Abstract
Electron spin resonance (ESR) studies have been performed on spin-labeled model membranes aligned using the isopotential spin-dry ultracentrifugation (ISDU) method of Clark and Rothschild. This method relies on sedimentation of the membrane fragments onto a gravitational isopotential surface with simultaneous evaporation of the solvent in a vacuum ultracentrifuge to promote alignment. The degree of alignment obtainable using ISDU, as monitored by ESR measurements of molecular ordering for both lipid (16-PC) and cholestane spin labels (CSL), in dipalmitoylphosphatidylcholine (DPPC) model membranes compares favorably with that obtainable by pressure-annealing. The much gentler conditions under which membranes may be aligned by ISDU greatly extends the range of macroscopically aligned membrane samples that may be investigated by ESR. We report the first ESR study of an integral membrane protein, bacteriorhodopsin (BR) in well-aligned multilayers. We have also examined ISDU-aligned DPPC multilayers incorporating a short peptide gramicidin A' (GA), with higher water content than previously studied. 0.24 mol% BR/DPPC membranes with CSL probe show two distinct components, primarily in the gel phase, which can be attributed to bulk and boundary regions of the bilayer. The boundary regions show sharply decreased molecular ordering and spectral effects comparable to those observed from 2 mol% GA/DPPC membranes. The boundary regions for both BR and GA also exhibit increased fluidity as monitored by the rotational diffusion rates. The high water content of the GA/DPPC membranes reduces the disordering effect as evidenced by the reduced populations of the disordered components. The ESR spectra obtained slightly below the main phase transition of DPPC from both the peptide- and protein-containing membranes