Abstract
The myosin lattice spacing of single intact muscle fibers of the frog, Rana temporaria, was studied in Ringer's solution (standard osmolarity 230 mOsm) and hyper- and hypotonic salines (1.4 and 0.8 times standard osmolarity respectively) in the relaxed state, during "fixed end" tetani, and during shortening, using synchrotron radiation. At standard tonicity, a tetanus was associated with an initial brief lattice expansion (and a small amount of sarcomere shortening), followed by a slow compression (unaccompanied by sarcomere length changes). In hypertonic saline (myosin lattice compressed by 8.1%), these spacing changes were suppressed, in hypotonic saline (lattice spacing increased by 7.5%), they were enhanced. During unloaded shortening of activated fibers, a rapid lattice expansion occurred at all tonicities, but became larger as tonicity was reduced. This expansion was caused in part by the change in length of the preparation, but also by a recoil of a stressed radial compliance associated with axial force. The lattice spacing during unloaded shortening was equal to or occasionally greater than predicted for a relaxed fiber at that sarcomere length, indicating that the lattice compression associated with activation is rapidly reversed upon loss of axial force. Lattice recompression occurred upon termination of shortening under standard and hypotonic conditions, but was almost absent under hypertonic conditions. These observations indicate that axial cross-bridge tension is associated with a compressive radial force in intact muscle fibers at full overlap; however, this radial force exhibits a much greater sensitivity to lattice spacing than does the axial force.
Full text
PDF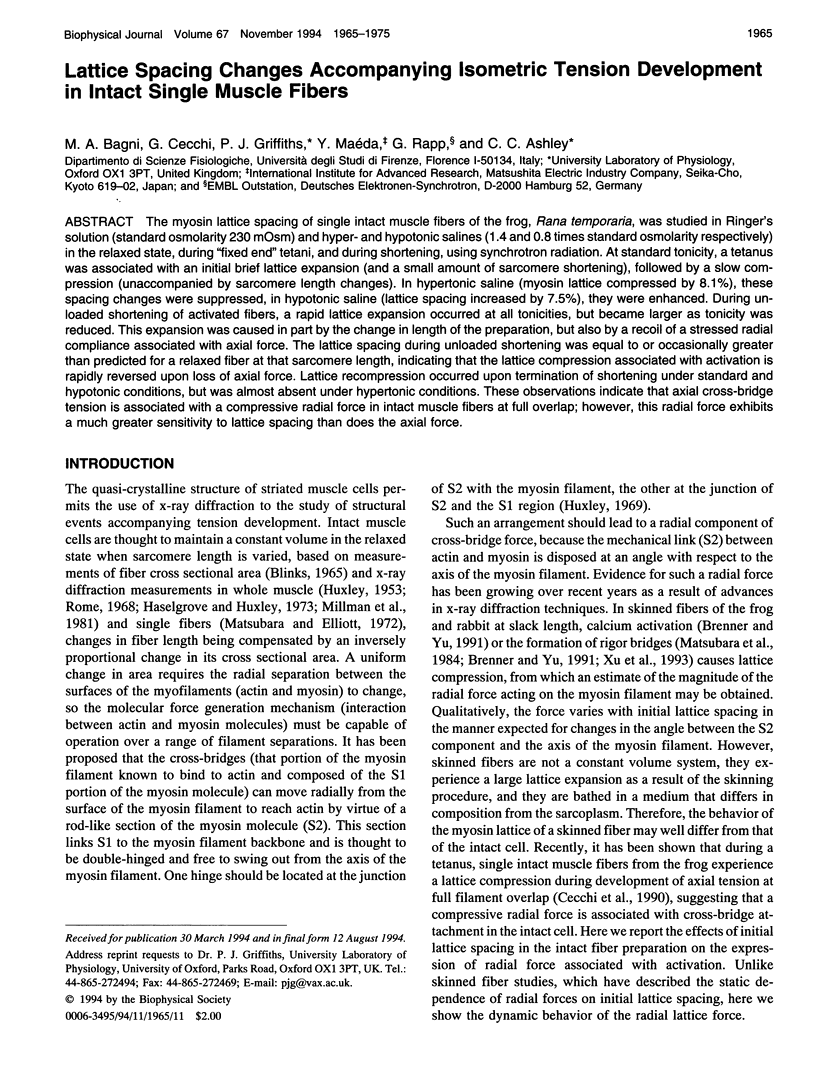
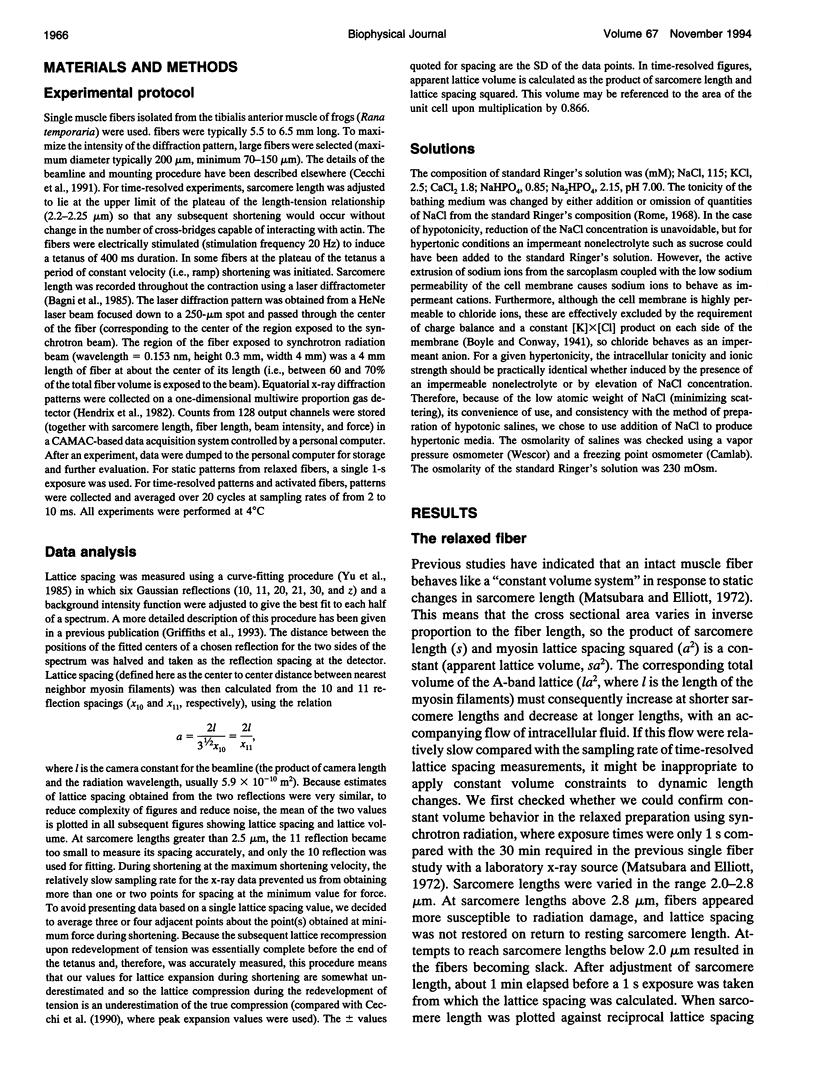
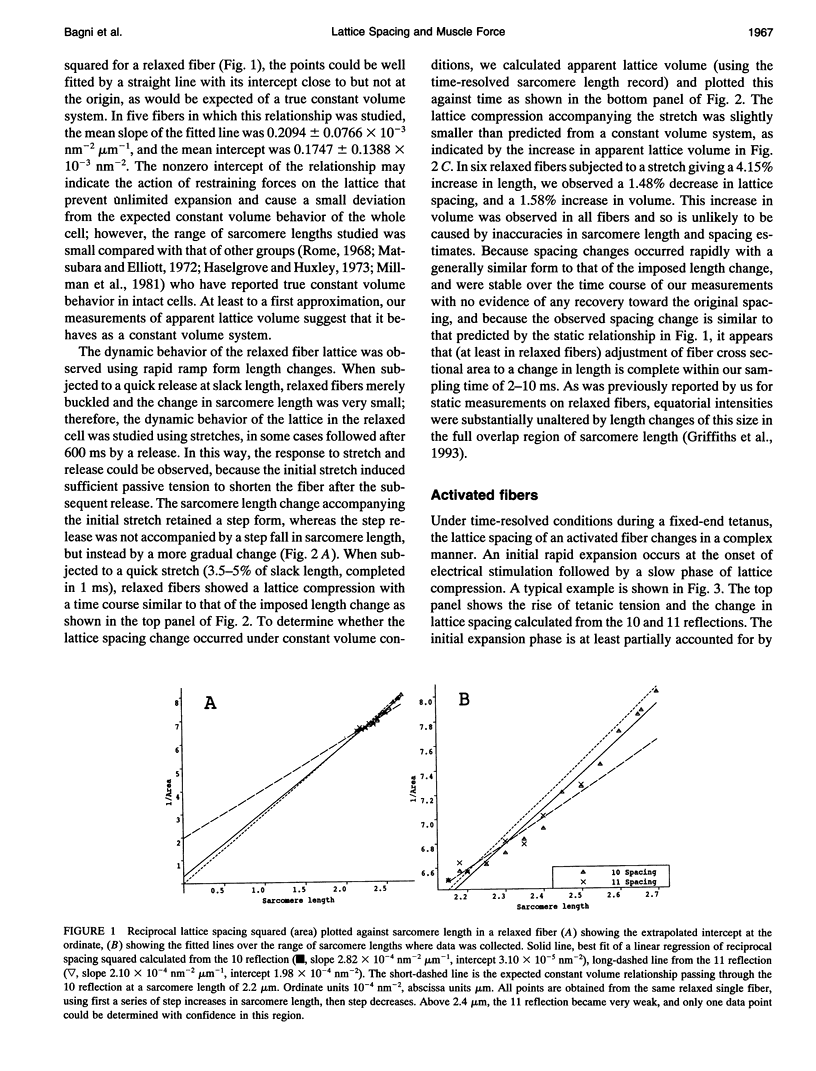
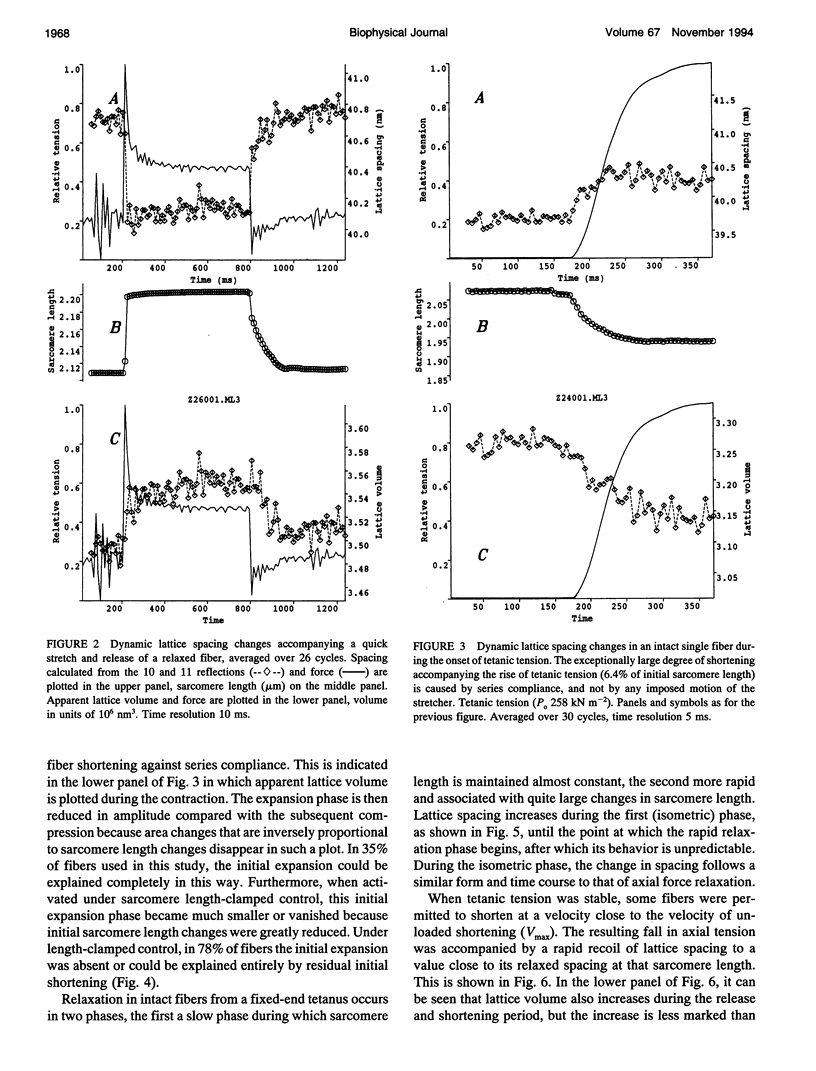
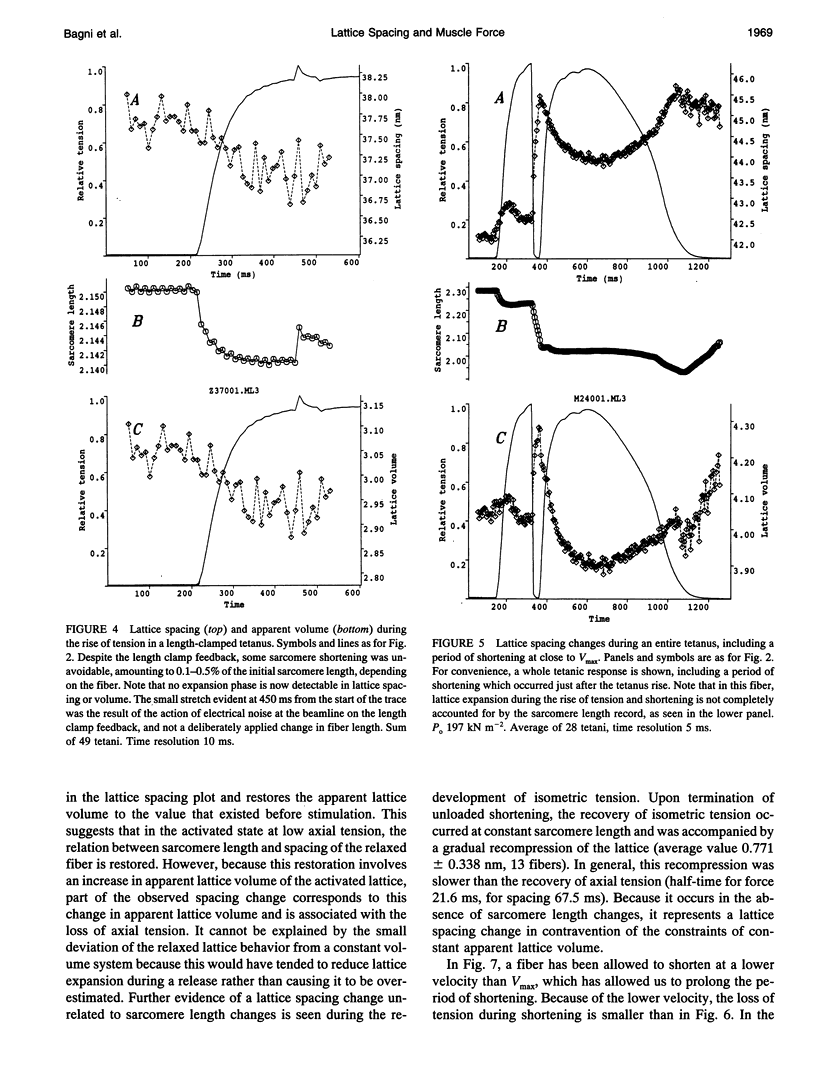
