Abstract
The modulation of the calcium release channel (CRC) by protein kinases and phosphatases was studied. For this purpose, we have developed a microsyringe applicator to achieve sequential and multiple treatments with highly purified kinases and phosphatases applied directly at the bilayer surface. Terminal cisternae vesicles of sarcoplasmic reticulum from rabbit fast twitch skeletal muscle were fused to planar lipid bilayers, and single-channel currents were measured at zero holding potential, at 0.15 microM free Ca2+, +/- 0.5 mM ATP and +/- 2.6 mM free Mg2+. Sequential dephosphorylation and rephosphorylation rendered the CRC sensitive and insensitive to block by Mg2+, respectively. Channel recovery from Mg2+ block was obtained by exogenous protein kinase A (PKA) or by Ca2+/calmodulin-dependent protein kinase II (CalPK II). Somewhat different characteristics were observed with the two kinases, suggesting two different states of phosphorylation. Channel block by Mg2+ was restored by dephosphorylation using protein phosphatase 1 (PPT1). Before application of protein kinases or phosphatases, channels were found to be "dephosphorylated" (inactive) in 60% and "phosphorylated" (active) in 40% of 51 single-channel experiments based on the criterion of sensitivity to block by Mg2+. Thus, these two states were interconvertable by treatment with exogenously added protein kinases and phosphatases. Endogenous Ca2+/calmodulin-dependent protein kinase (end CalPK) had an opposite action to exogenous CalPK II. Previously, dephosphorylated channels using PPT (Mg2+ absent) were blocked in the closed state by action of endogenous CalPK. This block was removed to normal activity by the action of either PPT or by exogenous CalPK II. Our findings are consistent with a physiological role for phosphorylation/dephosphorylation in the modulation of the calcium release channel of sarcoplasmic reticulum from skeletal muscle. A corollary of our studies is that only the phosphorylated channel is active under physiological conditions (mM Mg2+). Our studies suggest that phosphorylation can be at more than one site and, depending on the site, can have different functional consequences on the CRC.
