Abstract
1. The steady-state transfer of cationized ferritin by endothelial cell vesicles has been investigated quantitatively using electron microscopy. Single capillaries from the mesenteries of decerebrated frogs were perfused in vivo with solutions containing 3-5 g 100 ml-1 cationized ferritin or cationized ferritin (3-5 g 100 ml-1) and bovine albumin (1 g 100 ml-1). Perfusions lasted between 60 and 240 s, at which time the tissues were fixed in situ with osmium tetroxide.
2. Measurements of the free diffusion co-efficient of cationized ferritin in the presence and absence of 1 g 100 ml-1 albumin (0·400±0·09 × 10-6 cm2 s-1 and 0·361±0·08 × 10-6 cm2 s-1, respectively) were not significantly different which suggests that albumin does not bind to cationized ferritin. Together they yielded a value for the Stokes—Einstein radius of cationized ferritin of 5·59 nm, which was not significantly different from that of native ferritin.
3. Examination of transverse sections of perfused capillaries showed a layer of cationized ferritin molecules (> 26 nm thick) close to the luminal surface of the endothelial cell wall, in both the presence and absence of albumin. Estimates of the concentration of cationized ferritin within the layer showed it to be approximately twice that of the perfusate concentration, confirming that cationized ferritin binds and concentrates at the cell surface.
4. When no albumin was present in the cationized ferritin perfusate, all the luminal vesicles (those open to the luminal cell surface) were labelled with molecules of cationized ferritin. The mean number of ferritin molecules per labelled luminal vesicle (F/NL) was 5·0±0·7, a value close to that predicted from the concentration of cationized ferritin in the