Abstract
Deuterium and phosphorus nuclear magnetic resonance (NMR) has been used to investigate the dynamics of slow motional processes induced in bilayer cardiolipin upon binding with cytochrome c. 31P NMR line shapes suggest that protein binding induces less restricted, isotropic-like motions in the lipid phosphates within the ms time scale of this measurement. However, these motions impart rapid transverse relaxation to methylene deuterons adjacent to the phosphate in the lipid headgroup and so did not feature strongly in the NMR line shapes recorded from these nuclei by using the quadrupolar echo. Nonetheless, motional characteristics of the headgroup deuterons were accessible to a dynamic NMR approach using the Carr-Purcell-Meiboom-Gill multiple-pulse experiment. Compared to the well-studied case of deuterons in fatty acyl chains of bilayer phosphatidylcholine, the motions determining the 2H spin transverse relaxation in the headgroup of bilayer cardiolipin were much faster, having a lower limit in the 5-10 kHz range. On binding with cytochrome c, the T2 effecting motions in the cardiolipin headgroup became faster still, with rates comparable to the residual quadrupolar coupling frequency of the headgroup deuterons (approximately 25 kHz) and so coincided with the time scale for recording the quadrupolar echo (approximately 40 microseconds). It is concluded that the headgroup of cardiolipin does not exclusively report localized dynamic information but is particularly sensitive to collective motions occurring throughout the bilayer molecules. Although the rates of collective modes of motion may be dependent on the lipid type in pure lipid bilayers, these low-frequency fluctuations appear to occupy a similar dynamic range in a variety of lipid-protein systems, including the natural membranes.
Full text
PDF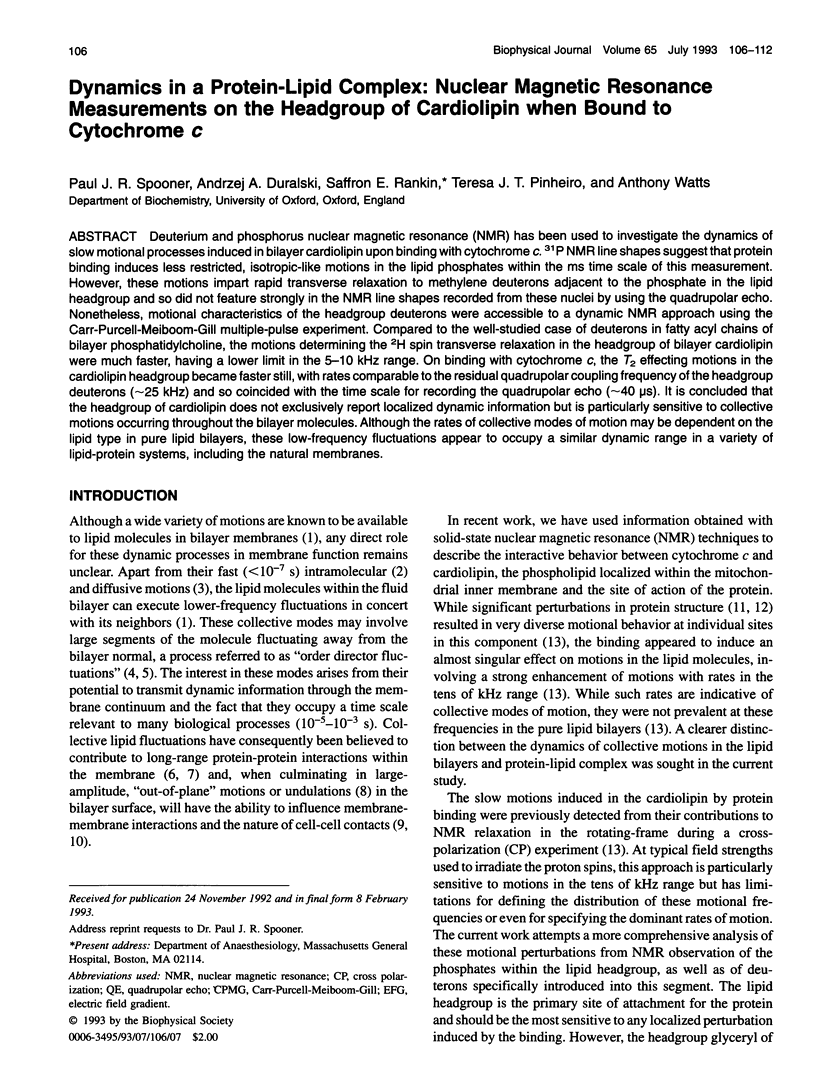
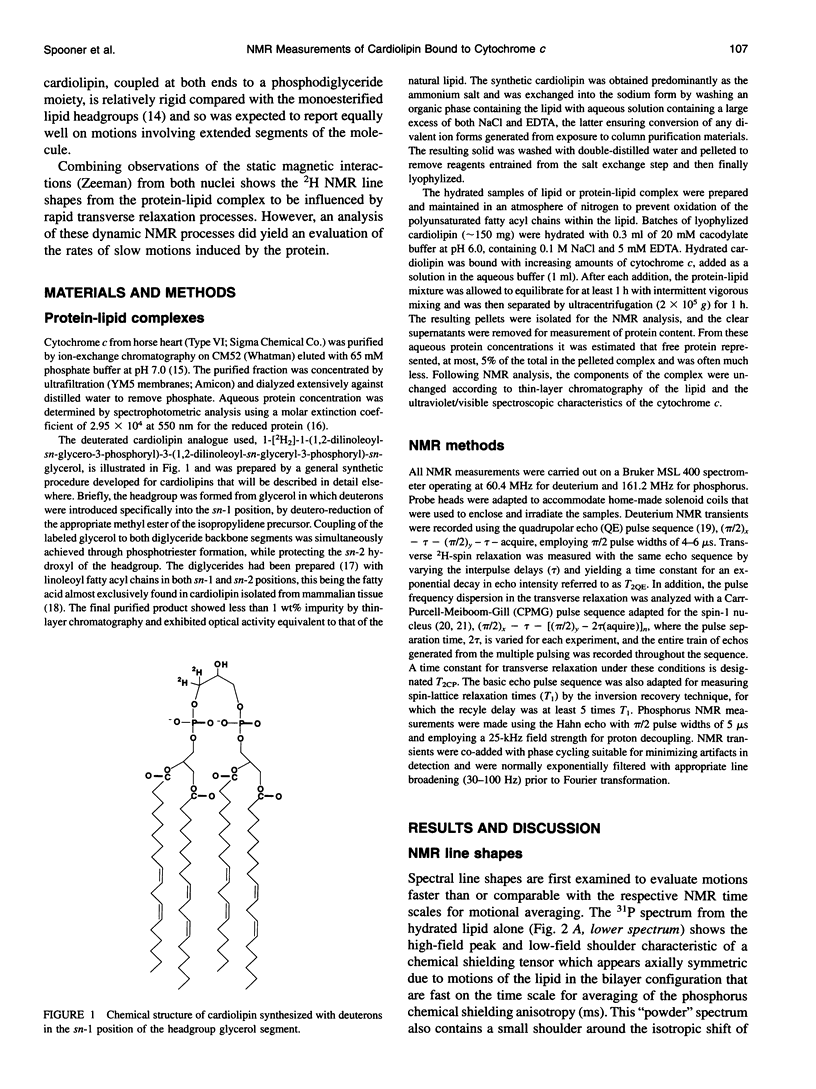
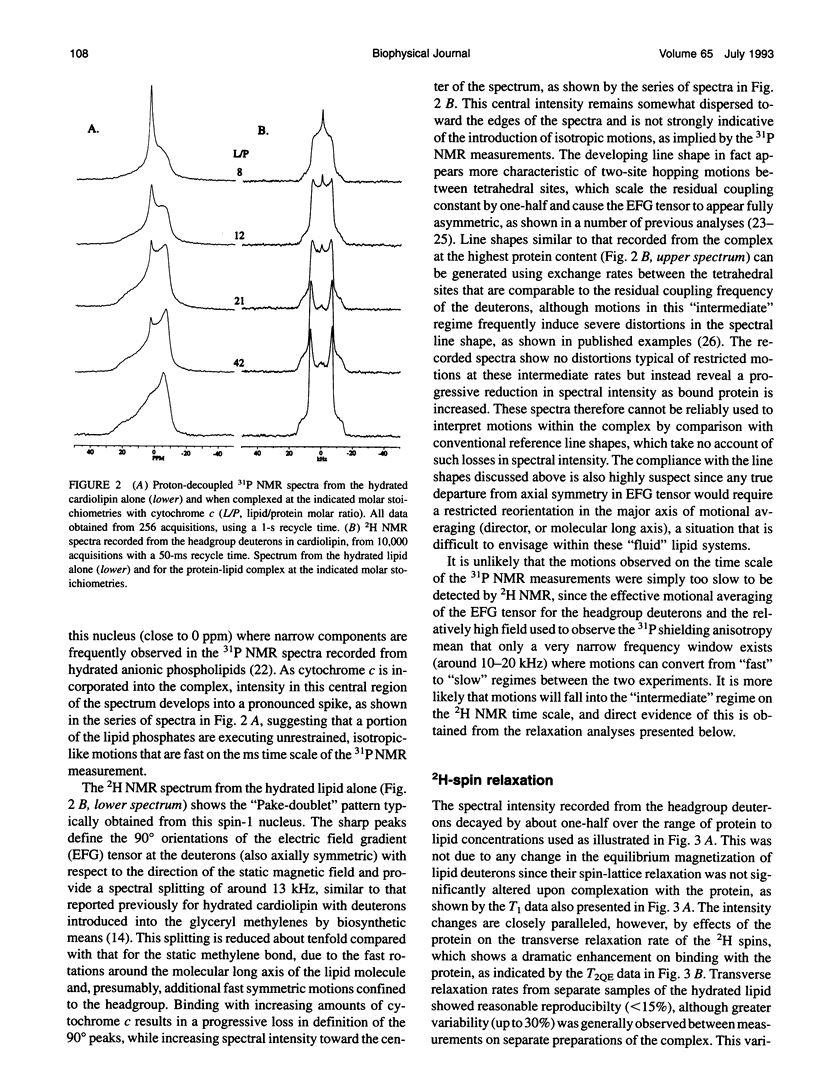
