Abstract
Single myofibrils were isolated from chemically skinned rabbit heart and mounted in an apparatus described previously (Fearn et al., 1993; Linke et al., 1993). We measured the passive length-tension relation and active isometric force, both normalized to cross sectional area. Myofibrillar cross sectional area was calculated based on measurements of myofibril diameter from both phase-contrast images and electron micrographs. Passive tension values up to sarcomere lengths of approximately 2.2 microns were similar to those reported in larger cardiac muscle specimens. Thus, the element responsible for most, if not all, passive force of cardiac muscle at physiological sarcomere lengths appears to reside within the myofibrils. Above 2.2 microns, passive tension continued to rise, but not as steeply as reported in multicellular preparations. Apparently, structures other than the myofibrils become increasingly important in determining the magnitude of passive tension at these stretched lengths. Knowing the myofibrillar component of passive tension allowed us to infer the stress-strain relation of titin, the polypeptide thought to support passive force in the sarcomere. The elastic modulus of titin is 3.5 x 10(6) dyn cm-2, a value similar to that reported for elastin. Maximum active isometric tension in the single myofibril at sarcomere lengths of 2.1-2.3 microns was 145 +/- 35 mN/mm2 (mean +/- SD; n = 15). This value is comparable with that measured in fixed-end contractions of larger cardiac specimens, when the amount of nonmyofibrillar space in those preparations is considered. However, it is about 4 times lower than the maximum active tension previously measured in single skeletal myofibrils under similar conditions (Bartoo et al., 1993).
Full text
PDF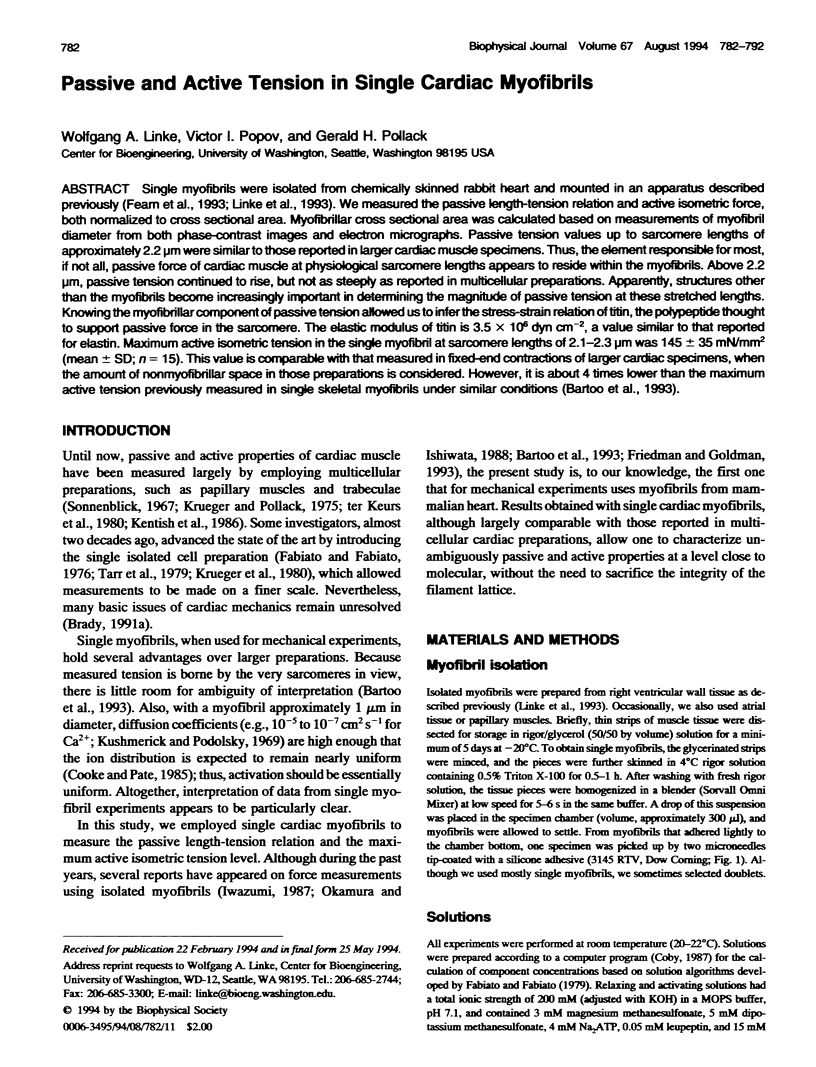
