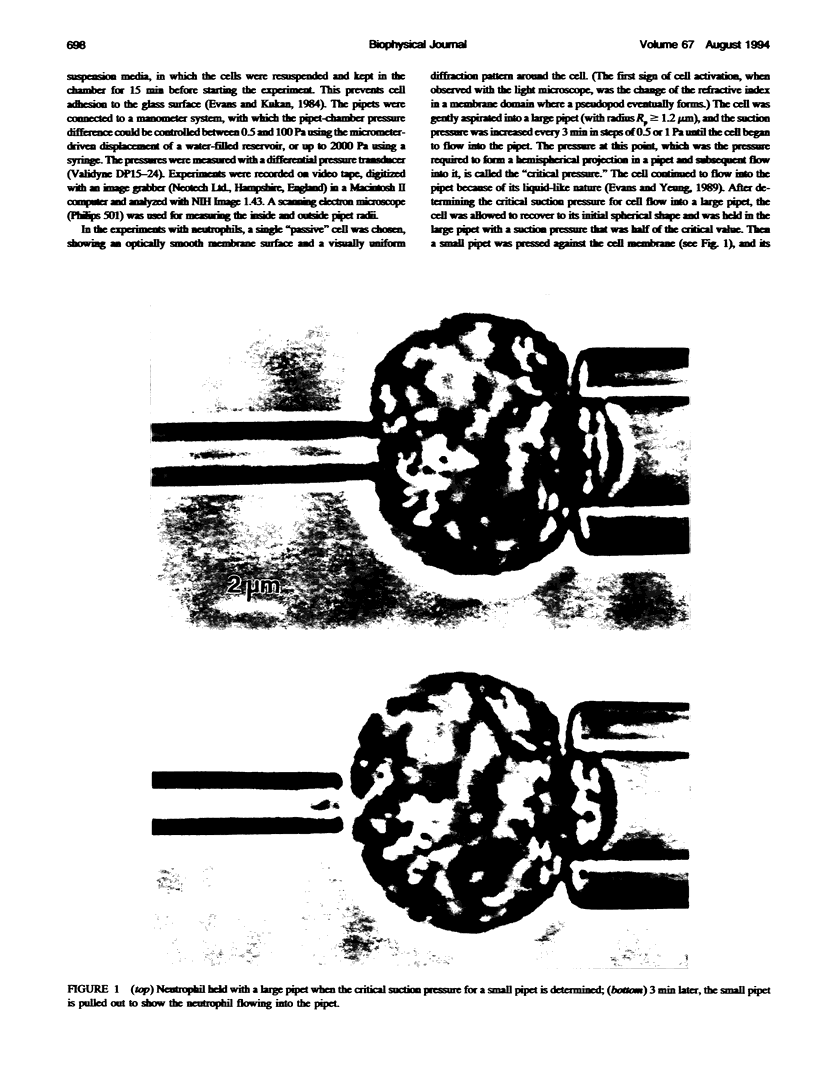
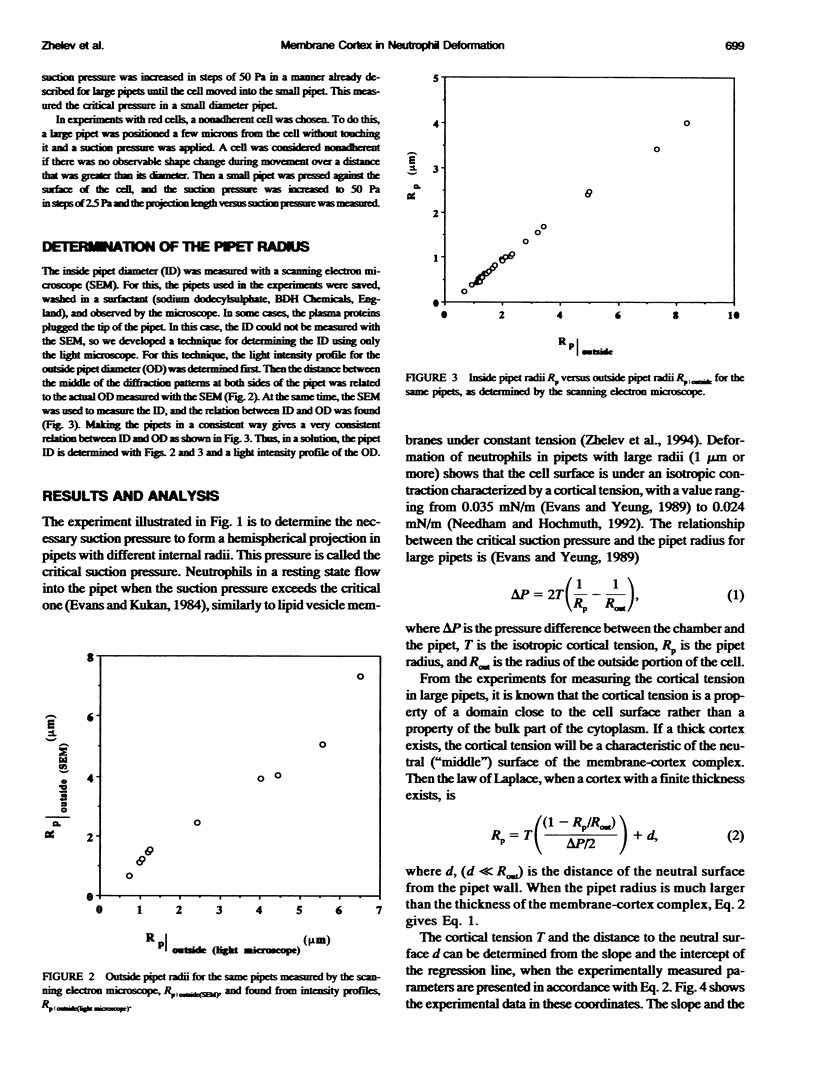