reveals a new component with increased ordering of the lipids associated with the peptide or protein. This increase coincides with a broad endothermic peak in the DSC, suggesting a disaggregation of both the peptide and the protein before the main phase transition of the lipid. Detailed simulations of the multicomponent ESR spectra have been performed by the latest nonlinear least-squares methods, which have helped to clarify the spectral interpretations. It is found that the simulations of ESR spectra from CSL in the gel phase for all the lipid membranes studied could be significantly improved by utilizing a model with CSL molecules existing as both hydrogen-bonded to the bilayer interface and non-hydrogen-bonded within the bilayer.
Full text
PDF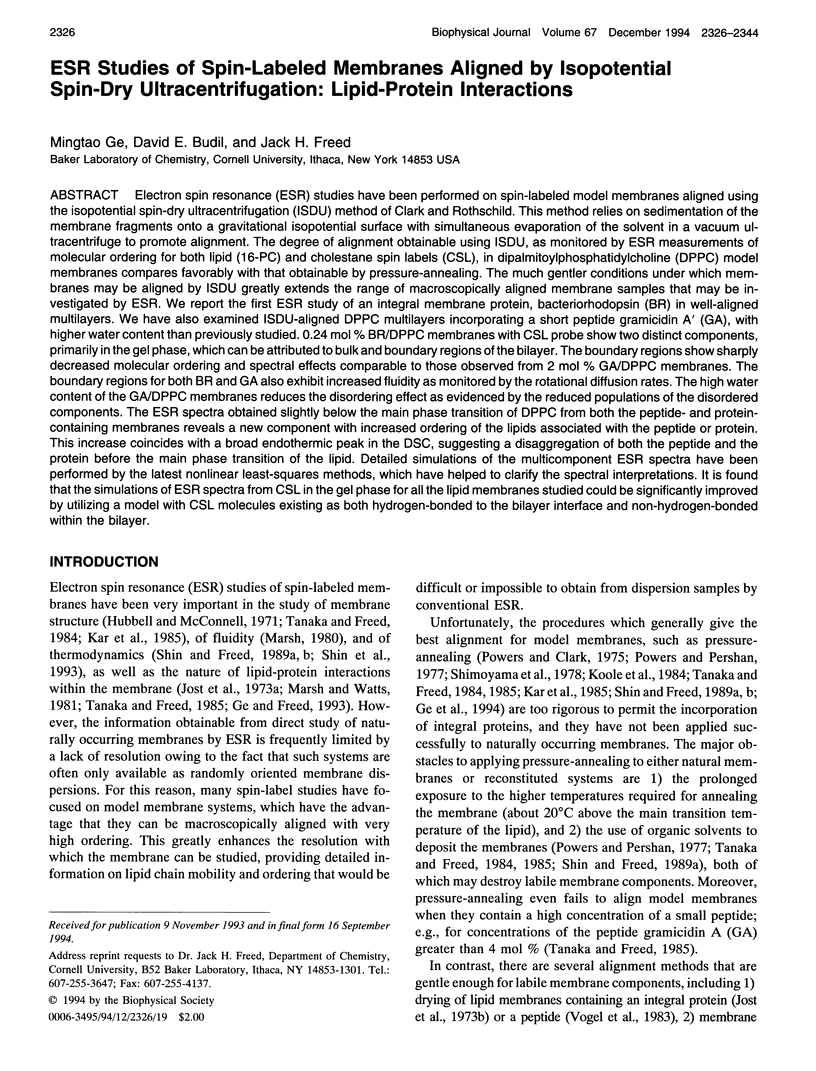
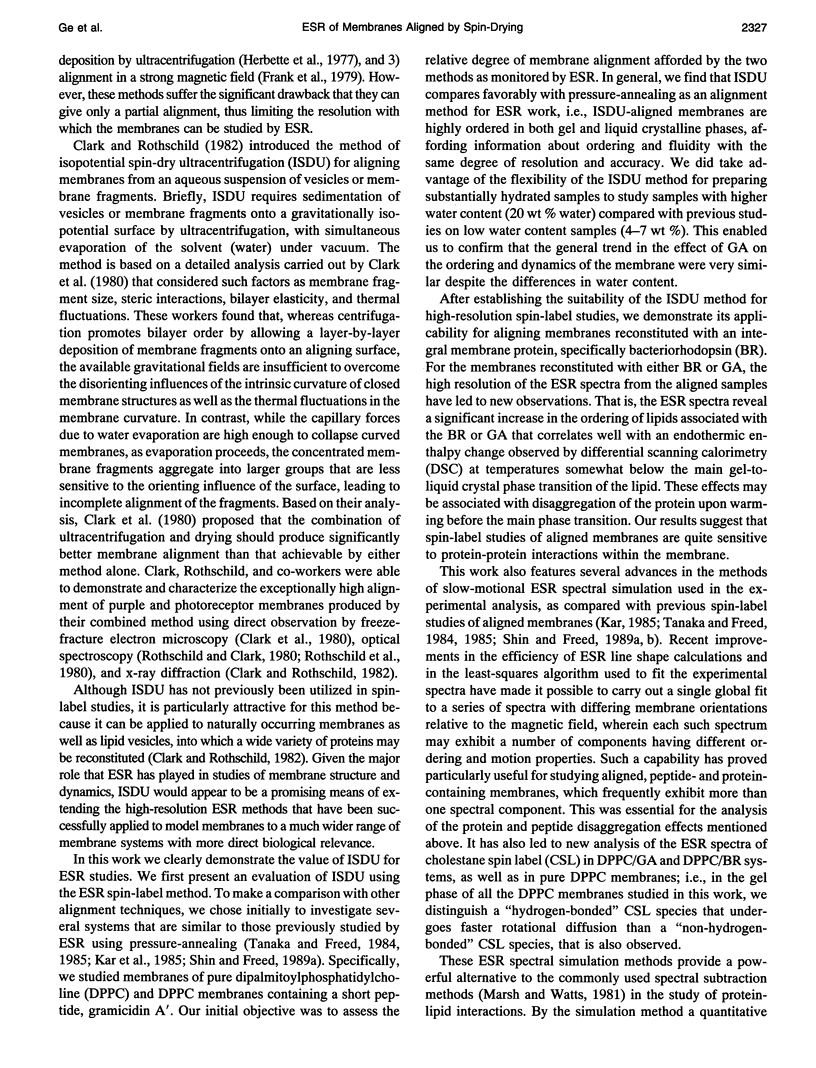
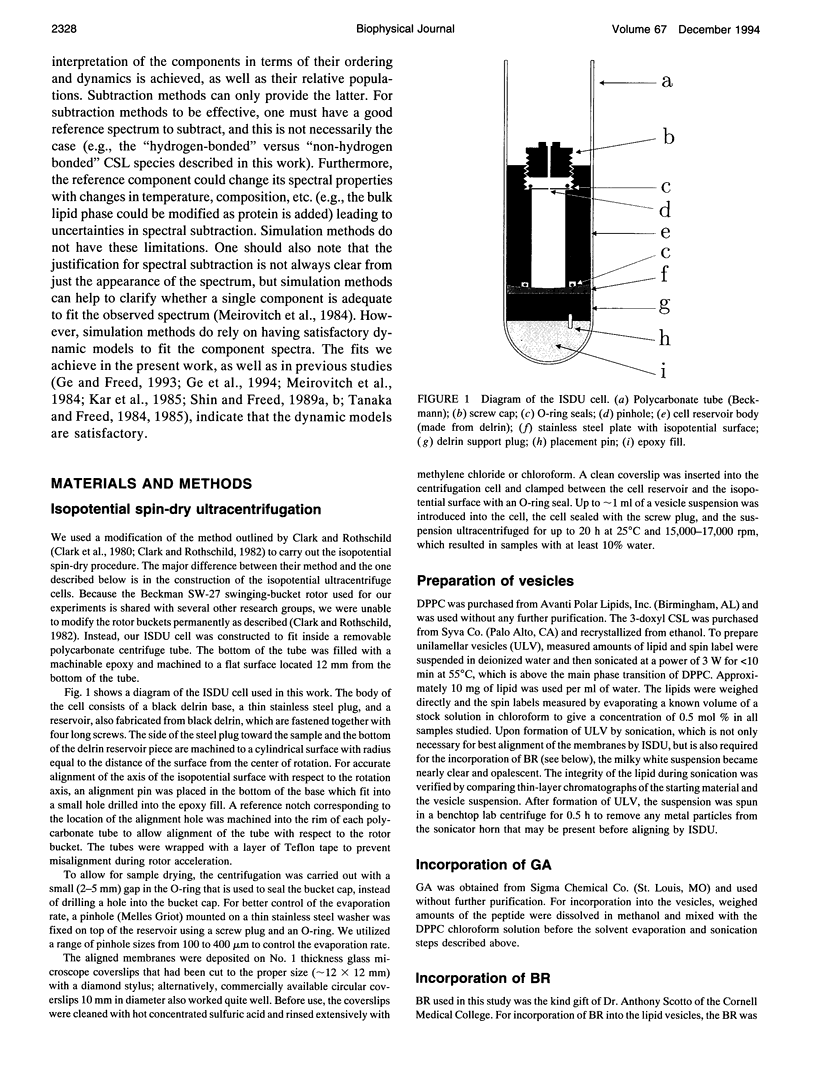
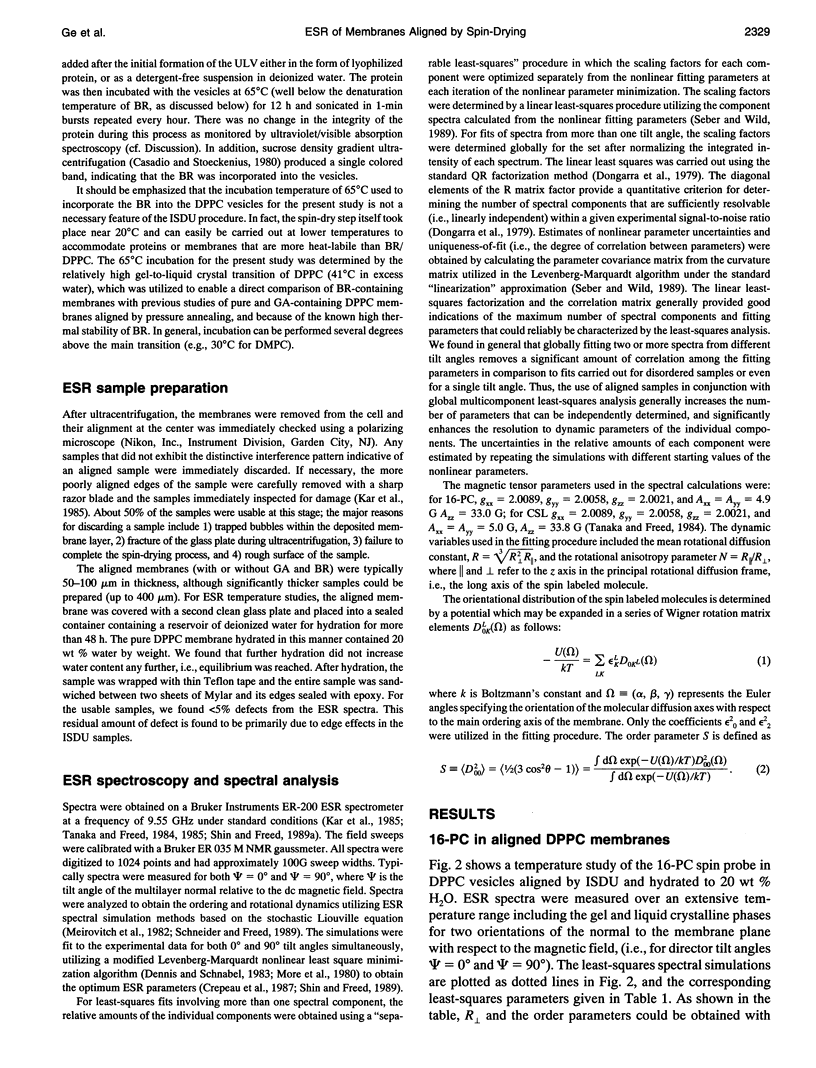
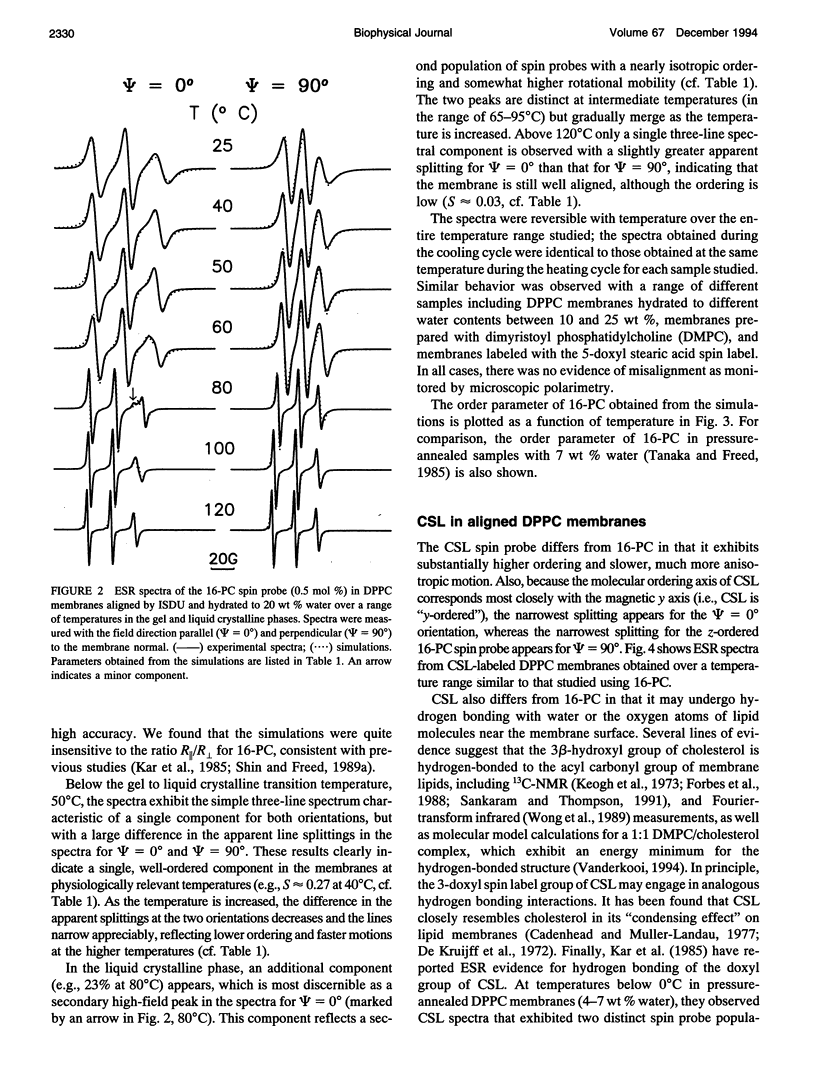
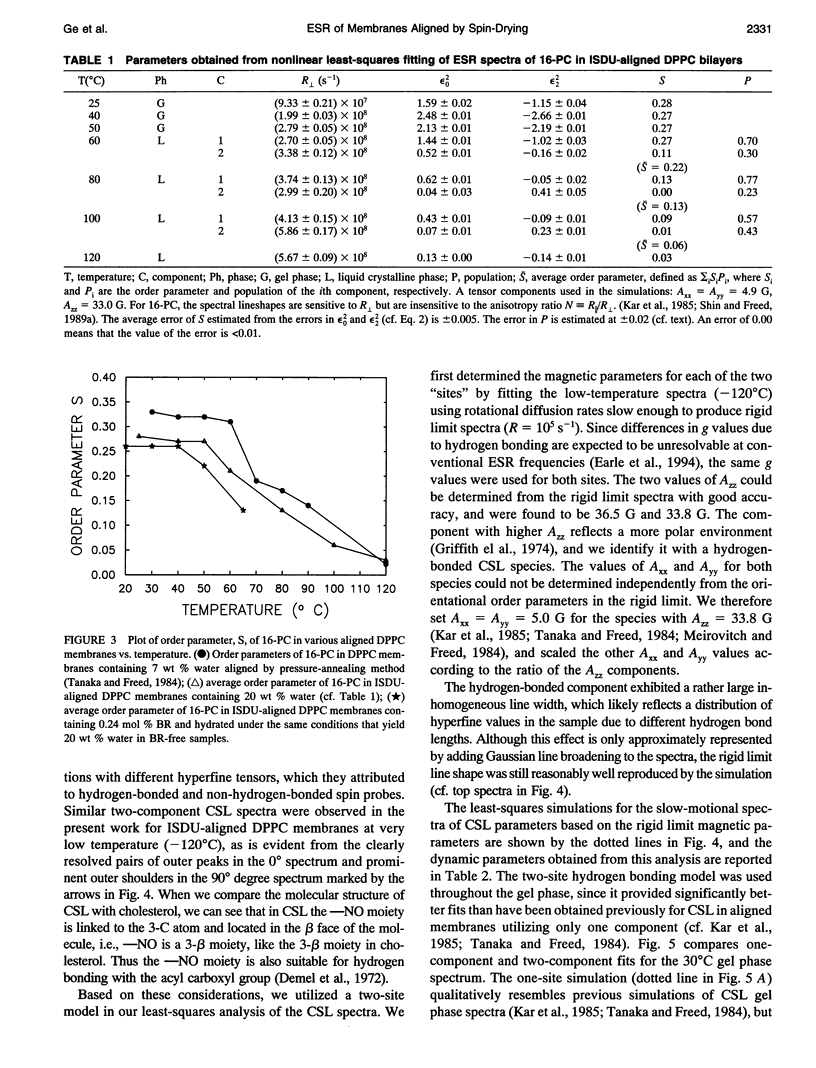
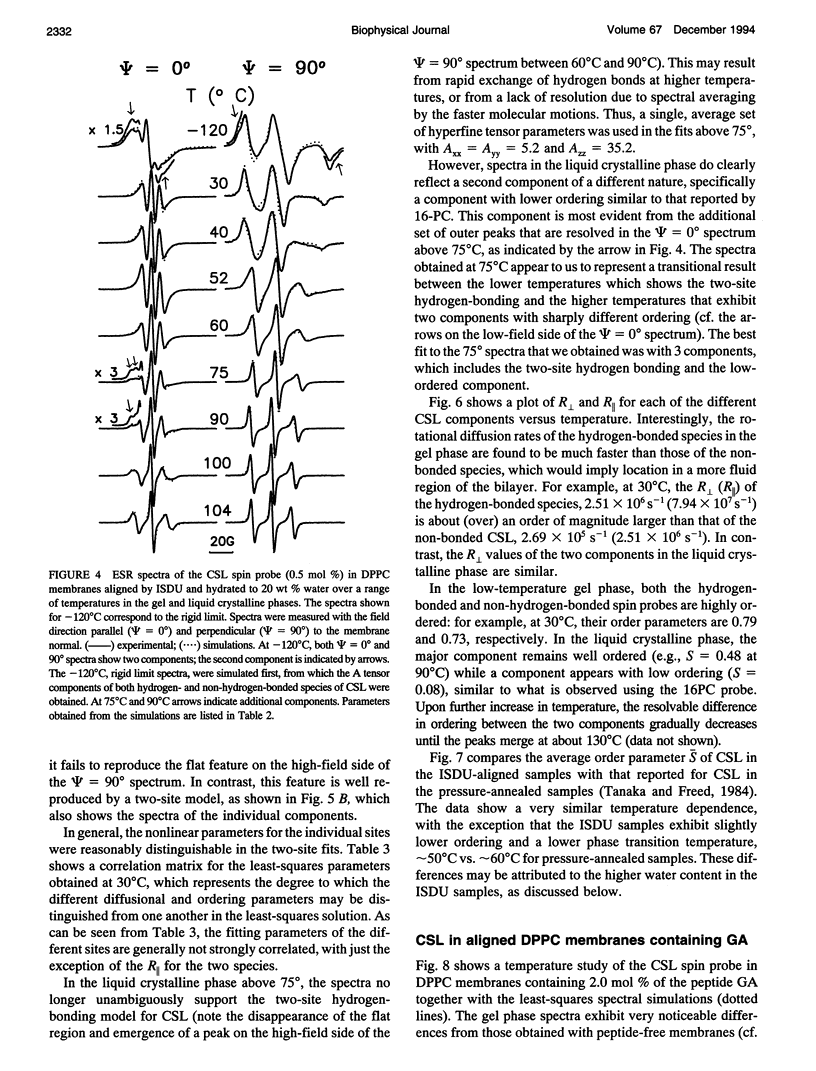
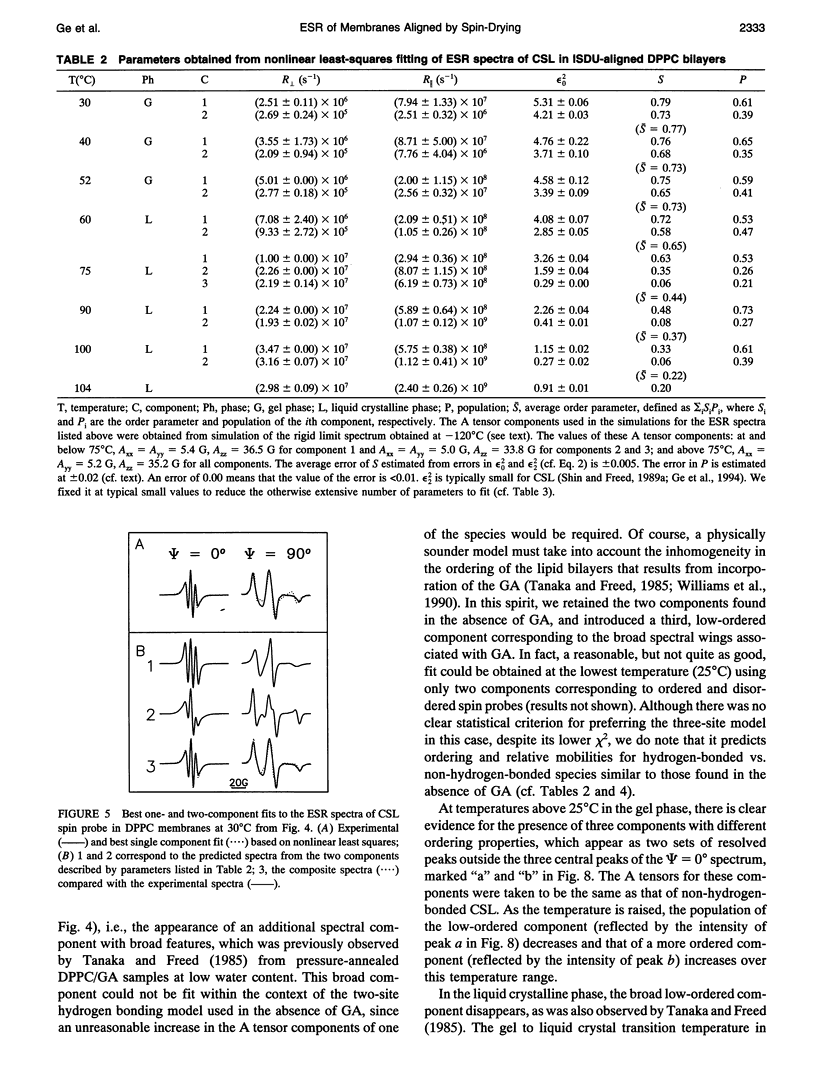
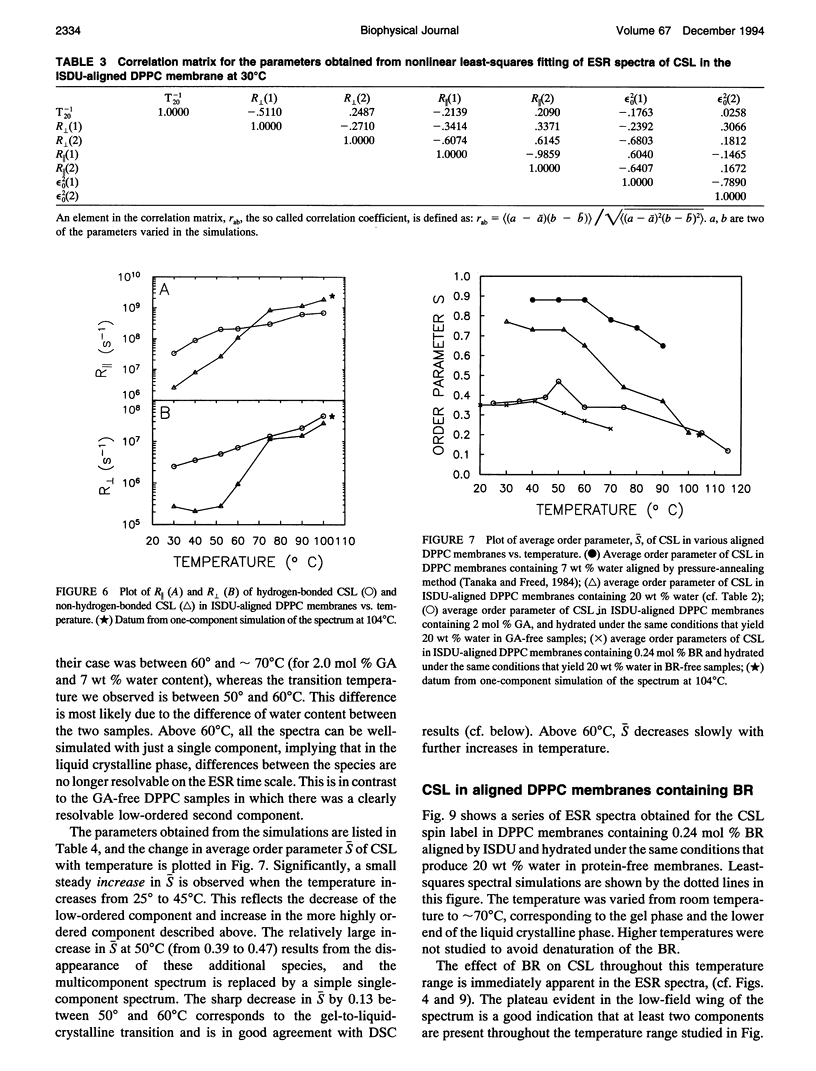
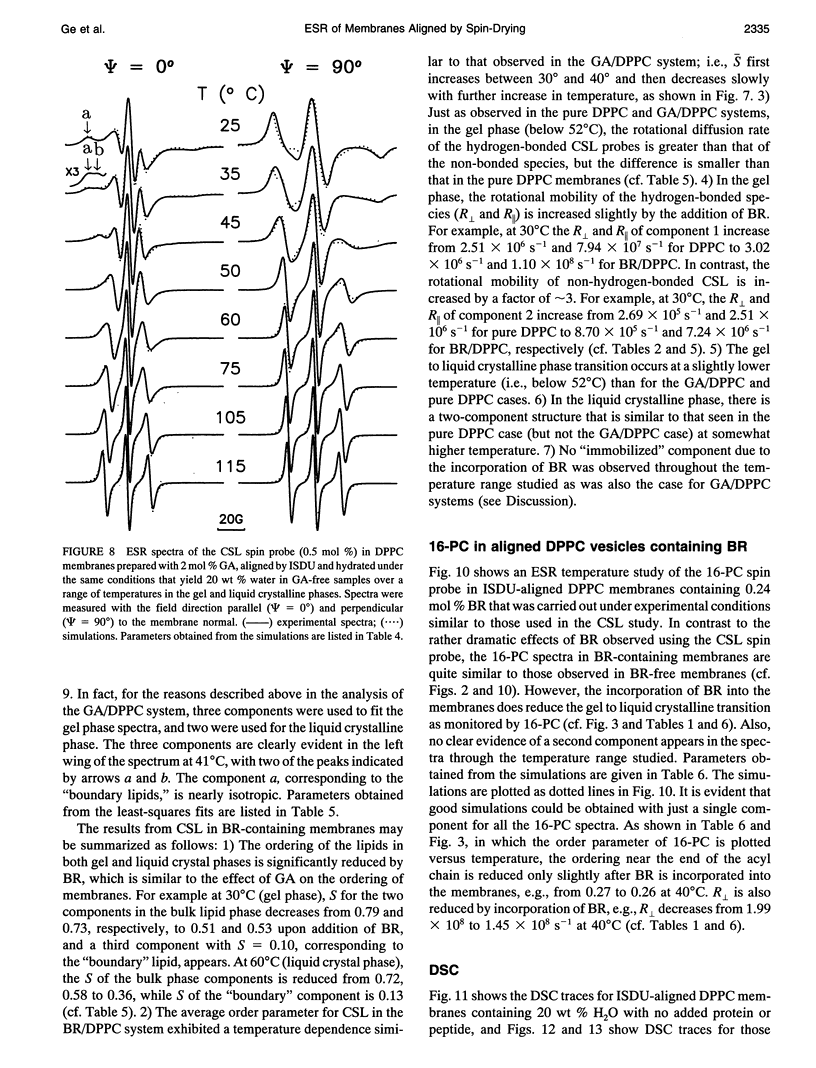
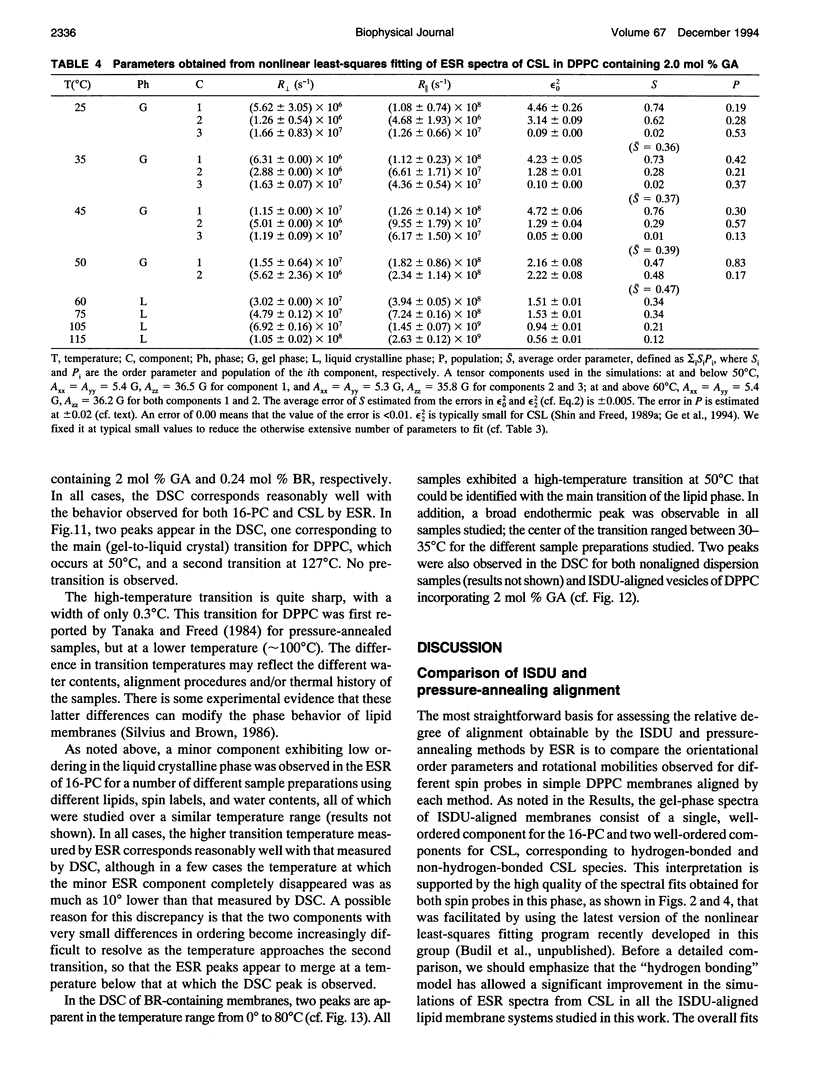
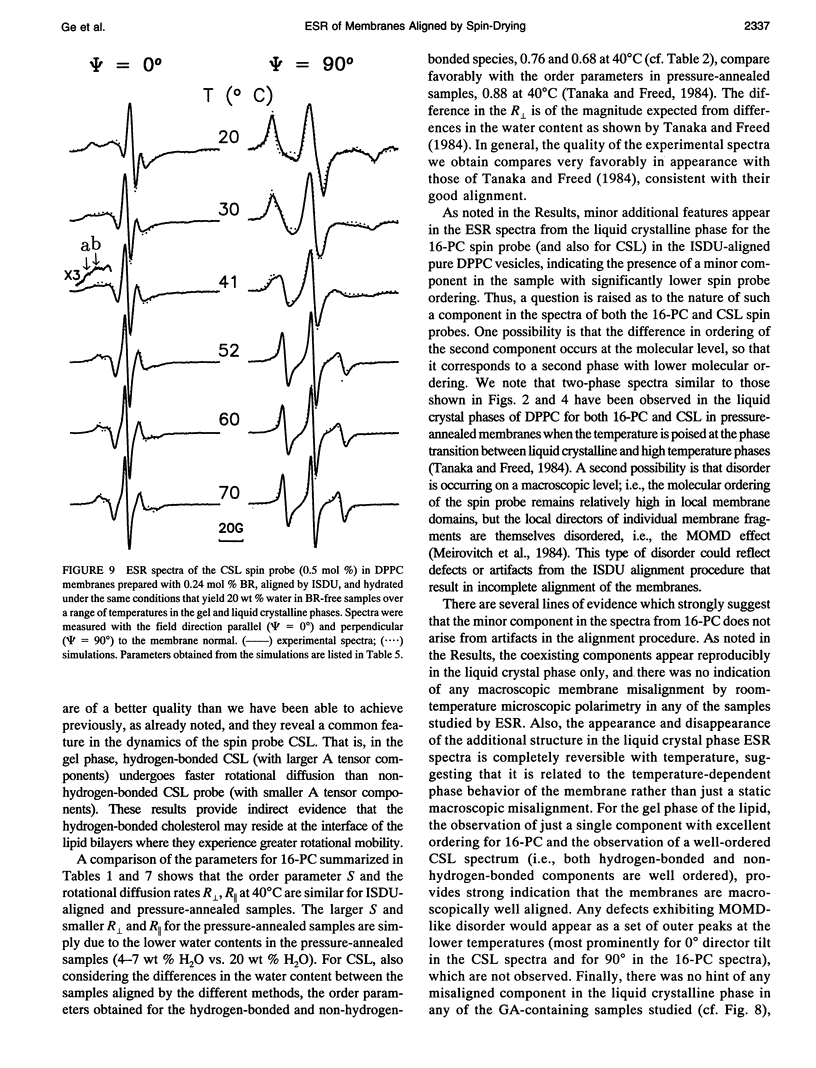
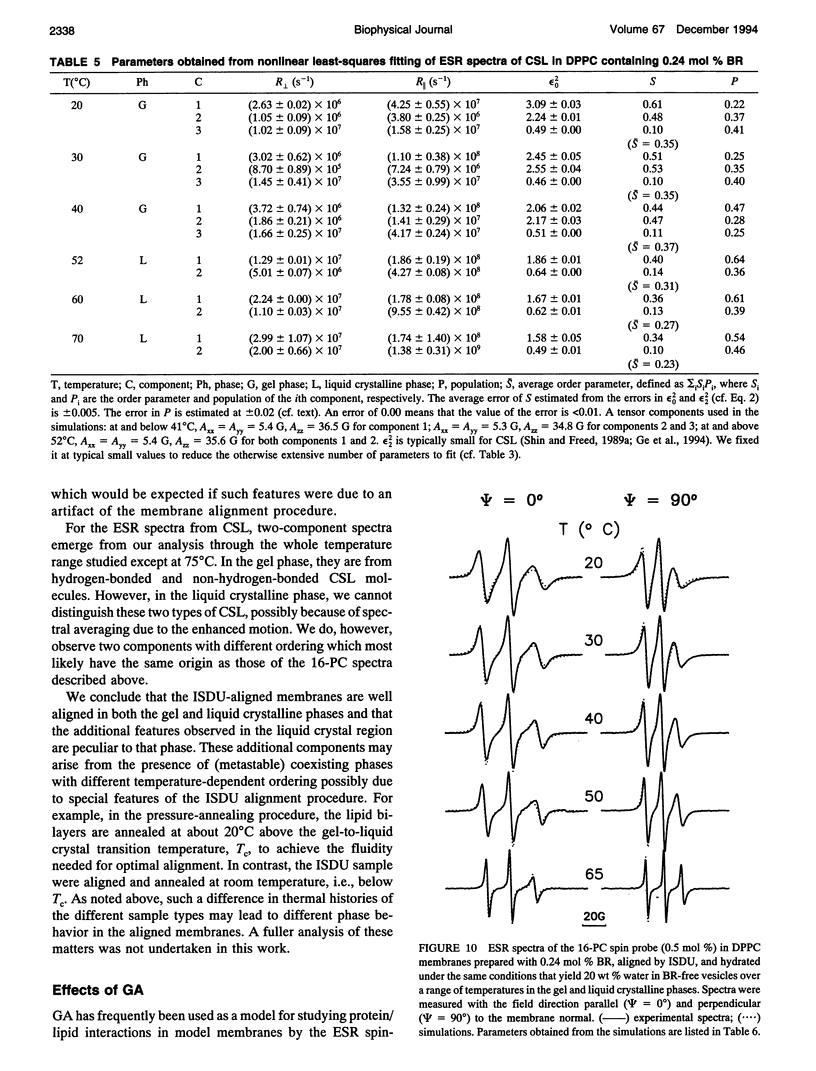
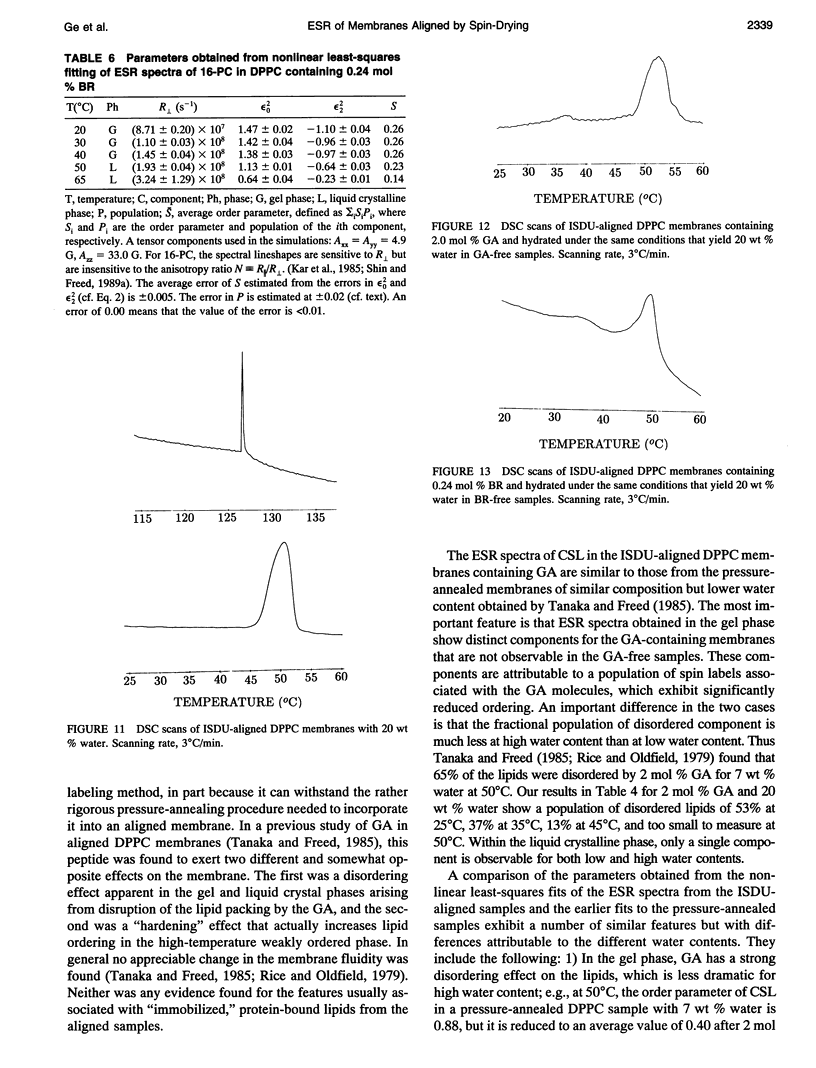
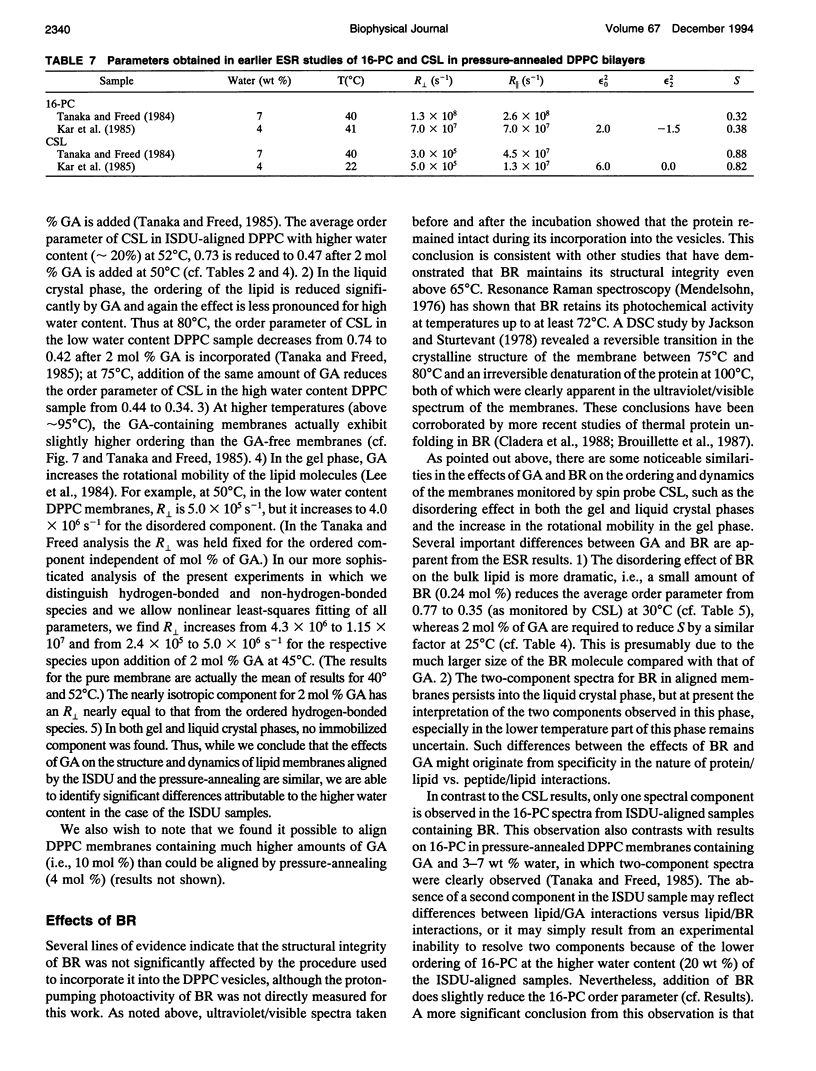
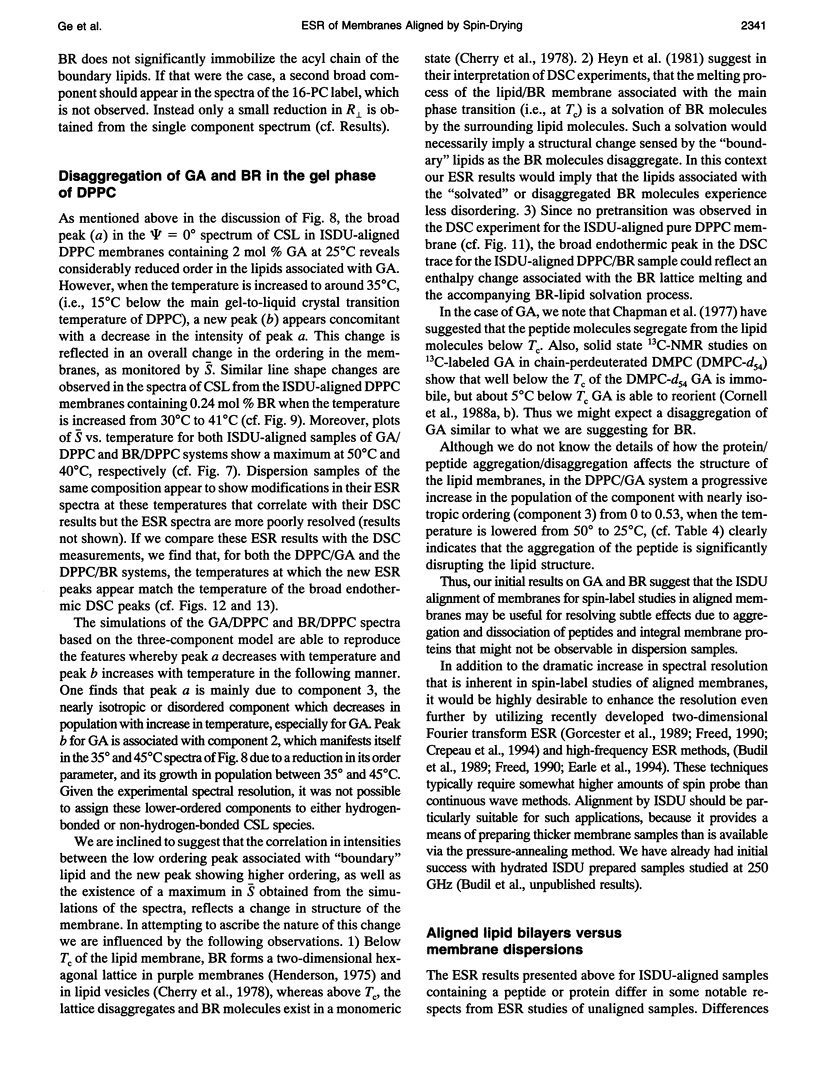
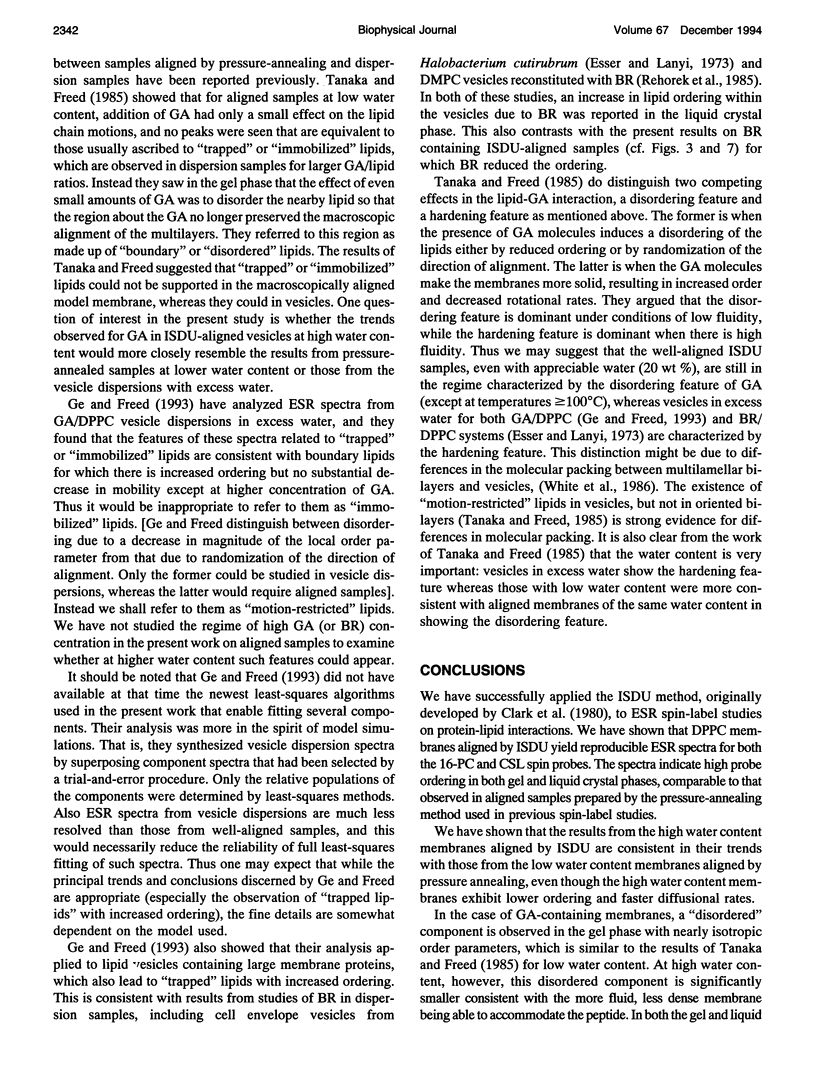
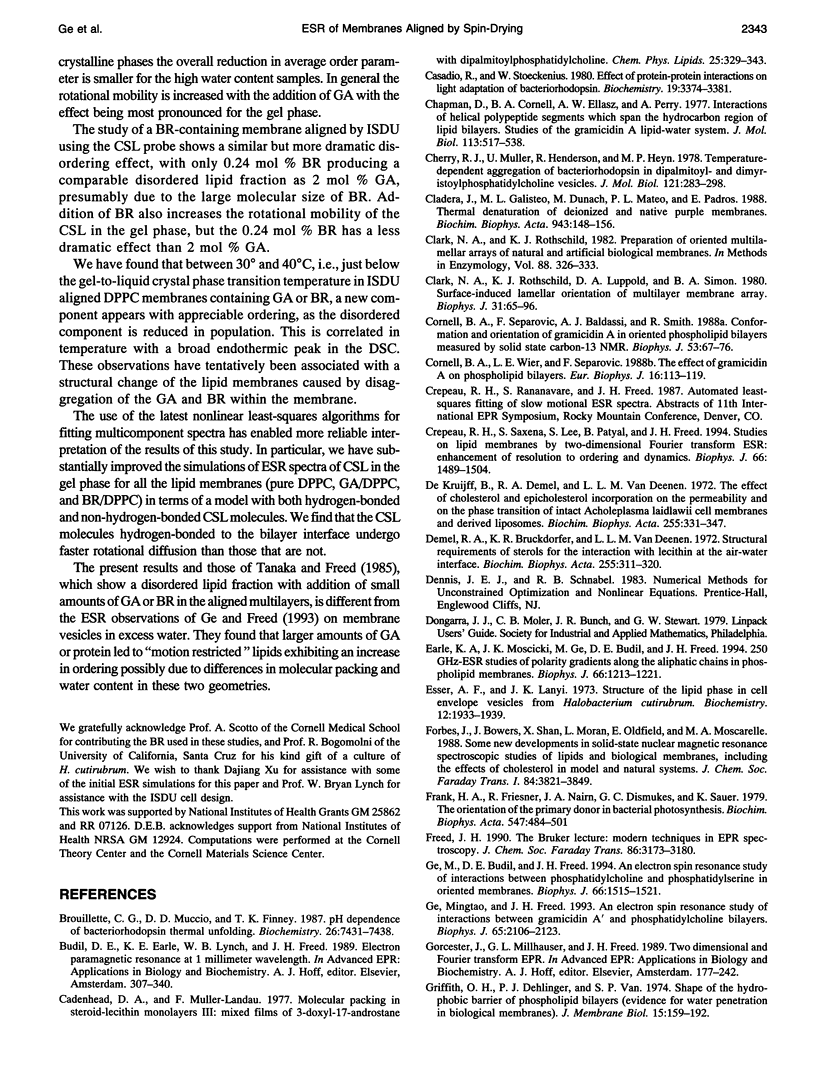
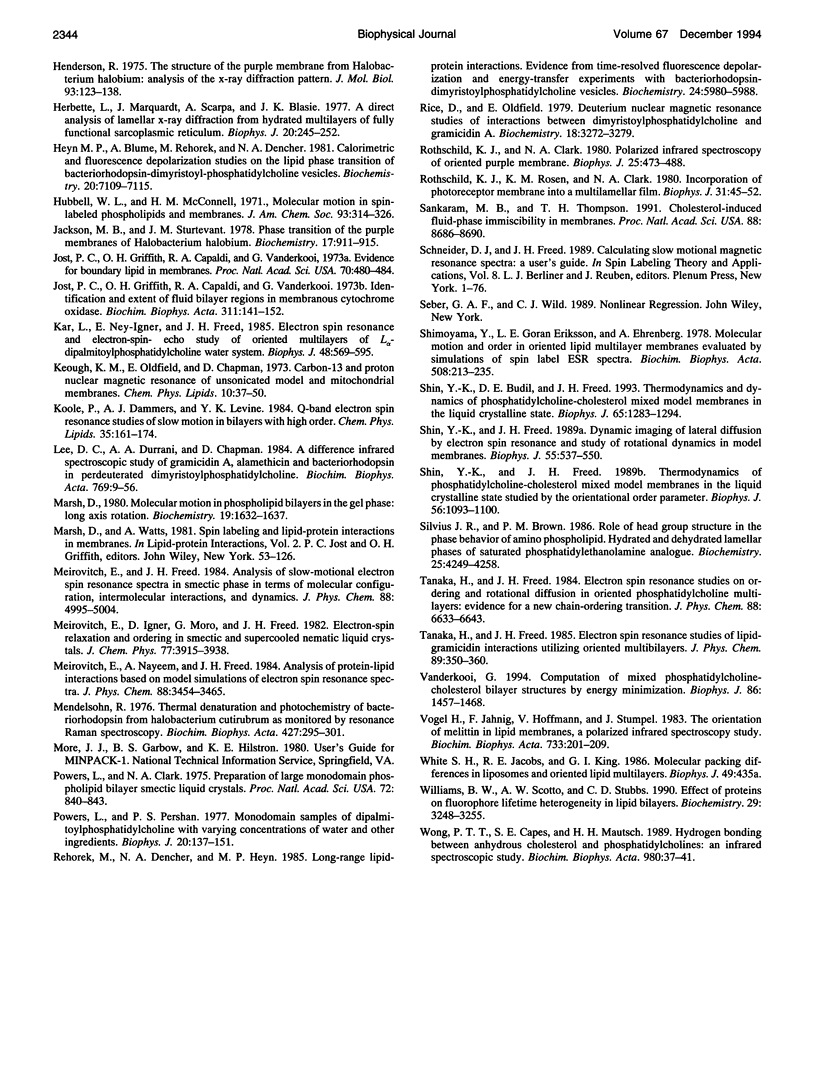
Images in this article
Selected References
These references are in PubMed. This may not be the complete list of references from this article.
- Brouillette C. G., Muccio D. D., Finney T. K. pH dependence of bacteriorhodopsin thermal unfolding. Biochemistry. 1987 Nov 17;26(23):7431–7438. doi: 10.1021/bi00397a035. [DOI] [PubMed] [Google Scholar]