layer if it was assumed that the whole of the vesicle volume was available to molecules of cationized ferritin, i.e. that cationized ferritin could penetrate the cell coat lining the vesicles as it does that covering the luminal cell surface.
5. Few cytoplasmic vesicles (0·26±0·04) and abluminal vesicles (0·07±0·03) were labelled with cationized ferritin in the absence of albumin. The mean number of cationized ferritin molecules per labelled vesicle in both vesicle populations was also low. In addition, labelled cytoplasmic vesicles F/NL = 1·87±0·33) always contained significantly fewer ferritin molecules than labelled luminal vesicles (F/NL = 5·0±0·7). These findings offer further support for the fusion model of the steady-state transfer of ferritin by endothelial cell vesicles (Clough & Michel, 1981) and are not consistent with the translocation of labelled luminal vesicles across the cell. They also suggest that cationized ferritin binds to the cell coat lining the vesicles, and is unavailable for transfer during transient fusions between vesicles.
6. The presence of albumin in the cationized ferritin perfusate reduced the fractional labelling of all three vesicle populations to one third of their values in its absence. It also reduced the mean number of ferritin molecules per labelled vesicle at all three sites in the cell. It is suggested that albumin reduces the volume of distribution of cationized ferritin within the vesicles either by competing with cationized ferritin for the same binding sites within the cell coat, or by simply occupying space within the extracellular matrix.
Full text
PDF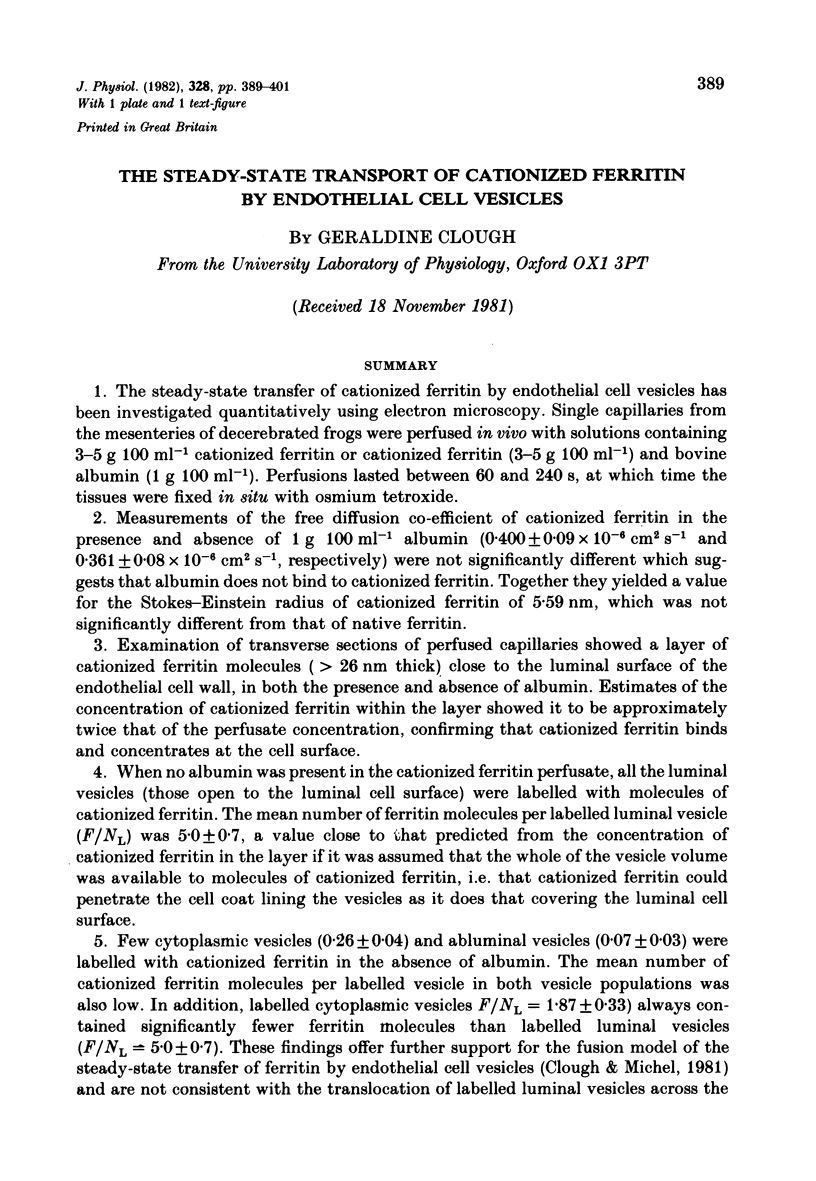
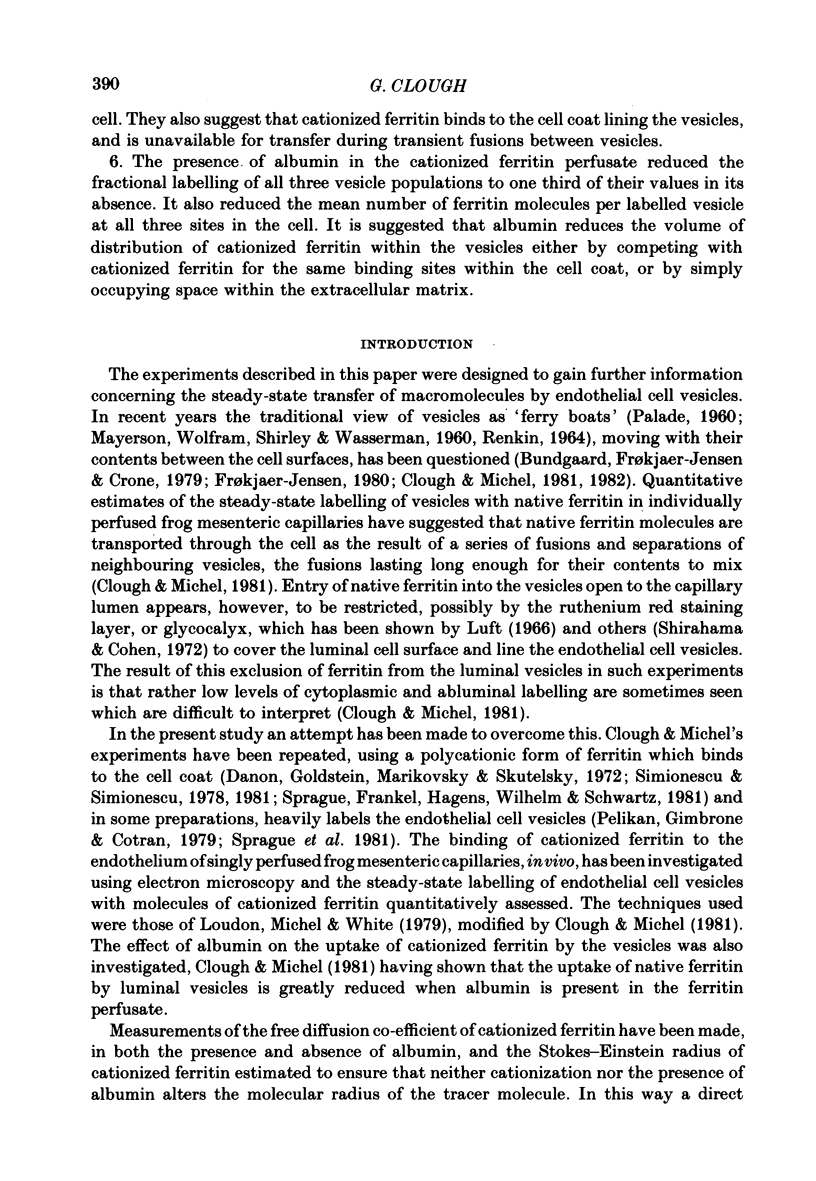
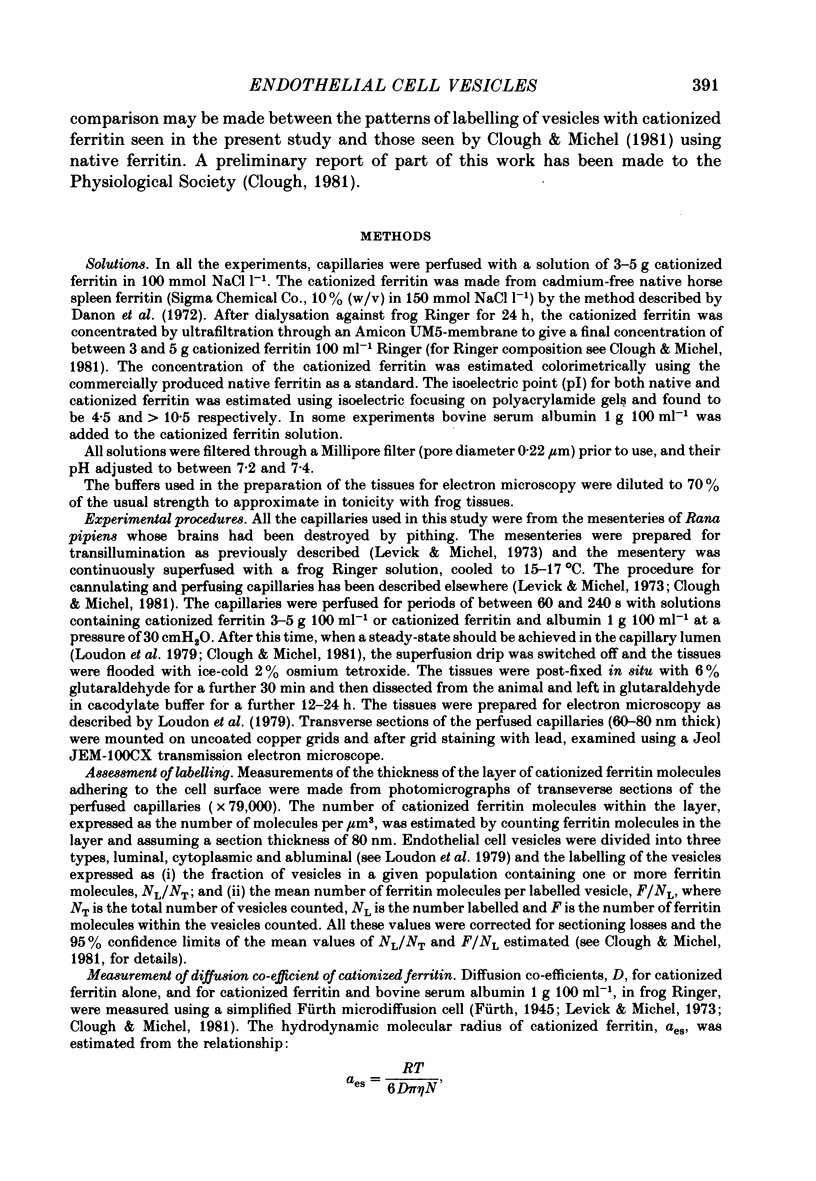
