Abstract
The ability of Ca ions to inhibit Ca channels presents one of the most intriguing problems in membrane biophysics. Because of this negative feedback, Ca channels can regulate the current that flows through them. The kinetics of the channels depend on voltage, and, because the voltage controls the current, a strong interaction exists between voltage dependence and Ca dependence. In addition to this interaction, the proximity of pores and the local concentration of ions also determine how effectively the Ca ions influence channel kinetics. The present article proposes a model that incorporates voltage-dependent kinetics, current-dependent kinetics, and channel clustering. We have based the model on previous voltage-clamp data and on Ca and Ba action currents measured during the action potential in beating heart cells. In general we observe that great variability exists in channel kinetics from patch to patch: Ba or Ca currents have low or high amplitudes and slow or fast kinetics during essentially the same voltage regime, either applied step-protocols or spontaneous cell action potentials. To explain this variability, we have postulated that Ca channels interact through shared ions. The model we propose expands on our previous model for Ba currents. We use the same voltage-dependent rate constants for the Ca currents that we did for the Ba currents. However, we vary the current-dependent rate constants according to the species of the conducting ion. The model reproduces the main features of our data, and we use it to predict Ca channel kinetics under physiological conditions. Preliminary reports of this work have appeared (DeFelice et al., 1991, Biophys. J. 59:551a; Risso et al., 1992, Biophys. J. 61:248a).
Full text
PDF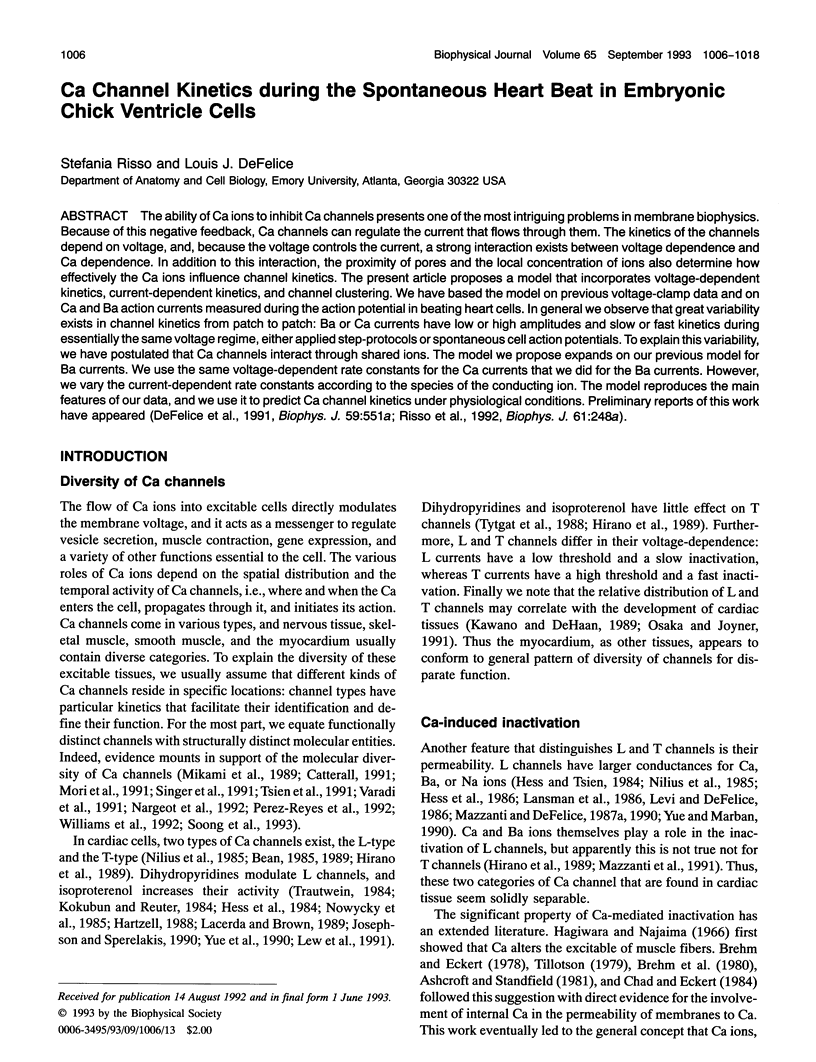
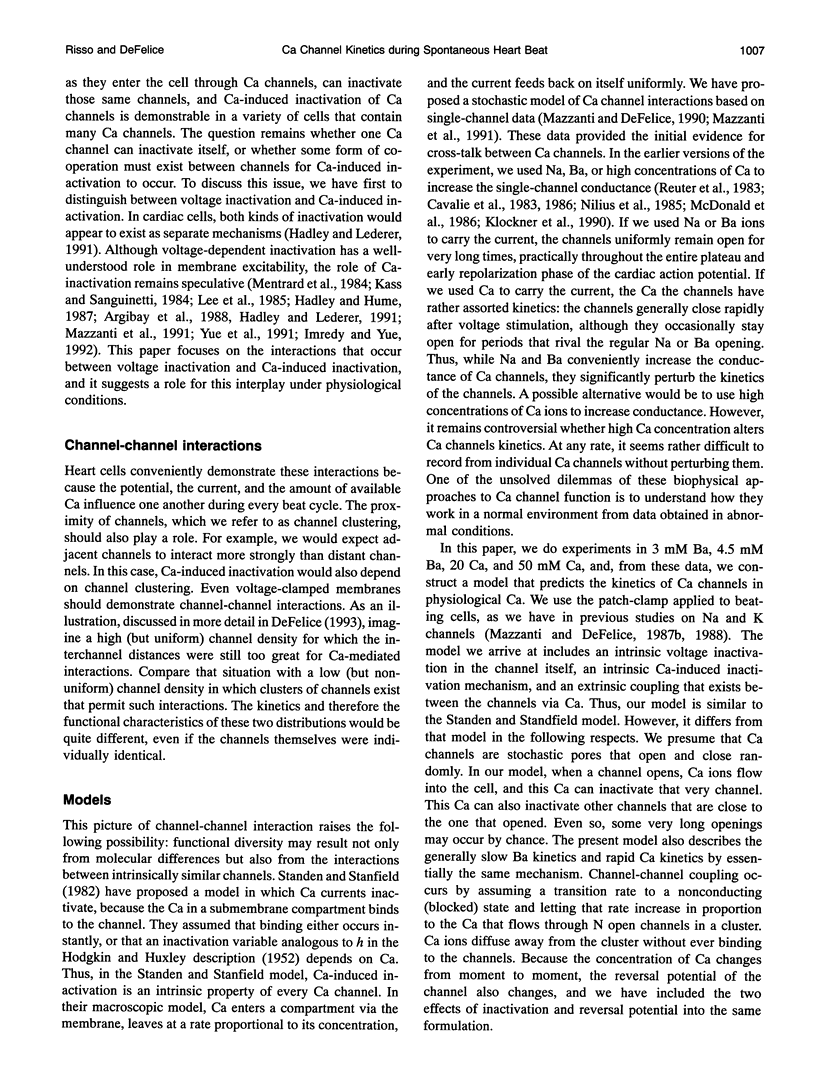
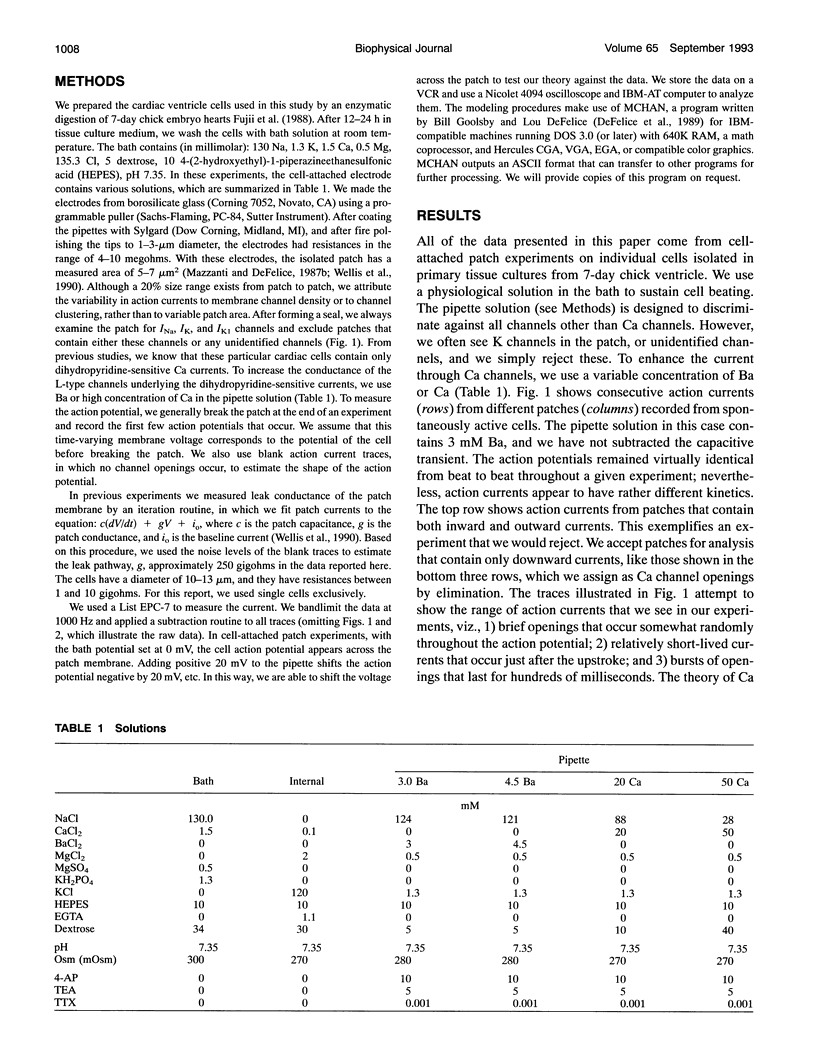
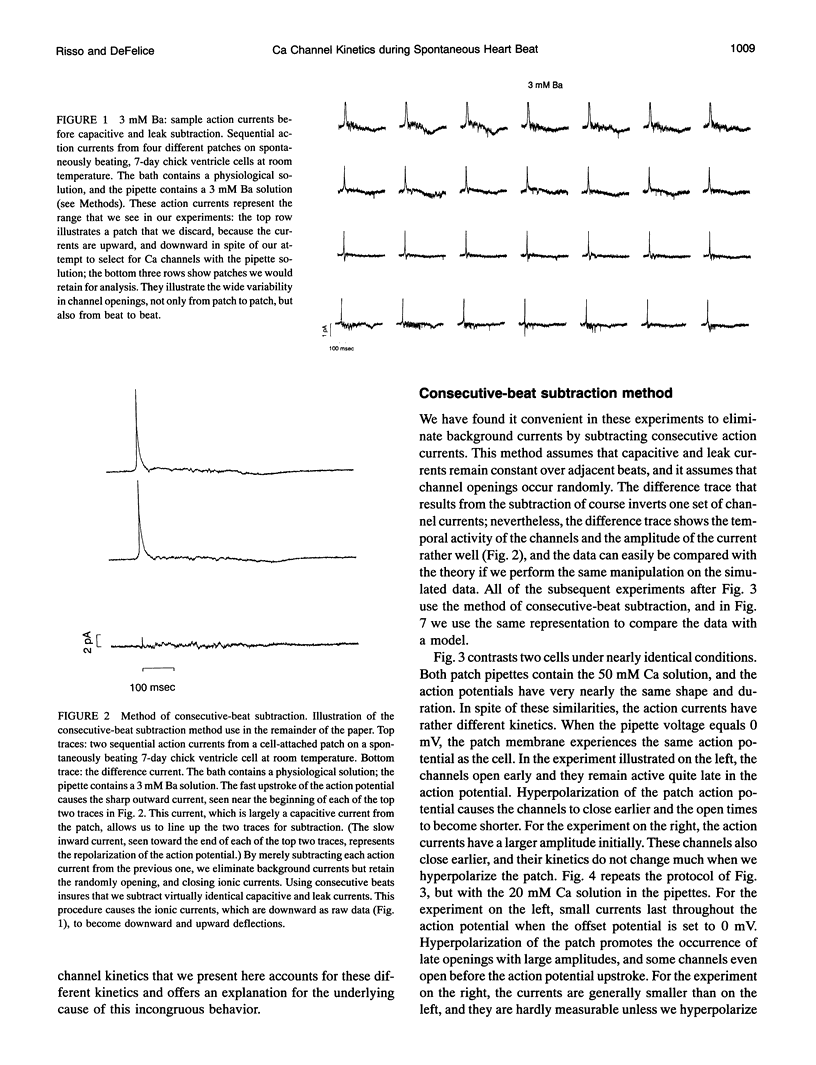