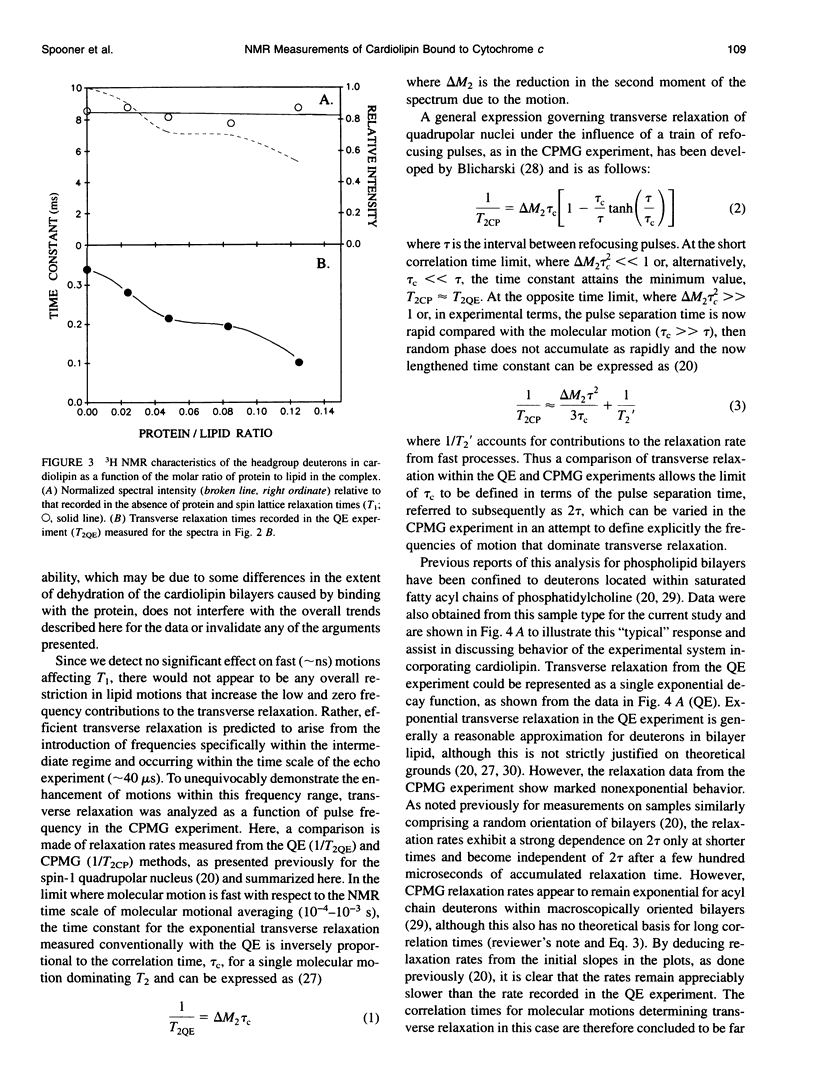
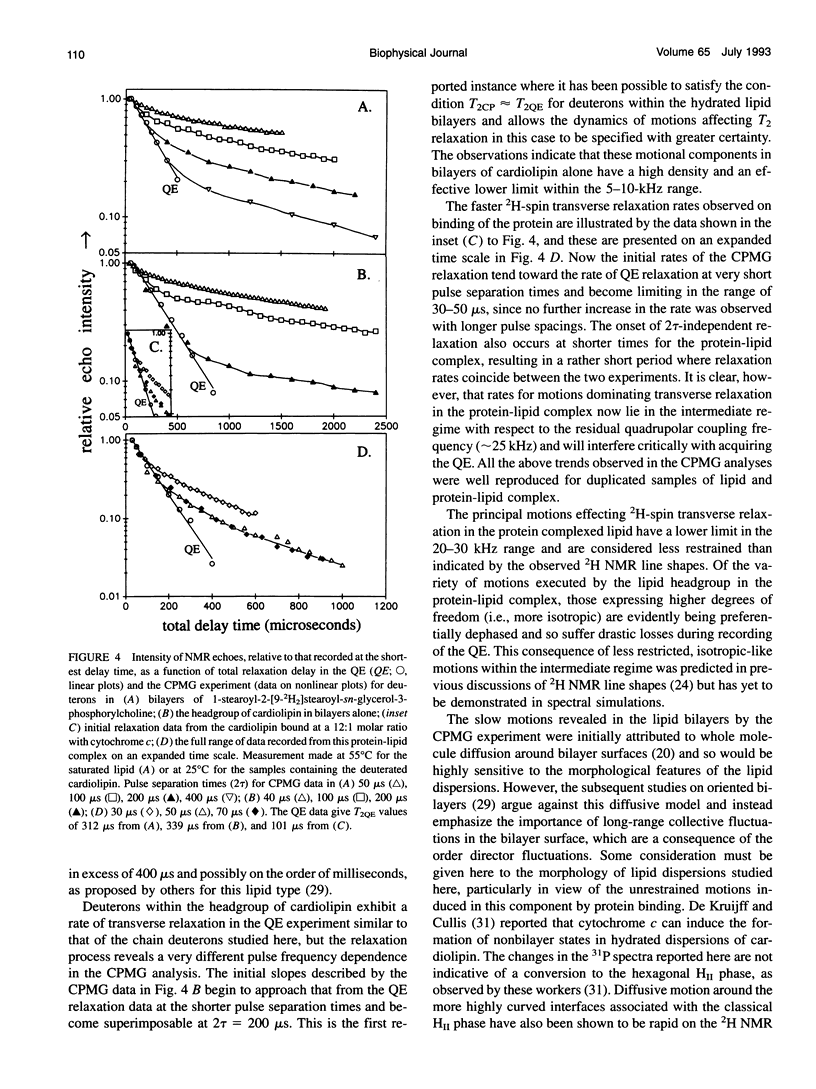
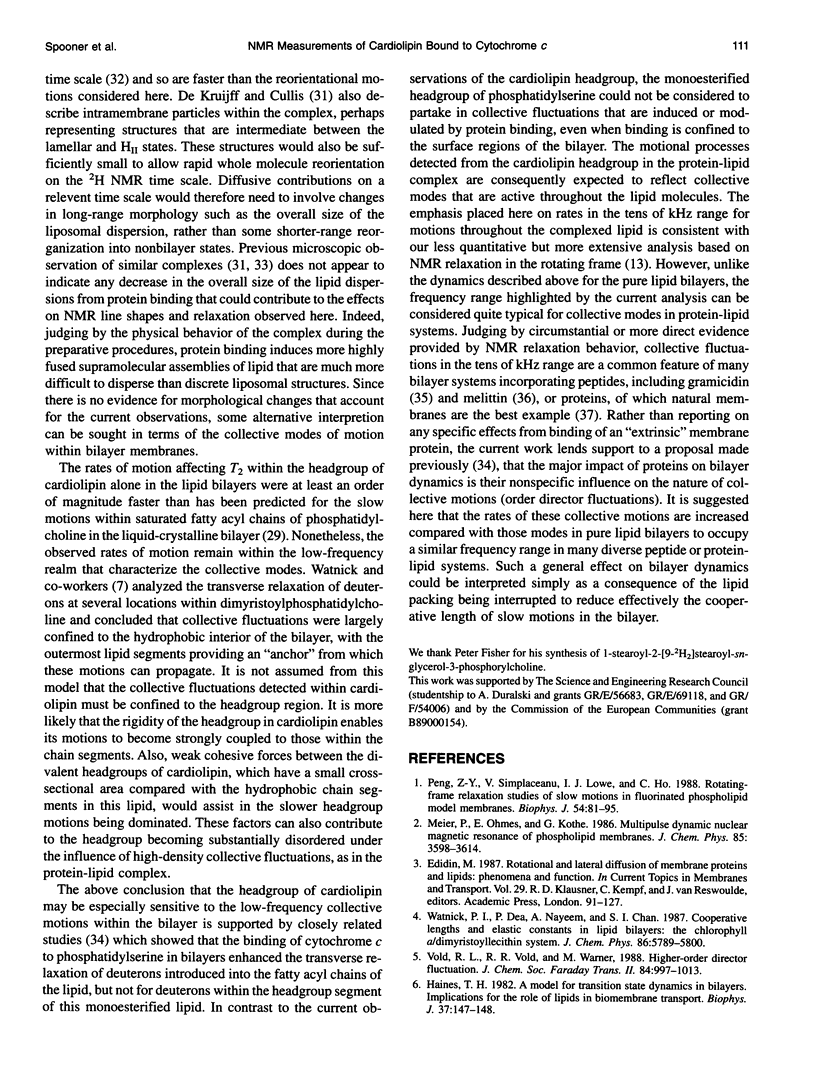
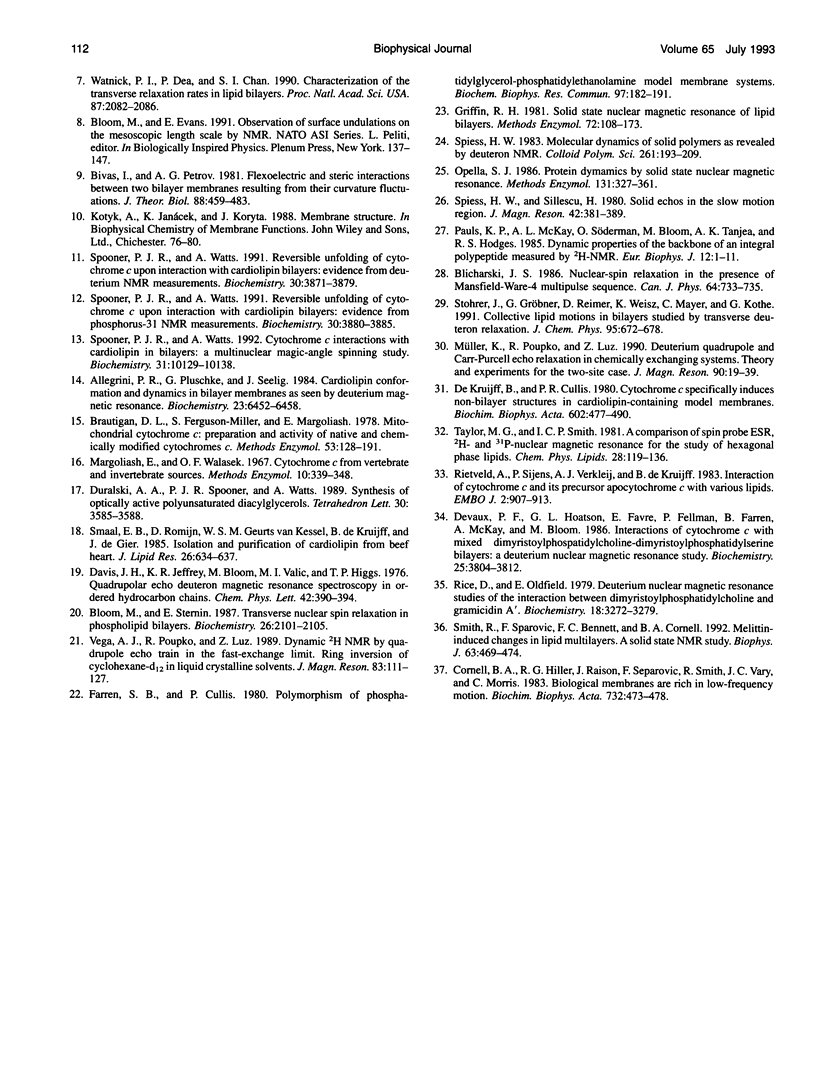
Selected References
These references are in PubMed. This may not be the complete list of references from this article.
- Bivas I., Petrov A. G. Flexoelectric and steric interactions between two bilayer lipid membranes resulting from their curvature fluctuations. J Theor Biol. 1981 Feb 7;88(3):459–483. doi: 10.1016/0022-5193(81)90277-0. [DOI] [PubMed] [Google Scholar]
- Brautigan D. L., Ferguson-Miller S., Margoliash E. Mitochondrial cytochrome c: preparation and activity of native and chemically modified cytochromes c. Methods Enzymol. 1978;53:128–164. doi: 10.1016/s0076-6879(78)53021-8. [DOI] [PubMed] [Google Scholar]
- Cornell B. A., Hiller R. G., Raison J., Separovic F., Smith R., Vary J. C., Morris C. Biological membranes are rich in low-frequency motion. Biochim Biophys Acta. 1983 Jul 27;732(2):473–478. doi: 10.1016/0005-2736(83)90065-2. [DOI] [PubMed] [Google Scholar]