- Casadio R., Stoeckenius W. Effect of protein-protein interaction on light adaptation of bacteriorhodopsin. Biochemistry. 1980 Jul 8;19(14):3374–3381. doi: 10.1021/bi00555a043. [DOI] [PubMed] [Google Scholar]
- Chapman D., Cornell B. A., Ellasz A. W., Perry A. Interactions of helical polypepetide segments which span the hydrocarbon region of lipid bilayers. Studies of the gramicidin A lipid-water system. J Mol Biol. 1977 Jul 5;113(3):517–538. doi: 10.1016/0022-2836(77)90236-4. [DOI] [PubMed] [Google Scholar]
- Cherry R. J., Müller U. Temperature-dependent aggregation of bacteriorhodopsin in dipalmitoyl- and dimyristoylphosphatidylcholine vesicles. J Mol Biol. 1978 May 15;121(2):283–298. doi: 10.1016/s0022-2836(78)80010-2. [DOI] [PubMed] [Google Scholar]
- Clark N. A., Rothschild K. J., Luippold D. A., Simon B. A. Surface-induced lamellar orientation of multilayer membrane arrays. Theoretical analysis and a new method with application to purple membrane fragments. Biophys J. 1980 Jul;31(1):65–96. doi: 10.1016/S0006-3495(80)85041-7. [DOI] [PMC free article] [PubMed] [Google Scholar]
- Cornell B. A., Separovic F., Baldassi A. J., Smith R. Conformation and orientation of gramicidin a in oriented phospholipid bilayers measured by solid state carbon-13 NMR. Biophys J. 1988 Jan;53(1):67–76. doi: 10.1016/S0006-3495(88)83066-2. [DOI] [PMC free article] [PubMed] [Google Scholar]
- Cornell B. A., Weir L. E., Separovic F. The effect of gramicidin A on phospholipid bilayers. Eur Biophys J. 1988;16(2):113–119. doi: 10.1007/BF00255521. [DOI] [PubMed] [Google Scholar]
- Crepeau R. H., Saxena S., Lee S., Patyal B., Freed J. H. Studies on lipid membranes by two-dimensional Fourier transform ESR: Enhancement of resolution to ordering and dynamics. Biophys J. 1994 May;66(5):1489–1504. doi: 10.1016/S0006-3495(94)80940-3. [DOI] [PMC free article] [PubMed] [Google Scholar]