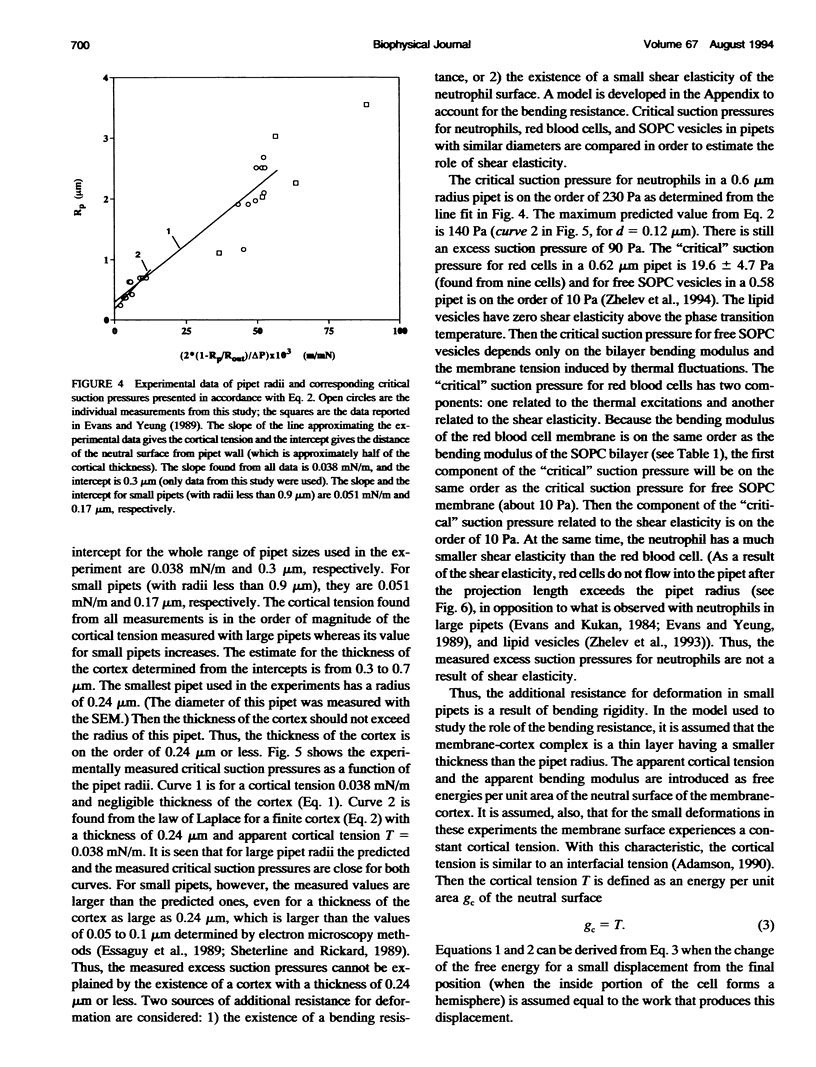
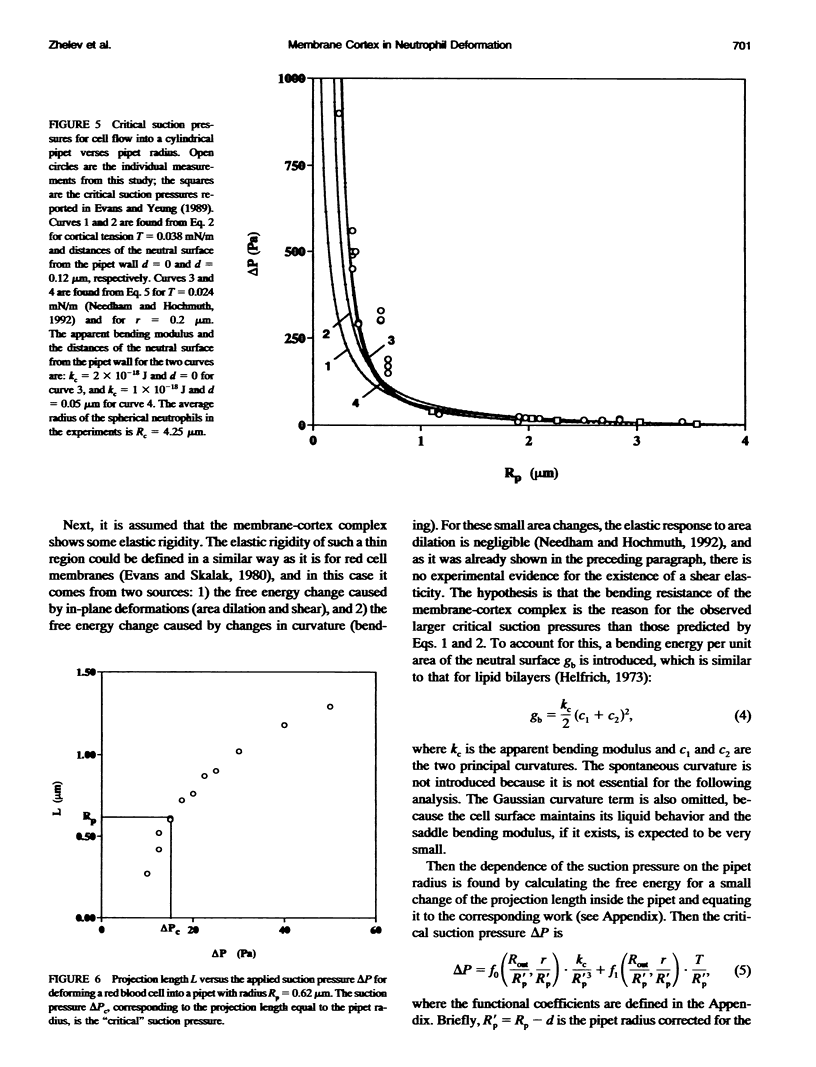