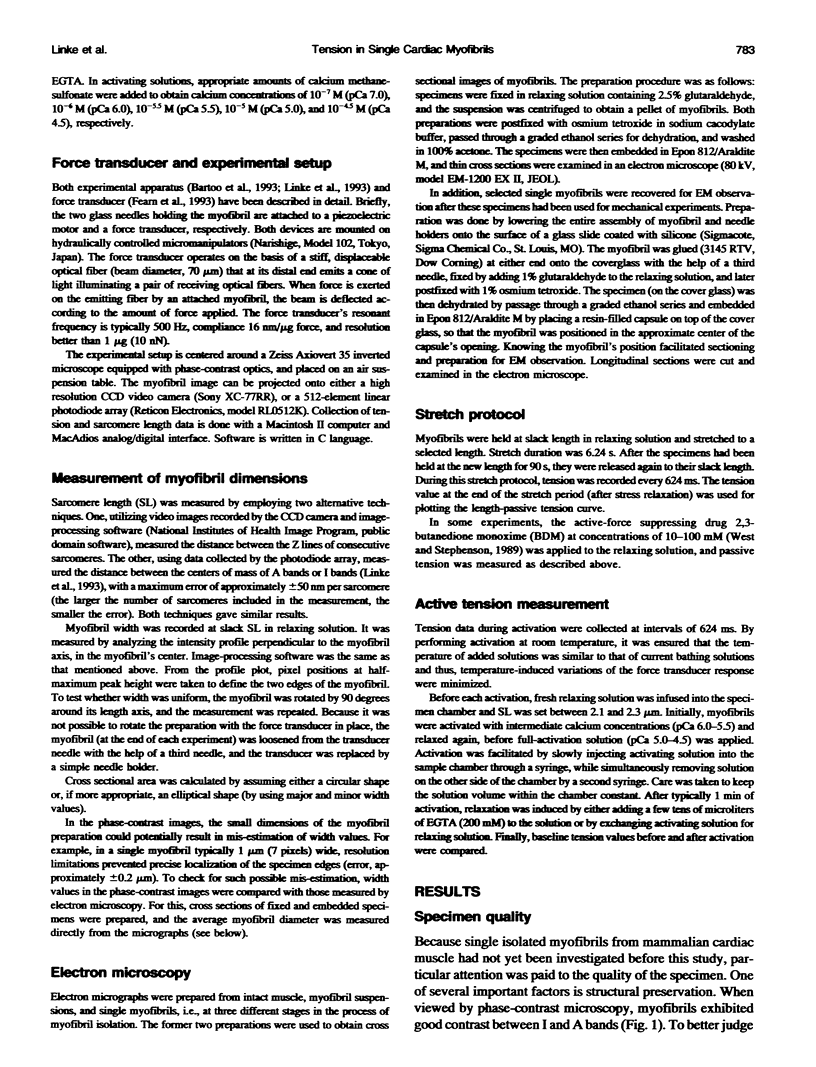
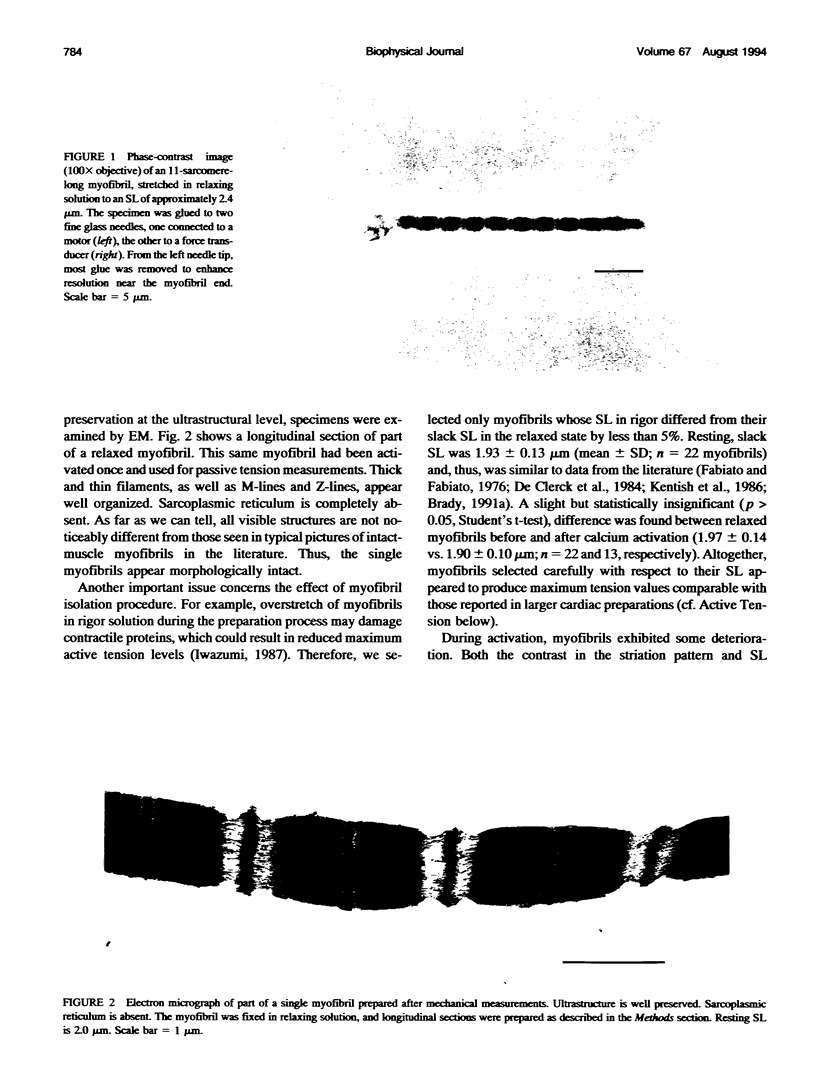
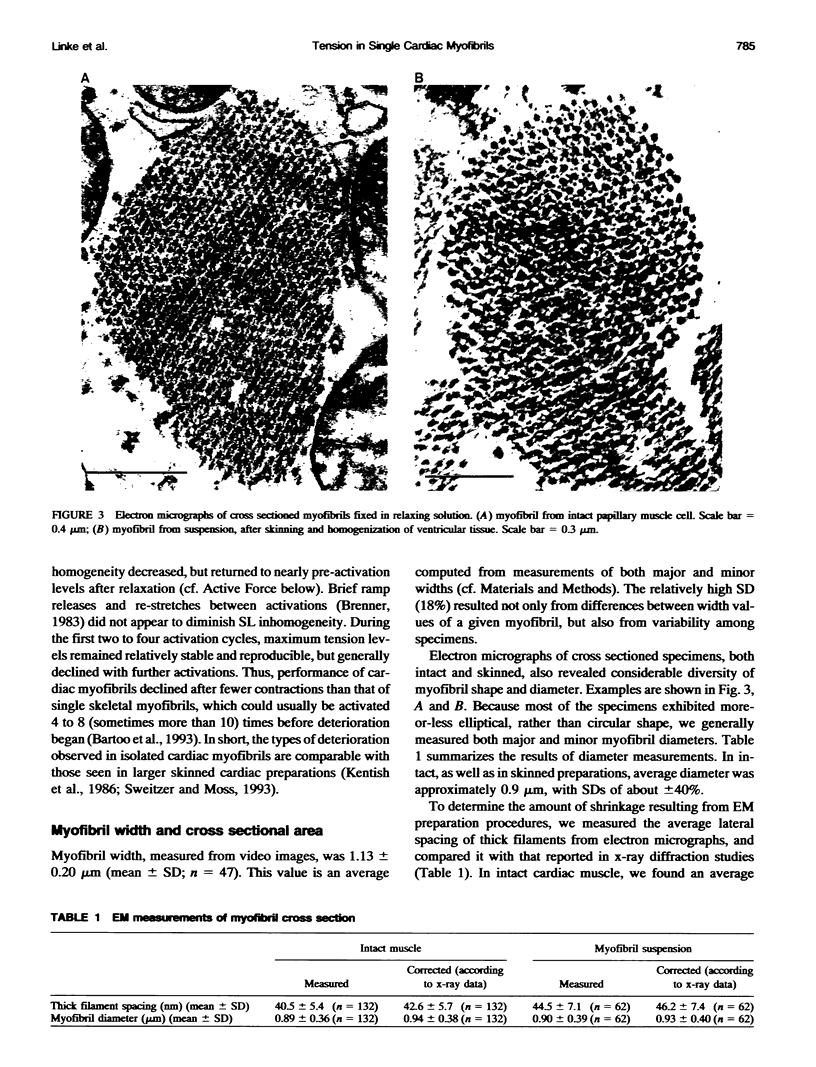
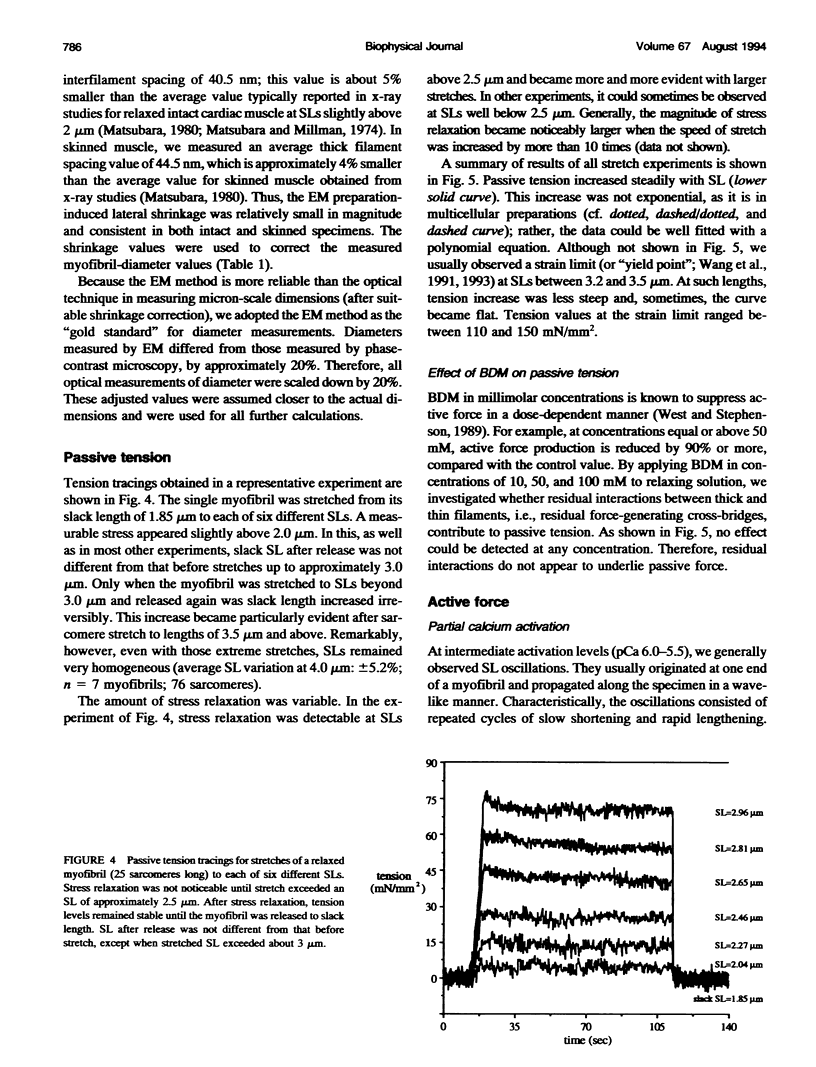
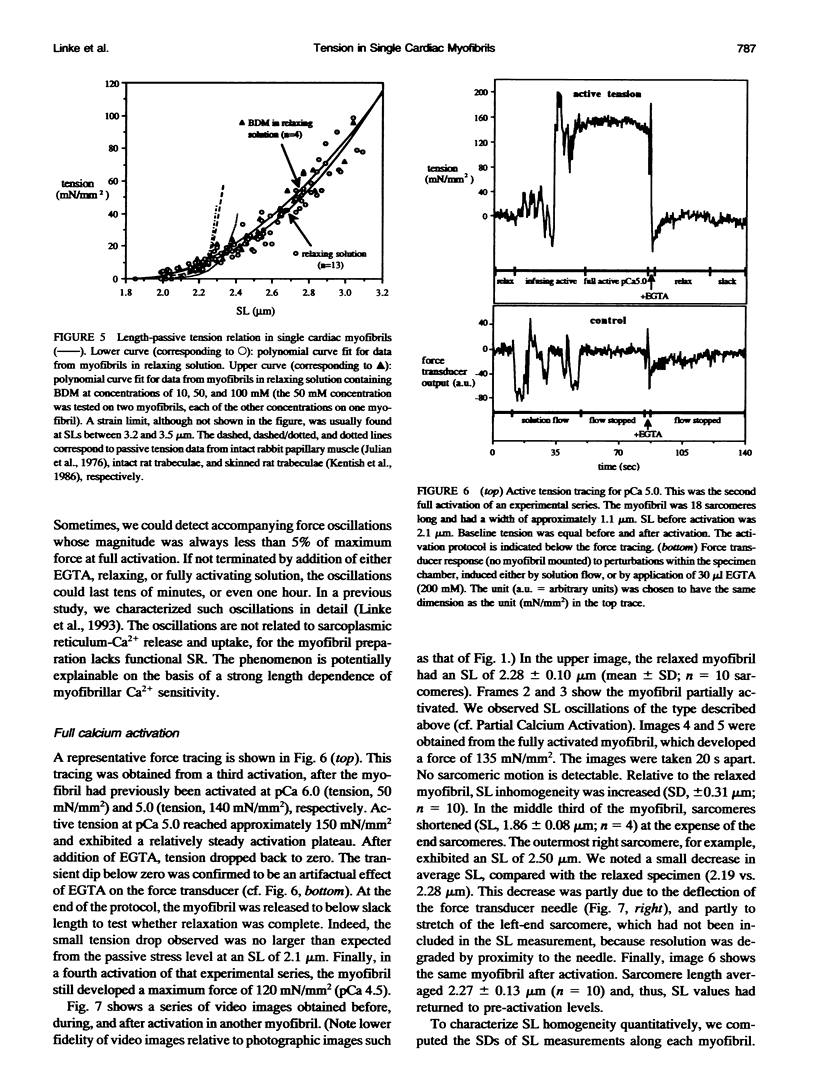
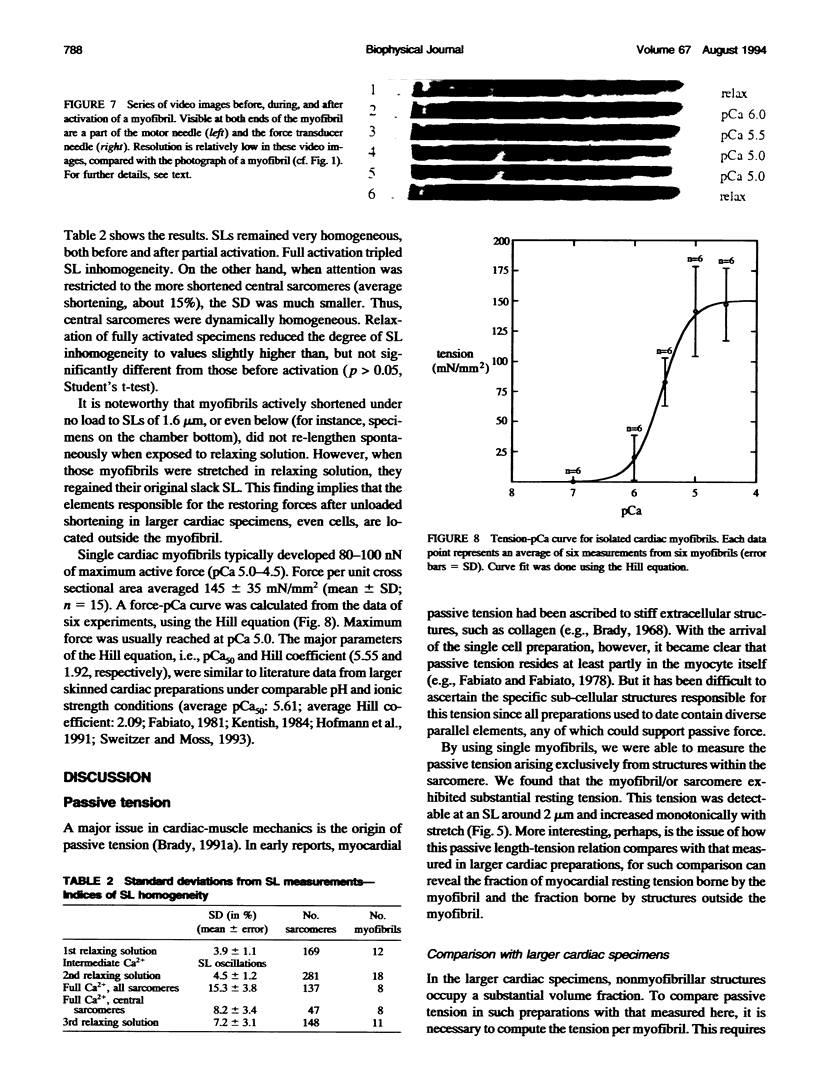
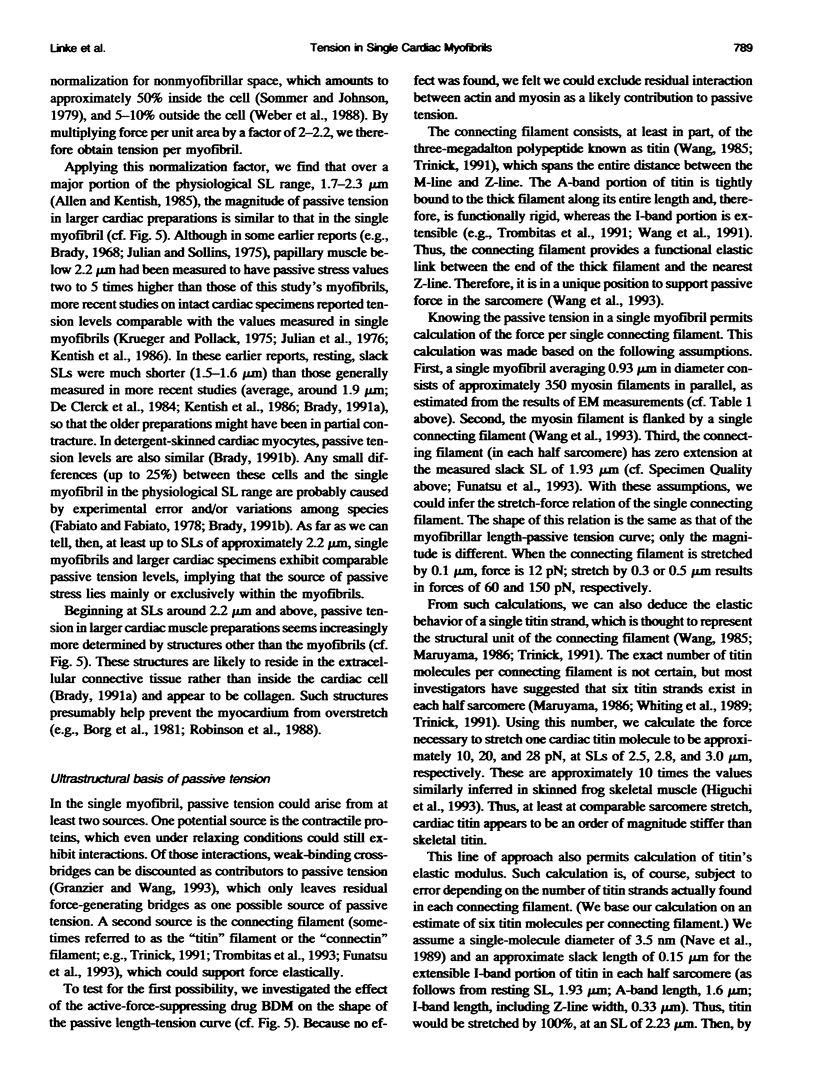
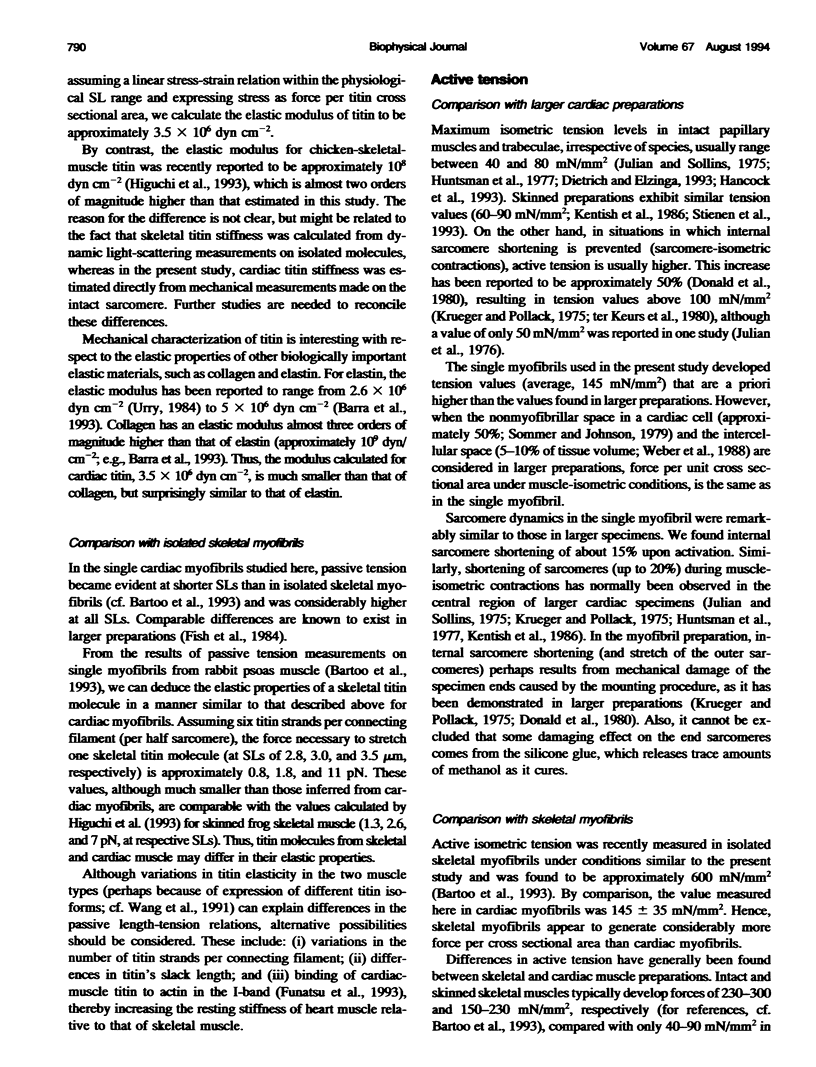
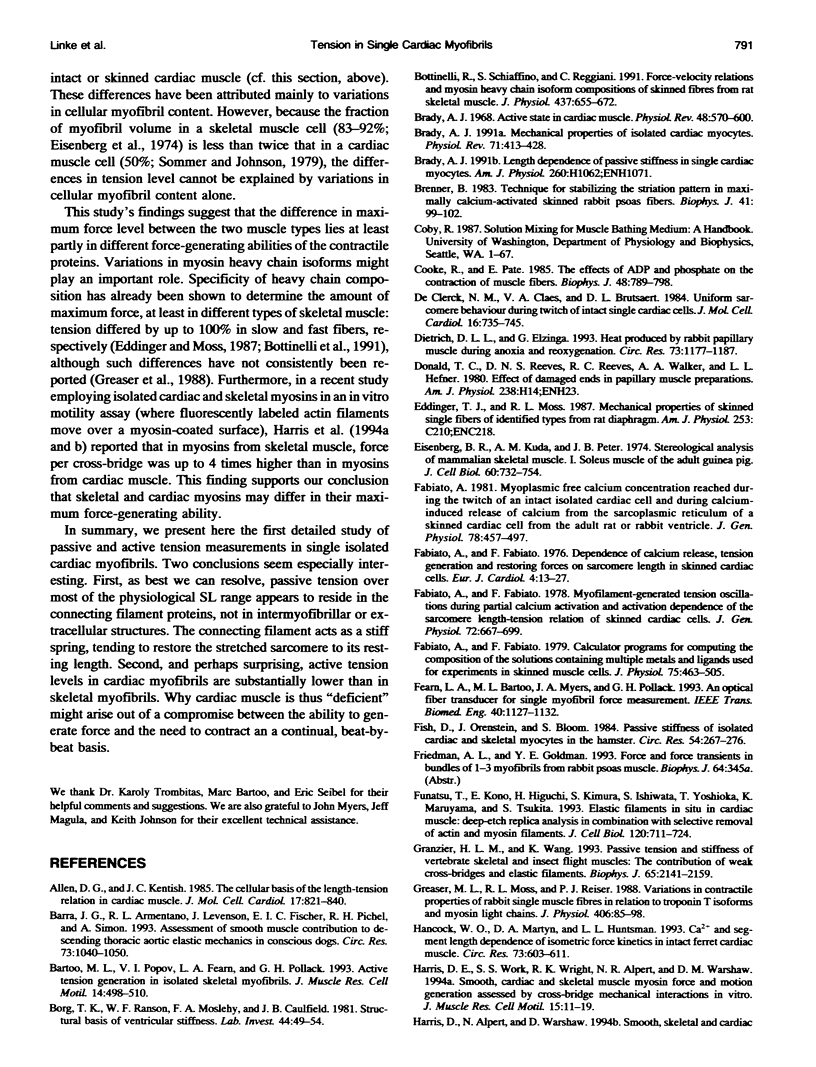
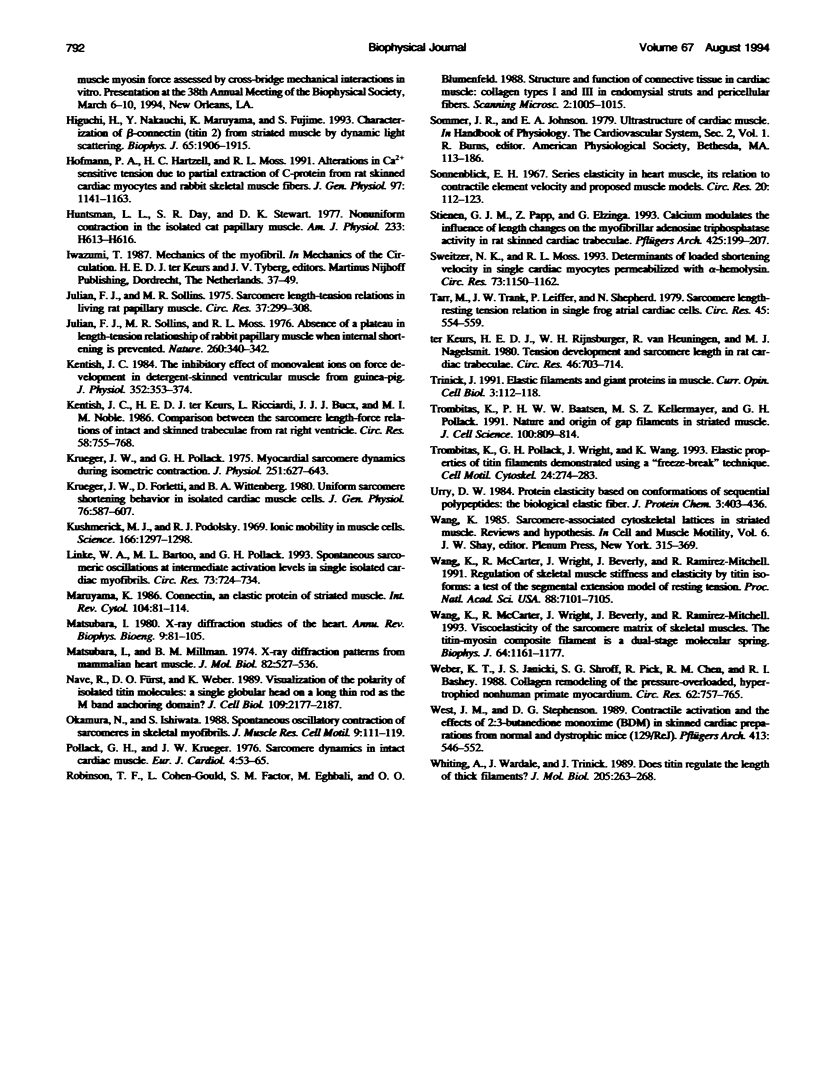
Images in this article
Selected References
These references are in PubMed. This may not be the complete list of references from this article.
- Allen D. G., Kentish J. C. The cellular basis of the length-tension relation in cardiac muscle. J Mol Cell Cardiol. 1985 Sep;17(9):821–840. doi: 10.1016/s0022-2828(85)80097-3. [DOI] [PubMed] [Google Scholar]
- Barra J. G., Armentano R. L., Levenson J., Fischer E. I., Pichel R. H., Simon A. Assessment of smooth muscle contribution to descending thoracic aortic elastic mechanics in conscious dogs. Circ Res. 1993 Dec;73(6):1040–1050. doi: 10.1161/01.res.73.6.1040. [DOI] [PubMed] [Google Scholar]
- Bartoo M. L., Popov V. I., Fearn L. A., Pollack G. H. Active tension generation in isolated skeletal myofibrils. J Muscle Res Cell Motil. 1993 Oct;14(5):498–510. doi: 10.1007/BF00297212. [DOI] [PubMed] [Google Scholar]
- Borg T. K., Ranson W. F., Moslehy F. A., Caulfield J. B. Structural basis of ventricular stiffness. Lab Invest. 1981 Jan;44(1):49–54. [PubMed] [Google Scholar]
- Bottinelli R., Schiaffino S., Reggiani C. Force-velocity relations and myosin heavy chain isoform compositions of skinned fibres from rat skeletal muscle. J Physiol. 1991 Jun;437:655–672. doi: 10.1113/jphysiol.1991.sp018617. [DOI] [PMC free article] [PubMed] [Google Scholar]
- Brady A. J. Active state in cardiac muscle. Physiol Rev. 1968 Jul;48(3):570–600. doi: 10.1152/physrev.1968.48.3.570. [DOI] [PubMed] [Google Scholar]