- Demel R. A., Bruckdorfer K. R., van Deenen L. L. Structural requirements of sterols for the interaction with lecithin at the air water interface. Biochim Biophys Acta. 1972 Jan 17;255(1):311–320. doi: 10.1016/0005-2736(72)90030-2. [DOI] [PubMed] [Google Scholar]
- Earle K. A., Moscicki J. K., Ge M., Budil D. E., Freed J. H. 250-GHz electron spin resonance studies of polarity gradients along the aliphatic chains in phospholipid membranes. Biophys J. 1994 Apr;66(4):1213–1221. doi: 10.1016/S0006-3495(94)80905-1. [DOI] [PMC free article] [PubMed] [Google Scholar]
- Esser A. F., Lanyi J. K. Structure of the lipid phase in cell envelope vesicles from Halobacterium cutirubrum. Biochemistry. 1973 May 8;12(10):1933–1939. doi: 10.1021/bi00734a016. [DOI] [PubMed] [Google Scholar]
- Frank H. A., Friesner R., Nairn J. A., Dismukes G. C., Sauer K. The orientation of the primary donor in bacterial photosynthesis. Biochim Biophys Acta. 1979 Sep 11;547(3):484–501. doi: 10.1016/0005-2728(79)90029-x. [DOI] [PubMed] [Google Scholar]
- Ge M., Budil D. E., Freed J. H. An electron spin resonance study of interactions between phosphatidylcholine and phosphatidylserine in oriented membranes. Biophys J. 1994 May;66(5):1515–1521. doi: 10.1016/S0006-3495(94)80942-7. [DOI] [PMC free article] [PubMed] [Google Scholar]
- Ge M., Freed J. H. An electron spin resonance study of interactions between gramicidin A' and phosphatidylcholine bilayers. Biophys J. 1993 Nov;65(5):2106–2123. doi: 10.1016/S0006-3495(93)81255-4. [DOI] [PMC free article] [PubMed] [Google Scholar]
- Griffith O. H., Dehlinger P. J., Van S. P. Shape of the hydrophobic barrier of phospholipid bilayers (evidence for water penetration in biological membranes). J Membr Biol. 1974;15(2):159–192. doi: 10.1007/BF01870086. [DOI] [PubMed] [Google Scholar]
