Abstract
An empirical model of a liquid crystalline (L alpha phase) phosphatidylcholine (PC) bilayer interface is presented along with a function which calculates the position-dependent energy of associated solutes. The model approximates the interface as a gradual two-step transition, the first step being from an aqueous phase to a phase of reduced polarity, but which maintains a high enough concentration of water and/or polar head group moieties to satisfy the hydrogen bond-forming potential of the solute. The second transition is from the hydrogen bonding/low polarity region to an effectively anhydrous hydrocarbon phase. The "interfacial energies" of solutes within this variable medium are calculated based upon atomic positions and atomic parameters describing general polarity and hydrogen bond donor/acceptor propensities. This function was tested for its ability to reproduce experimental water-solvent partitioning energies and water-bilayer partitioning data. In both cases, the experimental data was reproduced fairly well. Energy minimizations carried out on beta-hexyl glucopyranoside led to identification of a global minimum for the interface-associated glycolipid which exhibited glycosidic torsion angles in agreement with prior results (Hare, B.J., K.P. Howard, and J.H. Prestegard. 1993. Biophys. J. 64:392-398). Molecular dynamics simulations carried out upon this same molecule within the simulated interface led to results which were consistent with a number of experimentally based conclusions from previous work, but failed to quantitatively reproduce an available NMR quadrupolar/dipolar coupling data set (Sanders, C.R., and J.H. Prestegard. 1991. J. Am. Chem. Soc. 113:1987-1996). The proposed model and functions are readily incorporated into computational energy modeling algorithms and may prove useful in future studies of membrane-associated molecules.
Full text
PDF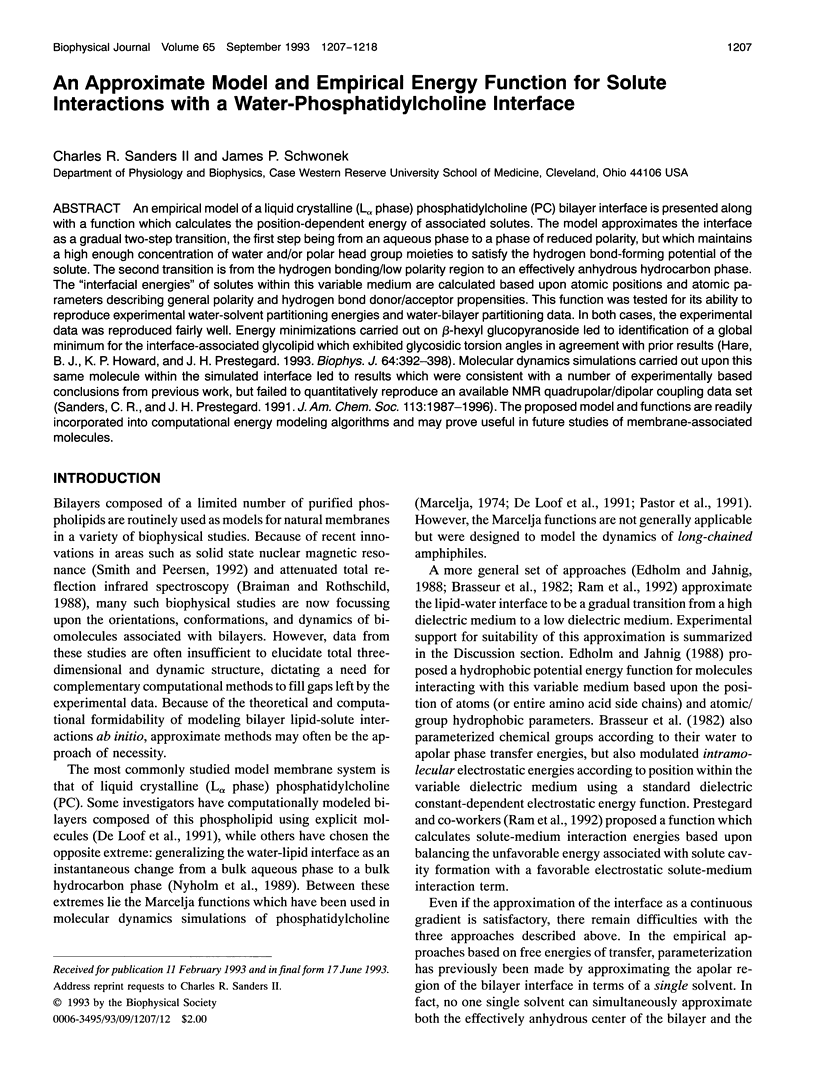
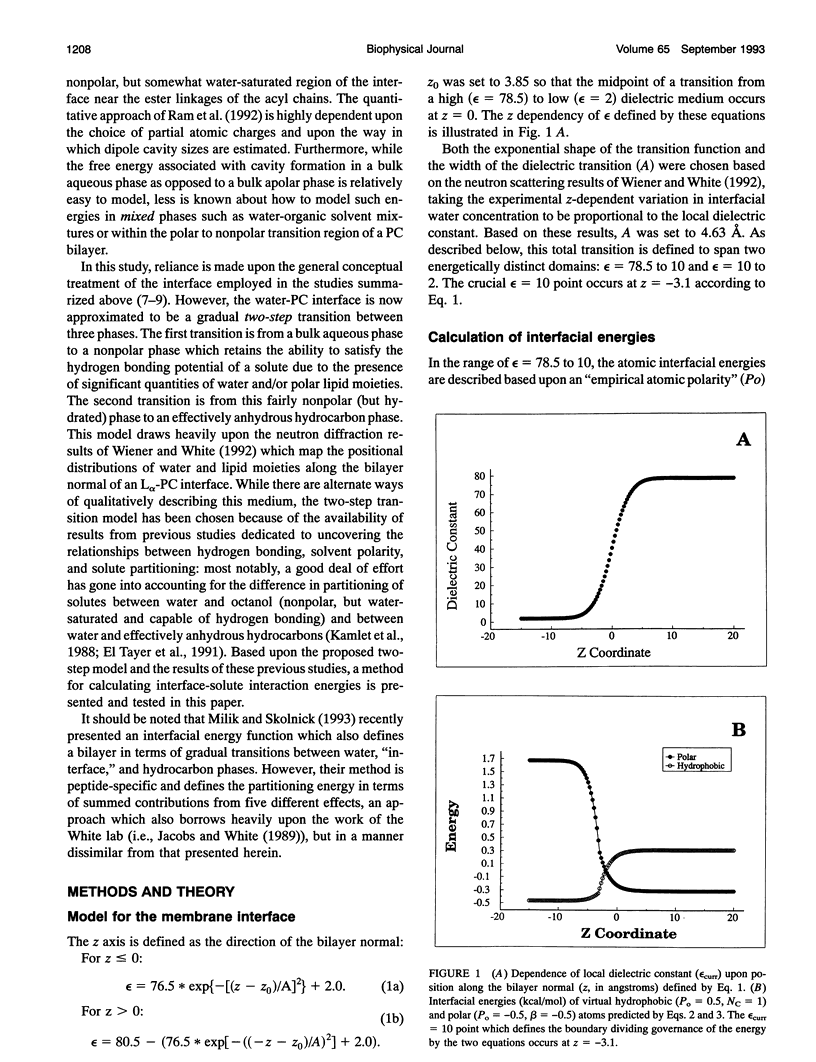
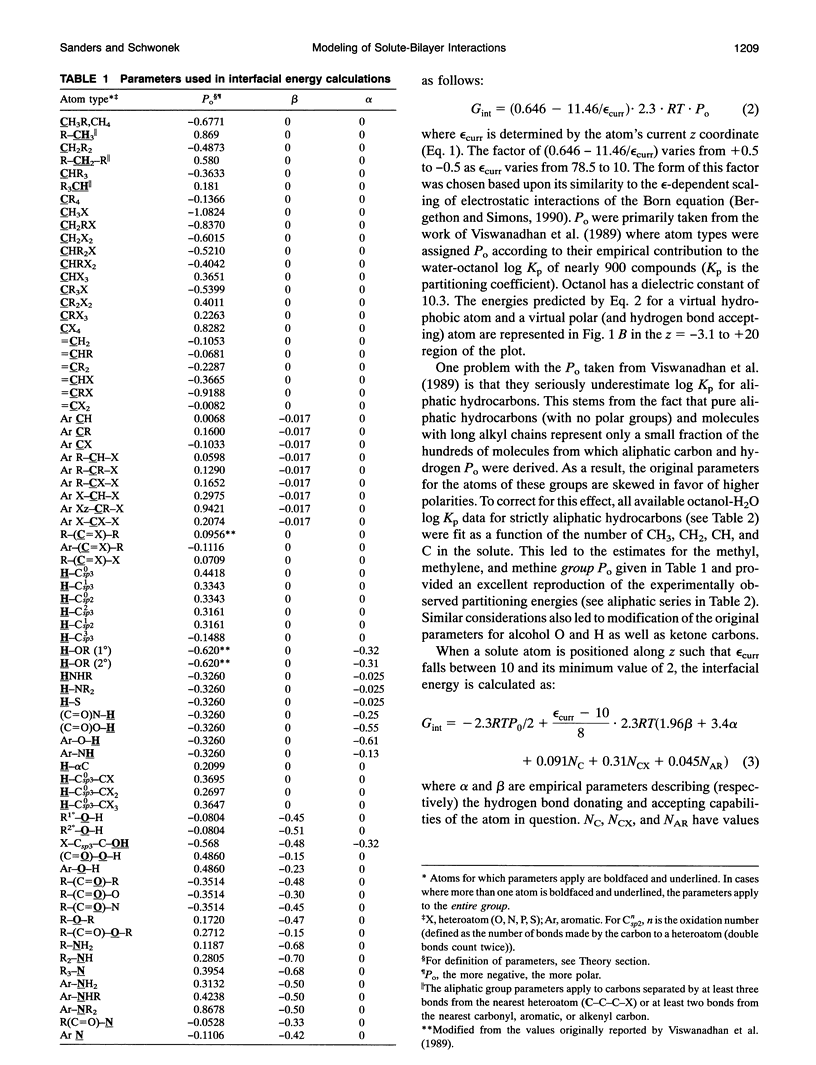
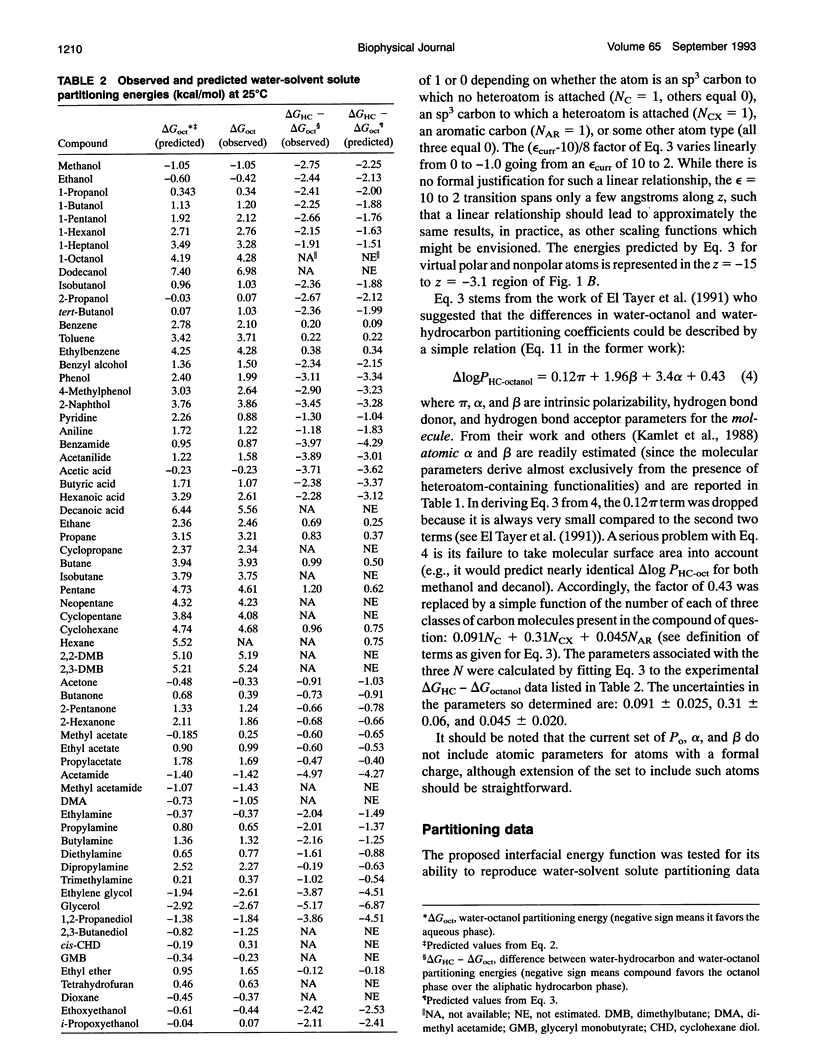
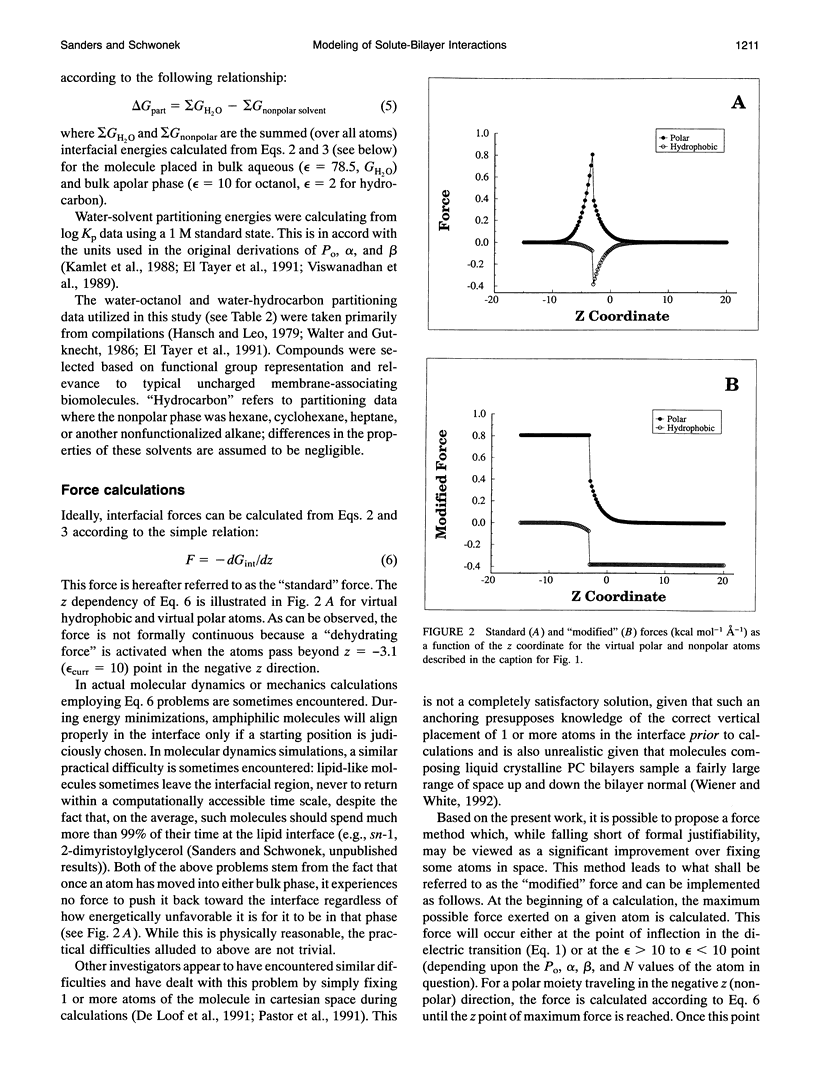
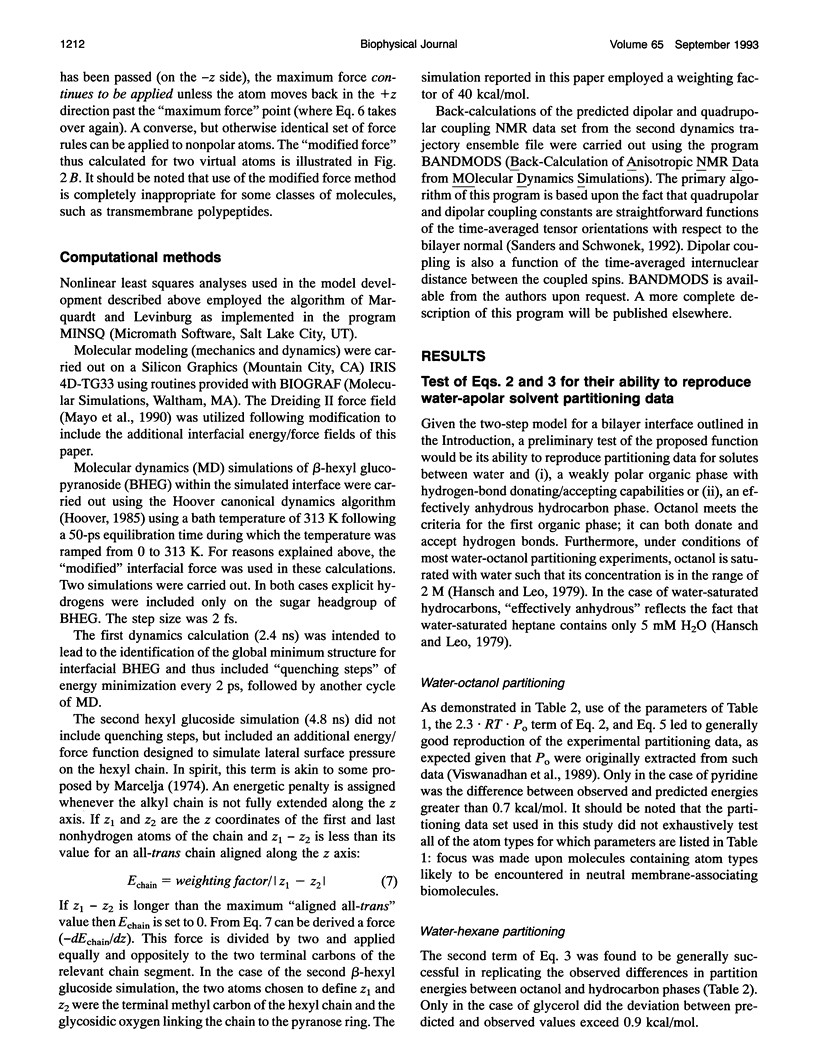
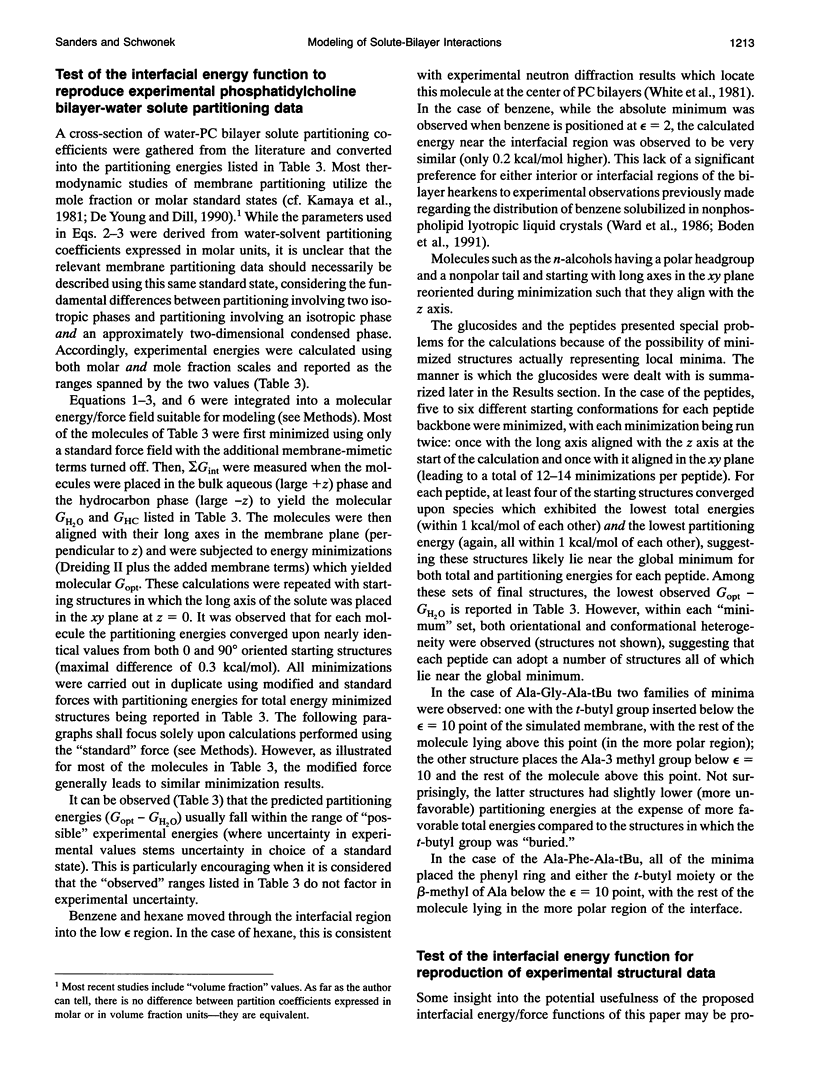
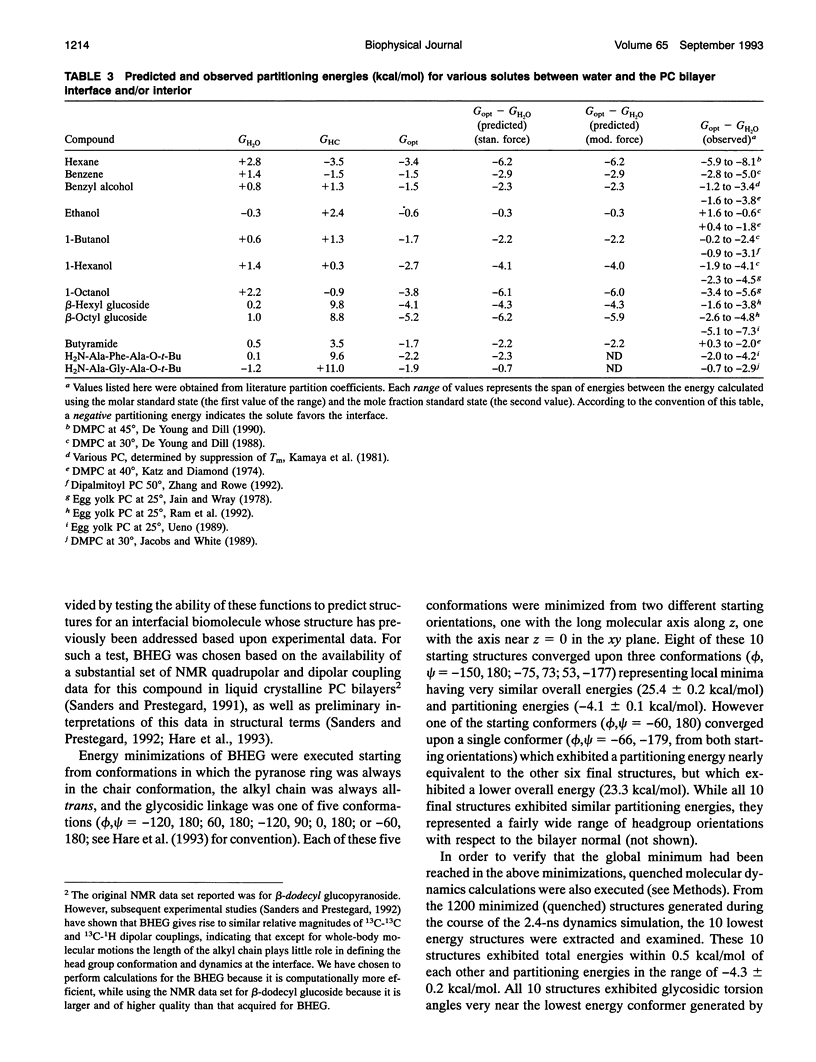
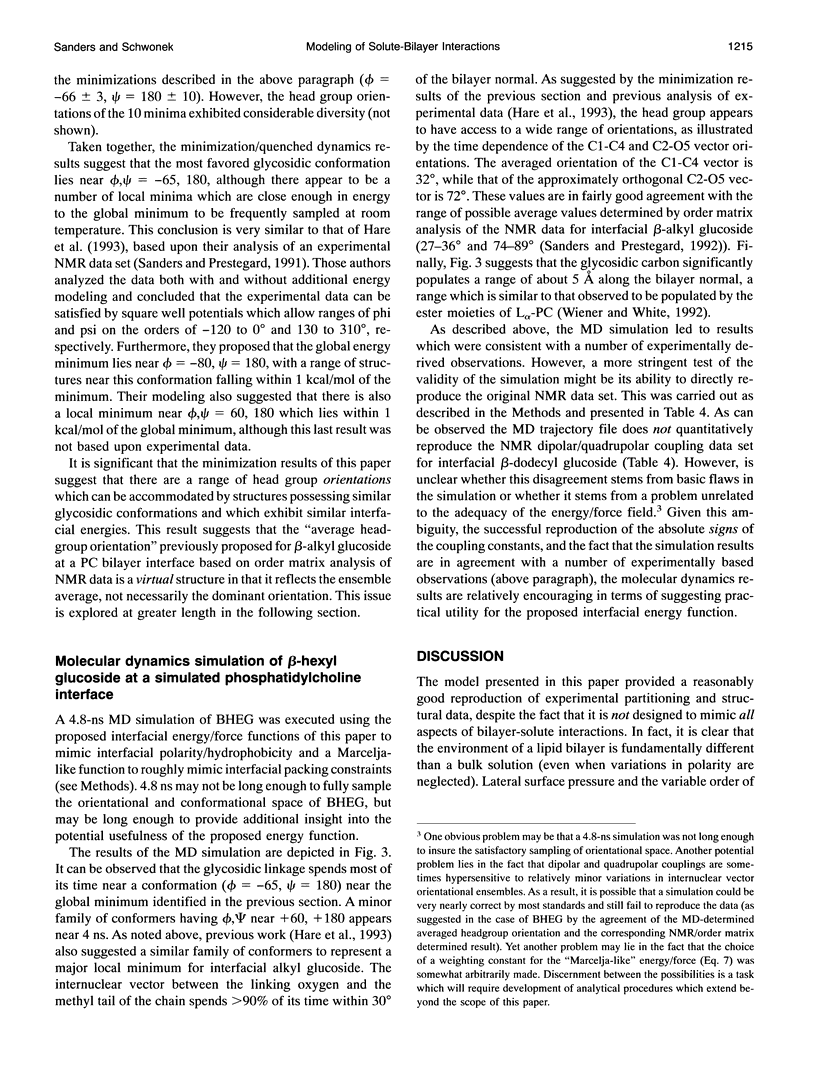
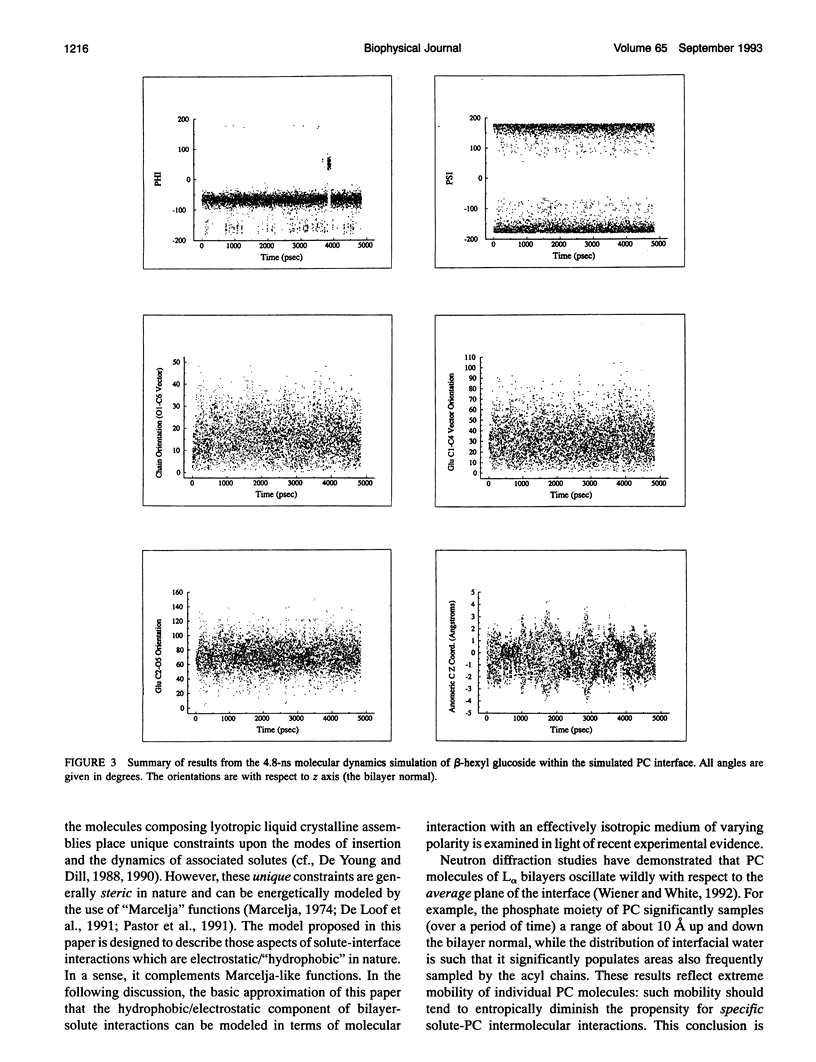