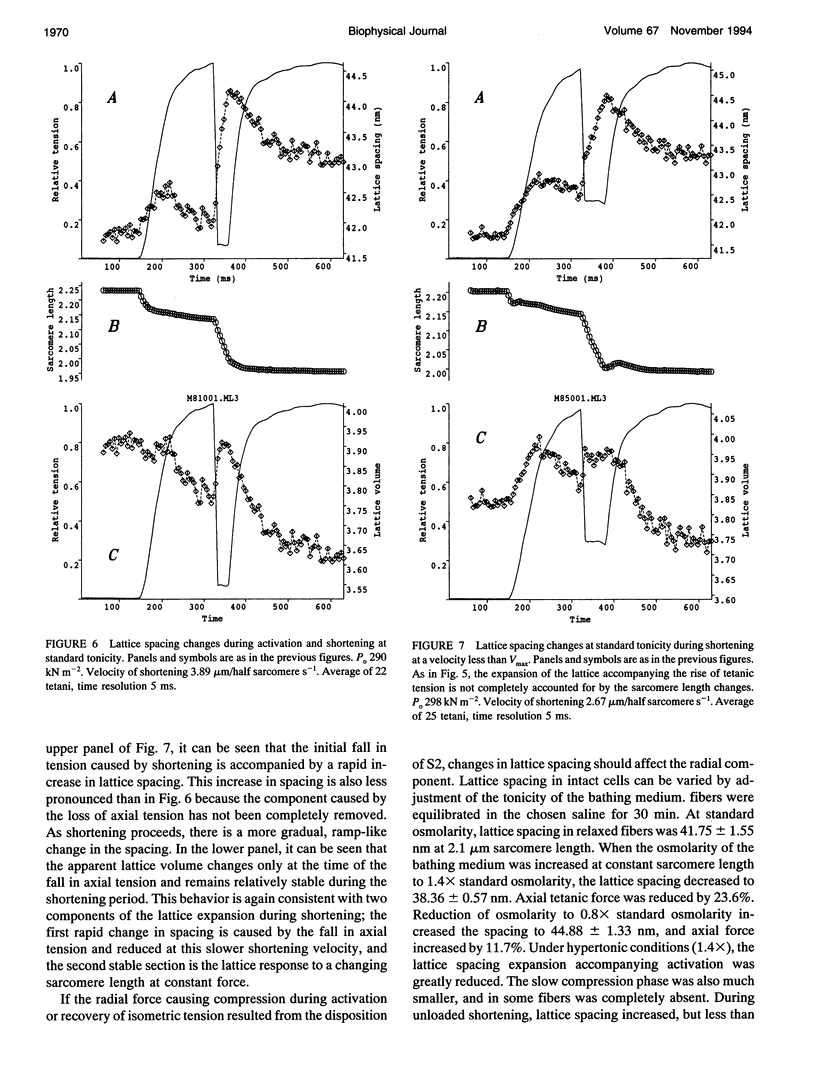
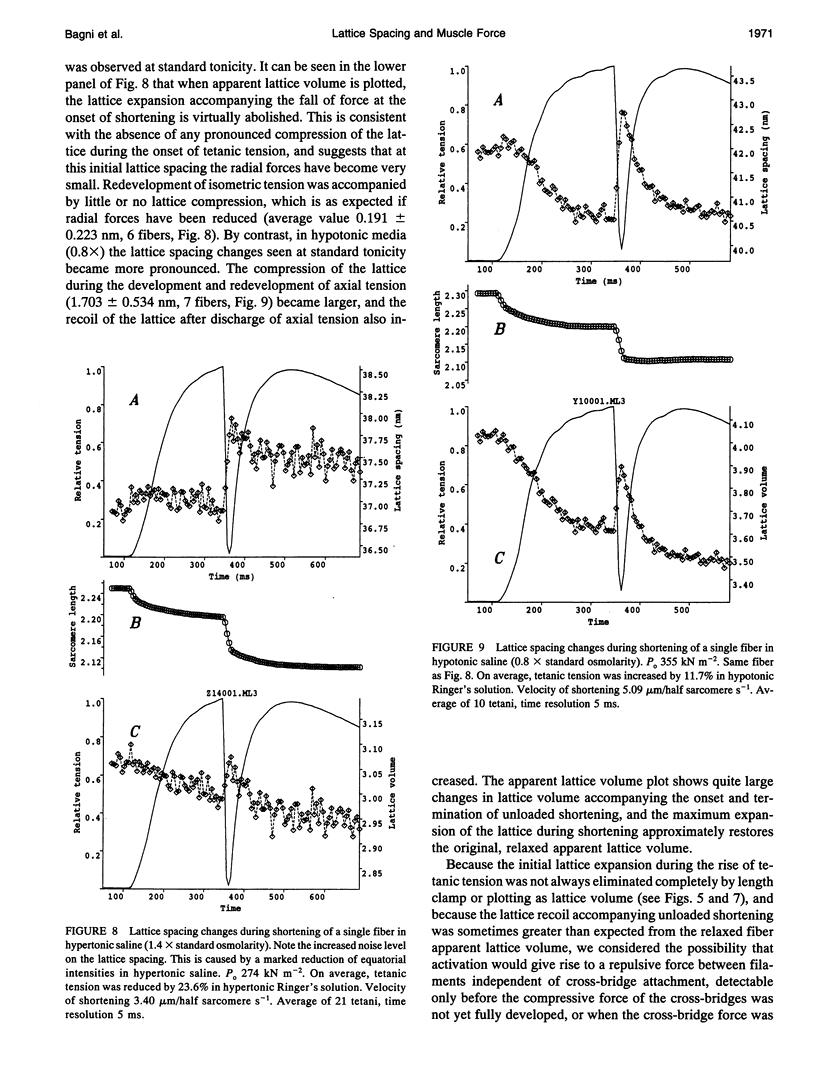
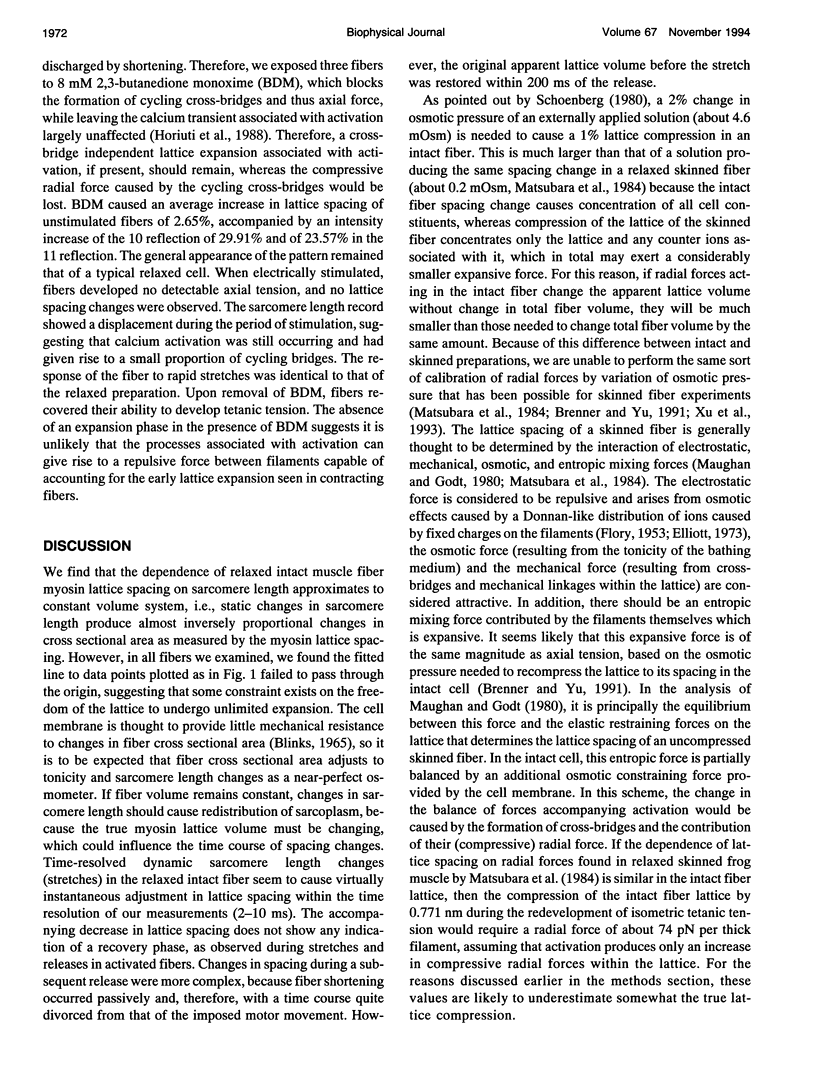
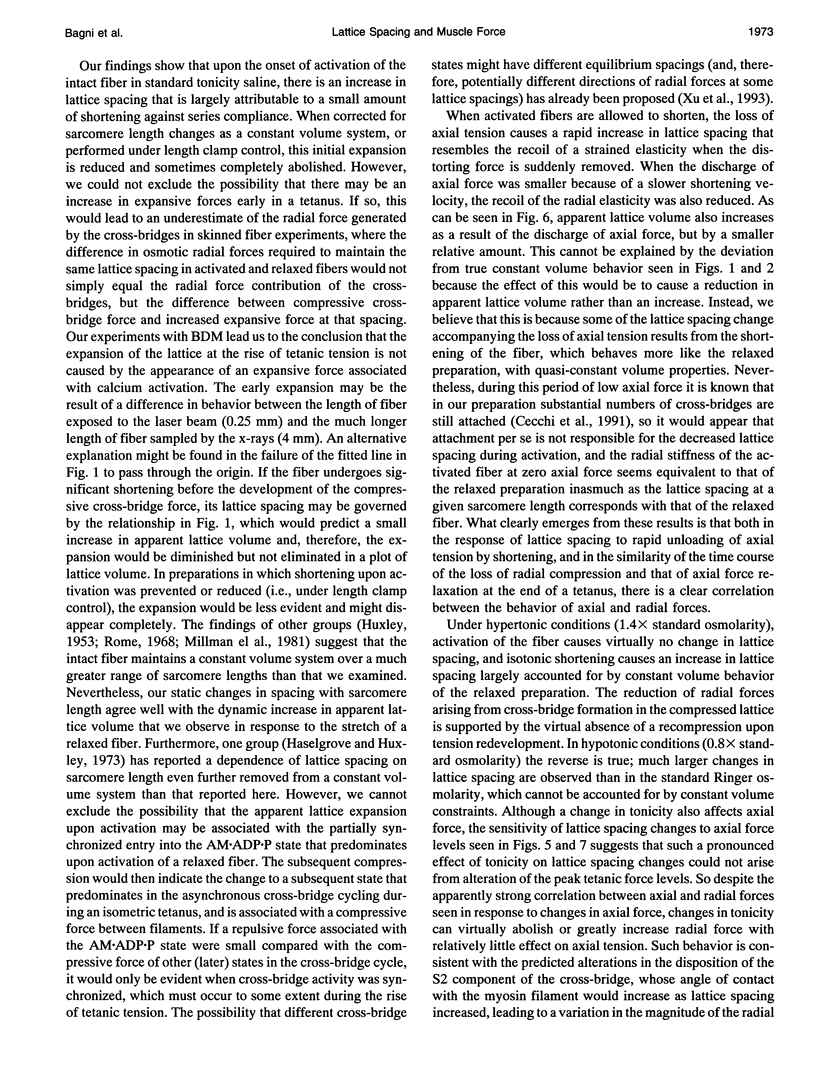
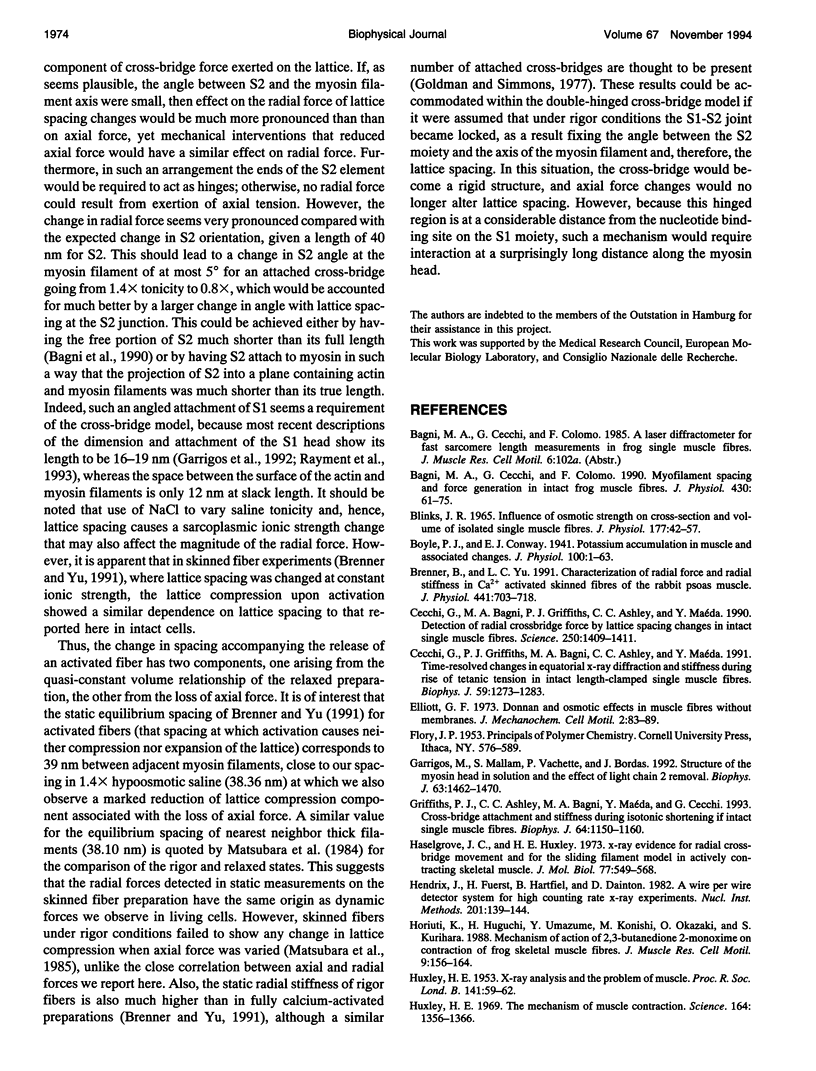
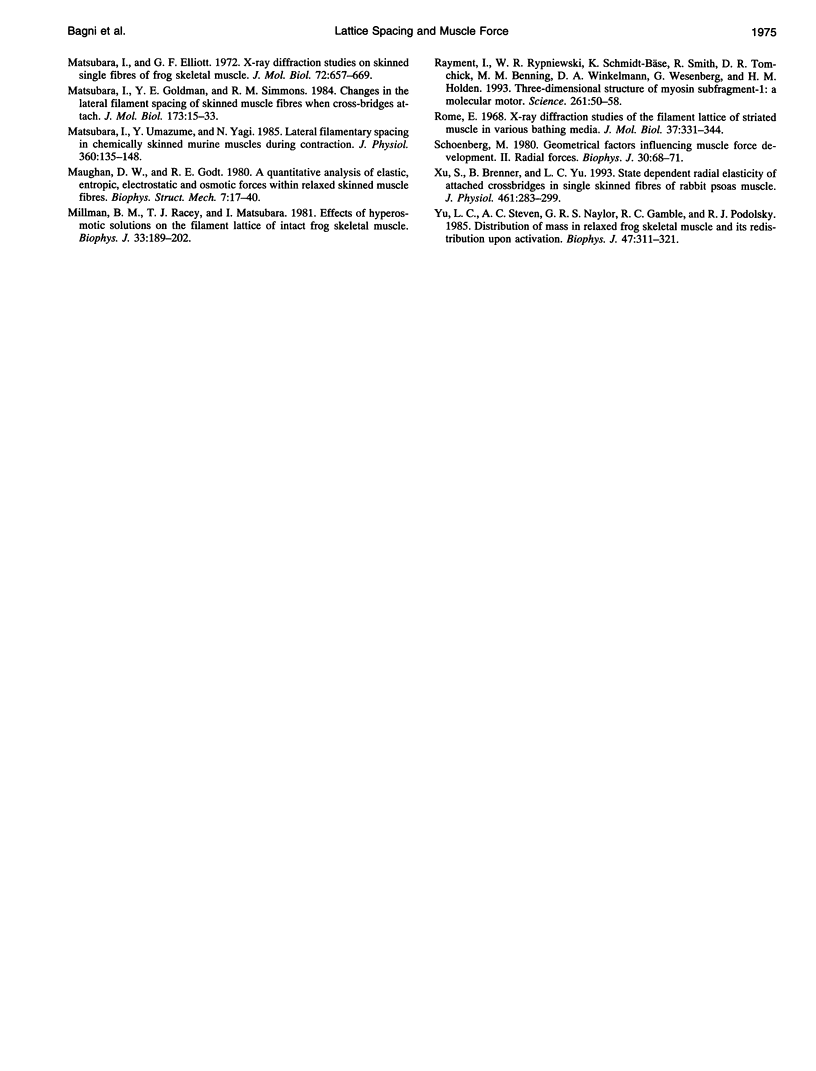
Selected References
These references are in PubMed. This may not be the complete list of references from this article.
- BLINKS J. R. INFLUENCE OF OSMOTIC STRENGTH ON CROSS-SECTION AND VOLUME OF ISOLATED SINGLE MUSCLE FIBRES. J Physiol. 1965 Mar;177:42–57. doi: 10.1113/jphysiol.1965.sp007574. [DOI] [PMC free article] [PubMed] [Google Scholar]
- Bagni M. A., Cecchi G., Colomo F. Myofilament spacing and force generation in intact frog muscle fibres. J Physiol. 1990 Nov;430:61–75. doi: 10.1113/jphysiol.1990.sp018281. [DOI] [PMC free article] [PubMed] [Google Scholar]
- Boyle P. J., Conway E. J. Potassium accumulation in muscle and associated changes. J Physiol. 1941 Aug 11;100(1):1–63. doi: 10.1113/jphysiol.1941.sp003922. [DOI] [PMC free article] [PubMed] [Google Scholar]
