Abstract
Highly ordered two-dimensional (2-D) crystals of Escherichia coli-expressed bacteriorhodopsin analog (e-bR) and its D96N variant (e-D96N) reconstituted in Halobacterium halobium lipids have been obtained by starting with the opsin protein purified in the denaturing detergent sodium dodecyl sulfate. These crystals embedded in glucose show electron diffraction in projection to better than 3.0 A at room temperature. This is the first instance that expressed bR or a variant has been crystallized in 2-D arrays showing such high order. The crystal lattice is homologous to that in wild-type bR (w-bR) in purple membranes (PM) and permit high resolution analyses of the structure of the functionally impaired D96N variant. The e-bR crystal is isomorphous to that in PM with an overall averaged fractional change of 12.7% (26-3.6-A resolution) in the projection structure factors. The projection difference Fourier map e-bR-PM at 3.6-A resolution indicates small conformational changes equivalent to movement of approximately < 7 C-atoms distributed within and in the neighborhood of the protein envelope. This result shows that relative to w-bR there are no global structural rearrangements in e-bR at this 3.6 A resolution level. The e-D96N crystal is isomorphous to the e-bR crystal with a smaller (9.2%) overall averaged fractional change in the structure factors. The significant structural differences between e-D96N and e-bR are concentrated at high resolution (5-3.6 A); however, these changes are small as quantified from the 3.6 A resolution e-D96N-e-bR Fourier difference map. The difference map showed no statistically significant peaks or valleys within 5 A in projection from the site of D96 substitution on helix C. Elsewhere within the protein envelope the integrated measure of peaks or valleys was < approximately 3 C-atom equivalents. Thus, our results show that for the isosteric