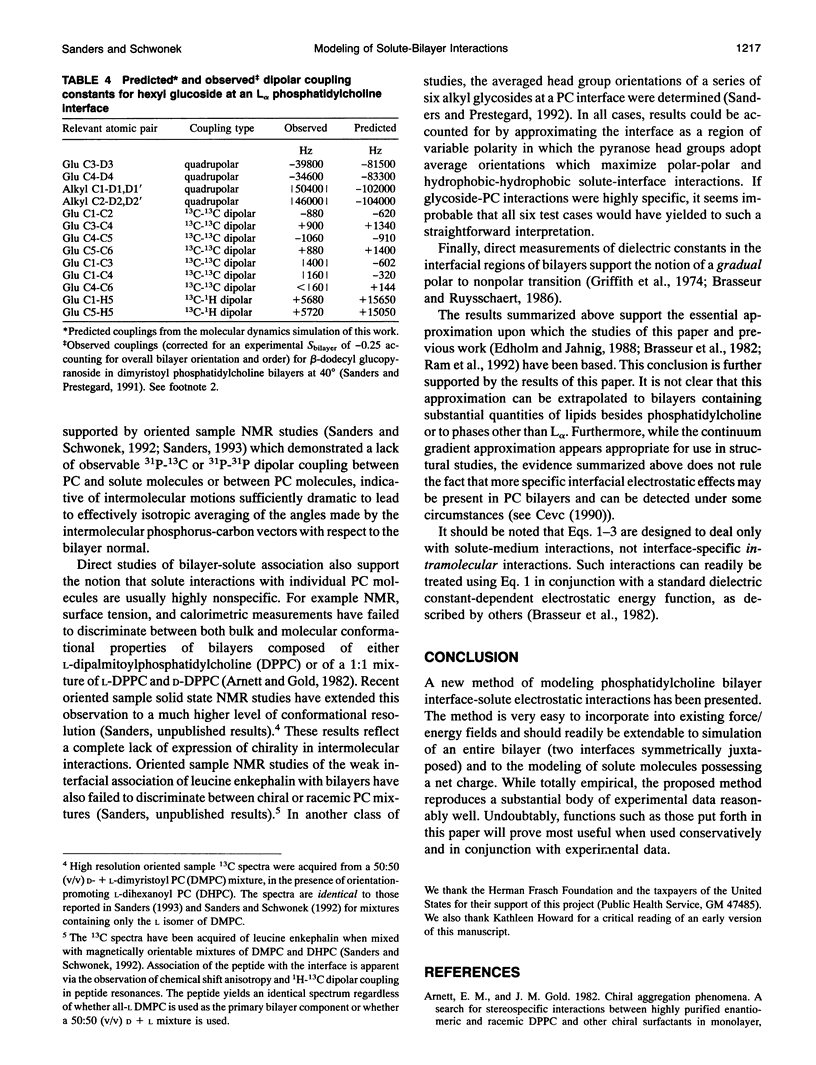
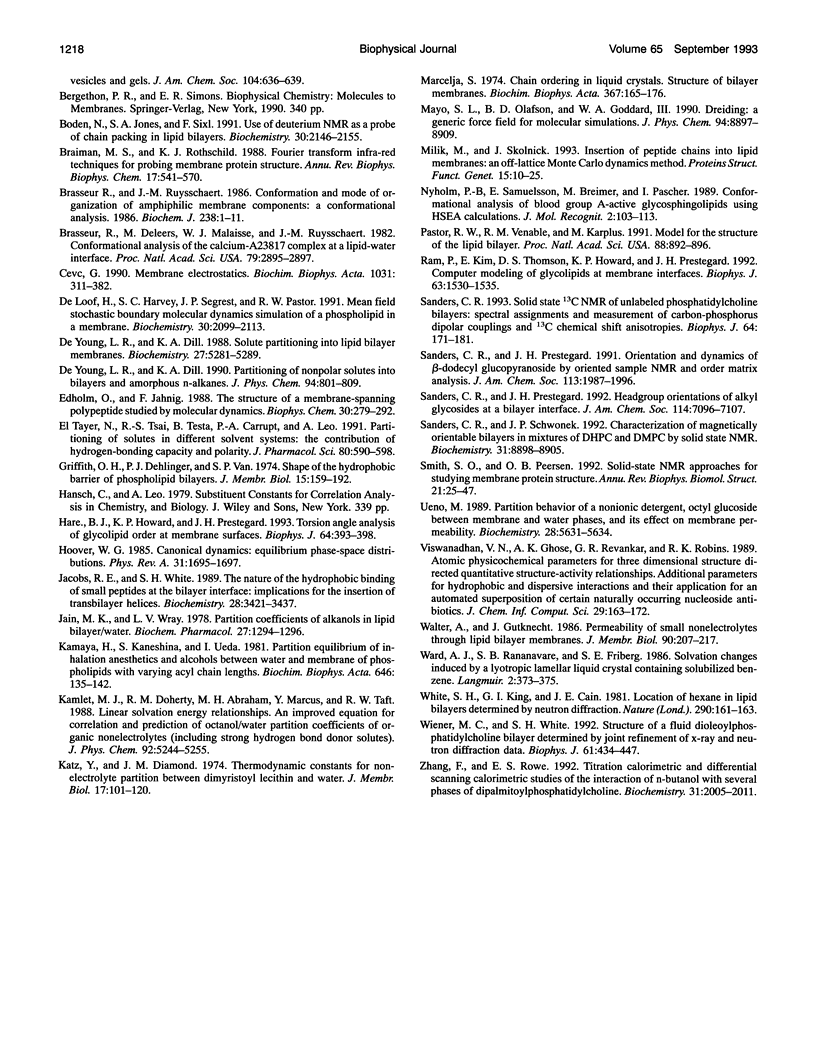
Selected References
These references are in PubMed. This may not be the complete list of references from this article.
- Boden N., Jones S. A., Sixl F. On the use of deuterium nuclear magnetic resonance as a probe of chain packing in lipid bilayers. Biochemistry. 1991 Feb 26;30(8):2146–2155. doi: 10.1021/bi00222a019. [DOI] [PubMed] [Google Scholar]
- Braiman M. S., Rothschild K. J. Fourier transform infrared techniques for probing membrane protein structure. Annu Rev Biophys Biophys Chem. 1988;17:541–570. doi: 10.1146/annurev.bb.17.060188.002545. [DOI] [PubMed] [Google Scholar]
- Brasseur R., Deleers M., Malaisse W. J., Ruysschaert J. M. Conformational analysis of the calcium--A23187 complex at a lipid--water interface. Proc Natl Acad Sci U S A. 1982 May;79(9):2895–2897. doi: 10.1073/pnas.79.9.2895. [DOI] [PMC free article] [PubMed] [Google Scholar]
- Brasseur R., Ruysschaert J. M. Conformation and mode of organization of amphiphilic membrane components: a conformational analysis. Biochem J. 1986 Aug 15;238(1):1–11. doi: 10.1042/bj2380001. [DOI] [PMC free article] [PubMed] [Google Scholar]