- Brady A. J. Length dependence of passive stiffness in single cardiac myocytes. Am J Physiol. 1991 Apr;260(4 Pt 2):H1062–H1071. doi: 10.1152/ajpheart.1991.260.4.H1062. [DOI] [PubMed] [Google Scholar]
- Brady A. J. Mechanical properties of isolated cardiac myocytes. Physiol Rev. 1991 Apr;71(2):413–428. doi: 10.1152/physrev.1991.71.2.413. [DOI] [PubMed] [Google Scholar]
- Brenner B. Technique for stabilizing the striation pattern in maximally calcium-activated skinned rabbit psoas fibers. Biophys J. 1983 Jan;41(1):99–102. doi: 10.1016/S0006-3495(83)84411-7. [DOI] [PMC free article] [PubMed] [Google Scholar]
- Cooke R., Pate E. The effects of ADP and phosphate on the contraction of muscle fibers. Biophys J. 1985 Nov;48(5):789–798. doi: 10.1016/S0006-3495(85)83837-6. [DOI] [PMC free article] [PubMed] [Google Scholar]
- Dietrich D. L., Elzinga G. Heat produced by rabbit papillary muscle during anoxia and reoxygenation. Circ Res. 1993 Dec;73(6):1177–1187. doi: 10.1161/01.res.73.6.1177. [DOI] [PubMed] [Google Scholar]
- Eddinger T. J., Moss R. L. Mechanical properties of skinned single fibers of identified types from rat diaphragm. Am J Physiol. 1987 Aug;253(2 Pt 1):C210–C218. doi: 10.1152/ajpcell.1987.253.2.C210. [DOI] [PubMed] [Google Scholar]
- Eisenberg B. R., Kuda A. M., Peter J. B. Stereological analysis of mammalian skeletal muscle. I. Soleus muscle of the adult guinea pig. J Cell Biol. 1974 Mar;60(3):732–754. doi: 10.1083/jcb.60.3.732. [DOI] [PMC free article] [PubMed] [Google Scholar]
- Fabiato A., Fabiato F. Calculator programs for computing the composition of the solutions containing multiple metals and ligands used for experiments in skinned muscle cells. J Physiol (Paris) 1979;75(5):463–505. [PubMed] [Google Scholar]