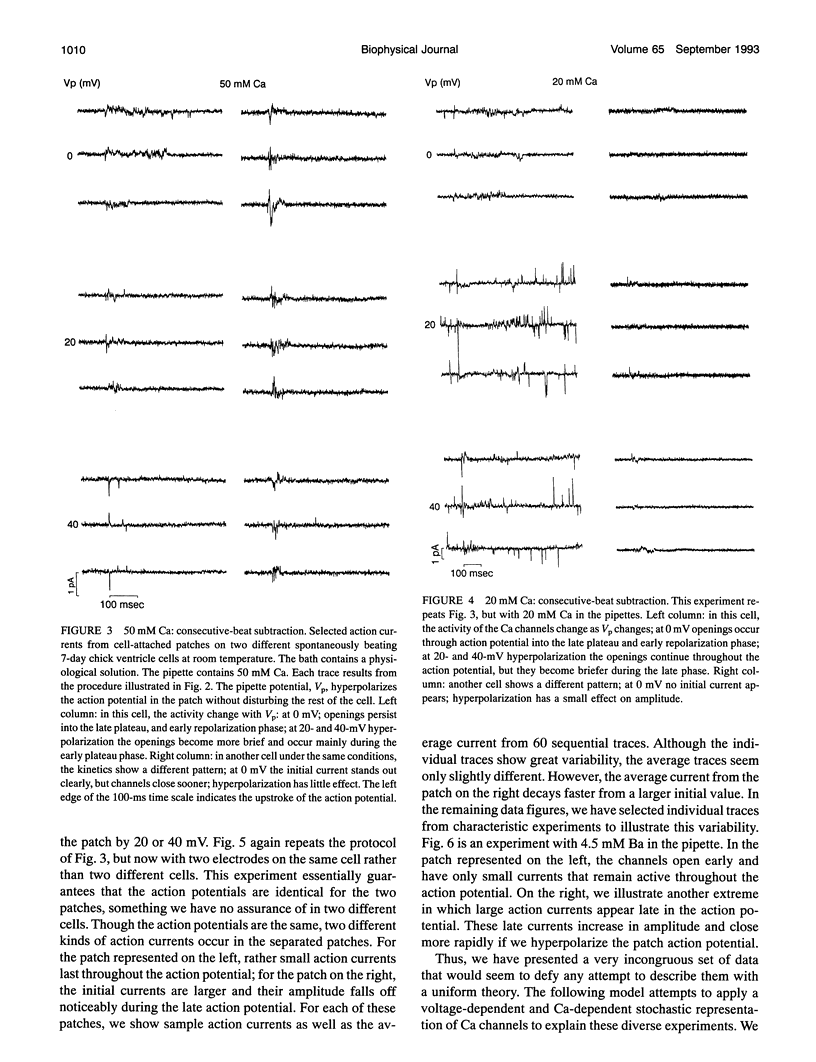
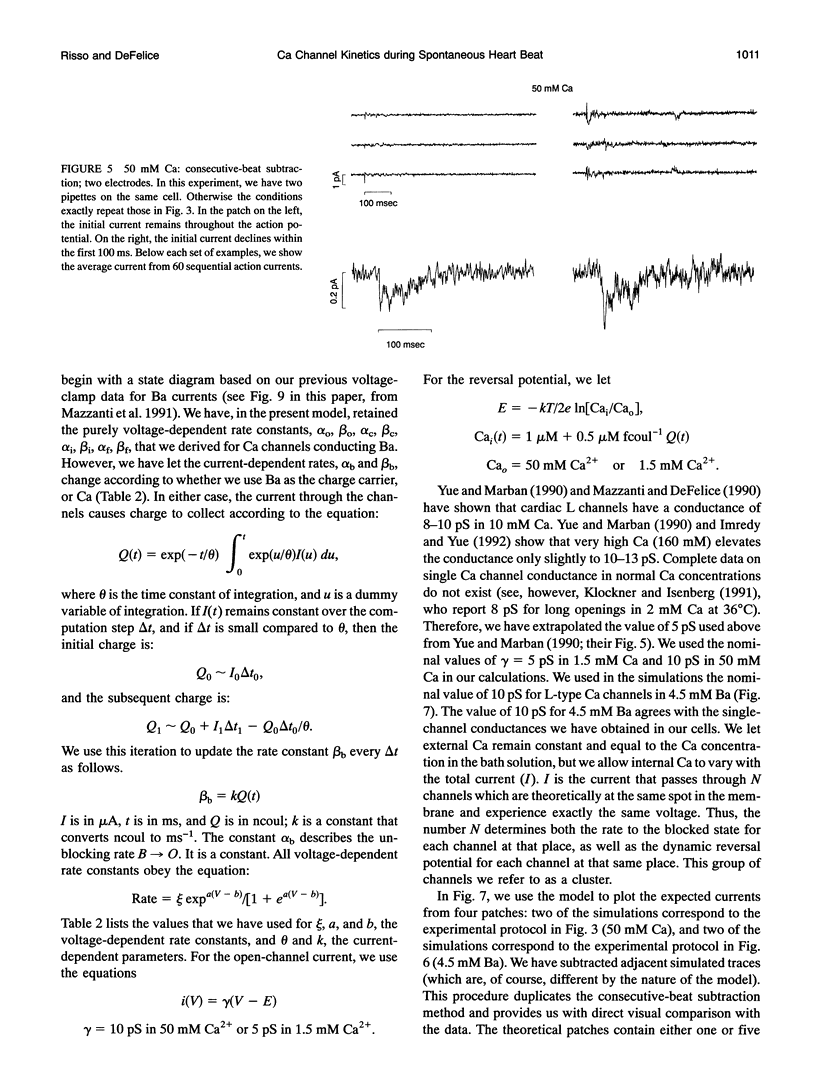
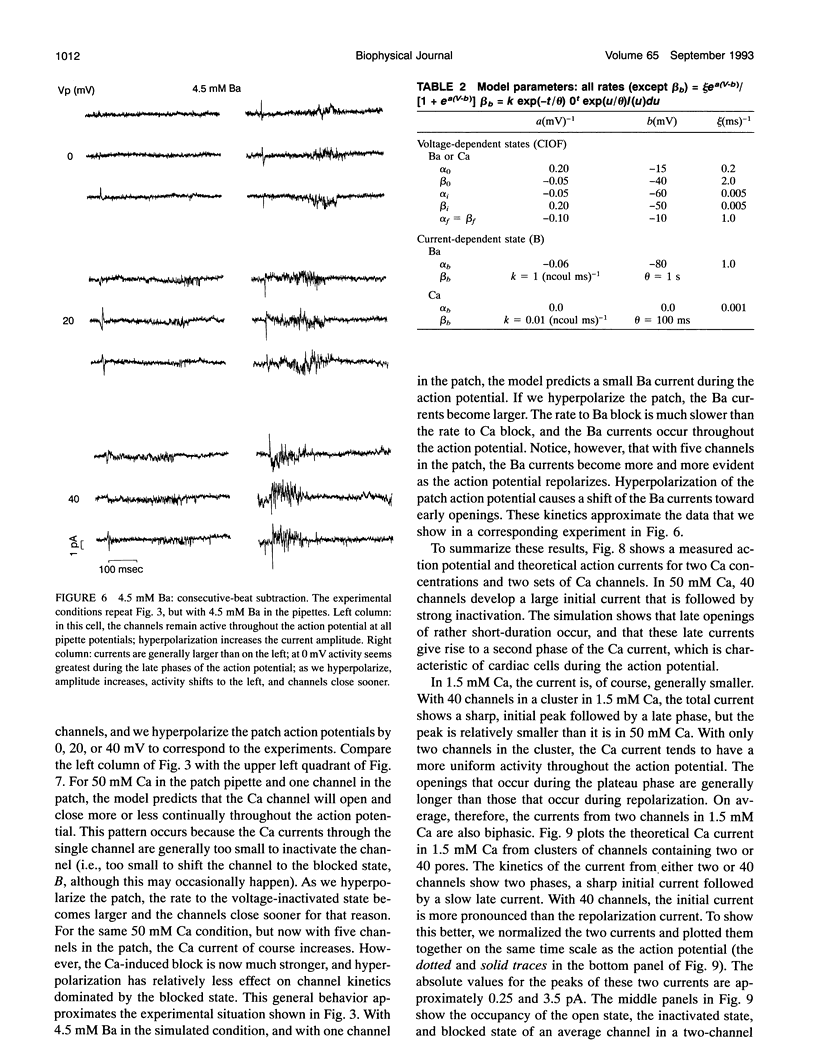
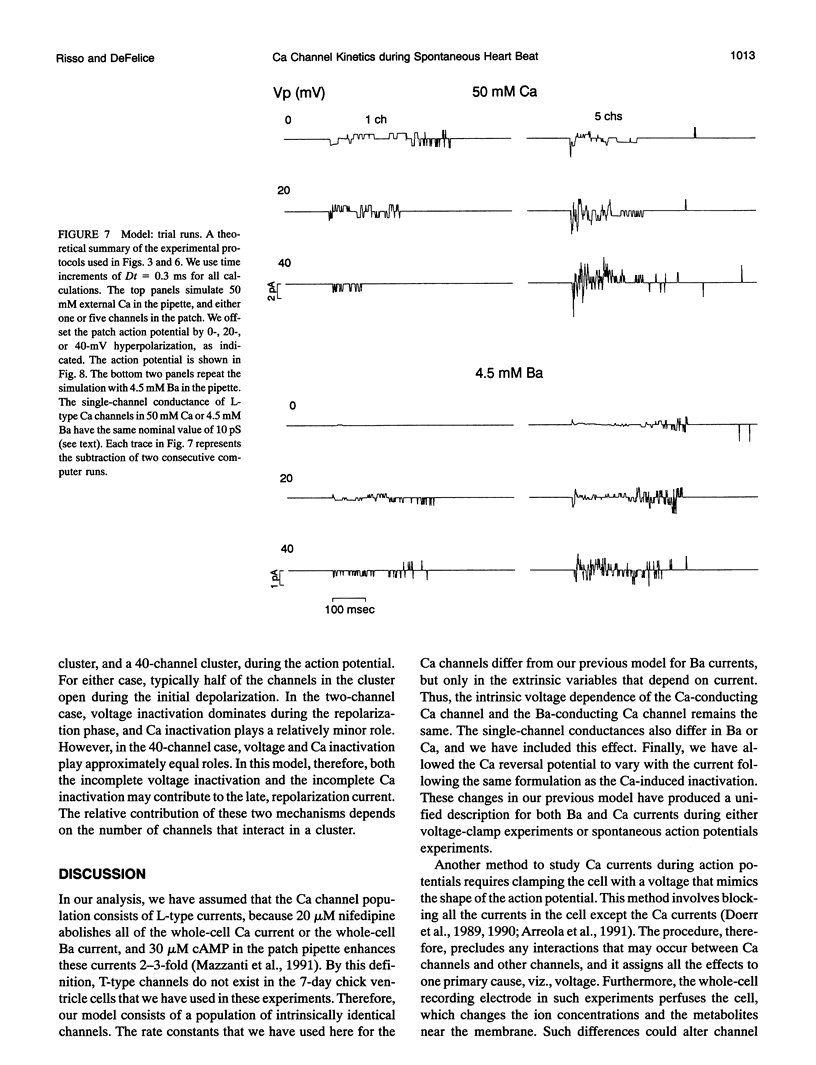
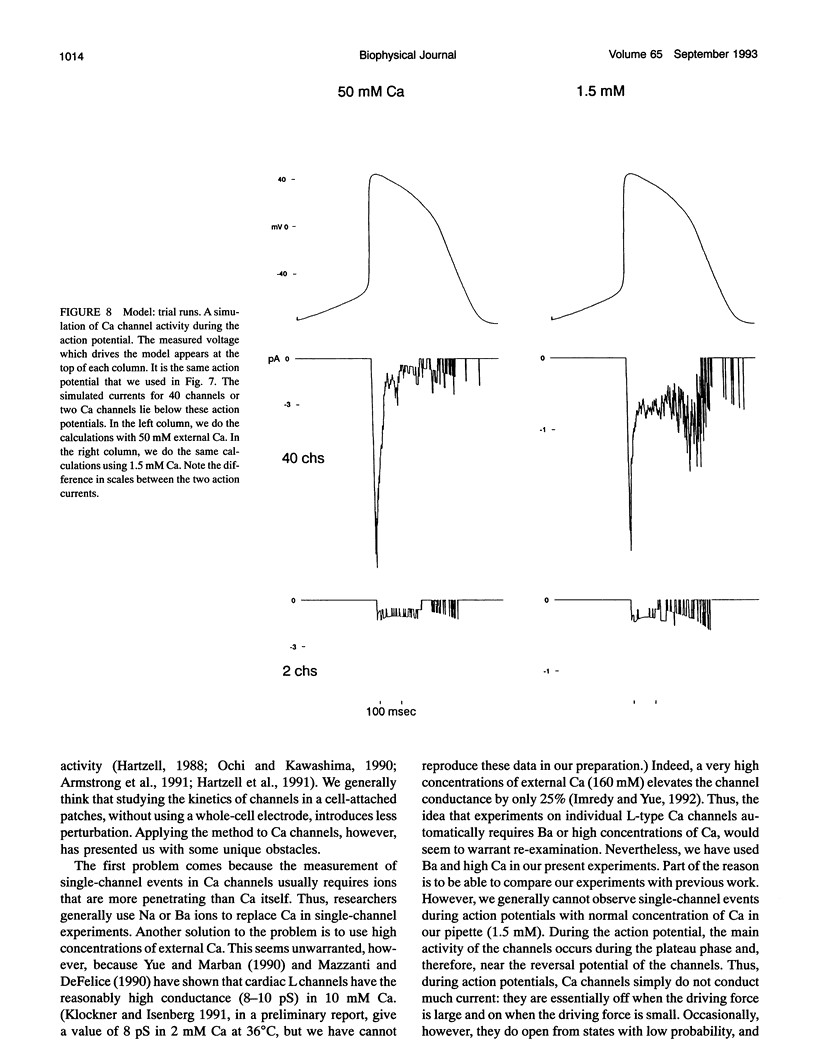
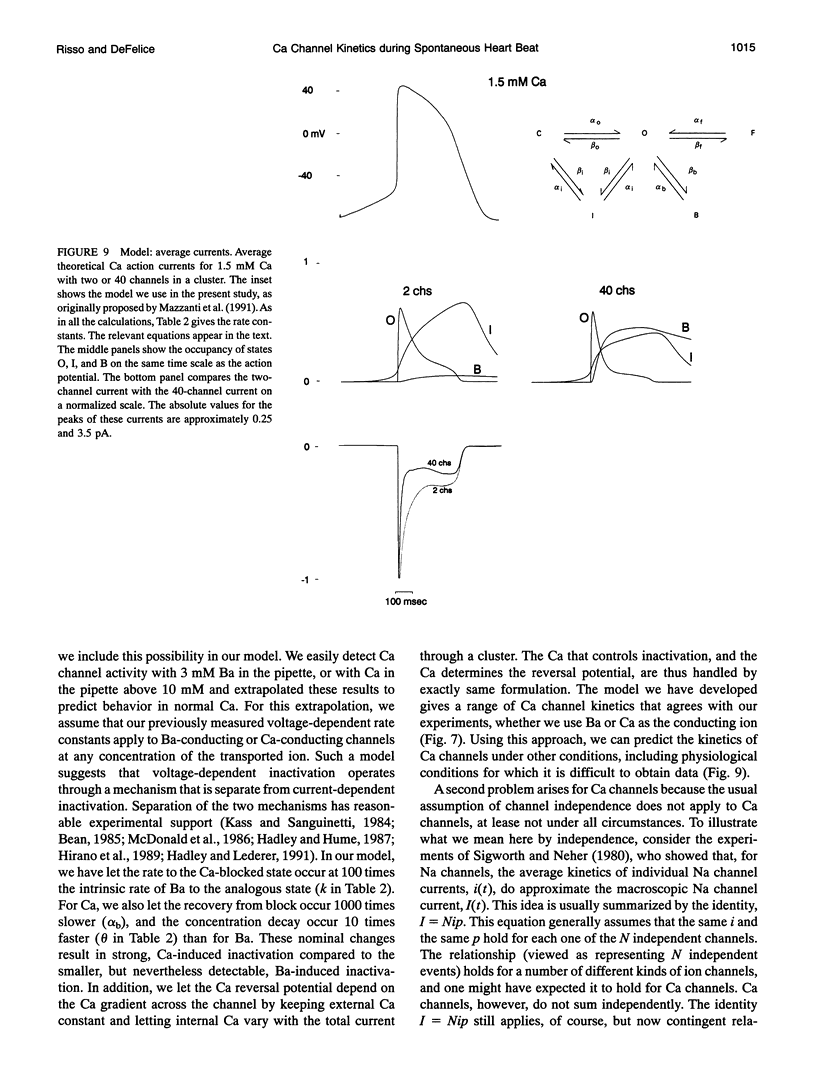
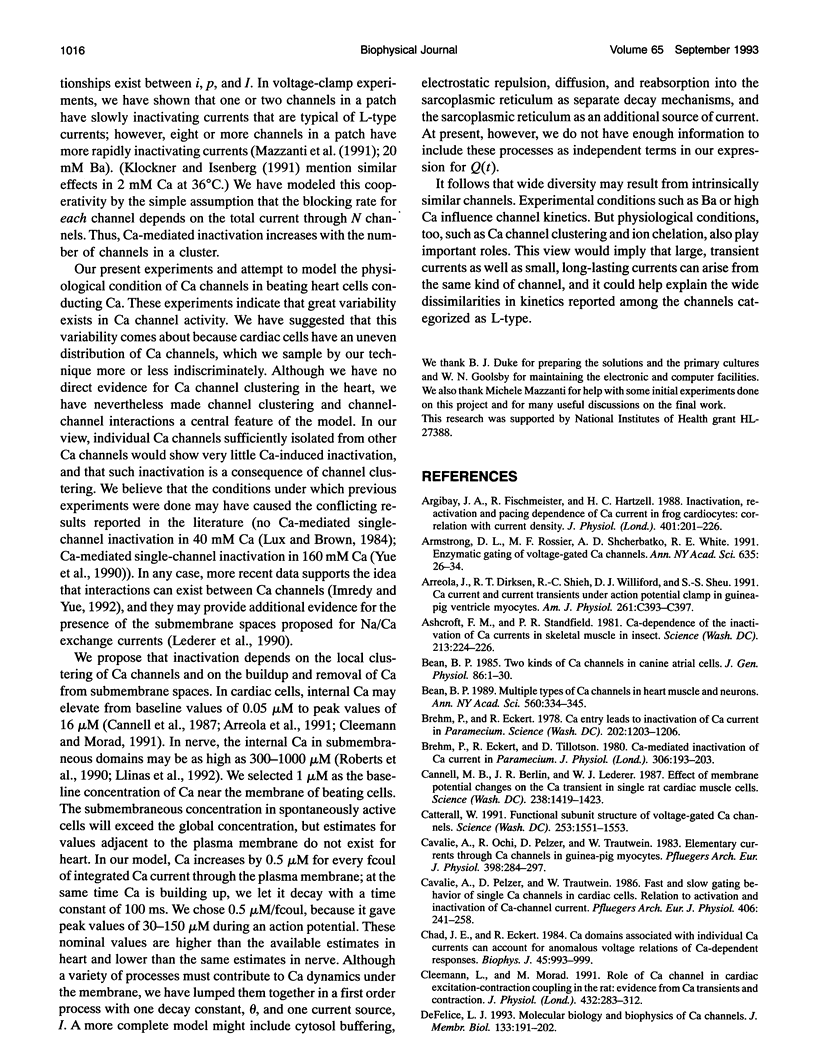
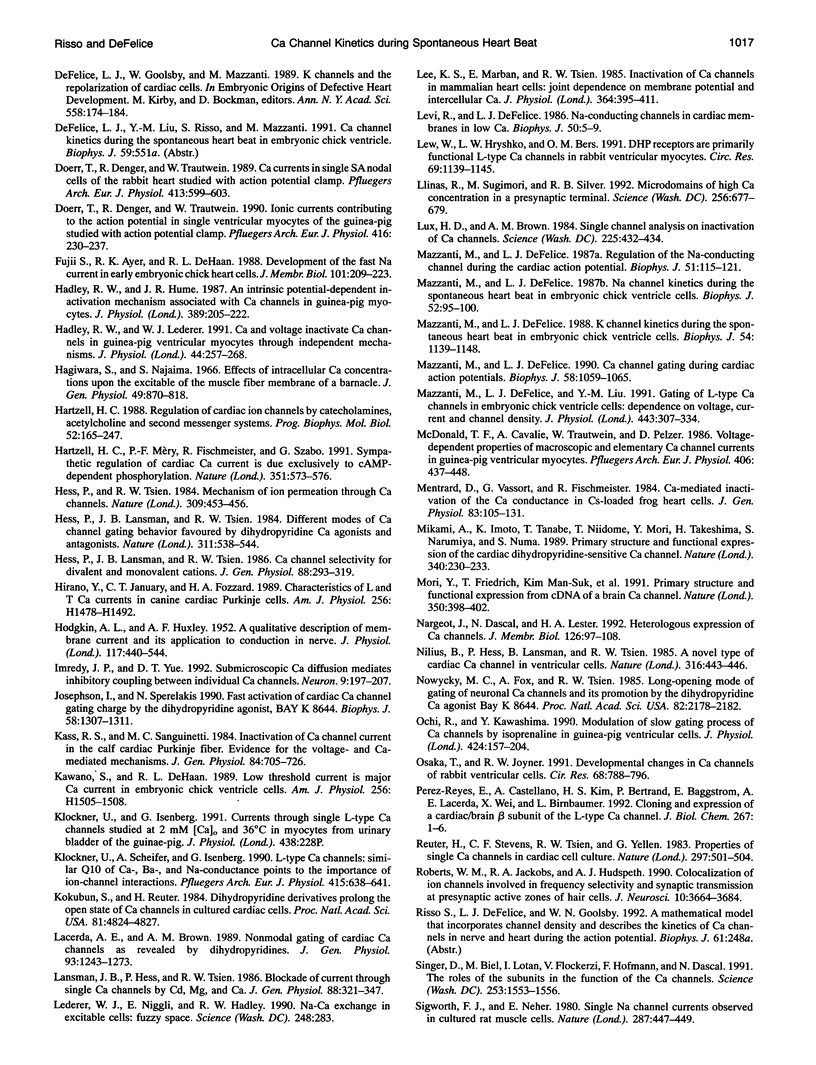
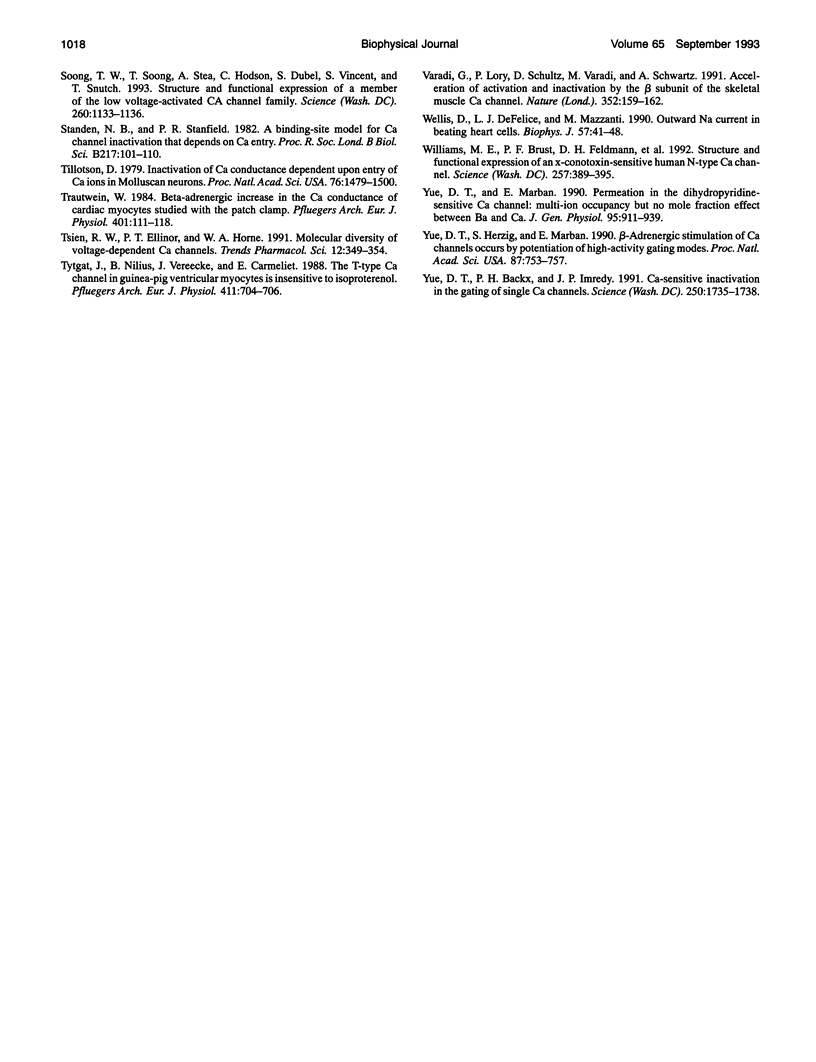
Selected References
These references are in PubMed. This may not be the complete list of references from this article.