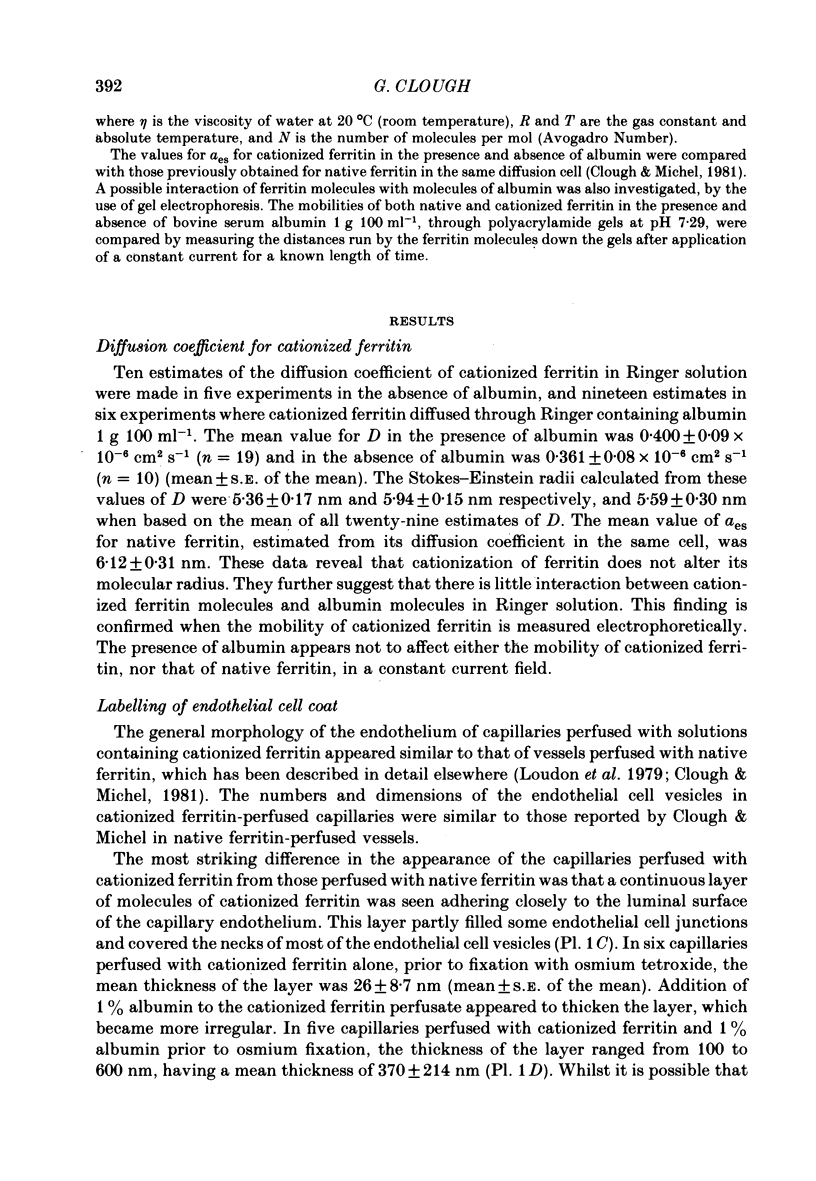
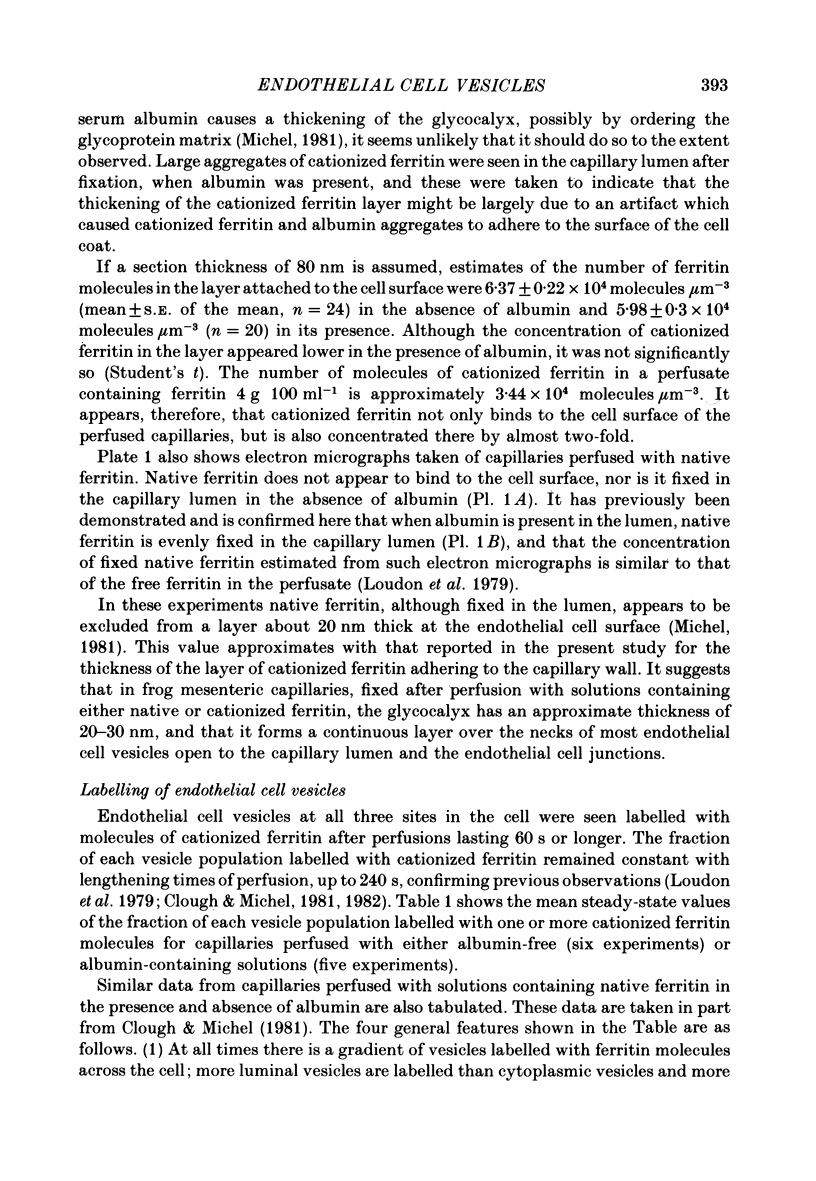
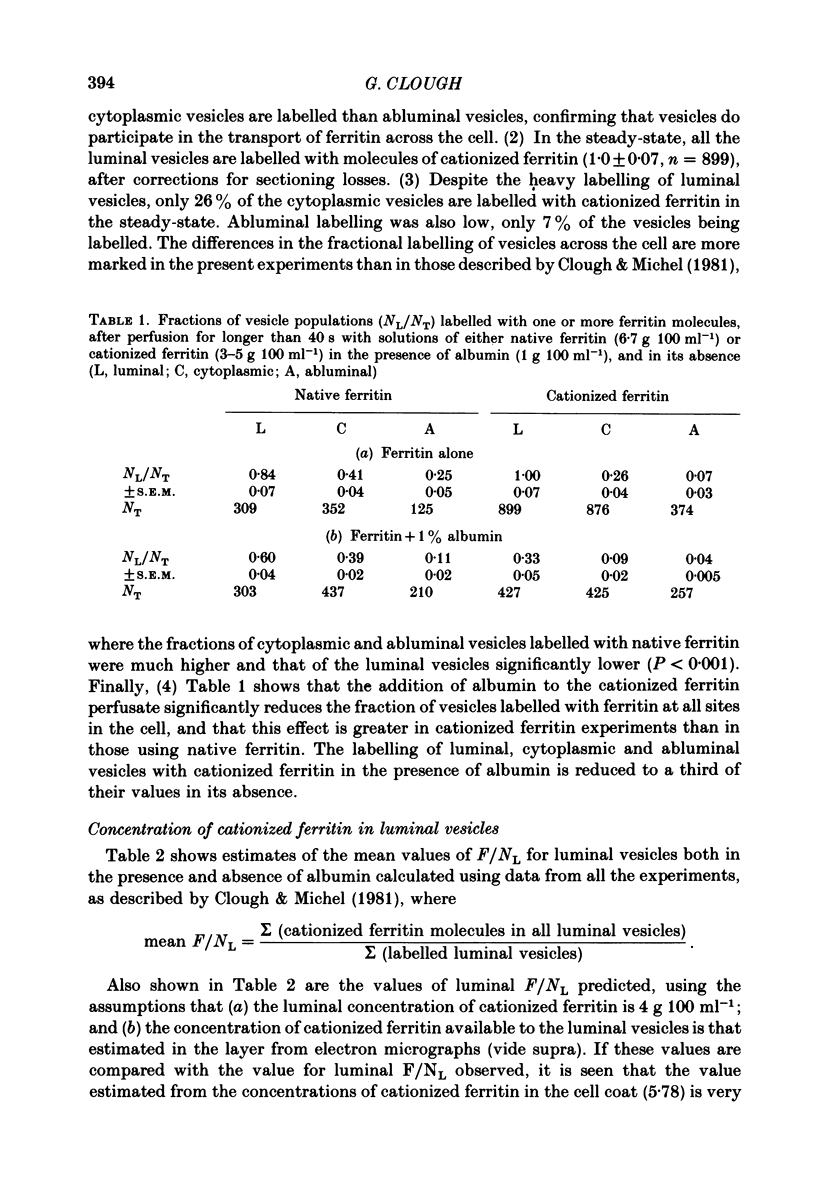
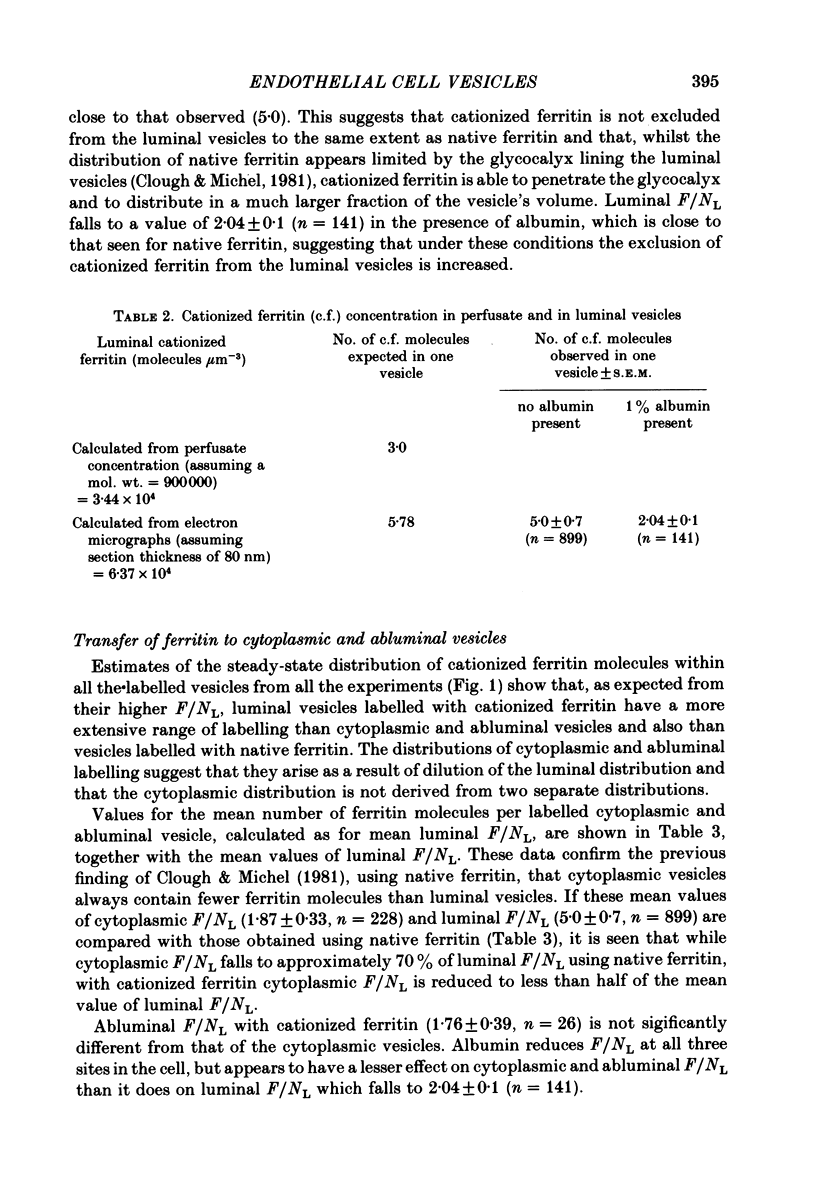
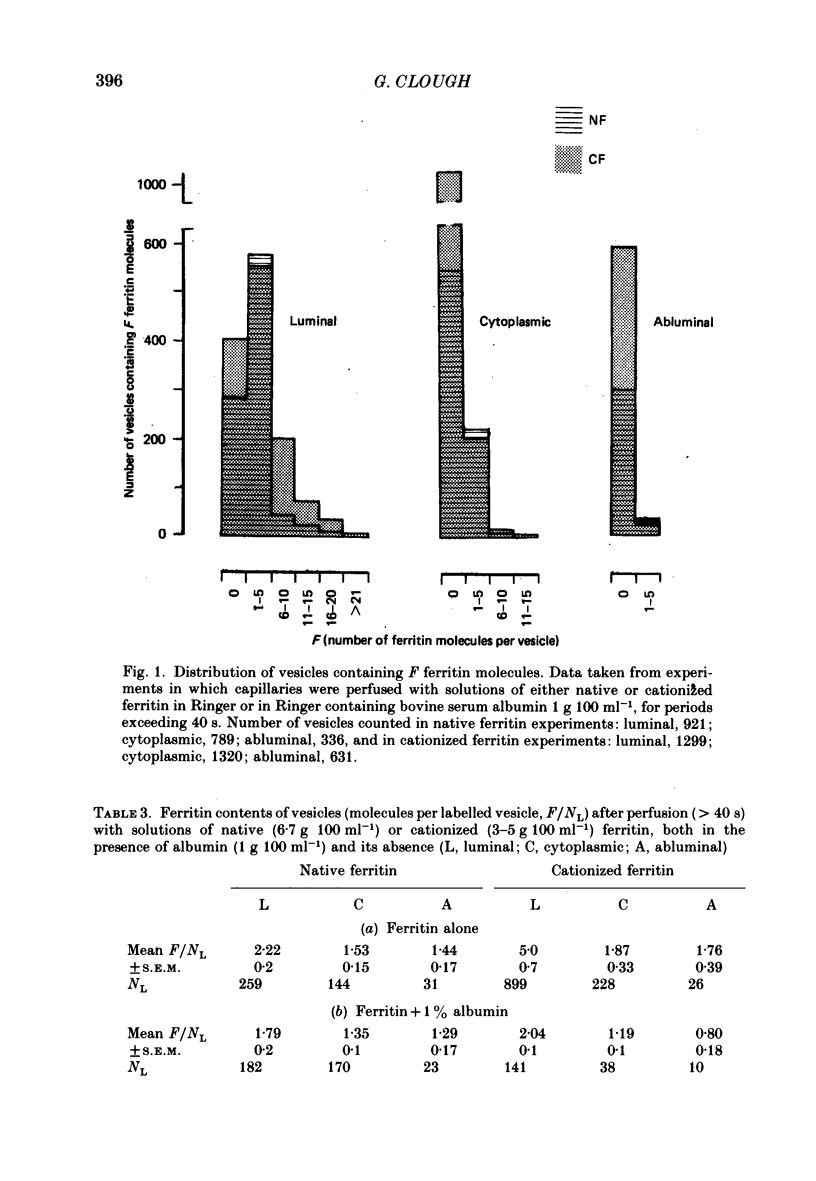
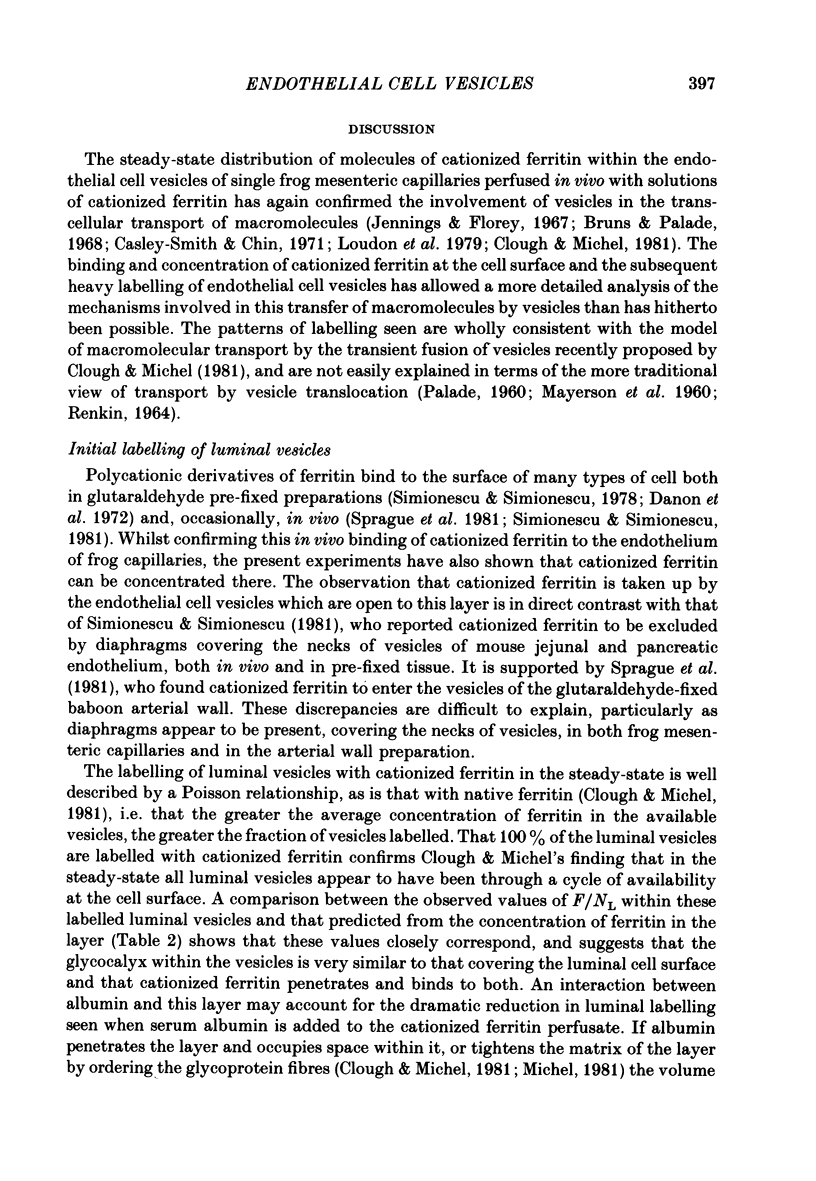
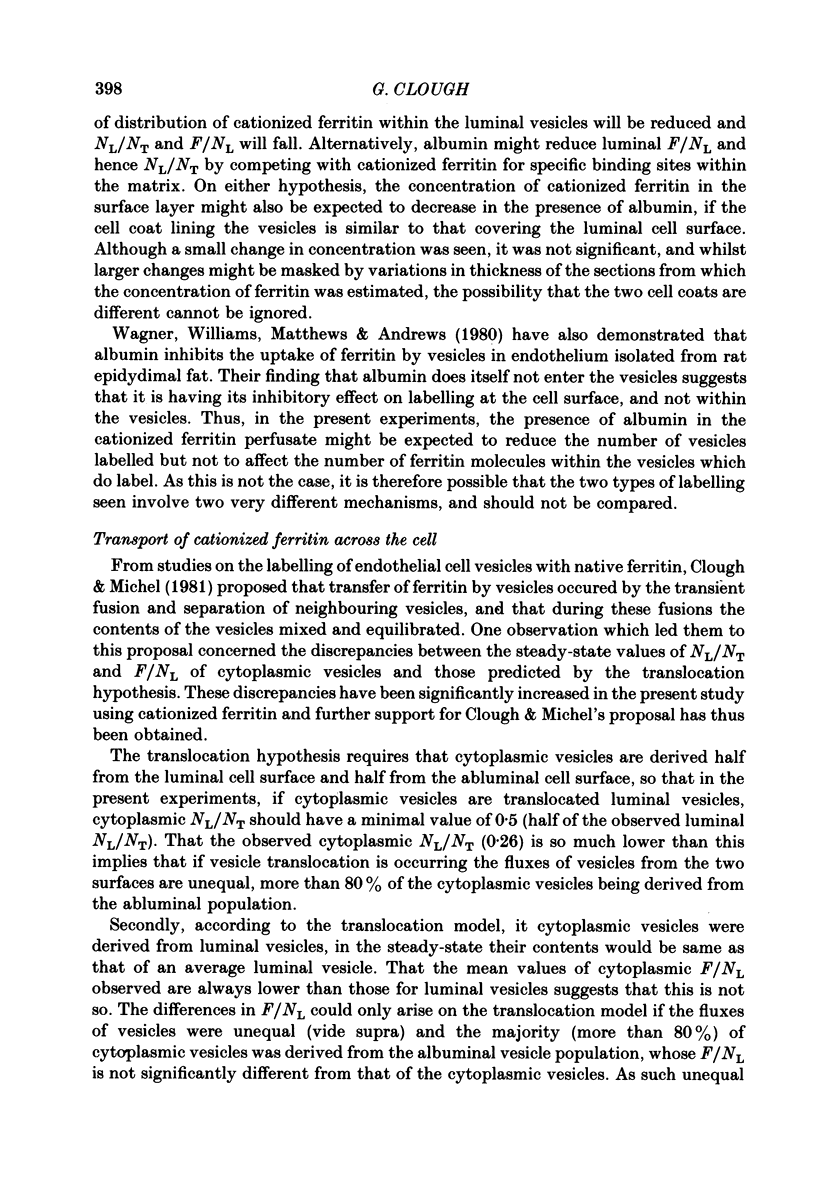
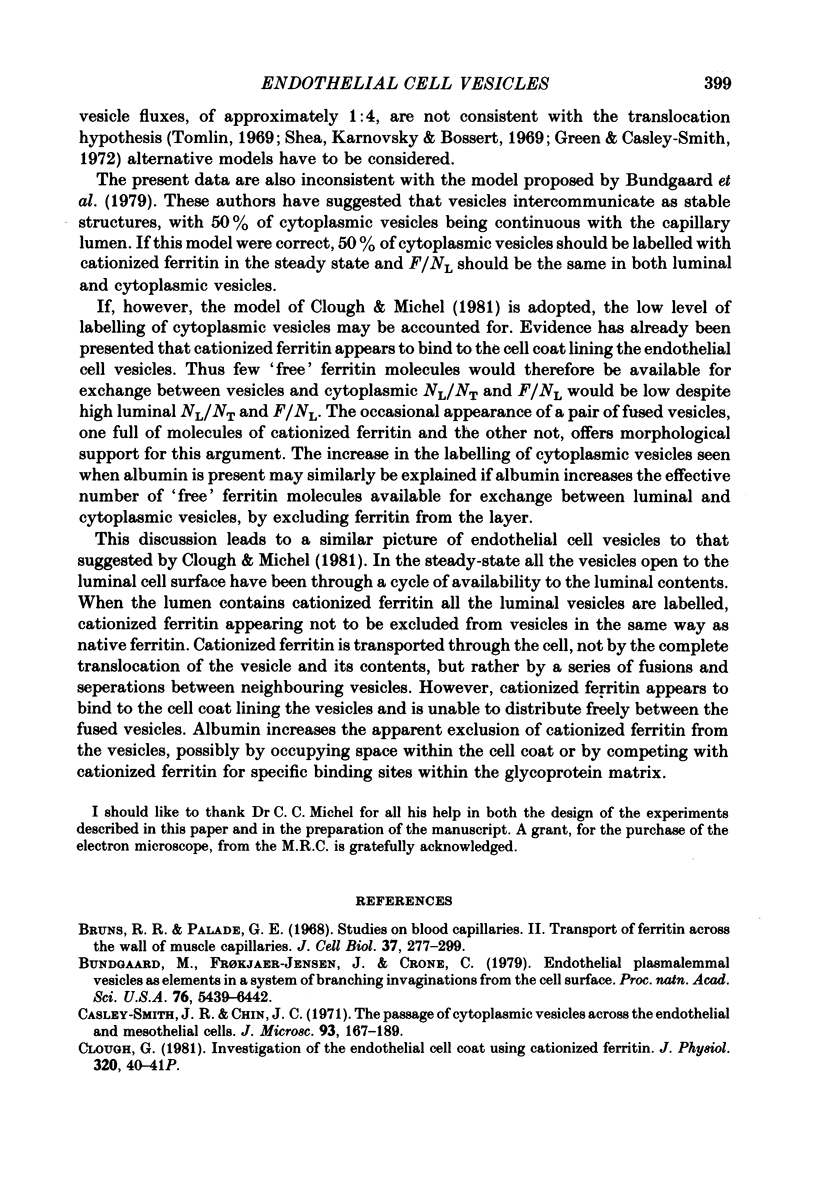
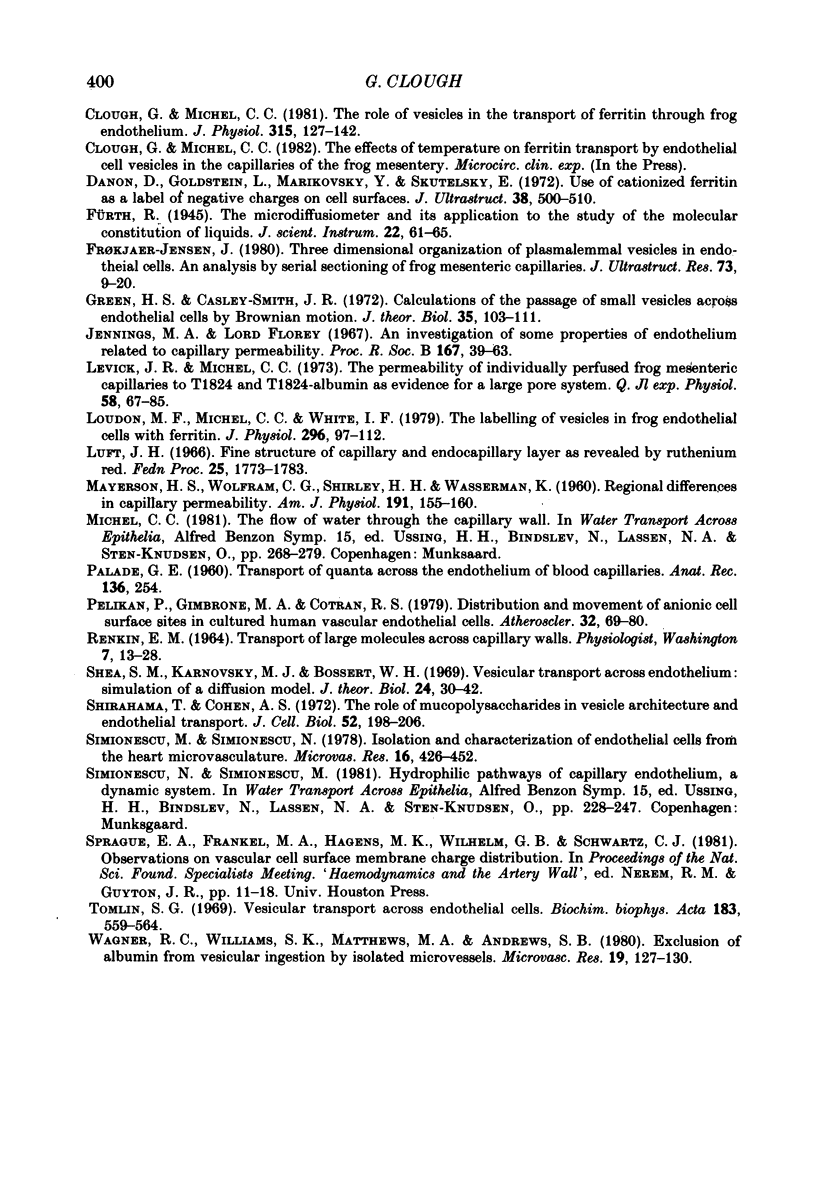
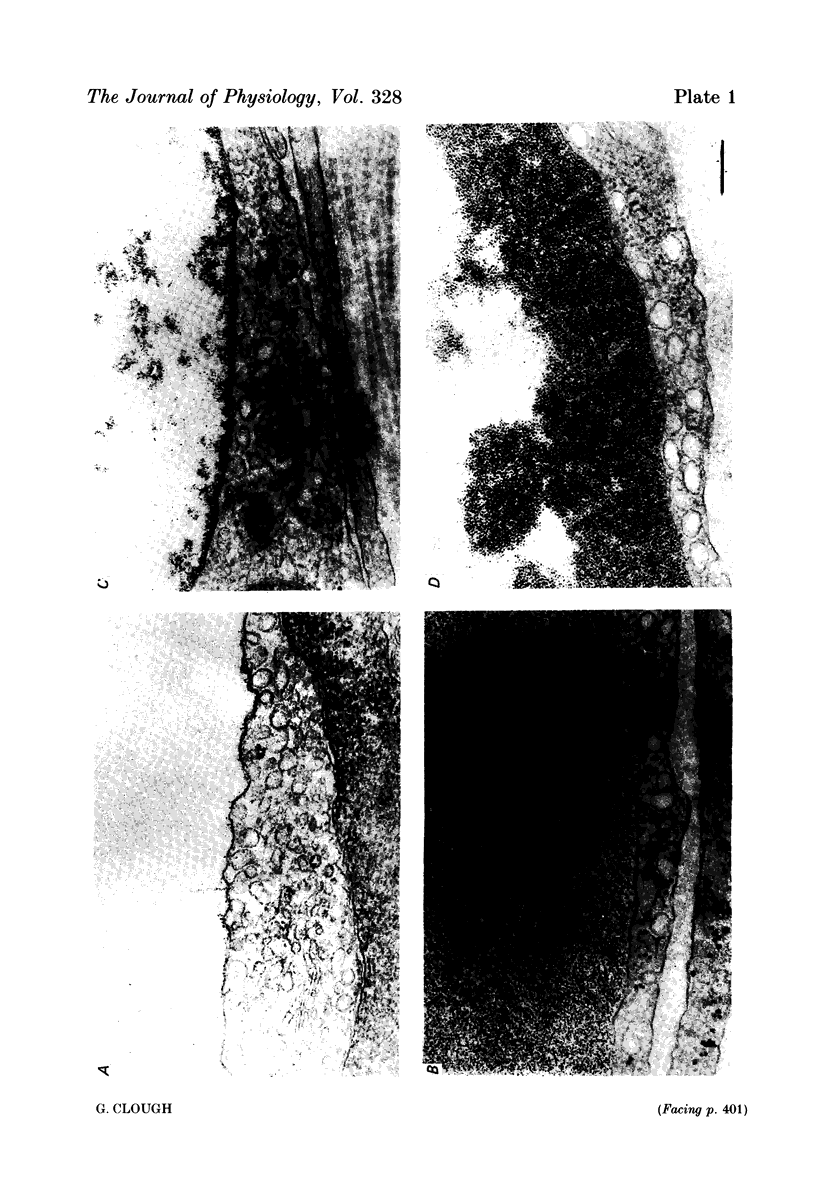
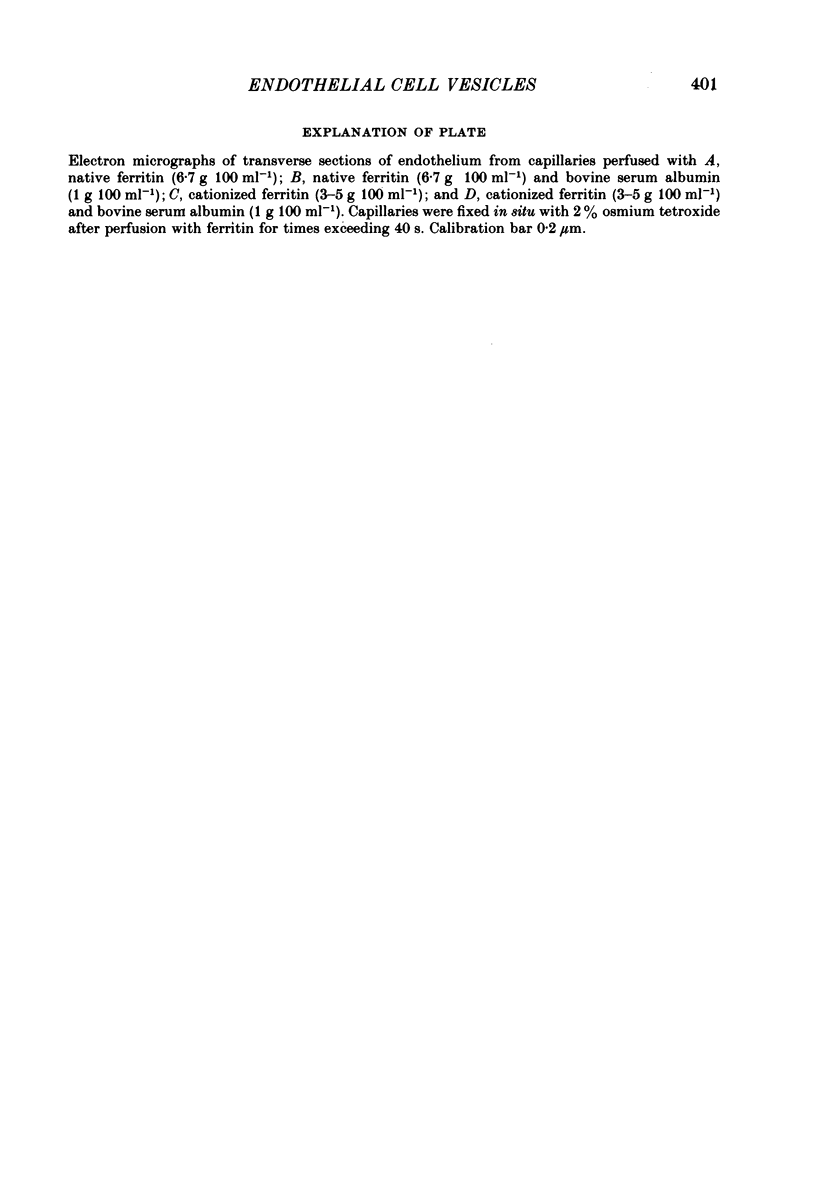
Images in this article
Selected References
These references are in PubMed. This may not be the complete list of references from this article.