- Fabiato A., Fabiato F. Dependence of calcium release, tension generation and restoring forces on sarcomere length in skinned cardiac cells. Eur J Cardiol. 1976 May;4 (Suppl):13–27. [PubMed] [Google Scholar]
- Fabiato A., Fabiato F. Myofilament-generated tension oscillations during partial calcium activation and activation dependence of the sarcomere length-tension relation of skinned cardiac cells. J Gen Physiol. 1978 Nov;72(5):667–699. doi: 10.1085/jgp.72.5.667. [DOI] [PMC free article] [PubMed] [Google Scholar]
- Fabiato A. Myoplasmic free calcium concentration reached during the twitch of an intact isolated cardiac cell and during calcium-induced release of calcium from the sarcoplasmic reticulum of a skinned cardiac cell from the adult rat or rabbit ventricle. J Gen Physiol. 1981 Nov;78(5):457–497. doi: 10.1085/jgp.78.5.457. [DOI] [PMC free article] [PubMed] [Google Scholar]
- Fearn L. A., Bartoo M. L., Myers J. A., Pollack G. H. An optical fiber transducer for single myofibril force measurement. IEEE Trans Biomed Eng. 1993 Nov;40(11):1127–1132. doi: 10.1109/10.245630. [DOI] [PubMed] [Google Scholar]
- Fish D., Orenstein J., Bloom S. Passive stiffness of isolated cardiac and skeletal myocytes in the hamster. Circ Res. 1984 Mar;54(3):267–276. doi: 10.1161/01.res.54.3.267. [DOI] [PubMed] [Google Scholar]
- Funatsu T., Kono E., Higuchi H., Kimura S., Ishiwata S., Yoshioka T., Maruyama K., Tsukita S. Elastic filaments in situ in cardiac muscle: deep-etch replica analysis in combination with selective removal of actin and myosin filaments. J Cell Biol. 1993 Feb;120(3):711–724. doi: 10.1083/jcb.120.3.711. [DOI] [PMC free article] [PubMed] [Google Scholar]
- Granzier H. L., Wang K. Passive tension and stiffness of vertebrate skeletal and insect flight muscles: the contribution of weak cross-bridges and elastic filaments. Biophys J. 1993 Nov;65(5):2141–2159. doi: 10.1016/S0006-3495(93)81262-1. [DOI] [PMC free article] [PubMed] [Google Scholar]