Full text
PDF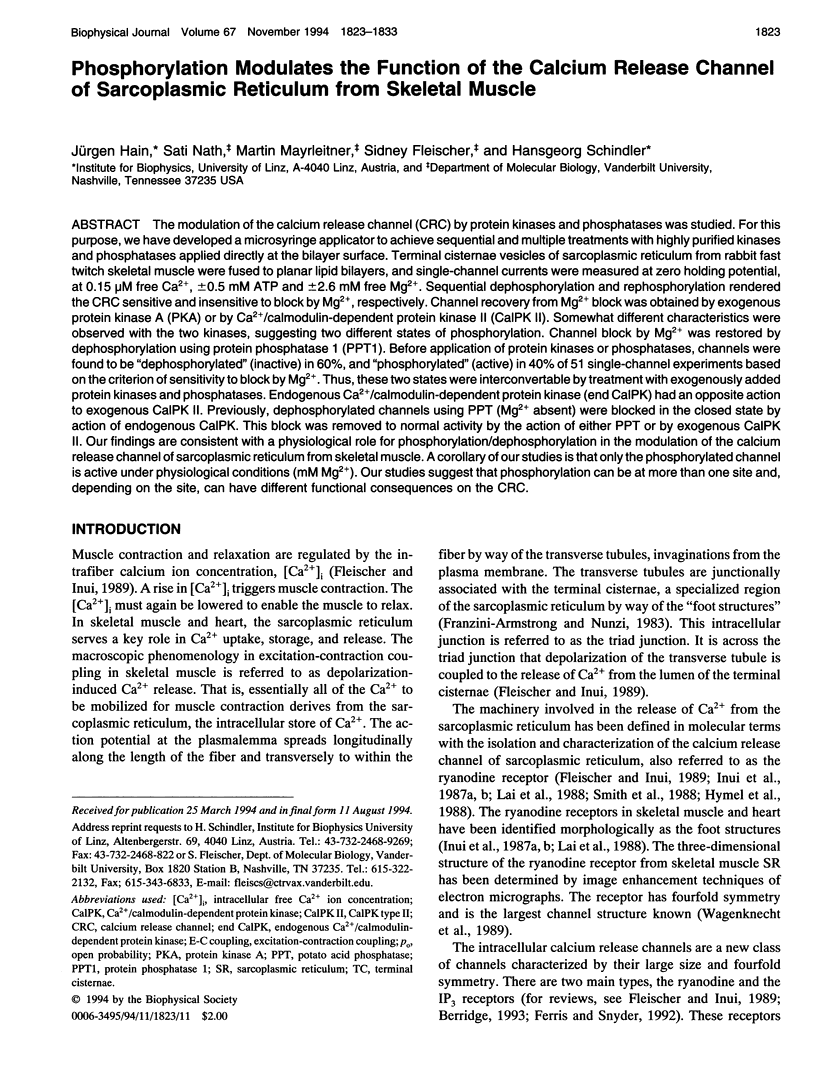
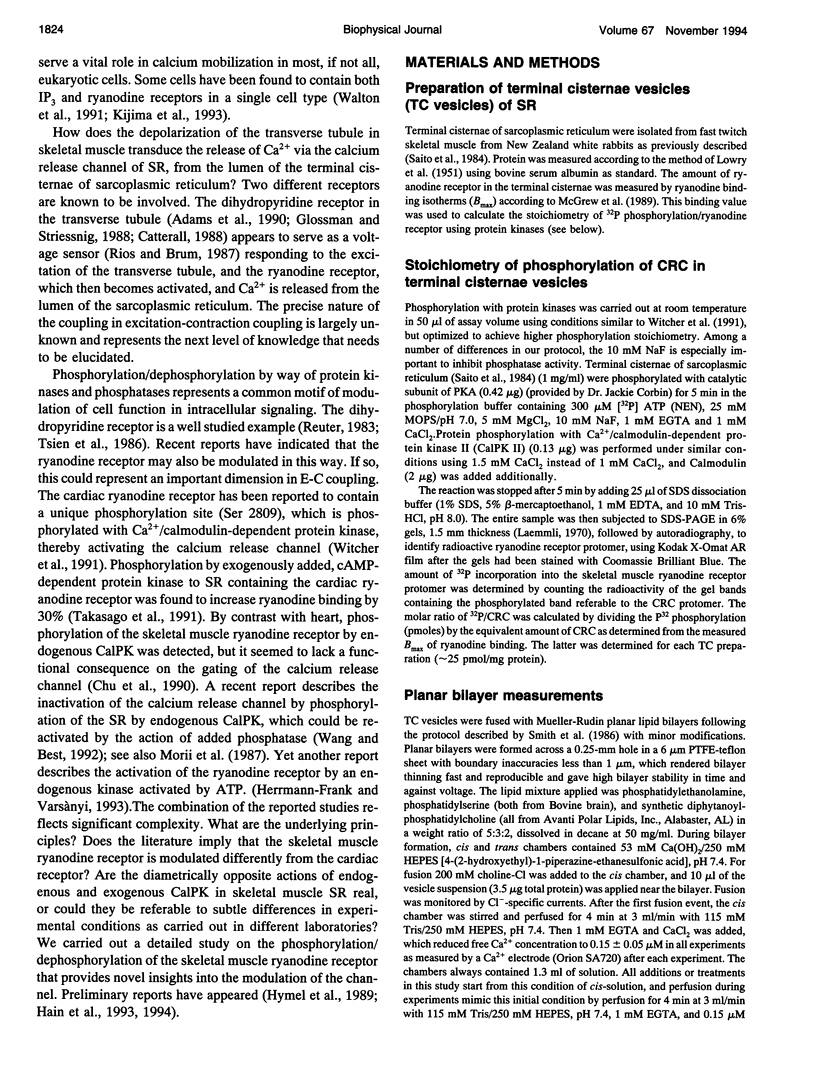
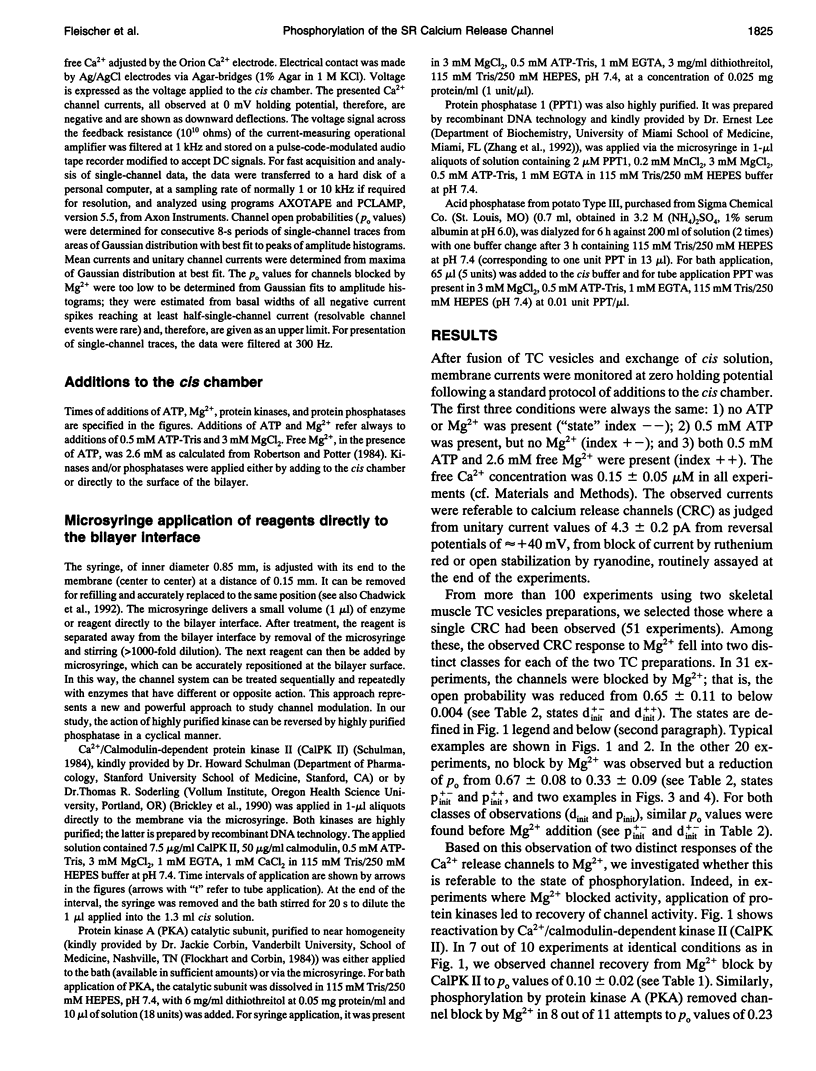
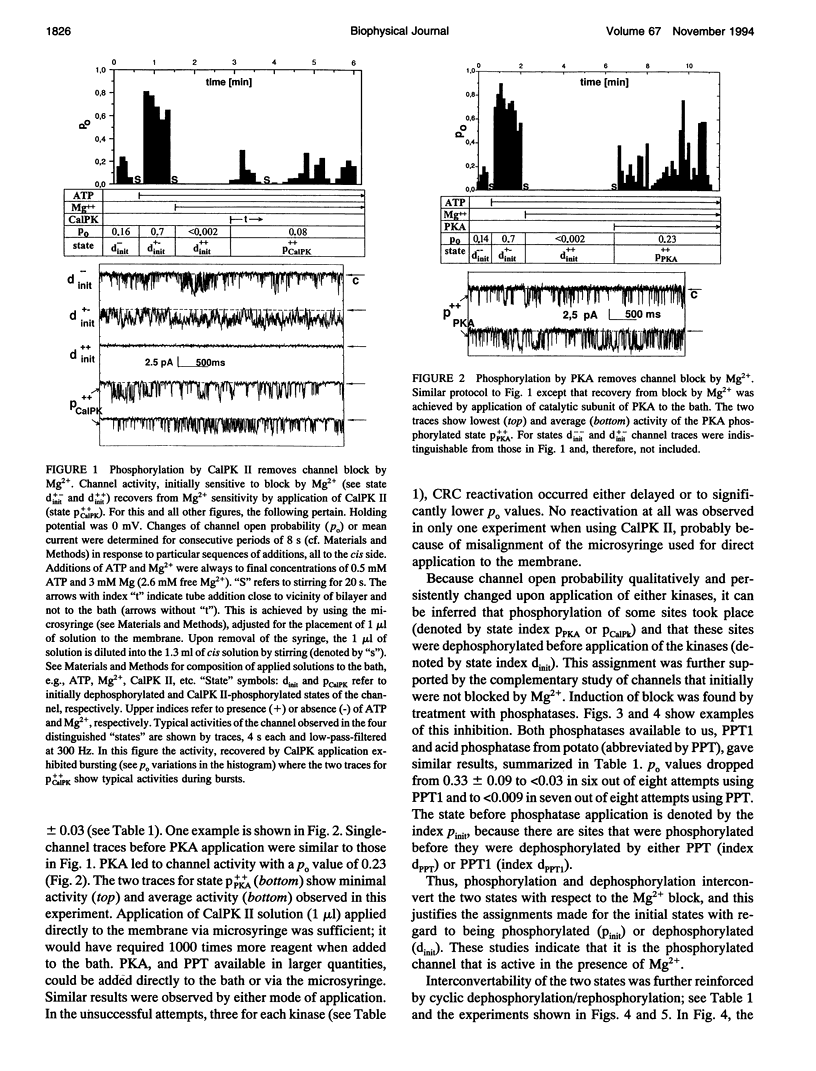
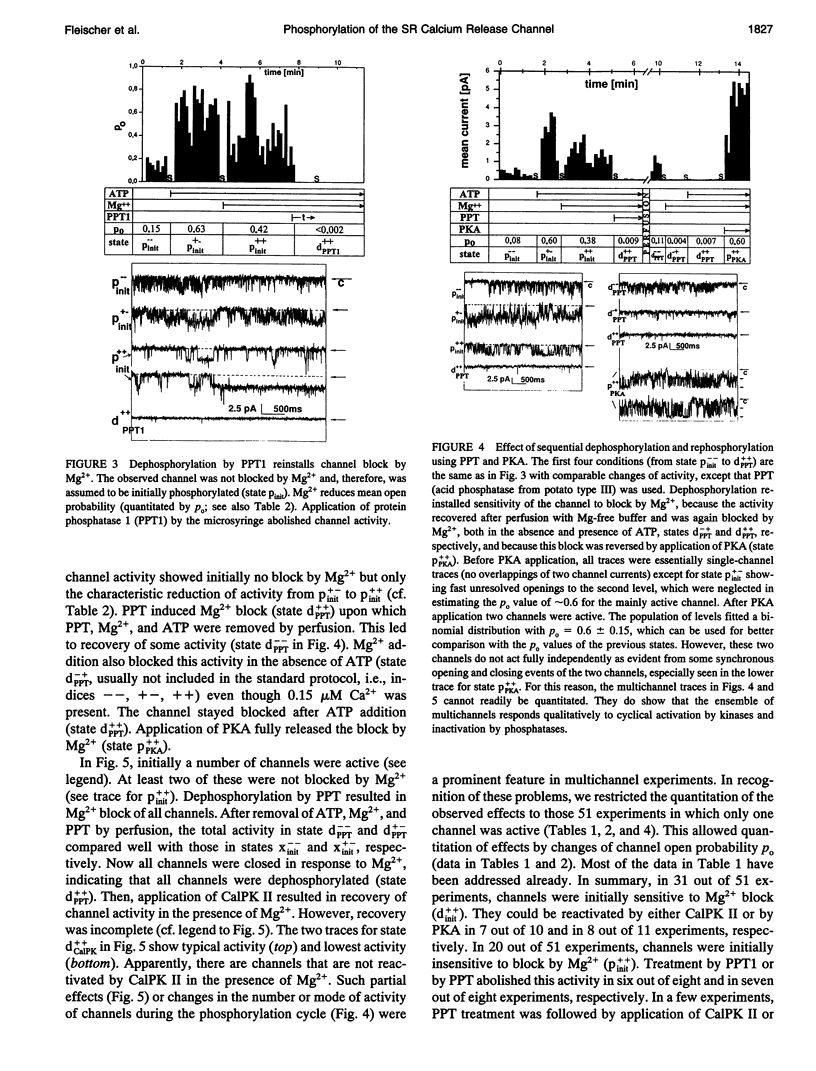
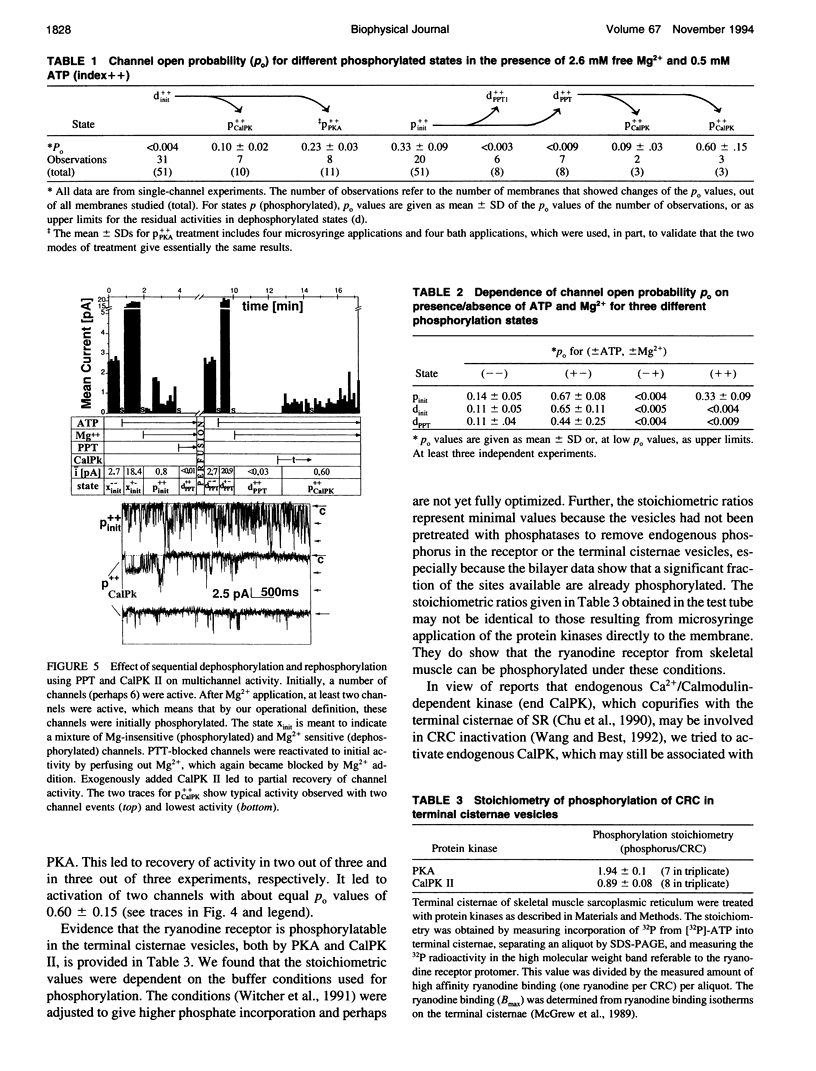
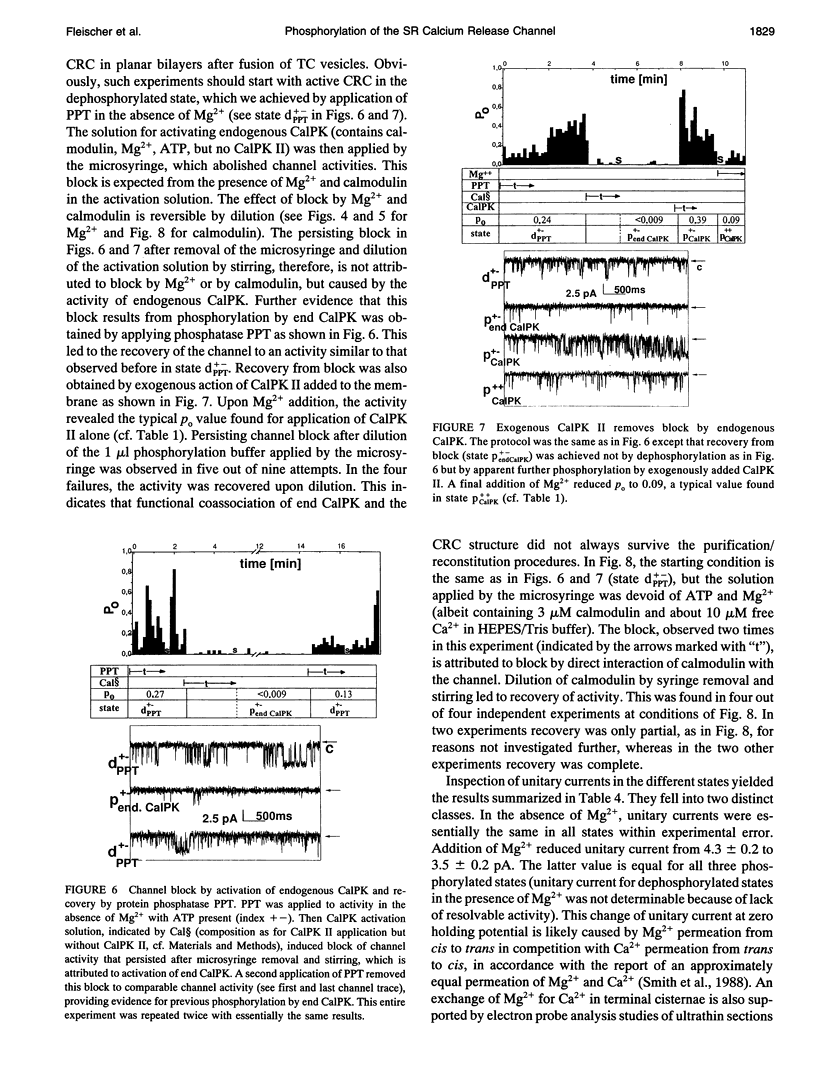
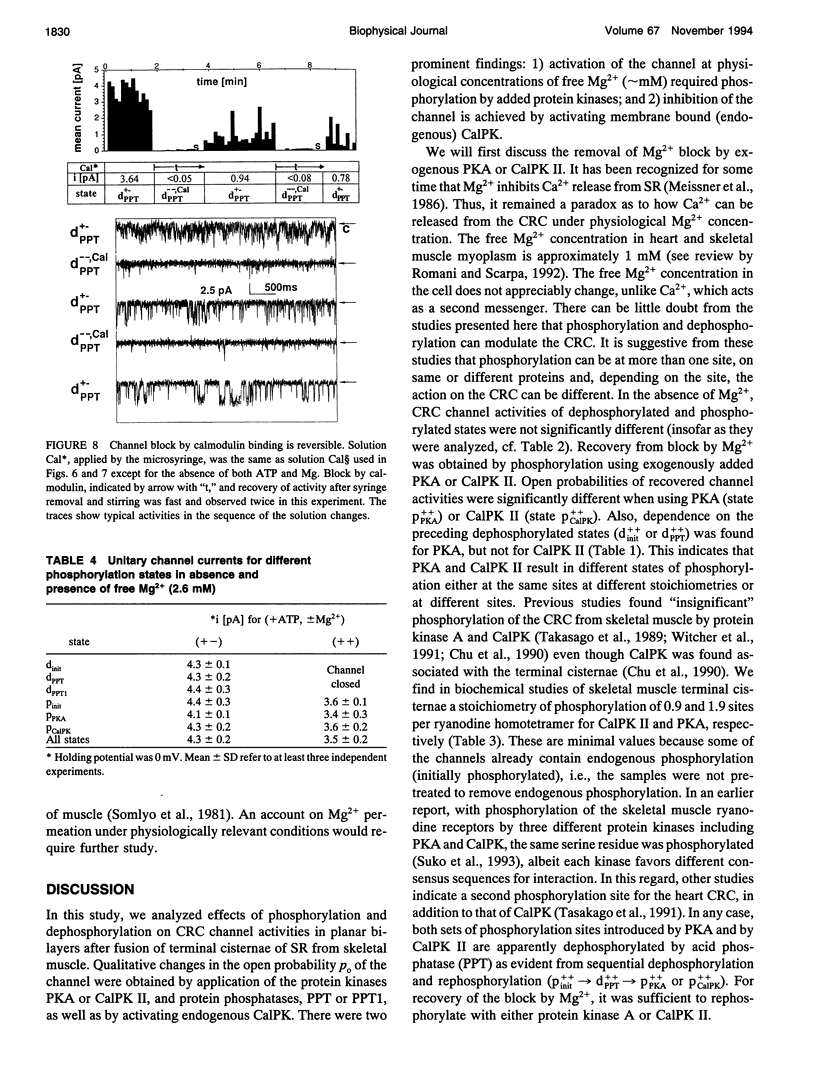
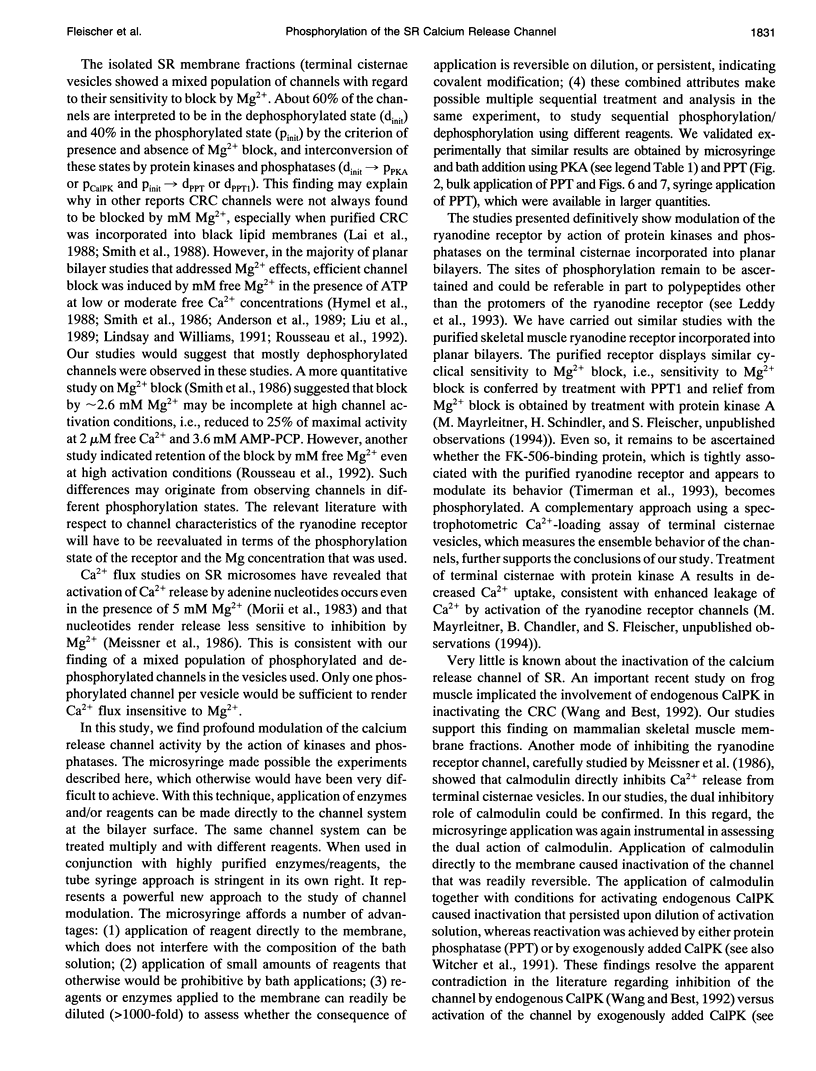
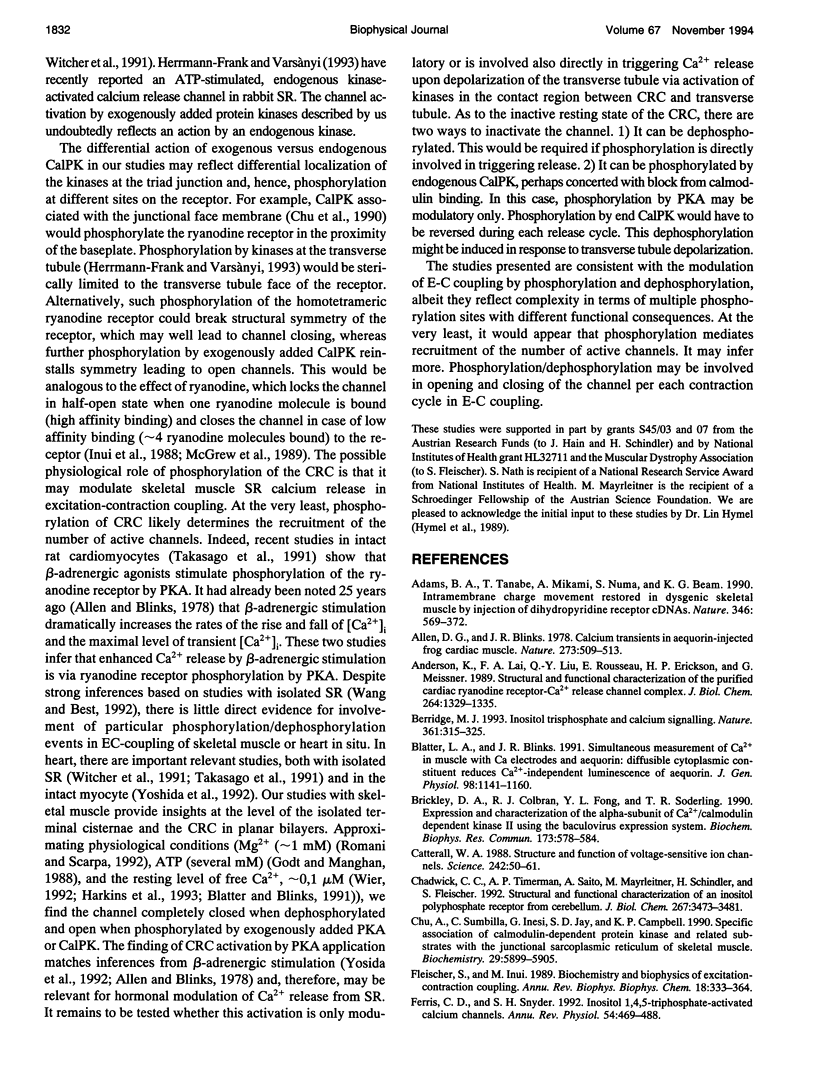
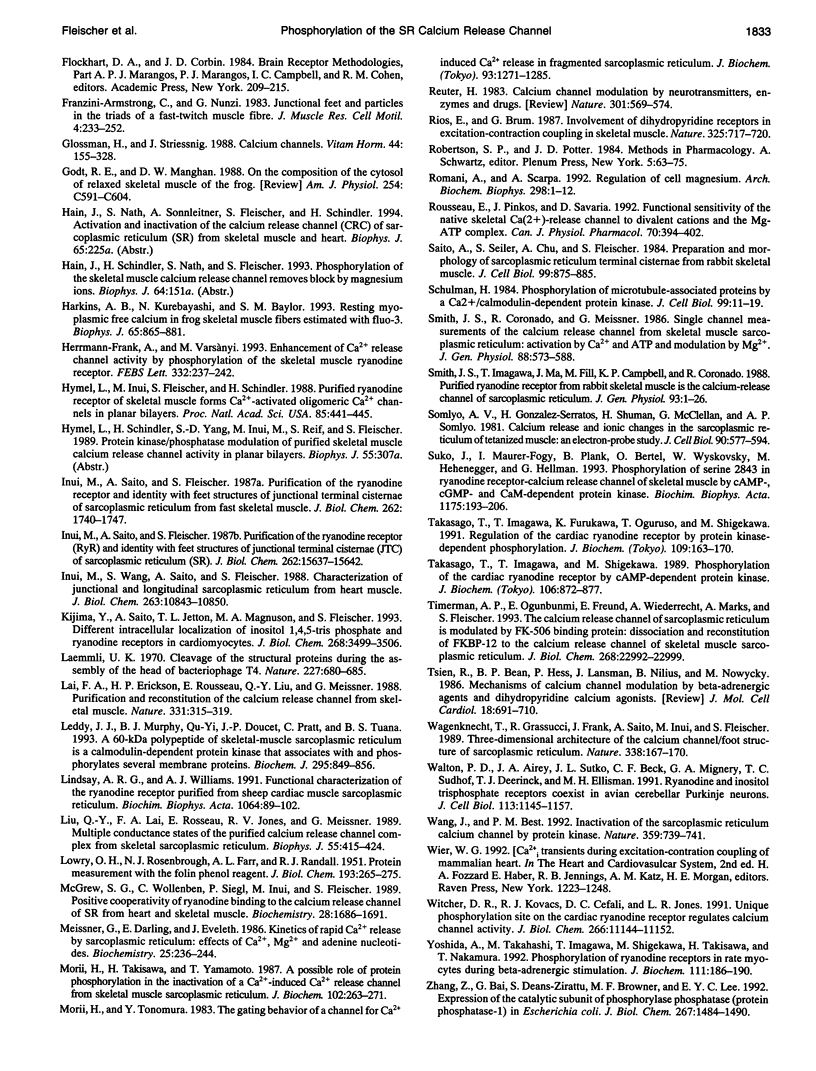
Selected References
These references are in PubMed. This may not be the complete list of references from this article.
- Adams B. A., Tanabe T., Mikami A., Numa S., Beam K. G. Intramembrane charge movement restored in dysgenic skeletal muscle by injection of dihydropyridine receptor cDNAs. Nature. 1990 Aug 9;346(6284):569–572. doi: 10.1038/346569a0. [DOI] [PubMed] [Google Scholar]
- Allen D. G., Blinks J. R. Calcium transients in aequorin-injected frog cardiac muscle. Nature. 1978 Jun 15;273(5663):509–513. doi: 10.1038/273509a0. [DOI] [PubMed] [Google Scholar]
- Anderson K., Lai F. A., Liu Q. Y., Rousseau E., Erickson H. P., Meissner G. Structural and functional characterization of the purified cardiac ryanodine receptor-Ca2+ release channel complex. J Biol Chem. 1989 Jan 15;264(2):1329–1335. [PubMed] [Google Scholar]
- Berridge M. J. Inositol trisphosphate and calcium signalling. Nature. 1993 Jan 28;361(6410):315–325. doi: 10.1038/361315a0. [DOI] [PubMed] [Google Scholar]
- Blatter L. A., Blinks J. R. Simultaneous measurement of Ca2+ in muscle with Ca electrodes and aequorin. Diffusible cytoplasmic constituent reduces Ca(2+)-independent luminescence of aequorin. J Gen Physiol. 1991 Dec;98(6):1141–1160. doi: 10.1085/jgp.98.6.1141. [DOI] [PMC free article] [PubMed] [Google Scholar]