- Brenner B., Yu L. C. Characterization of radial force and radial stiffness in Ca(2+)-activated skinned fibres of the rabbit psoas muscle. J Physiol. 1991 Sep;441:703–718. doi: 10.1113/jphysiol.1991.sp018774. [DOI] [PMC free article] [PubMed] [Google Scholar]
- Cecchi G., Bagni M. A., Griffiths P. J., Ashley C. C., Maeda Y. Detection of radial crossbridge force by lattice spacing changes in intact single muscle fibers. Science. 1990 Dec 7;250(4986):1409–1411. doi: 10.1126/science.2255911. [DOI] [PubMed] [Google Scholar]
- Cecchi G., Griffiths P. J., Bagni M. A., Ashley C. C., Maeda Y. Time-resolved changes in equatorial x-ray diffraction and stiffness during rise of tetanic tension in intact length-clamped single muscle fibers. Biophys J. 1991 Jun;59(6):1273–1283. doi: 10.1016/S0006-3495(91)82342-6. [DOI] [PMC free article] [PubMed] [Google Scholar]
- Elliott G. F. Donnan and osmotic effects in muscle fibres without membranes. J Mechanochem Cell Motil. 1973 May;2(1):83–89. [PubMed] [Google Scholar]
- Garrigos M., Mallam S., Vachette P., Bordas J. Structure of the myosin head in solution and the effect of light chain 2 removal. Biophys J. 1992 Dec;63(6):1462–1470. doi: 10.1016/S0006-3495(92)81743-5. [DOI] [PMC free article] [PubMed] [Google Scholar]
- Griffiths P. J., Ashley C. C., Bagni M. A., Maéda Y., Cecchi G. Cross-bridge attachment and stiffness during isotonic shortening of intact single muscle fibers. Biophys J. 1993 Apr;64(4):1150–1160. doi: 10.1016/S0006-3495(93)81481-4. [DOI] [PMC free article] [PubMed] [Google Scholar]
- HUXLEY H. E. X-ray analysis and the problem of muscle. Proc R Soc Lond B Biol Sci. 1953 Mar 11;141(902):59–62. doi: 10.1098/rspb.1953.0017. [DOI] [PubMed] [Google Scholar]