- Greaser M. L., Moss R. L., Reiser P. J. Variations in contractile properties of rabbit single muscle fibres in relation to troponin T isoforms and myosin light chains. J Physiol. 1988 Dec;406:85–98. doi: 10.1113/jphysiol.1988.sp017370. [DOI] [PMC free article] [PubMed] [Google Scholar]
- Hancock W. O., Martyn D. A., Huntsman L. L. Ca2+ and segment length dependence of isometric force kinetics in intact ferret cardiac muscle. Circ Res. 1993 Oct;73(4):603–611. doi: 10.1161/01.res.73.4.603. [DOI] [PubMed] [Google Scholar]
- Harris D. E., Work S. S., Wright R. K., Alpert N. R., Warshaw D. M. Smooth, cardiac and skeletal muscle myosin force and motion generation assessed by cross-bridge mechanical interactions in vitro. J Muscle Res Cell Motil. 1994 Feb;15(1):11–19. doi: 10.1007/BF00123828. [DOI] [PubMed] [Google Scholar]
- Higuchi H., Nakauchi Y., Maruyama K., Fujime S. Characterization of beta-connectin (titin 2) from striated muscle by dynamic light scattering. Biophys J. 1993 Nov;65(5):1906–1915. doi: 10.1016/S0006-3495(93)81261-X. [DOI] [PMC free article] [PubMed] [Google Scholar]
- Hofmann P. A., Hartzell H. C., Moss R. L. Alterations in Ca2+ sensitive tension due to partial extraction of C-protein from rat skinned cardiac myocytes and rabbit skeletal muscle fibers. J Gen Physiol. 1991 Jun;97(6):1141–1163. doi: 10.1085/jgp.97.6.1141. [DOI] [PMC free article] [PubMed] [Google Scholar]
- Huntsman L. L., Day S. R., Stewart D. K. Nonuniform contraction in the isolated cat papillary muscle. Am J Physiol. 1977 Nov;233(5):H613–H616. doi: 10.1152/ajpheart.1977.233.5.H613. [DOI] [PubMed] [Google Scholar]