- Brickey D. A., Colbran R. J., Fong Y. L., Soderling T. R. Expression and characterization of the alpha-subunit of Ca2+/calmodulin-dependent protein kinase II using the baculovirus expression system. Biochem Biophys Res Commun. 1990 Dec 14;173(2):578–584. doi: 10.1016/s0006-291x(05)80074-9. [DOI] [PubMed] [Google Scholar]
- Catterall W. A. Structure and function of voltage-sensitive ion channels. Science. 1988 Oct 7;242(4875):50–61. doi: 10.1126/science.2459775. [DOI] [PubMed] [Google Scholar]
- Chadwick C. C., Timerman A. P., Saito A., Mayrleitner M., Schindler H., Fleischer S. Structural and functional characterization of an inositol polyphosphate receptor from cerebellum. J Biol Chem. 1992 Feb 15;267(5):3473–3481. [PubMed] [Google Scholar]
- Chu A., Sumbilla C., Inesi G., Jay S. D., Campbell K. P. Specific association of calmodulin-dependent protein kinase and related substrates with the junctional sarcoplasmic reticulum of skeletal muscle. Biochemistry. 1990 Jun 26;29(25):5899–5905. doi: 10.1021/bi00477a003. [DOI] [PubMed] [Google Scholar]
- Ferris C. D., Snyder S. H. Inositol 1,4,5-trisphosphate-activated calcium channels. Annu Rev Physiol. 1992;54:469–488. doi: 10.1146/annurev.ph.54.030192.002345. [DOI] [PubMed] [Google Scholar]
- Fleischer S., Inui M. Biochemistry and biophysics of excitation-contraction coupling. Annu Rev Biophys Biophys Chem. 1989;18:333–364. doi: 10.1146/annurev.bb.18.060189.002001. [DOI] [PubMed] [Google Scholar]
- Franzini-Armstrong C., Nunzi G. Junctional feet and particles in the triads of a fast-twitch muscle fibre. J Muscle Res Cell Motil. 1983 Apr;4(2):233–252. doi: 10.1007/BF00712033. [DOI] [PubMed] [Google Scholar]
- Glossmann H., Striessnig J. Calcium channels. Vitam Horm. 1988;44:155–328. doi: 10.1016/s0083-6729(08)60695-0. [DOI] [PubMed] [Google Scholar]