- Argibay J. A., Fischmeister R., Hartzell H. C. Inactivation, reactivation and pacing dependence of calcium current in frog cardiocytes: correlation with current density. J Physiol. 1988 Jul;401:201–226. doi: 10.1113/jphysiol.1988.sp017158. [DOI] [PMC free article] [PubMed] [Google Scholar]
- Armstrong D. L., Rossier M. F., Shcherbatko A. D., White R. E. Enzymatic gating of voltage-activated calcium channels. Ann N Y Acad Sci. 1991;635:26–34. doi: 10.1111/j.1749-6632.1991.tb36478.x. [DOI] [PubMed] [Google Scholar]
- Arreola J., Dirksen R. T., Shieh R. C., Williford D. J., Sheu S. S. Ca2+ current and Ca2+ transients under action potential clamp in guinea pig ventricular myocytes. Am J Physiol. 1991 Aug;261(2 Pt 1):C393–C397. doi: 10.1152/ajpcell.1991.261.2.C393. [DOI] [PubMed] [Google Scholar]
- Ashcroft F. M., Stanfield P. R. Calcium dependence of the inactivation of calcium currents in skeletal muscle fibers of an insect. Science. 1981 Jul 10;213(4504):224–226. doi: 10.1126/science.213.4504.224. [DOI] [PubMed] [Google Scholar]
- Bean B. P. Multiple types of calcium channels in heart muscle and neurons. Modulation by drugs and neurotransmitters. Ann N Y Acad Sci. 1989;560:334–345. doi: 10.1111/j.1749-6632.1989.tb24113.x. [DOI] [PubMed] [Google Scholar]
- Bean B. P. Two kinds of calcium channels in canine atrial cells. Differences in kinetics, selectivity, and pharmacology. J Gen Physiol. 1985 Jul;86(1):1–30. doi: 10.1085/jgp.86.1.1. [DOI] [PMC free article] [PubMed] [Google Scholar]
- Brehm P., Eckert R. Calcium entry leads to inactivation of calcium channel in Paramecium. Science. 1978 Dec 15;202(4373):1203–1206. doi: 10.1126/science.103199. [DOI] [PubMed] [Google Scholar]
- Brehm P., Eckert R., Tillotson D. Calcium-mediated inactivation of calcium current in Paramecium. J Physiol. 1980 Sep;306:193–203. doi: 10.1113/jphysiol.1980.sp013391. [DOI] [PMC free article] [PubMed] [Google Scholar]
- Brum G., Osterrieder W., Trautwein W. Beta-adrenergic increase in the calcium conductance of cardiac myocytes studied with the patch clamp. Pflugers Arch. 1984 Jun;401(2):111–118. doi: 10.1007/BF00583870. [DOI] [PubMed] [Google Scholar]
- Cannell M. B., Berlin J. R., Lederer W. J. Effect of membrane potential changes on the calcium transient in single rat cardiac muscle cells. Science. 1987 Dec 4;238(4832):1419–1423. doi: 10.1126/science.2446391. [DOI] [PubMed] [Google Scholar]
- Cavalié A., Ochi R., Pelzer D., Trautwein W. Elementary currents through Ca2+ channels in guinea pig myocytes. Pflugers Arch. 1983 Sep;398(4):284–297. doi: 10.1007/BF00657238. [DOI] [PubMed] [Google Scholar]
- Cavalié A., Pelzer D., Trautwein W. Fast and slow gating behaviour of single calcium channels in cardiac cells. Relation to activation and inactivation of calcium-channel current. Pflugers Arch. 1986 Mar;406(3):241–258. doi: 10.1007/BF00640910. [DOI] [PubMed] [Google Scholar]
- Chad J. E., Eckert R. Calcium domains associated with individual channels can account for anomalous voltage relations of CA-dependent responses. Biophys J. 1984 May;45(5):993–999. doi: 10.1016/S0006-3495(84)84244-7. [DOI] [PMC free article] [PubMed] [Google Scholar]
- Cleemann L., Morad M. Role of Ca2+ channel in cardiac excitation-contraction coupling in the rat: evidence from Ca2+ transients and contraction. J Physiol. 1991 Jan;432:283–312. doi: 10.1113/jphysiol.1991.sp018385. [DOI] [PMC free article] [PubMed] [Google Scholar]
- DeFelice L. J. Molecular and biophysical view of the Ca channel: a hypothesis regarding oligomeric structure, channel clustering, and macroscopic current. J Membr Biol. 1993 May;133(3):191–202. doi: 10.1007/BF00232019. [DOI] [PubMed] [Google Scholar]
- Doerr T., Denger R., Doerr A., Trautwein W. Ionic currents contributing to the action potential in single ventricular myocytes of the guinea pig studied with action potential clamp. Pflugers Arch. 1990 May;416(3):230–237. doi: 10.1007/BF00392058. [DOI] [PubMed] [Google Scholar]
- Doerr T., Denger R., Trautwein W. Calcium currents in single SA nodal cells of the rabbit heart studied with action potential clamp. Pflugers Arch. 1989 Apr;413(6):599–603. doi: 10.1007/BF00581808. [DOI] [PubMed] [Google Scholar]
- Fujii S., Ayer R. K., Jr, DeHaan R. L. Development of the fast sodium current in early embryonic chick heart cells. J Membr Biol. 1988 Mar;101(3):209–223. doi: 10.1007/BF01872836. [DOI] [PubMed] [Google Scholar]
- HODGKIN A. L., HUXLEY A. F. A quantitative description of membrane current and its application to conduction and excitation in nerve. J Physiol. 1952 Aug;117(4):500–544. doi: 10.1113/jphysiol.1952.sp004764. [DOI] [PMC free article] [PubMed] [Google Scholar]
- Hadley R. W., Hume J. R. An intrinsic potential-dependent inactivation mechanism associated with calcium channels in guinea-pig myocytes. J Physiol. 1987 Aug;389:205–222. doi: 10.1113/jphysiol.1987.sp016654. [DOI] [PMC free article] [PubMed] [Google Scholar]
- Hadley R. W., Lederer W. J. Ca2+ and voltage inactivate Ca2+ channels in guinea-pig ventricular myocytes through independent mechanisms. J Physiol. 1991 Dec;444:257–268. doi: 10.1113/jphysiol.1991.sp018876. [DOI] [PMC free article] [PubMed] [Google Scholar]
- Hagiwara S., Nakajima S. Effects of the intracellular Ca ion concentration upon the excitability of the muscle fiber membrane of a barnacle. J Gen Physiol. 1966 Mar;49(4):807–818. doi: 10.1085/jgp.49.4.807. [DOI] [PMC free article] [PubMed] [Google Scholar]
- Hartzell H. C., Méry P. F., Fischmeister R., Szabo G. Sympathetic regulation of cardiac calcium current is due exclusively to cAMP-dependent phosphorylation. Nature. 1991 Jun 13;351(6327):573–576. doi: 10.1038/351573a0. [DOI] [PubMed] [Google Scholar]
- Hartzell H. C. Regulation of cardiac ion channels by catecholamines, acetylcholine and second messenger systems. Prog Biophys Mol Biol. 1988;52(3):165–247. doi: 10.1016/0079-6107(88)90014-4. [DOI] [PubMed] [Google Scholar]