- Cevc G. Membrane electrostatics. Biochim Biophys Acta. 1990 Oct 8;1031(3):311–382. doi: 10.1016/0304-4157(90)90015-5. [DOI] [PubMed] [Google Scholar]
- De Loof H., Harvey S. C., Segrest J. P., Pastor R. W. Mean field stochastic boundary molecular dynamics simulation of a phospholipid in a membrane. Biochemistry. 1991 Feb 26;30(8):2099–2113. doi: 10.1021/bi00222a015. [DOI] [PubMed] [Google Scholar]
- De Young L. R., Dill K. A. Solute partitioning into lipid bilayer membranes. Biochemistry. 1988 Jul 12;27(14):5281–5289. doi: 10.1021/bi00414a050. [DOI] [PubMed] [Google Scholar]
- Edholm O., Jähnig F. The structure of a membrane-spanning polypeptide studied by molecular dynamics. Biophys Chem. 1988 Jul 15;30(3):279–292. doi: 10.1016/0301-4622(88)85023-3. [DOI] [PubMed] [Google Scholar]
- Griffith O. H., Dehlinger P. J., Van S. P. Shape of the hydrophobic barrier of phospholipid bilayers (evidence for water penetration in biological membranes). J Membr Biol. 1974;15(2):159–192. doi: 10.1007/BF01870086. [DOI] [PubMed] [Google Scholar]