- Henderson R. The structure of the purple membrane from Halobacterium hallobium: analysis of the X-ray diffraction pattern. J Mol Biol. 1975 Apr 5;93(2):123–138. doi: 10.1016/0022-2836(75)90123-0. [DOI] [PubMed] [Google Scholar]
- Herbette L., Marquardt J., Scarpa A., Blasie J. K. A direct analysis of lamellar x-ray diffraction from hydrated oriented multilayers of fully functional sarcoplasmic reticulum. Biophys J. 1977 Nov;20(2):245–272. doi: 10.1016/S0006-3495(77)85547-1. [DOI] [PMC free article] [PubMed] [Google Scholar]
- Heyn M. P., Blume A., Rehorek M., Dencher N. A. Calorimetric and fluorescence depolarization studies on the lipid phase transition of bacteriorhodopsin--dimyristoylphosphatidylcholine vesicles. Biochemistry. 1981 Dec 8;20(25):7109–7115. doi: 10.1021/bi00528a009. [DOI] [PubMed] [Google Scholar]
- Hubbell W. L., McConnell H. M. Molecular motion in spin-labeled phospholipids and membranes. J Am Chem Soc. 1971 Jan 27;93(2):314–326. doi: 10.1021/ja00731a005. [DOI] [PubMed] [Google Scholar]
- Jackson M. B., Sturtevant J. M. Phase transitions of the purple membranes of Halobacterium halobium. Biochemistry. 1978 Mar 7;17(5):911–915. doi: 10.1021/bi00598a026. [DOI] [PubMed] [Google Scholar]
- Jost P. C., Griffith O. H., Capaldi R. A., Vanderkooi G. Evidence for boundary lipid in membranes. Proc Natl Acad Sci U S A. 1973 Feb;70(2):480–484. doi: 10.1073/pnas.70.2.480. [DOI] [PMC free article] [PubMed] [Google Scholar]
- Jost P., Griffith O. H., Capaldi R. A., Vanderkooi G. Identification and extent of fluid bilayer regions in membranous cytochrome oxidase. Biochim Biophys Acta. 1973 Jun 22;311(2):141–152. doi: 10.1016/0005-2736(73)90261-7. [DOI] [PubMed] [Google Scholar]