- Haselgrove J. C., Huxley H. E. X-ray evidence for radial cross-bridge movement and for the sliding filament model in actively contracting skeletal muscle. J Mol Biol. 1973 Jul 15;77(4):549–568. doi: 10.1016/0022-2836(73)90222-2. [DOI] [PubMed] [Google Scholar]
- Horiuti K., Higuchi H., Umazume Y., Konishi M., Okazaki O., Kurihara S. Mechanism of action of 2, 3-butanedione 2-monoxime on contraction of frog skeletal muscle fibres. J Muscle Res Cell Motil. 1988 Apr;9(2):156–164. doi: 10.1007/BF01773737. [DOI] [PubMed] [Google Scholar]
- Huxley H. E. The mechanism of muscular contraction. Science. 1969 Jun 20;164(3886):1356–1365. doi: 10.1126/science.164.3886.1356. [DOI] [PubMed] [Google Scholar]
- Matsubara I., Elliott G. F. X-ray diffraction studies on skinned single fibres of frog skeletal muscle. J Mol Biol. 1972 Dec 30;72(3):657–669. doi: 10.1016/0022-2836(72)90183-0. [DOI] [PubMed] [Google Scholar]
- Matsubara I., Goldman Y. E., Simmons R. M. Changes in the lateral filament spacing of skinned muscle fibres when cross-bridges attach. J Mol Biol. 1984 Feb 15;173(1):15–33. doi: 10.1016/0022-2836(84)90401-7. [DOI] [PubMed] [Google Scholar]