- Julian F. J., Sollins M. R. Sarcomere length-tension relations in living rat papillary muscle. Circ Res. 1975 Sep;37(3):299–308. doi: 10.1161/01.res.37.3.299. [DOI] [PubMed] [Google Scholar]
- Kentish J. C. The inhibitory effects of monovalent ions on force development in detergent-skinned ventricular muscle from guinea-pig. J Physiol. 1984 Jul;352:353–374. doi: 10.1113/jphysiol.1984.sp015296. [DOI] [PMC free article] [PubMed] [Google Scholar]
- Kentish J. C., ter Keurs H. E., Ricciardi L., Bucx J. J., Noble M. I. Comparison between the sarcomere length-force relations of intact and skinned trabeculae from rat right ventricle. Influence of calcium concentrations on these relations. Circ Res. 1986 Jun;58(6):755–768. doi: 10.1161/01.res.58.6.755. [DOI] [PubMed] [Google Scholar]
- Krueger J. W., Pollack G. H. Myocardial sarcomere dynamics during isometric contraction. J Physiol. 1975 Oct;251(3):627–643. doi: 10.1113/jphysiol.1975.sp011112. [DOI] [PMC free article] [PubMed] [Google Scholar]
- Linke W. A., Bartoo M. L., Pollack G. H. Spontaneous sarcomeric oscillations at intermediate activation levels in single isolated cardiac myofibrils. Circ Res. 1993 Oct;73(4):724–734. doi: 10.1161/01.res.73.4.724. [DOI] [PubMed] [Google Scholar]
- Maruyama K. Connectin, an elastic filamentous protein of striated muscle. Int Rev Cytol. 1986;104:81–114. doi: 10.1016/s0074-7696(08)61924-5. [DOI] [PubMed] [Google Scholar]
- Nave R., Fürst D. O., Weber K. Visualization of the polarity of isolated titin molecules: a single globular head on a long thin rod as the M band anchoring domain? J Cell Biol. 1989 Nov;109(5):2177–2187. doi: 10.1083/jcb.109.5.2177. [DOI] [PMC free article] [PubMed] [Google Scholar]
- Okamura N., Ishiwata S. Spontaneous oscillatory contraction of sarcomeres in skeletal myofibrils. J Muscle Res Cell Motil. 1988 Apr;9(2):111–119. doi: 10.1007/BF01773733. [DOI] [PubMed] [Google Scholar]