- Devaux P. F., Hoatson G. L., Favre E., Fellmann P., Farren B., MacKay A. L., Bloom M. Interaction of cytochrome c with mixed dimyristoylphosphatidylcholine-dimyristoylphosphatidylserine bilayers: a deuterium nuclear magnetic resonance study. Biochemistry. 1986 Jul 1;25(13):3804–3812. doi: 10.1021/bi00361a011. [DOI] [PubMed] [Google Scholar]
- Farren S. B., Cullis P. R. Polymorphism of phosphatidylglycerol-phosphatidylethanolamine model membrane systems: a 31p NMR study. Biochem Biophys Res Commun. 1980 Nov 17;97(1):182–191. doi: 10.1016/s0006-291x(80)80152-5. [DOI] [PubMed] [Google Scholar]
- Griffin R. G. Solid state nuclear magnetic resonance of lipid bilayers. Methods Enzymol. 1981;72:108–174. doi: 10.1016/s0076-6879(81)72010-x. [DOI] [PubMed] [Google Scholar]
- Haines T. H. A model for transition state dynamics in bilayers: implications for the role of lipids in biomembrane transport. Biophys J. 1982 Jan;37(1):147–148. doi: 10.1016/S0006-3495(82)84644-4. [DOI] [PMC free article] [PubMed] [Google Scholar]