- Hess P., Lansman J. B., Tsien R. W. Calcium channel selectivity for divalent and monovalent cations. Voltage and concentration dependence of single channel current in ventricular heart cells. J Gen Physiol. 1986 Sep;88(3):293–319. doi: 10.1085/jgp.88.3.293. [DOI] [PMC free article] [PubMed] [Google Scholar]
- Hess P., Lansman J. B., Tsien R. W. Different modes of Ca channel gating behaviour favoured by dihydropyridine Ca agonists and antagonists. Nature. 1984 Oct 11;311(5986):538–544. doi: 10.1038/311538a0. [DOI] [PubMed] [Google Scholar]
- Hess P., Tsien R. W. Mechanism of ion permeation through calcium channels. 1984 May 31-Jun 6Nature. 309(5967):453–456. doi: 10.1038/309453a0. [DOI] [PubMed] [Google Scholar]
- Hirano Y., Fozzard H. A., January C. T. Characteristics of L- and T-type Ca2+ currents in canine cardiac Purkinje cells. Am J Physiol. 1989 May;256(5 Pt 2):H1478–H1492. doi: 10.1152/ajpheart.1989.256.5.H1478. [DOI] [PubMed] [Google Scholar]
- Imredy J. P., Yue D. T. Submicroscopic Ca2+ diffusion mediates inhibitory coupling between individual Ca2+ channels. Neuron. 1992 Aug;9(2):197–207. doi: 10.1016/0896-6273(92)90159-b. [DOI] [PubMed] [Google Scholar]
- Josephson I. R., Sperelakis N. Fast activation of cardiac Ca++ channel gating charge by the dihydropyridine agonist, BAY K 8644. Biophys J. 1990 Nov;58(5):1307–1311. doi: 10.1016/S0006-3495(90)82471-1. [DOI] [PMC free article] [PubMed] [Google Scholar]
- Kass R. S., Sanguinetti M. C. Inactivation of calcium channel current in the calf cardiac Purkinje fiber. Evidence for voltage- and calcium-mediated mechanisms. J Gen Physiol. 1984 Nov;84(5):705–726. doi: 10.1085/jgp.84.5.705. [DOI] [PMC free article] [PubMed] [Google Scholar]
- Kawano S., DeHaan R. L. Low-threshold current is major calcium current in chick ventricle cells. Am J Physiol. 1989 May;256(5 Pt 2):H1505–H1508. doi: 10.1152/ajpheart.1989.256.5.H1505. [DOI] [PubMed] [Google Scholar]
- Khorana H. G. Rhodopsin, photoreceptor of the rod cell. An emerging pattern for structure and function. J Biol Chem. 1992 Jan 5;267(1):1–4. [PubMed] [Google Scholar]
- Klöckner U., Schiefer A., Isenberg G. L-type Ca-channels: similar Q10 of Ca-, Ba- and Na-conductance points to the importance of ion-channel interaction. Pflugers Arch. 1990 Feb;415(5):638–641. doi: 10.1007/BF02583518. [DOI] [PubMed] [Google Scholar]
- Kokubun S., Reuter H. Dihydropyridine derivatives prolong the open state of Ca channels in cultured cardiac cells. Proc Natl Acad Sci U S A. 1984 Aug;81(15):4824–4827. doi: 10.1073/pnas.81.15.4824. [DOI] [PMC free article] [PubMed] [Google Scholar]
- Lacerda A. E., Brown A. M. Nonmodal gating of cardiac calcium channels as revealed by dihydropyridines. J Gen Physiol. 1989 Jun;93(6):1243–1273. doi: 10.1085/jgp.93.6.1243. [DOI] [PMC free article] [PubMed] [Google Scholar]
- Lansman J. B., Hess P., Tsien R. W. Blockade of current through single calcium channels by Cd2+, Mg2+, and Ca2+. Voltage and concentration dependence of calcium entry into the pore. J Gen Physiol. 1986 Sep;88(3):321–347. doi: 10.1085/jgp.88.3.321. [DOI] [PMC free article] [PubMed] [Google Scholar]
- Lederer W. J., Niggli E., Hadley R. W. Sodium-calcium exchange in excitable cells: fuzzy space. Science. 1990 Apr 20;248(4953):283–283. doi: 10.1126/science.2326638. [DOI] [PubMed] [Google Scholar]
- Lee K. S., Marban E., Tsien R. W. Inactivation of calcium channels in mammalian heart cells: joint dependence on membrane potential and intracellular calcium. J Physiol. 1985 Jul;364:395–411. doi: 10.1113/jphysiol.1985.sp015752. [DOI] [PMC free article] [PubMed] [Google Scholar]
- Levi R., DeFelice L. J. Sodium-conducting channels in cardiac membranes in low calcium. Biophys J. 1986 Jul;50(1):5–9. doi: 10.1016/S0006-3495(86)83433-6. [DOI] [PMC free article] [PubMed] [Google Scholar]
- Lew W. Y., Hryshko L. V., Bers D. M. Dihydropyridine receptors are primarily functional L-type calcium channels in rabbit ventricular myocytes. Circ Res. 1991 Oct;69(4):1139–1145. doi: 10.1161/01.res.69.4.1139. [DOI] [PubMed] [Google Scholar]
- Llinás R., Sugimori M., Silver R. B. Microdomains of high calcium concentration in a presynaptic terminal. Science. 1992 May 1;256(5057):677–679. doi: 10.1126/science.1350109. [DOI] [PubMed] [Google Scholar]
- Lux H. D., Brown A. M. Single channel studies on inactivation of calcium currents. Science. 1984 Jul 27;225(4660):432–434. doi: 10.1126/science.6330896. [DOI] [PubMed] [Google Scholar]
- Mazzanti M., DeFelice L. J. Ca channel gating during cardiac action potentials. Biophys J. 1990 Oct;58(4):1059–1065. doi: 10.1016/S0006-3495(90)82448-6. [DOI] [PMC free article] [PubMed] [Google Scholar]
- Mazzanti M., DeFelice L. J. K channel kinetics during the spontaneous heart beat in embryonic chick ventricle cells. Biophys J. 1988 Dec;54(6):1139–1148. doi: 10.1016/S0006-3495(88)83048-0. [DOI] [PMC free article] [PubMed] [Google Scholar]
- Mazzanti M., DeFelice L. J., Liu Y. M. Gating of L-type Ca2+ channels in embryonic chick ventricle cells: dependence on voltage, current and channel density. J Physiol. 1991 Nov;443:307–334. doi: 10.1113/jphysiol.1991.sp018835. [DOI] [PMC free article] [PubMed] [Google Scholar]
- Mazzanti M., DeFelice L. J. Na channel kinetics during the spontaneous heart beat in embryonic chick ventricle cells. Biophys J. 1987 Jul;52(1):95–100. doi: 10.1016/S0006-3495(87)83192-2. [DOI] [PMC free article] [PubMed] [Google Scholar]
- Mazzanti M., DeFelice L. J. Regulation of the Na-conducting Ca channel during the cardiac action potential. Biophys J. 1987 Jan;51(1):115–121. doi: 10.1016/S0006-3495(87)83316-7. [DOI] [PMC free article] [PubMed] [Google Scholar]