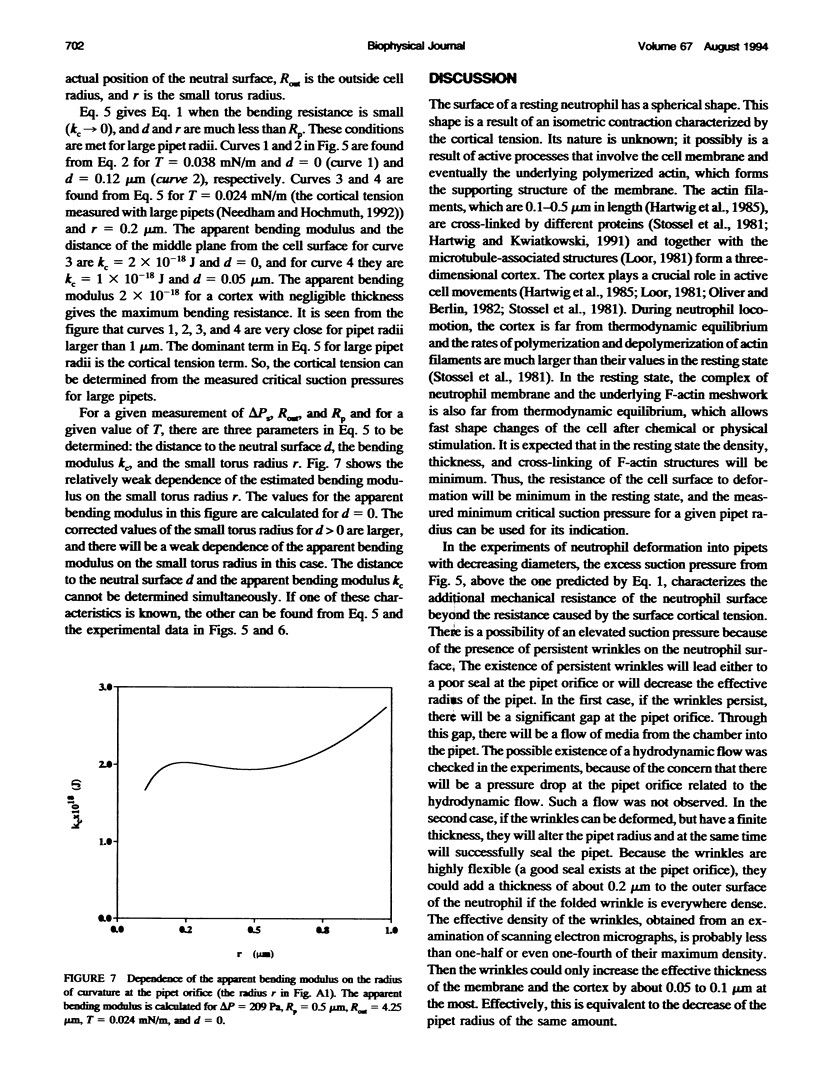
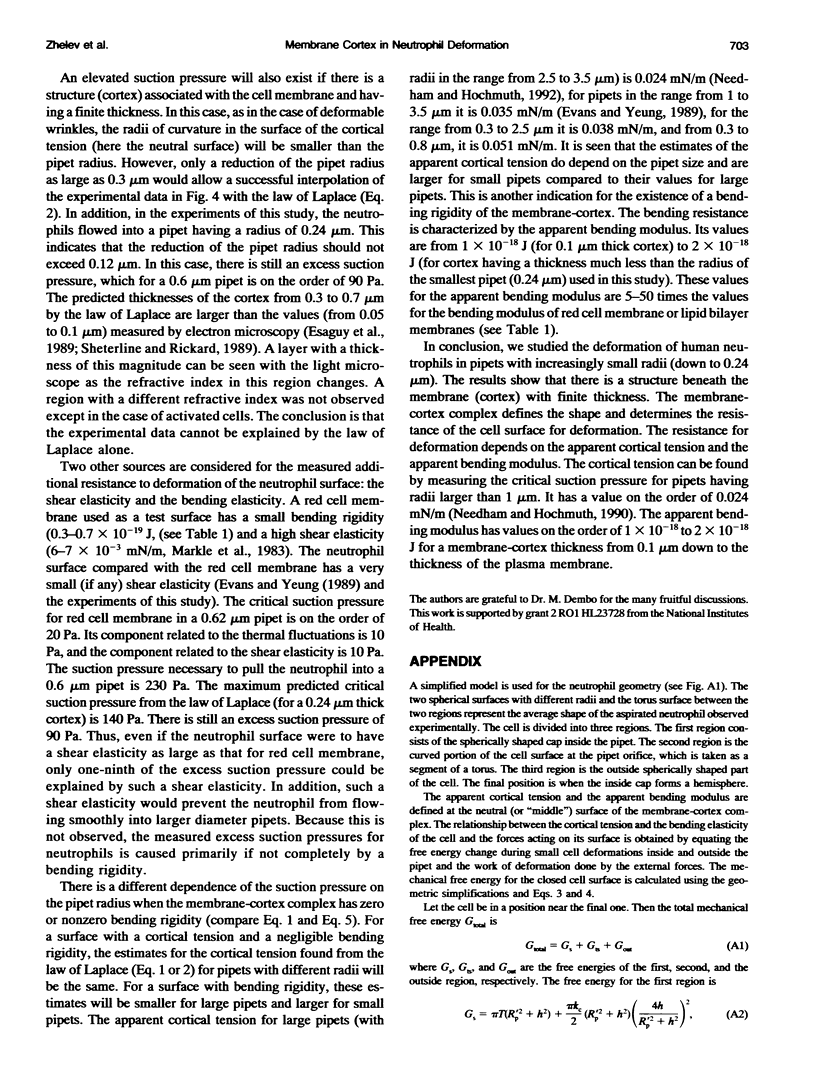