- Pollack G. H., Krueger J. W. Sarcomere dynamics in intact cardiac muscle. Eur J Cardiol. 1976 May;4 (Suppl):53–65. [PubMed] [Google Scholar]
- Robinson T. F., Cohen-Gould L., Factor S. M., Eghbali M., Blumenfeld O. O. Structure and function of connective tissue in cardiac muscle: collagen types I and III in endomysial struts and pericellular fibers. Scanning Microsc. 1988 Jun;2(2):1005–1015. [PubMed] [Google Scholar]
- Stienen G. J., Papp Z., Elzinga G. Calcium modulates the influence of length changes on the myofibrillar adenosine triphosphatase activity in rat skinned cardiac trabeculae. Pflugers Arch. 1993 Nov;425(3-4):199–207. doi: 10.1007/BF00374167. [DOI] [PubMed] [Google Scholar]
- Sweitzer N. K., Moss R. L. Determinants of loaded shortening velocity in single cardiac myocytes permeabilized with alpha-hemolysin. Circ Res. 1993 Dec;73(6):1150–1162. doi: 10.1161/01.res.73.6.1150. [DOI] [PubMed] [Google Scholar]
- Tarr M., Trank J. W., Leiffer P., Shepherd N. Sarcomere length-resting tension relation in single frog atrial cardiac cells. Circ Res. 1979 Oct;45(4):554–559. doi: 10.1161/01.res.45.4.554. [DOI] [PubMed] [Google Scholar]
- Trinick J. Elastic filaments and giant proteins in muscle. Curr Opin Cell Biol. 1991 Feb;3(1):112–119. doi: 10.1016/0955-0674(91)90173-v. [DOI] [PubMed] [Google Scholar]
- Trombitás K., Baatsen P. H., Kellermayer M. S., Pollack G. H. Nature and origin of gap filaments in striated muscle. J Cell Sci. 1991 Dec;100(Pt 4):809–814. doi: 10.1242/jcs.100.4.809. [DOI] [PubMed] [Google Scholar]
- Trombitás K., Pollack G. H., Wright J., Wang K. Elastic properties of titin filaments demonstrated using a "freeze-break" technique. Cell Motil Cytoskeleton. 1993;24(4):274–283. doi: 10.1002/cm.970240408. [DOI] [PubMed] [Google Scholar]