- Godt R. E., Maughan D. W. On the composition of the cytosol of relaxed skeletal muscle of the frog. Am J Physiol. 1988 May;254(5 Pt 1):C591–C604. doi: 10.1152/ajpcell.1988.254.5.C591. [DOI] [PubMed] [Google Scholar]
- Harkins A. B., Kurebayashi N., Baylor S. M. Resting myoplasmic free calcium in frog skeletal muscle fibers estimated with fluo-3. Biophys J. 1993 Aug;65(2):865–881. doi: 10.1016/S0006-3495(93)81112-3. [DOI] [PMC free article] [PubMed] [Google Scholar]
- Herrmann-Frank A., Varsányi M. Enhancement of Ca2+ release channel activity by phosphorylation of the skeletal muscle ryanodine receptor. FEBS Lett. 1993 Oct 18;332(3):237–242. doi: 10.1016/0014-5793(93)80640-g. [DOI] [PubMed] [Google Scholar]
- Hymel L., Inui M., Fleischer S., Schindler H. Purified ryanodine receptor of skeletal muscle sarcoplasmic reticulum forms Ca2+-activated oligomeric Ca2+ channels in planar bilayers. Proc Natl Acad Sci U S A. 1988 Jan;85(2):441–445. doi: 10.1073/pnas.85.2.441. [DOI] [PMC free article] [PubMed] [Google Scholar]
- Inui M., Saito A., Fleischer S. Isolation of the ryanodine receptor from cardiac sarcoplasmic reticulum and identity with the feet structures. J Biol Chem. 1987 Nov 15;262(32):15637–15642. [PubMed] [Google Scholar]
- Inui M., Saito A., Fleischer S. Purification of the ryanodine receptor and identity with feet structures of junctional terminal cisternae of sarcoplasmic reticulum from fast skeletal muscle. J Biol Chem. 1987 Feb 5;262(4):1740–1747. [PubMed] [Google Scholar]
- Inui M., Wang S., Saito A., Fleischer S. Characterization of junctional and longitudinal sarcoplasmic reticulum from heart muscle. J Biol Chem. 1988 Aug 5;263(22):10843–10850. [PubMed] [Google Scholar]
- Kijima Y., Saito A., Jetton T. L., Magnuson M. A., Fleischer S. Different intracellular localization of inositol 1,4,5-trisphosphate and ryanodine receptors in cardiomyocytes. J Biol Chem. 1993 Feb 15;268(5):3499–3506. [PubMed] [Google Scholar]