- Kar L., Ney-Igner E., Freed J. H. Electron spin resonance and electron-spin-echo study of oriented multilayers of L alpha-dipalmitoylphosphatidylcholine water systems. Biophys J. 1985 Oct;48(4):569–595. doi: 10.1016/S0006-3495(85)83814-5. [DOI] [PMC free article] [PubMed] [Google Scholar]
- Keough K. M., Oldfield E., Chapman D. Carbon-13 and proton nuclear magnetic resonance of unsonicated model and mitochondrial membranes. Chem Phys Lipids. 1973 Jan;10(1):37–50. doi: 10.1016/0009-3084(73)90039-x. [DOI] [PubMed] [Google Scholar]
- Lee D. C., Durrani A. A., Chapman D. A difference infrared spectroscopic study of gramicidin A, alamethicin and bacteriorhodopsin in perdeuterated dimyristoylphosphatidylcholine. Biochim Biophys Acta. 1984 Jan 11;769(1):49–56. doi: 10.1016/0005-2736(84)90008-7. [DOI] [PubMed] [Google Scholar]
- Marsh D. Molecular motion in phospholipid bilayers in the gel phase: long axis rotation. Biochemistry. 1980 Apr 15;19(8):1632–1637. doi: 10.1021/bi00549a017. [DOI] [PubMed] [Google Scholar]
- Mendelsohn R. Thermal denaturation and photochemistry of bacteriorhodopsin from Halobacterium cutirubrum as monitored by resonance Raman spectroscopy. Biochim Biophys Acta. 1976 Mar 18;427(1):295–301. doi: 10.1016/0005-2795(76)90305-6. [DOI] [PubMed] [Google Scholar]
- Powers L., Clark N. A. Preparation of large monodomain phospholipid bilayer smectic liquid crystals. Proc Natl Acad Sci U S A. 1975 Mar;72(3):840–843. doi: 10.1073/pnas.72.3.840. [DOI] [PMC free article] [PubMed] [Google Scholar]
- Powers L., Pershan P. S. Monodomain samples of dipalmitoyl phosphatidylcholine with varying concentrations of water and other ingredients. Biophys J. 1977 Nov;20(2):137–152. doi: 10.1016/S0006-3495(77)85540-9. [DOI] [PMC free article] [PubMed] [Google Scholar]