- McDonald T. F., Cavalié A., Trautwein W., Pelzer D. Voltage-dependent properties of macroscopic and elementary calcium channel currents in guinea pig ventricular myocytes. Pflugers Arch. 1986 May;406(5):437–448. doi: 10.1007/BF00583365. [DOI] [PubMed] [Google Scholar]
- Mentrard D., Vassort G., Fischmeister R. Calcium-mediated inactivation of the calcium conductance in cesium-loaded frog heart cells. J Gen Physiol. 1984 Jan;83(1):105–131. doi: 10.1085/jgp.83.1.105. [DOI] [PMC free article] [PubMed] [Google Scholar]
- Mikami A., Imoto K., Tanabe T., Niidome T., Mori Y., Takeshima H., Narumiya S., Numa S. Primary structure and functional expression of the cardiac dihydropyridine-sensitive calcium channel. Nature. 1989 Jul 20;340(6230):230–233. doi: 10.1038/340230a0. [DOI] [PubMed] [Google Scholar]
- Mori Y., Friedrich T., Kim M. S., Mikami A., Nakai J., Ruth P., Bosse E., Hofmann F., Flockerzi V., Furuichi T. Primary structure and functional expression from complementary DNA of a brain calcium channel. Nature. 1991 Apr 4;350(6317):398–402. doi: 10.1038/350398a0. [DOI] [PubMed] [Google Scholar]
- Nargeot J., Dascal N., Lester H. A. Heterologous expression of calcium channels. J Membr Biol. 1992 Mar;126(2):97–108. doi: 10.1007/BF00231908. [DOI] [PubMed] [Google Scholar]
- Nilius B., Hess P., Lansman J. B., Tsien R. W. A novel type of cardiac calcium channel in ventricular cells. Nature. 1985 Aug 1;316(6027):443–446. doi: 10.1038/316443a0. [DOI] [PubMed] [Google Scholar]
- Nowycky M. C., Fox A. P., Tsien R. W. Long-opening mode of gating of neuronal calcium channels and its promotion by the dihydropyridine calcium agonist Bay K 8644. Proc Natl Acad Sci U S A. 1985 Apr;82(7):2178–2182. doi: 10.1073/pnas.82.7.2178. [DOI] [PMC free article] [PubMed] [Google Scholar]
- Ochi R., Kawashima Y. Modulation of slow gating process of calcium channels by isoprenaline in guinea-pig ventricular cells. J Physiol. 1990 May;424:187–204. doi: 10.1113/jphysiol.1990.sp018062. [DOI] [PMC free article] [PubMed] [Google Scholar]
- Osaka T., Joyner R. W. Developmental changes in calcium currents of rabbit ventricular cells. Circ Res. 1991 Mar;68(3):788–796. doi: 10.1161/01.res.68.3.788. [DOI] [PubMed] [Google Scholar]
- Reuter H., Stevens C. F., Tsien R. W., Yellen G. Properties of single calcium channels in cardiac cell culture. Nature. 1982 Jun 10;297(5866):501–504. doi: 10.1038/297501a0. [DOI] [PubMed] [Google Scholar]
- Roberts W. M., Jacobs R. A., Hudspeth A. J. Colocalization of ion channels involved in frequency selectivity and synaptic transmission at presynaptic active zones of hair cells. J Neurosci. 1990 Nov;10(11):3664–3684. doi: 10.1523/JNEUROSCI.10-11-03664.1990. [DOI] [PMC free article] [PubMed] [Google Scholar]
- Sigworth F. J., Neher E. Single Na+ channel currents observed in cultured rat muscle cells. Nature. 1980 Oct 2;287(5781):447–449. doi: 10.1038/287447a0. [DOI] [PubMed] [Google Scholar]
- Singer D., Biel M., Lotan I., Flockerzi V., Hofmann F., Dascal N. The roles of the subunits in the function of the calcium channel. Science. 1991 Sep 27;253(5027):1553–1557. doi: 10.1126/science.1716787. [DOI] [PubMed] [Google Scholar]
- Soong T. W., Stea A., Hodson C. D., Dubel S. J., Vincent S. R., Snutch T. P. Structure and functional expression of a member of the low voltage-activated calcium channel family. Science. 1993 May 21;260(5111):1133–1136. doi: 10.1126/science.8388125. [DOI] [PubMed] [Google Scholar]
- Standen N. B., Stanfield P. R. A binding-site model for calcium channel inactivation that depends on calcium entry. Proc R Soc Lond B Biol Sci. 1982 Dec 22;217(1206):101–110. doi: 10.1098/rspb.1982.0097. [DOI] [PubMed] [Google Scholar]
- Tillotson D. Inactivation of Ca conductance dependent on entry of Ca ions in molluscan neurons. Proc Natl Acad Sci U S A. 1979 Mar;76(3):1497–1500. doi: 10.1073/pnas.76.3.1497. [DOI] [PMC free article] [PubMed] [Google Scholar]
- Tsien R. W., Ellinor P. T., Horne W. A. Molecular diversity of voltage-dependent Ca2+ channels. Trends Pharmacol Sci. 1991 Sep;12(9):349–354. doi: 10.1016/0165-6147(91)90595-j. [DOI] [PubMed] [Google Scholar]
- Tytgat J., Nilius B., Vereecke J., Carmeliet E. The T-type Ca channel in guinea-pig ventricular myocytes is insensitive to isoproterenol. Pflugers Arch. 1988 Jun;411(6):704–706. doi: 10.1007/BF00580872. [DOI] [PubMed] [Google Scholar]
- Varadi G., Lory P., Schultz D., Varadi M., Schwartz A. Acceleration of activation and inactivation by the beta subunit of the skeletal muscle calcium channel. Nature. 1991 Jul 11;352(6331):159–162. doi: 10.1038/352159a0. [DOI] [PubMed] [Google Scholar]
- Wellis D. P., DeFelice L. J., Mazzanti M. Outward sodium current in beating heart cells. Biophys J. 1990 Jan;57(1):41–48. doi: 10.1016/S0006-3495(90)82505-4. [DOI] [PMC free article] [PubMed] [Google Scholar]
- Williams M. E., Brust P. F., Feldman D. H., Patthi S., Simerson S., Maroufi A., McCue A. F., Veliçelebi G., Ellis S. B., Harpold M. M. Structure and functional expression of an omega-conotoxin-sensitive human N-type calcium channel. Science. 1992 Jul 17;257(5068):389–395. doi: 10.1126/science.1321501. [DOI] [PubMed] [Google Scholar]
- Yue D. T., Backx P. H., Imredy J. P. Calcium-sensitive inactivation in the gating of single calcium channels. Science. 1990 Dec 21;250(4988):1735–1738. doi: 10.1126/science.2176745. [DOI] [PubMed] [Google Scholar]
- Yue D. T., Herzig S., Marban E. Beta-adrenergic stimulation of calcium channels occurs by potentiation of high-activity gating modes. Proc Natl Acad Sci U S A. 1990 Jan;87(2):753–757. doi: 10.1073/pnas.87.2.753. [DOI] [PMC free article] [PubMed] [Google Scholar]
- Yue D. T., Marban E. Permeation in the dihydropyridine-sensitive calcium channel. Multi-ion occupancy but no anomalous mole-fraction effect between Ba2+ and Ca2+. J Gen Physiol. 1990 May;95(5):911–939. doi: 10.1085/jgp.95.5.911. [DOI] [PMC free article] [PubMed] [Google Scholar]