- LOWRY O. H., ROSEBROUGH N. J., FARR A. L., RANDALL R. J. Protein measurement with the Folin phenol reagent. J Biol Chem. 1951 Nov;193(1):265–275. [PubMed] [Google Scholar]
- Laemmli U. K. Cleavage of structural proteins during the assembly of the head of bacteriophage T4. Nature. 1970 Aug 15;227(5259):680–685. doi: 10.1038/227680a0. [DOI] [PubMed] [Google Scholar]
- Lai F. A., Erickson H. P., Rousseau E., Liu Q. Y., Meissner G. Purification and reconstitution of the calcium release channel from skeletal muscle. Nature. 1988 Jan 28;331(6154):315–319. doi: 10.1038/331315a0. [DOI] [PubMed] [Google Scholar]
- Leddy J. J., Murphy B. J., Qu-Yi, Doucet J. P., Pratt C., Tuana B. S. A 60 kDa polypeptide of skeletal-muscle sarcoplasmic reticulum is a calmodulin-dependent protein kinase that associates with and phosphorylates several membrane proteins. Biochem J. 1993 Nov 1;295(Pt 3):849–856. doi: 10.1042/bj2950849. [DOI] [PMC free article] [PubMed] [Google Scholar]
- Lindsay A. R., Williams A. J. Functional characterisation of the ryanodine receptor purified from sheep cardiac muscle sarcoplasmic reticulum. Biochim Biophys Acta. 1991 Apr 26;1064(1):89–102. doi: 10.1016/0005-2736(91)90415-5. [DOI] [PubMed] [Google Scholar]
- Liu Q. Y., Lai F. A., Rousseau E., Jones R. V., Meissner G. Multiple conductance states of the purified calcium release channel complex from skeletal sarcoplasmic reticulum. Biophys J. 1989 Mar;55(3):415–424. doi: 10.1016/S0006-3495(89)82835-8. [DOI] [PMC free article] [PubMed] [Google Scholar]
- McGrew S. G., Wolleben C., Siegl P., Inui M., Fleischer S. Positive cooperativity of ryanodine binding to the calcium release channel of sarcoplasmic reticulum from heart and skeletal muscle. Biochemistry. 1989 Feb 21;28(4):1686–1691. doi: 10.1021/bi00430a039. [DOI] [PubMed] [Google Scholar]
- Meissner G., Darling E., Eveleth J. Kinetics of rapid Ca2+ release by sarcoplasmic reticulum. Effects of Ca2+, Mg2+, and adenine nucleotides. Biochemistry. 1986 Jan 14;25(1):236–244. doi: 10.1021/bi00349a033. [DOI] [PubMed] [Google Scholar]