- Rice D., Oldfield E. Deuterium nuclear magnetic resonance studies of the interaction between dimyristoylphosphatidylcholine and gramicidin A'. Biochemistry. 1979 Jul 24;18(15):3272–3279. doi: 10.1021/bi00582a012. [DOI] [PubMed] [Google Scholar]
- Rothschild K. J., Clark N. A. Polarized infrared spectroscopy of oriented purple membrane. Biophys J. 1979 Mar;25(3):473–487. doi: 10.1016/S0006-3495(79)85317-5. [DOI] [PMC free article] [PubMed] [Google Scholar]
- Rothschild K. J., Rosen K. M., Clark N. A. Incorporation of photoreceptor membrane into a multilamellar film. Biophys J. 1980 Jul;31(1):45–52. doi: 10.1016/S0006-3495(80)85039-9. [DOI] [PMC free article] [PubMed] [Google Scholar]
- Sankaram M. B., Thompson T. E. Cholesterol-induced fluid-phase immiscibility in membranes. Proc Natl Acad Sci U S A. 1991 Oct 1;88(19):8686–8690. doi: 10.1073/pnas.88.19.8686. [DOI] [PMC free article] [PubMed] [Google Scholar]
- Shimoyama Y., Eriksson L. E., Ehrenberg A. Molecular motion and order in oriented lipid multibilayer membranes evaluated by simulations of spin label ESR spectra. Effects of temperature, cholesterol and magnetic field. Biochim Biophys Acta. 1978 Apr 4;508(2):213–235. doi: 10.1016/0005-2736(78)90326-7. [DOI] [PubMed] [Google Scholar]
- Shin Y. K., Budil D. E., Freed J. H. Thermodynamics and dynamics of phosphatidylcholine-cholesterol mixed model membranes in the liquid crystalline state: effects of water. Biophys J. 1993 Sep;65(3):1283–1294. doi: 10.1016/S0006-3495(93)81160-3. [DOI] [PMC free article] [PubMed] [Google Scholar]
- Shin Y. K., Freed J. H. Dynamic imaging of lateral diffusion by electron spin resonance and study of rotational dynamics in model membranes. Effect of cholesterol. Biophys J. 1989 Mar;55(3):537–550. doi: 10.1016/S0006-3495(89)82847-4. [DOI] [PMC free article] [PubMed] [Google Scholar]