- Hare B. J., Howard K. P., Prestegard J. H. Torsion angle analysis of glycolipid order at membrane surfaces. Biophys J. 1993 Feb;64(2):392–398. doi: 10.1016/S0006-3495(93)81380-8. [DOI] [PMC free article] [PubMed] [Google Scholar]
- Hoover WG. Canonical dynamics: Equilibrium phase-space distributions. Phys Rev A Gen Phys. 1985 Mar;31(3):1695–1697. doi: 10.1103/physreva.31.1695. [DOI] [PubMed] [Google Scholar]
- Jacobs R. E., White S. H. The nature of the hydrophobic binding of small peptides at the bilayer interface: implications for the insertion of transbilayer helices. Biochemistry. 1989 Apr 18;28(8):3421–3437. doi: 10.1021/bi00434a042. [DOI] [PubMed] [Google Scholar]
- Jain M. K., Wray L. V., Jr Partition coefficients of alkanols in lipid bilayer/water. Biochem Pharmacol. 1978;27(8):1294–1295. doi: 10.1016/0006-2952(78)90469-0. [DOI] [PubMed] [Google Scholar]
- Kamaya H., Kaneshina S., Ueda I. Partition equilibrium of inhalation anesthetics and alcohols between water and membranes of phospholipids with varying acyl chain-lengths. Biochim Biophys Acta. 1981 Aug 6;646(1):135–142. doi: 10.1016/0005-2736(81)90280-7. [DOI] [PubMed] [Google Scholar]