- Morii H., Takisawa H., Yamamoto T. A possible role of protein phosphorylation in the inactivation of a Ca2+-induced Ca2+ release channel from skeletal muscle sarcoplasmic reticulum. J Biochem. 1987 Aug;102(2):263–271. doi: 10.1093/oxfordjournals.jbchem.a122050. [DOI] [PubMed] [Google Scholar]
- Morii H., Tonomura Y. The gating behavior of a channel for Ca2+-induced Ca2+ release in fragmented sarcoplasmic reticulum. J Biochem. 1983 May;93(5):1271–1285. doi: 10.1093/oxfordjournals.jbchem.a134261. [DOI] [PubMed] [Google Scholar]
- Reuter H. Calcium channel modulation by neurotransmitters, enzymes and drugs. Nature. 1983 Feb 17;301(5901):569–574. doi: 10.1038/301569a0. [DOI] [PubMed] [Google Scholar]
- Rios E., Brum G. Involvement of dihydropyridine receptors in excitation-contraction coupling in skeletal muscle. Nature. 1987 Feb 19;325(6106):717–720. doi: 10.1038/325717a0. [DOI] [PubMed] [Google Scholar]
- Romani A., Scarpa A. Regulation of cell magnesium. Arch Biochem Biophys. 1992 Oct;298(1):1–12. doi: 10.1016/0003-9861(92)90086-c. [DOI] [PubMed] [Google Scholar]
- Rousseau E., Pinkos J., Savaria D. Functional sensitivity of the native skeletal Ca(2+)-release channel to divalent cations and the Mg-ATP complex. Can J Physiol Pharmacol. 1992 Mar;70(3):394–402. doi: 10.1139/y92-049. [DOI] [PubMed] [Google Scholar]
- Saito A., Seiler S., Chu A., Fleischer S. Preparation and morphology of sarcoplasmic reticulum terminal cisternae from rabbit skeletal muscle. J Cell Biol. 1984 Sep;99(3):875–885. doi: 10.1083/jcb.99.3.875. [DOI] [PMC free article] [PubMed] [Google Scholar]
- Schulman H. Phosphorylation of microtubule-associated proteins by a Ca2+/calmodulin-dependent protein kinase. J Cell Biol. 1984 Jul;99(1 Pt 1):11–19. doi: 10.1083/jcb.99.1.11. [DOI] [PMC free article] [PubMed] [Google Scholar]