- Bruns R. R., Palade G. E. Studies on blood capillaries. II. Transport of ferritin molecules across the wall of muscle capillaries. J Cell Biol. 1968 May;37(2):277–299. doi: 10.1083/jcb.37.2.277. [DOI] [PMC free article] [PubMed] [Google Scholar]
- Bundgaard M., Frøkjaer-Jensen J., Crone C. Endothelial plasmalemmal vesicles as elements in a system of branching invaginations from the cell surface. Proc Natl Acad Sci U S A. 1979 Dec;76(12):6439–6442. doi: 10.1073/pnas.76.12.6439. [DOI] [PMC free article] [PubMed] [Google Scholar]
- Clough G., Michel C. C. The role of vesicles in the transport of ferritin through frog endothelium. J Physiol. 1981 Jun;315:127–142. doi: 10.1113/jphysiol.1981.sp013737. [DOI] [PMC free article] [PubMed] [Google Scholar]
- Danon D., Goldstein L., Marikovsky Y., Skutelsky E. Use of cationized ferritin as a label of negative charges on cell surfaces. J Ultrastruct Res. 1972 Mar;38(5):500–510. doi: 10.1016/0022-5320(72)90087-1. [DOI] [PubMed] [Google Scholar]
- Frøkjaer-Jensen J. Three-dimensional organization of plasmalemmal vesicles in endothelial cells. An analysis by serial sectioning of frog mesenteric capillaries. J Ultrastruct Res. 1980 Oct;73(1):9–20. doi: 10.1016/0022-5320(80)90111-2. [DOI] [PubMed] [Google Scholar]
- Green H. S., Casley-Smith J. R. Calculations on the passage of small vesicles across endothelial cells by Brownian motion. J Theor Biol. 1972 Apr;35(1):103–111. doi: 10.1016/0022-5193(72)90195-6. [DOI] [PubMed] [Google Scholar]
- Jennings M. A., Florey L. An investigation of some properties of endothelium related to capillary permeability. Proc R Soc Lond B Biol Sci. 1967 Jan 31;167(1006):39–63. doi: 10.1098/rspb.1967.0012. [DOI] [PubMed] [Google Scholar]
- Levick J. R., Michel C. C. The permeability of individually perfused frog mesenteric capillaries to T1824 and T1824-albumin as evidence for a large pore system. Q J Exp Physiol Cogn Med Sci. 1973 Jan;58(1):67–85. doi: 10.1113/expphysiol.1973.sp002192. [DOI] [PubMed] [Google Scholar]
- Loudon M. F., Michel C. C., White I. F. The labelling of vesicles in frog endothelial cells with ferritin. J Physiol. 1979 Nov;296:97–112. doi: 10.1113/jphysiol.1979.sp012993. [DOI] [PMC free article] [PubMed] [Google Scholar]
- Pelikan P., Gimbrone M. A., Jr, Cotran R. S. Distribution and movement of anionic cell surface sites in cultured human vascular endothelial cells. Atherosclerosis. 1979 Jan;32(1):69–80. doi: 10.1016/0021-9150(79)90148-5. [DOI] [PubMed] [Google Scholar]
- RENKIN E. M. TRANSPORT OF LARGE MOLECULES ACROSS CAPILLARY WALLS. Physiologist. 1964 Feb;60:13–28. [PubMed] [Google Scholar]
- Shea S. M., Karnovsky M. J., Bossert W. H. Vesicular transport across endothelium: simulation of a diffusion model. J Theor Biol. 1969 Jul;24(1):30–42. doi: 10.1016/s0022-5193(69)80004-4. [DOI] [PubMed] [Google Scholar]
- Shirahama T., Cohen A. S. The role of mucopolysaccharides in vesicle architecture and endothelial transport. An electron microscope study of myocardial blood vessels. J Cell Biol. 1972 Jan;52(1):198–206. doi: 10.1083/jcb.52.1.198. [DOI] [PMC free article] [PubMed] [Google Scholar]
- Simionescu M., Simionescu N. Isolation and characterization of endothelial cells from the heart microvasculature. Microvasc Res. 1978 Nov;16(3):426–452. doi: 10.1016/0026-2862(78)90074-2. [DOI] [PubMed] [Google Scholar]
- Tomlin S. G. Vesicular transport across endothelial cells. Biochim Biophys Acta. 1969;183(3):559–564. doi: 10.1016/0005-2736(69)90169-2. [DOI] [PubMed] [Google Scholar]
- Wagner R. C., Williams S. K., Matthews M. A., Andrews S. B. Exclusion of albumin from vesicular ingestion by isolated microvessels. Microvasc Res. 1980 Jan;19(1):127–130. doi: 10.1016/0026-2862(80)90088-6. [DOI] [PubMed] [Google Scholar]