- Katz Y., Diamond J. M. Thermodynamic constants for nonelectrolyte partition between dimyristoyl lecithin and water. J Membr Biol. 1974;17(2):101–120. doi: 10.1007/BF01870175. [DOI] [PubMed] [Google Scholar]
- Marcelja S. Chain ordering in liquid crystals. II. Structure of bilayer membranes. Biochim Biophys Acta. 1974 Oct 29;367(2):165–176. doi: 10.1016/0005-2736(74)90040-6. [DOI] [PubMed] [Google Scholar]
- Milik M., Skolnick J. Insertion of peptide chains into lipid membranes: an off-lattice Monte Carlo dynamics model. Proteins. 1993 Jan;15(1):10–25. doi: 10.1002/prot.340150104. [DOI] [PubMed] [Google Scholar]
- Nyholm P. G., Samuelsson B. E., Breimer M., Pascher I. Conformational analysis of blood group A-active glycosphingolipids using HSEA-calculations. The possible significance of the core oligosaccharide chain for the presentation and recognition of the A-determinant. J Mol Recognit. 1989 Nov;2(3):103–113. doi: 10.1002/jmr.300020302. [DOI] [PubMed] [Google Scholar]
- Pastor R. W., Venable R. M., Karplus M. Model for the structure of the lipid bilayer. Proc Natl Acad Sci U S A. 1991 Feb 1;88(3):892–896. doi: 10.1073/pnas.88.3.892. [DOI] [PMC free article] [PubMed] [Google Scholar]