- Smith J. S., Coronado R., Meissner G. Single channel measurements of the calcium release channel from skeletal muscle sarcoplasmic reticulum. Activation by Ca2+ and ATP and modulation by Mg2+. J Gen Physiol. 1986 Nov;88(5):573–588. doi: 10.1085/jgp.88.5.573. [DOI] [PMC free article] [PubMed] [Google Scholar]
- Smith J. S., Imagawa T., Ma J., Fill M., Campbell K. P., Coronado R. Purified ryanodine receptor from rabbit skeletal muscle is the calcium-release channel of sarcoplasmic reticulum. J Gen Physiol. 1988 Jul;92(1):1–26. doi: 10.1085/jgp.92.1.1. [DOI] [PMC free article] [PubMed] [Google Scholar]
- Somlyo A. V., Gonzalez-Serratos H. G., Shuman H., McClellan G., Somlyo A. P. Calcium release and ionic changes in the sarcoplasmic reticulum of tetanized muscle: an electron-probe study. J Cell Biol. 1981 Sep;90(3):577–594. doi: 10.1083/jcb.90.3.577. [DOI] [PMC free article] [PubMed] [Google Scholar]
- Suko J., Maurer-Fogy I., Plank B., Bertel O., Wyskovsky W., Hohenegger M., Hellmann G. Phosphorylation of serine 2843 in ryanodine receptor-calcium release channel of skeletal muscle by cAMP-, cGMP- and CaM-dependent protein kinase. Biochim Biophys Acta. 1993 Jan 17;1175(2):193–206. doi: 10.1016/0167-4889(93)90023-i. [DOI] [PubMed] [Google Scholar]
- Takasago T., Imagawa T., Furukawa K., Ogurusu T., Shigekawa M. Regulation of the cardiac ryanodine receptor by protein kinase-dependent phosphorylation. J Biochem. 1991 Jan;109(1):163–170. doi: 10.1093/oxfordjournals.jbchem.a123339. [DOI] [PubMed] [Google Scholar]
- Takasago T., Imagawa T., Shigekawa M. Phosphorylation of the cardiac ryanodine receptor by cAMP-dependent protein kinase. J Biochem. 1989 Nov;106(5):872–877. doi: 10.1093/oxfordjournals.jbchem.a122945. [DOI] [PubMed] [Google Scholar]
- Timerman A. P., Ogunbumni E., Freund E., Wiederrecht G., Marks A. R., Fleischer S. The calcium release channel of sarcoplasmic reticulum is modulated by FK-506-binding protein. Dissociation and reconstitution of FKBP-12 to the calcium release channel of skeletal muscle sarcoplasmic reticulum. J Biol Chem. 1993 Nov 5;268(31):22992–22999. [PubMed] [Google Scholar]