- Wang K., McCarter R., Wright J., Beverly J., Ramirez-Mitchell R. Regulation of skeletal muscle stiffness and elasticity by titin isoforms: a test of the segmental extension model of resting tension. Proc Natl Acad Sci U S A. 1991 Aug 15;88(16):7101–7105. doi: 10.1073/pnas.88.16.7101. [DOI] [PMC free article] [PubMed] [Google Scholar]
- Wang K., McCarter R., Wright J., Beverly J., Ramirez-Mitchell R. Viscoelasticity of the sarcomere matrix of skeletal muscles. The titin-myosin composite filament is a dual-stage molecular spring. Biophys J. 1993 Apr;64(4):1161–1177. doi: 10.1016/S0006-3495(93)81482-6. [DOI] [PMC free article] [PubMed] [Google Scholar]
- Wang K. Sarcomere-associated cytoskeletal lattices in striated muscle. Review and hypothesis. Cell Muscle Motil. 1985;6:315–369. doi: 10.1007/978-1-4757-4723-2_10. [DOI] [PubMed] [Google Scholar]
- Weber K. T., Janicki J. S., Shroff S. G., Pick R., Chen R. M., Bashey R. I. Collagen remodeling of the pressure-overloaded, hypertrophied nonhuman primate myocardium. Circ Res. 1988 Apr;62(4):757–765. doi: 10.1161/01.res.62.4.757. [DOI] [PubMed] [Google Scholar]
- West J. M., Stephenson D. G. Contractile activation and the effects of 2,3-butanedione monoxime (BDM) in skinned cardiac preparations from normal and dystrophic mice (129/ReJ). Pflugers Arch. 1989 Mar;413(5):546–552. doi: 10.1007/BF00594187. [DOI] [PubMed] [Google Scholar]
- Whiting A., Wardale J., Trinick J. Does titin regulate the length of muscle thick filaments? J Mol Biol. 1989 Jan 5;205(1):263–268. doi: 10.1016/0022-2836(89)90381-1. [DOI] [PubMed] [Google Scholar]
- ter Keurs H. E., Rijnsburger W. H., van Heuningen R., Nagelsmit M. J. Tension development and sarcomere length in rat cardiac trabeculae. Evidence of length-dependent activation. Circ Res. 1980 May;46(5):703–714. doi: 10.1161/01.res.46.5.703. [DOI] [PubMed] [Google Scholar]