- Matsubara I., Umazume Y., Yagi N. Lateral filamentary spacing in chemically skinned murine muscles during contraction. J Physiol. 1985 Mar;360:135–148. doi: 10.1113/jphysiol.1985.sp015608. [DOI] [PMC free article] [PubMed] [Google Scholar]
- Maughan D. W., Godt R. E. A quantitative analysis of elastic, entropic, electrostatic, and osmotic forces within relaxed skinned muscle fibers. Biophys Struct Mech. 1980;7(1):17–40. doi: 10.1007/BF00538156. [DOI] [PubMed] [Google Scholar]
- Millman B. M., Racey T. J., Matsubara I. Effects of hyperosmotic solutions on the filament lattice of intact frog skeletal muscle. Biophys J. 1981 Feb;33(2):189–202. doi: 10.1016/S0006-3495(81)84880-1. [DOI] [PMC free article] [PubMed] [Google Scholar]
- Rayment I., Rypniewski W. R., Schmidt-Bäse K., Smith R., Tomchick D. R., Benning M. M., Winkelmann D. A., Wesenberg G., Holden H. M. Three-dimensional structure of myosin subfragment-1: a molecular motor. Science. 1993 Jul 2;261(5117):50–58. doi: 10.1126/science.8316857. [DOI] [PubMed] [Google Scholar]
- Rome E. X-ray diffraction studies of the filament lattice of striated muscle in various bathing media. J Mol Biol. 1968 Oct 28;37(2):331–344. doi: 10.1016/0022-2836(68)90272-6. [DOI] [PubMed] [Google Scholar]
- Xu S., Brenner B., Yu L. C. State-dependent radial elasticity of attached cross-bridges in single skinned fibres of rabbit psoas muscle. J Physiol. 1993 Feb;461:283–299. doi: 10.1113/jphysiol.1993.sp019514. [DOI] [PMC free article] [PubMed] [Google Scholar]
- Yu L. C., Steven A. C., Naylor G. R., Gamble R. C., Podolsky R. J. Distribution of mass in relaxed frog skeletal muscle and its redistribution upon activation. Biophys J. 1985 Mar;47(3):311–321. doi: 10.1016/S0006-3495(85)83921-7. [DOI] [PMC free article] [PubMed] [Google Scholar]