- Opella S. J. Protein dynamics by solid state nuclear magnetic resonance. Methods Enzymol. 1986;131:327–361. doi: 10.1016/0076-6879(86)31048-6. [DOI] [PubMed] [Google Scholar]
- Pauls K. P., MacKay A. L., Söderman O., Bloom M., Tanjea A. K., Hodges R. S. Dynamic properties of the backbone of an integral membrane polypeptide measured by 2H-NMR. Eur Biophys J. 1985;12(1):1–11. doi: 10.1007/BF00254089. [DOI] [PubMed] [Google Scholar]
- Peng Z. Y., Simplaceanu V., Lowe I. J., Ho C. Rotating-frame relaxation studies of slow motions in fluorinated phospholipid model membranes. Biophys J. 1988 Jul;54(1):81–95. doi: 10.1016/S0006-3495(88)82933-3. [DOI] [PMC free article] [PubMed] [Google Scholar]
- Rice D., Oldfield E. Deuterium nuclear magnetic resonance studies of the interaction between dimyristoylphosphatidylcholine and gramicidin A'. Biochemistry. 1979 Jul 24;18(15):3272–3279. doi: 10.1021/bi00582a012. [DOI] [PubMed] [Google Scholar]
- Rietveld A., Sijens P., Verkleij A. J., Kruijff B. Interaction of cytochrome c and its precursor apocytochrome c with various phospholipids. EMBO J. 1983;2(6):907–913. doi: 10.1002/j.1460-2075.1983.tb01520.x. [DOI] [PMC free article] [PubMed] [Google Scholar]
- Smaal E. B., Romijn D., Geurts van Kessel W. S., de Kruijff B., de Gier J. Isolation and purification of cardiolipin from beef heart. J Lipid Res. 1985 May;26(5):634–637. [PubMed] [Google Scholar]
- Smith R., Separovic F., Bennett F. C., Cornell B. A. Melittin-induced changes in lipid multilayers. A solid-state NMR study. Biophys J. 1992 Aug;63(2):469–474. doi: 10.1016/S0006-3495(92)81623-5. [DOI] [PMC free article] [PubMed] [Google Scholar]
- Spooner P. J., Watts A. Cytochrome c interactions with cardiolipin in bilayers: a multinuclear magic-angle spinning NMR study. Biochemistry. 1992 Oct 20;31(41):10129–10138. doi: 10.1021/bi00156a037. [DOI] [PubMed] [Google Scholar]
- Spooner P. J., Watts A. Reversible unfolding of cytochrome c upon interaction with cardiolipin bilayers. 1. Evidence from deuterium NMR measurements. Biochemistry. 1991 Apr 23;30(16):3871–3879. doi: 10.1021/bi00230a010. [DOI] [PubMed] [Google Scholar]
- Spooner P. J., Watts A. Reversible unfolding of cytochrome c upon interaction with cardiolipin bilayers. 2. Evidence from phosphorus-31 NMR measurements. Biochemistry. 1991 Apr 23;30(16):3880–3885. doi: 10.1021/bi00230a011. [DOI] [PubMed] [Google Scholar]
- Watnick P. I., Dea P., Chan S. I. Characterization of the transverse relaxation rates in lipid bilayers. Proc Natl Acad Sci U S A. 1990 Mar;87(6):2082–2086. doi: 10.1073/pnas.87.6.2082. [DOI] [PMC free article] [PubMed] [Google Scholar]
- de Kruijff B., Cullis P. R. Cytochrome c specifically induces non-bilayer structures in cardiolipin-containing model membranes. Biochim Biophys Acta. 1980 Nov 18;602(3):477–490. doi: 10.1016/0005-2736(80)90327-2. [DOI] [PubMed] [Google Scholar]