- Tsien R. W., Bean B. P., Hess P., Lansman J. B., Nilius B., Nowycky M. C. Mechanisms of calcium channel modulation by beta-adrenergic agents and dihydropyridine calcium agonists. J Mol Cell Cardiol. 1986 Jul;18(7):691–710. doi: 10.1016/s0022-2828(86)80941-5. [DOI] [PubMed] [Google Scholar]
- Wagenknecht T., Grassucci R., Frank J., Saito A., Inui M., Fleischer S. Three-dimensional architecture of the calcium channel/foot structure of sarcoplasmic reticulum. Nature. 1989 Mar 9;338(6211):167–170. doi: 10.1038/338167a0. [DOI] [PubMed] [Google Scholar]
- Walton P. D., Airey J. A., Sutko J. L., Beck C. F., Mignery G. A., Südhof T. C., Deerinck T. J., Ellisman M. H. Ryanodine and inositol trisphosphate receptors coexist in avian cerebellar Purkinje neurons. J Cell Biol. 1991 Jun;113(5):1145–1157. doi: 10.1083/jcb.113.5.1145. [DOI] [PMC free article] [PubMed] [Google Scholar]
- Wang J., Best P. M. Inactivation of the sarcoplasmic reticulum calcium channel by protein kinase. Nature. 1992 Oct 22;359(6397):739–741. doi: 10.1038/359739a0. [DOI] [PubMed] [Google Scholar]
- Witcher D. R., Kovacs R. J., Schulman H., Cefali D. C., Jones L. R. Unique phosphorylation site on the cardiac ryanodine receptor regulates calcium channel activity. J Biol Chem. 1991 Jun 15;266(17):11144–11152. [PubMed] [Google Scholar]
- Yoshida A., Takahashi M., Imagawa T., Shigekawa M., Takisawa H., Nakamura T. Phosphorylation of ryanodine receptors in rat myocytes during beta-adrenergic stimulation. J Biochem. 1992 Feb;111(2):186–190. doi: 10.1093/oxfordjournals.jbchem.a123735. [DOI] [PubMed] [Google Scholar]
- Zhang A. J., Bai G., Deans-Zirattu S., Browner M. F., Lee E. Y. Expression of the catalytic subunit of phosphorylase phosphatase (protein phosphatase-1) in Escherichia coli. J Biol Chem. 1992 Jan 25;267(3):1484–1490. [PubMed] [Google Scholar]