- Ram P., Kim E., Thomson D. S., Howard K. P., Prestegard J. H. Computer modelling of glycolipids at membrane surfaces. Biophys J. 1992 Dec;63(6):1530–1535. doi: 10.1016/S0006-3495(92)81729-0. [DOI] [PMC free article] [PubMed] [Google Scholar]
- Sanders C. R., 2nd, Schwonek J. P. Characterization of magnetically orientable bilayers in mixtures of dihexanoylphosphatidylcholine and dimyristoylphosphatidylcholine by solid-state NMR. Biochemistry. 1992 Sep 22;31(37):8898–8905. doi: 10.1021/bi00152a029. [DOI] [PubMed] [Google Scholar]
- Sanders C. R., 2nd Solid state 13C NMR of unlabeled phosphatidylcholine bilayers: spectral assignments and measurement of carbon-phosphorus dipolar couplings and 13C chemical shift anisotropies. Biophys J. 1993 Jan;64(1):171–181. doi: 10.1016/S0006-3495(93)81352-3. [DOI] [PMC free article] [PubMed] [Google Scholar]
- Smith S. O., Peersen O. B. Solid-state NMR approaches for studying membrane protein structure. Annu Rev Biophys Biomol Struct. 1992;21:25–47. doi: 10.1146/annurev.bb.21.060192.000325. [DOI] [PubMed] [Google Scholar]
- Ueno M. Partition behavior of a nonionic detergent, octyl glucoside, between membrane and water phases, and its effect on membrane permeability. Biochemistry. 1989 Jun 27;28(13):5631–5634. doi: 10.1021/bi00439a044. [DOI] [PubMed] [Google Scholar]
- Walter A., Gutknecht J. Permeability of small nonelectrolytes through lipid bilayer membranes. J Membr Biol. 1986;90(3):207–217. doi: 10.1007/BF01870127. [DOI] [PubMed] [Google Scholar]
- Wiener M. C., White S. H. Structure of a fluid dioleoylphosphatidylcholine bilayer determined by joint refinement of x-ray and neutron diffraction data. III. Complete structure. Biophys J. 1992 Feb;61(2):434–447. doi: 10.1016/S0006-3495(92)81849-0. [DOI] [PMC free article] [PubMed] [Google Scholar]
- Zhang F., Rowe E. S. Titration calorimetric and differential scanning calorimetric studies of the interactions of n-butanol with several phases of dipalmitoylphosphatidylcholine. Biochemistry. 1992 Feb 25;31(7):2005–2011. doi: 10.1021/bi00122a016. [DOI] [PubMed] [Google Scholar]
- el Tayar N., Tsai R. S., Testa B., Carrupt P. A., Leo A. Partitioning of solutes in different solvent systems: the contribution of hydrogen-bonding capacity and polarity. J Pharm Sci. 1991 Jun;80(6):590–598. doi: 10.1002/jps.2600800619. [DOI] [PubMed] [Google Scholar]