- Shin Y. K., Freed J. H. Thermodynamics of phosphatidylcholine-cholesterol mixed model membranes in the liquid crystalline state studied by the orientational order parameter. Biophys J. 1989 Dec;56(6):1093–1100. doi: 10.1016/S0006-3495(89)82757-2. [DOI] [PMC free article] [PubMed] [Google Scholar]
- Silvius J. R., Brown P. M., O'Leary T. J. Role of head group structure in the phase behavior of amino phospholipids. 1. Hydrated and dehydrated lamellar phases of saturated phosphatidylethanolamine analogues. Biochemistry. 1986 Jul 29;25(15):4249–4258. doi: 10.1021/bi00363a012. [DOI] [PubMed] [Google Scholar]
- Vanderkooi G. Computation of mixed phosphatidylcholine-cholesterol bilayer structures by energy minimization. Biophys J. 1994 May;66(5):1457–1468. doi: 10.1016/S0006-3495(94)80936-1. [DOI] [PMC free article] [PubMed] [Google Scholar]
- Vogel H., Jähnig F., Hoffmann V., Stümpel J. The orientation of melittin in lipid membranes. A polarized infrared spectroscopy study. Biochim Biophys Acta. 1983 Sep 7;733(2):201–209. doi: 10.1016/0005-2736(83)90523-0. [DOI] [PubMed] [Google Scholar]
- Williams B. W., Scotto A. W., Stubbs C. D. Effect of proteins on fluorophore lifetime heterogeneity in lipid bilayers. Biochemistry. 1990 Apr 3;29(13):3248–3255. doi: 10.1021/bi00465a016. [DOI] [PubMed] [Google Scholar]
- Wong P. T., Capes S. E., Mantsch H. H. Hydrogen bonding between anhydrous cholesterol and phosphatidylcholines: an infrared spectroscopic study. Biochim Biophys Acta. 1989 Mar 27;980(1):37–41. doi: 10.1016/0005-2736(89)90197-1. [DOI] [PubMed] [Google Scholar]
- de Kruyff B., Demel R. A., van Deenen L. L. The effect of cholesterol and epicholesterol incorporation on the permeability and on the phase transition of intact Acholeplasma laidlawii cell membranes and derived liposomes. Biochim Biophys Acta. 1972 Jan 17;255(1):331–347. doi: 10.1016/0005-2736(72)90032-6. [DOI] [PubMed] [Google Scholar]