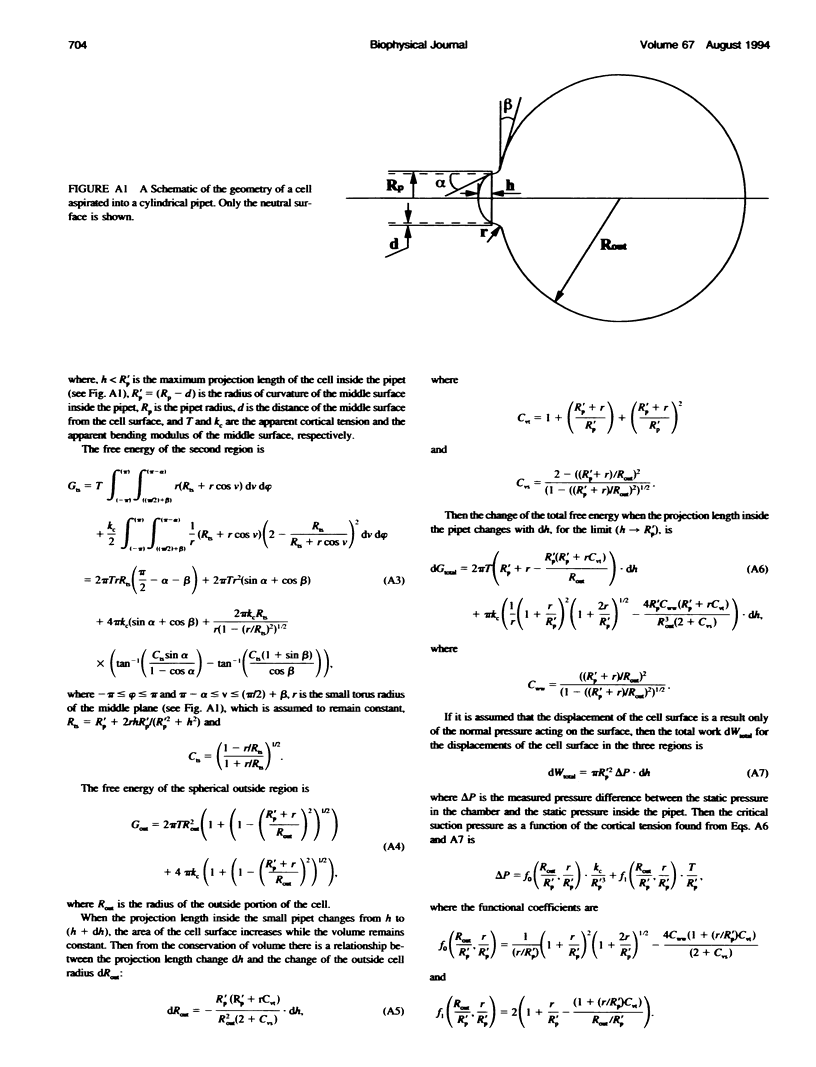
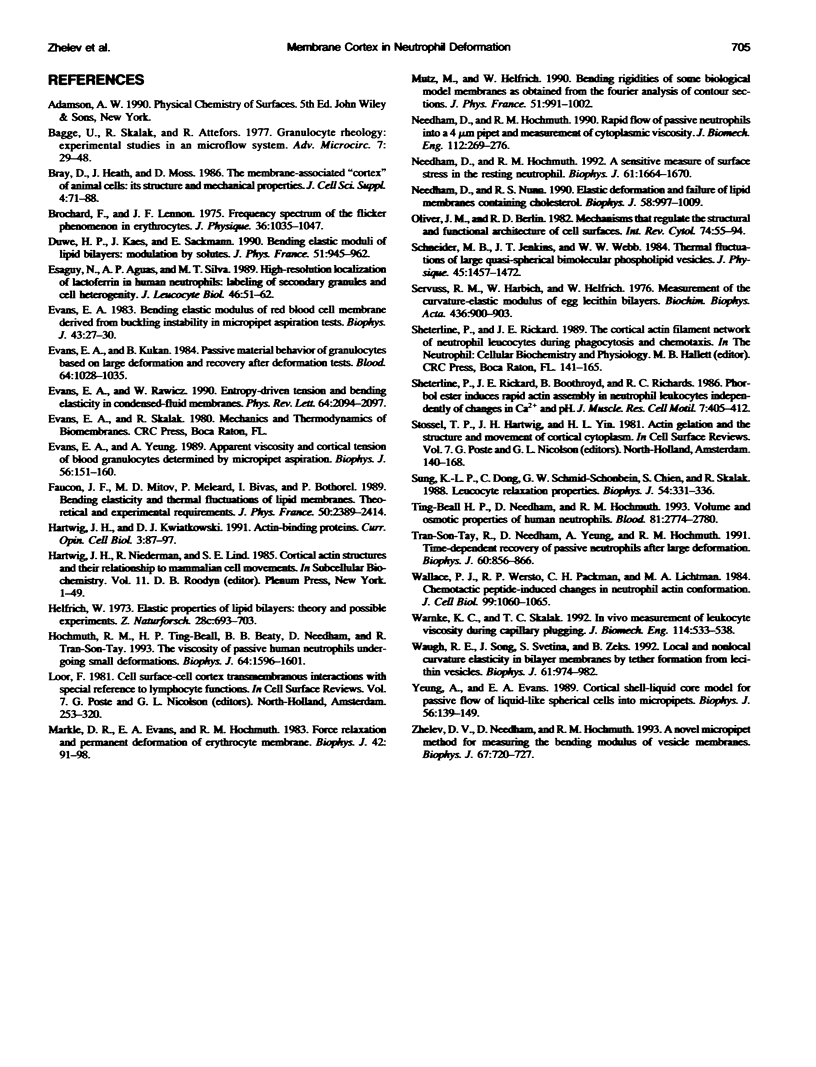
Images in this article
Selected References
These references are in PubMed. This may not be the complete list of references from this article.
- Bray D., Heath J., Moss D. The membrane-associated 'cortex' of animal cells: its structure and mechanical properties. J Cell Sci Suppl. 1986;4:71–88. doi: 10.1242/jcs.1986.supplement_4.5. [DOI] [PubMed] [Google Scholar]
- Evans E. A. Bending elastic modulus of red blood cell membrane derived from buckling instability in micropipet aspiration tests. Biophys J. 1983 Jul;43(1):27–30. doi: 10.1016/S0006-3495(83)84319-7. [DOI] [PMC free article] [PubMed] [Google Scholar]
- Evans E., Kukan B. Passive material behavior of granulocytes based on large deformation and recovery after deformation tests. Blood. 1984 Nov;64(5):1028–1035. [PubMed] [Google Scholar]
- Evans E., Yeung A. Apparent viscosity and cortical tension of blood granulocytes determined by micropipet aspiration. Biophys J. 1989 Jul;56(1):151–160. doi: 10.1016/S0006-3495(89)82660-8. [DOI] [PMC free article] [PubMed] [Google Scholar]
- Evans E, Rawicz W. Entropy-driven tension and bending elasticity in condensed-fluid membranes. Phys Rev Lett. 1990 Apr 23;64(17):2094–2097. doi: 10.1103/PhysRevLett.64.2094. [DOI] [PubMed] [Google Scholar]
- Hochmuth R. M., Ting-Beall H. P., Beaty B. B., Needham D., Tran-Son-Tay R. Viscosity of passive human neutrophils undergoing small deformations. Biophys J. 1993 May;64(5):1596–1601. doi: 10.1016/S0006-3495(93)81530-3. [DOI] [PMC free article] [PubMed] [Google Scholar]
- Markle D. R., Evans E. A., Hochmuth R. M. Force relaxation and permanent deformation of erythrocyte membrane. Biophys J. 1983 Apr;42(1):91–98. doi: 10.1016/S0006-3495(83)84372-0. [DOI] [PMC free article] [PubMed] [Google Scholar]
- Needham D., Hochmuth R. M. A sensitive measure of surface stress in the resting neutrophil. Biophys J. 1992 Jun;61(6):1664–1670. doi: 10.1016/S0006-3495(92)81970-7. [DOI] [PMC free article] [PubMed] [Google Scholar]
- Needham D., Hochmuth R. M. Rapid flow of passive neutrophils into a 4 microns pipet and measurement of cytoplasmic viscosity. J Biomech Eng. 1990 Aug;112(3):269–276. doi: 10.1115/1.2891184. [DOI] [PubMed] [Google Scholar]
- Servuss R. M., Harbich W., Helfrich W. Measurement of the curvature-elastic modulus of egg lecithin bilayers. Biochim Biophys Acta. 1976 Jul 15;436(4):900–903. doi: 10.1016/0005-2736(76)90422-3. [DOI] [PubMed] [Google Scholar]
- Sheterline P., Rickard J. E., Boothroyd B., Richards R. C. Phorbol ester induces rapid actin assembly in neutrophil leucocytes independently of changes in [Ca2+]i and pHi. J Muscle Res Cell Motil. 1986 Oct;7(5):405–412. doi: 10.1007/BF01753583. [DOI] [PubMed] [Google Scholar]
- Sung K. L., Dong C., Schmid-Schönbein G. W., Chien S., Skalak R. Leukocyte relaxation properties. Biophys J. 1988 Aug;54(2):331–336. doi: 10.1016/S0006-3495(88)82963-1. [DOI] [PMC free article] [PubMed] [Google Scholar]
- Ting-Beall H. P., Needham D., Hochmuth R. M. Volume and osmotic properties of human neutrophils. Blood. 1993 May 15;81(10):2774–2780. [PubMed] [Google Scholar]
- Waugh R. E., Song J., Svetina S., Zeks B. Local and nonlocal curvature elasticity in bilayer membranes by tether formation from lecithin vesicles. Biophys J. 1992 Apr;61(4):974–982. doi: 10.1016/S0006-3495(92)81904-5. [DOI] [PMC free article] [PubMed] [Google Scholar]
- Yeung A., Evans E. Cortical shell-liquid core model for passive flow of liquid-like spherical cells into micropipets. Biophys J. 1989 Jul;56(1):139–149. doi: 10.1016/S0006-3495(89)82659-1. [DOI] [PMC free article] [PubMed] [Google Scholar]