substitution of Asp96 by Asn, the molecular conformation of bR in its ground state is essentially unaltered. Therefore, the known effect of D96N on the slowed M412 decay is not due to ground-state structural perturbations.
Full text
PDF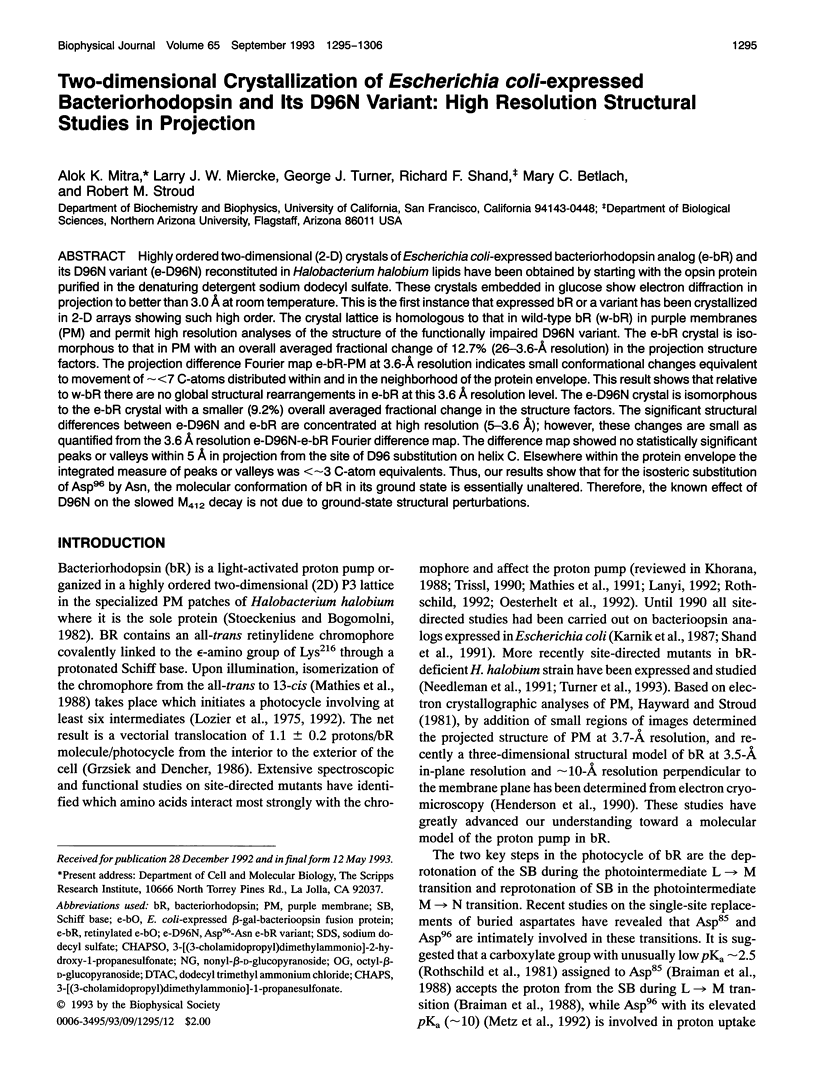
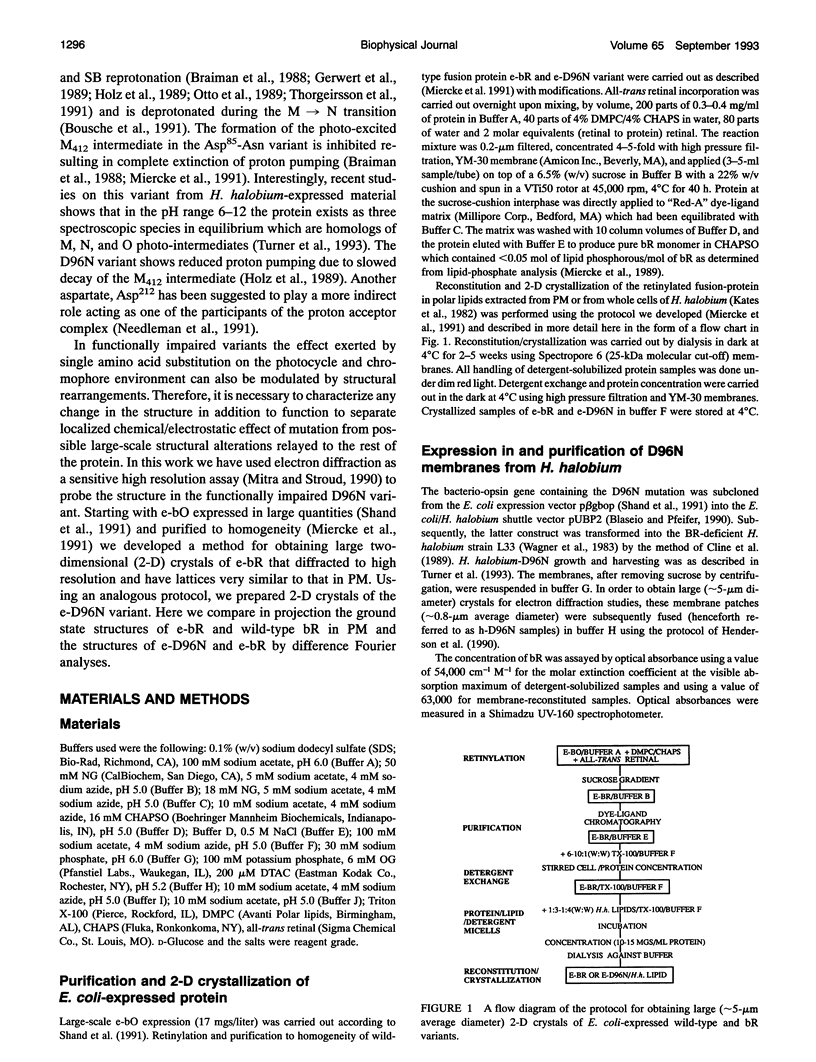
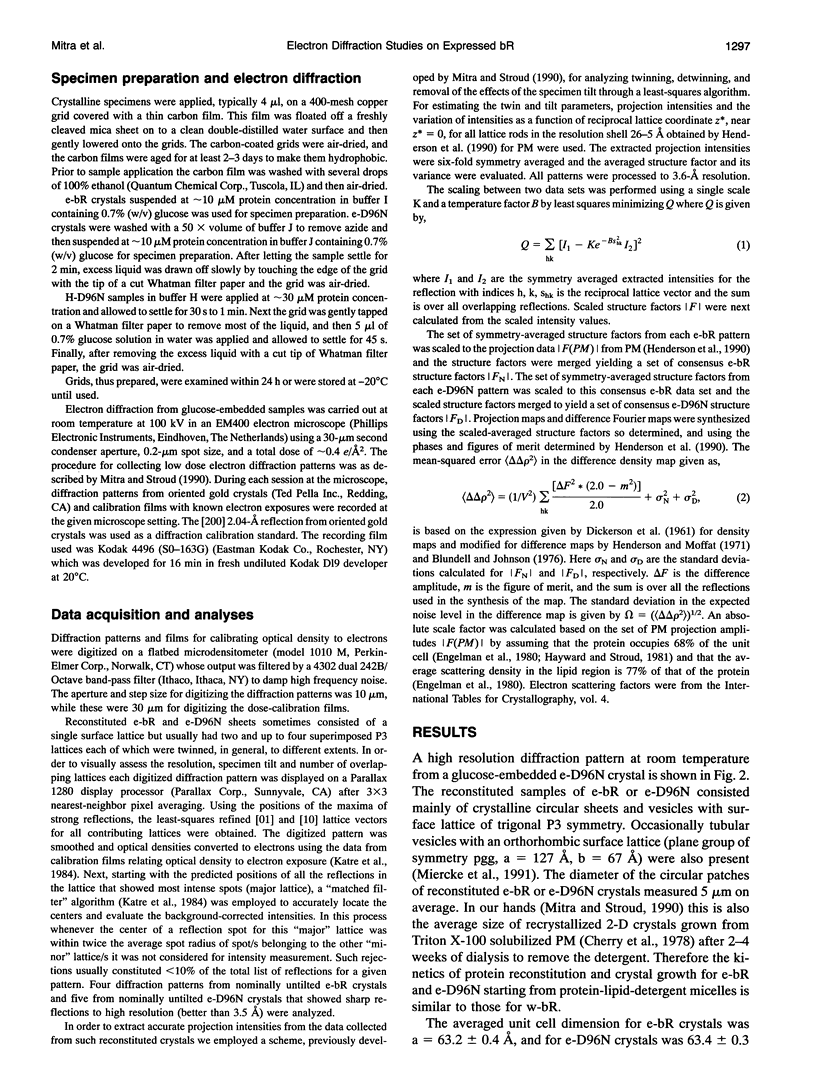
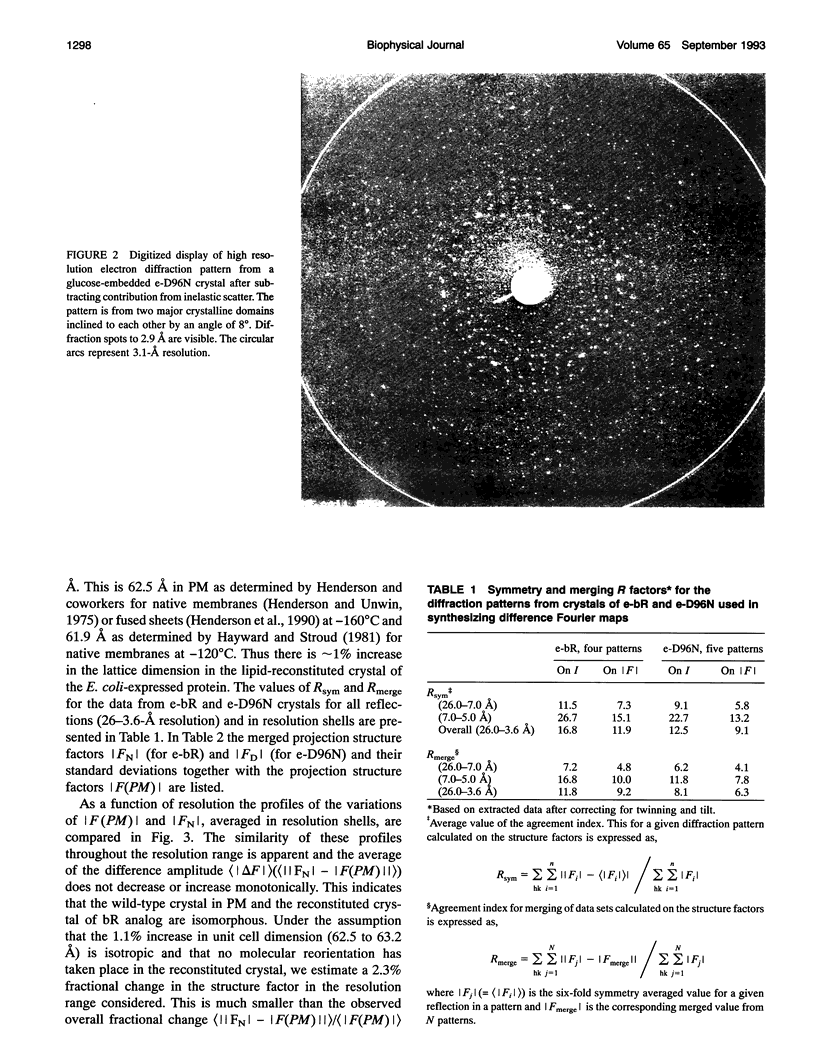
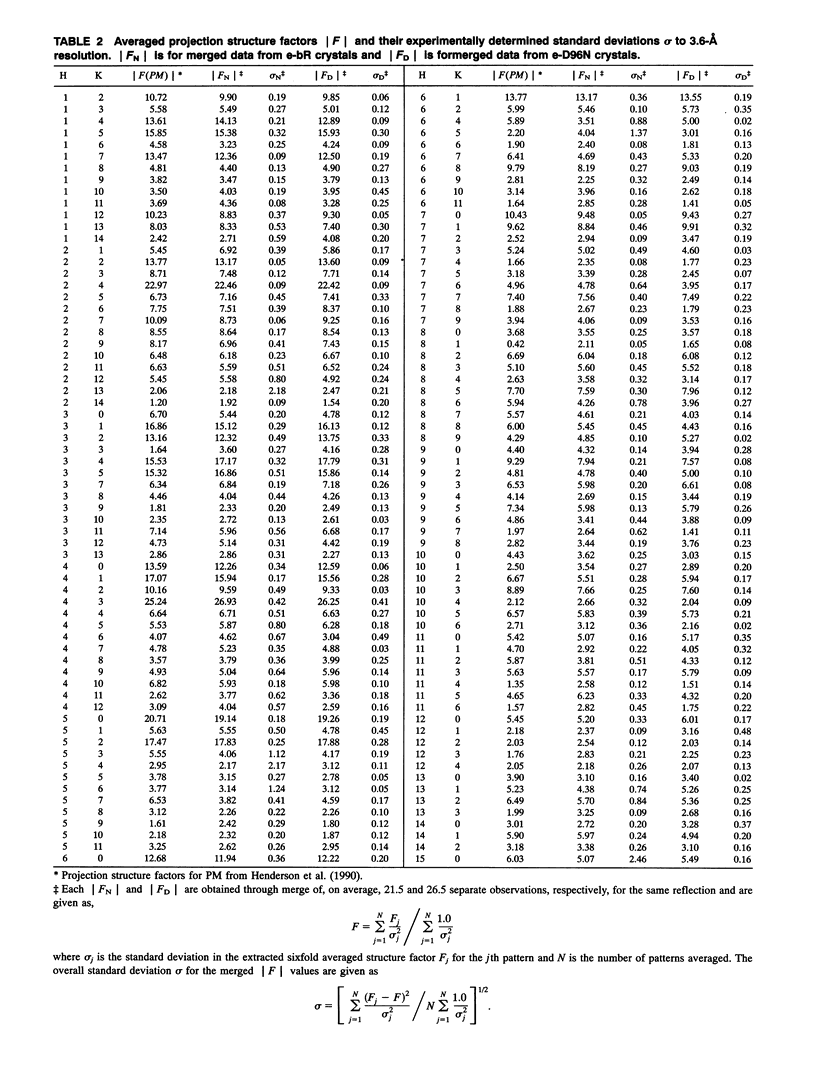
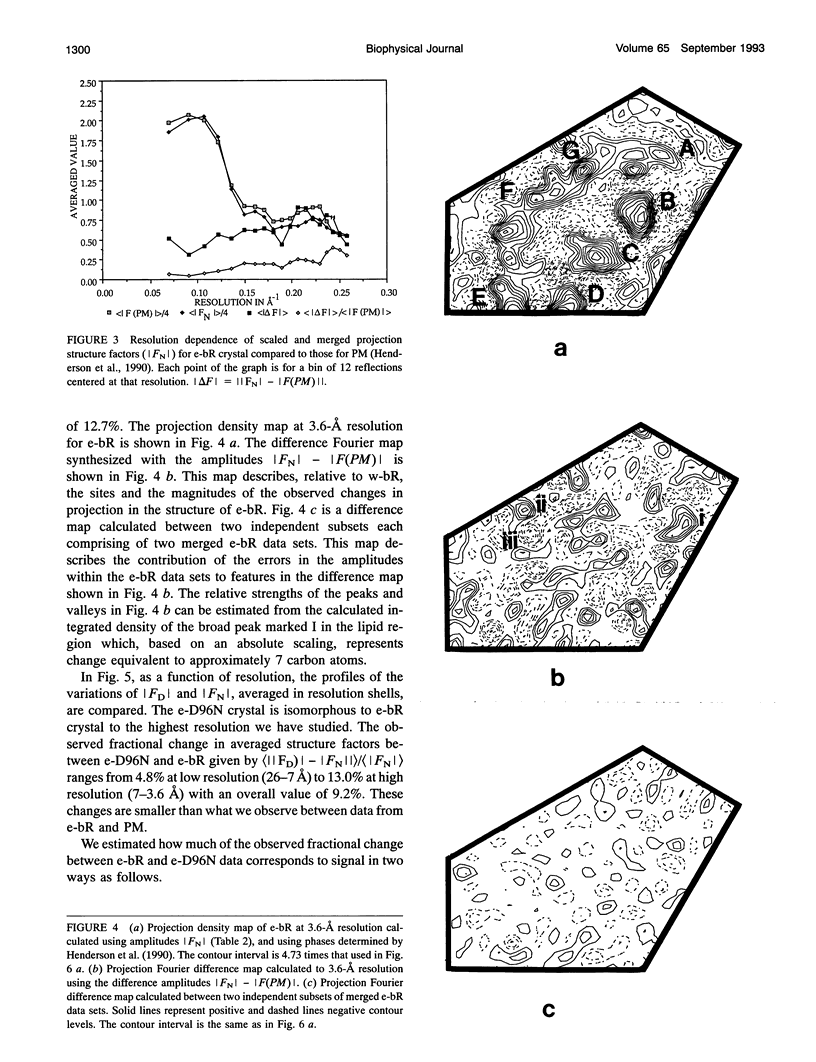
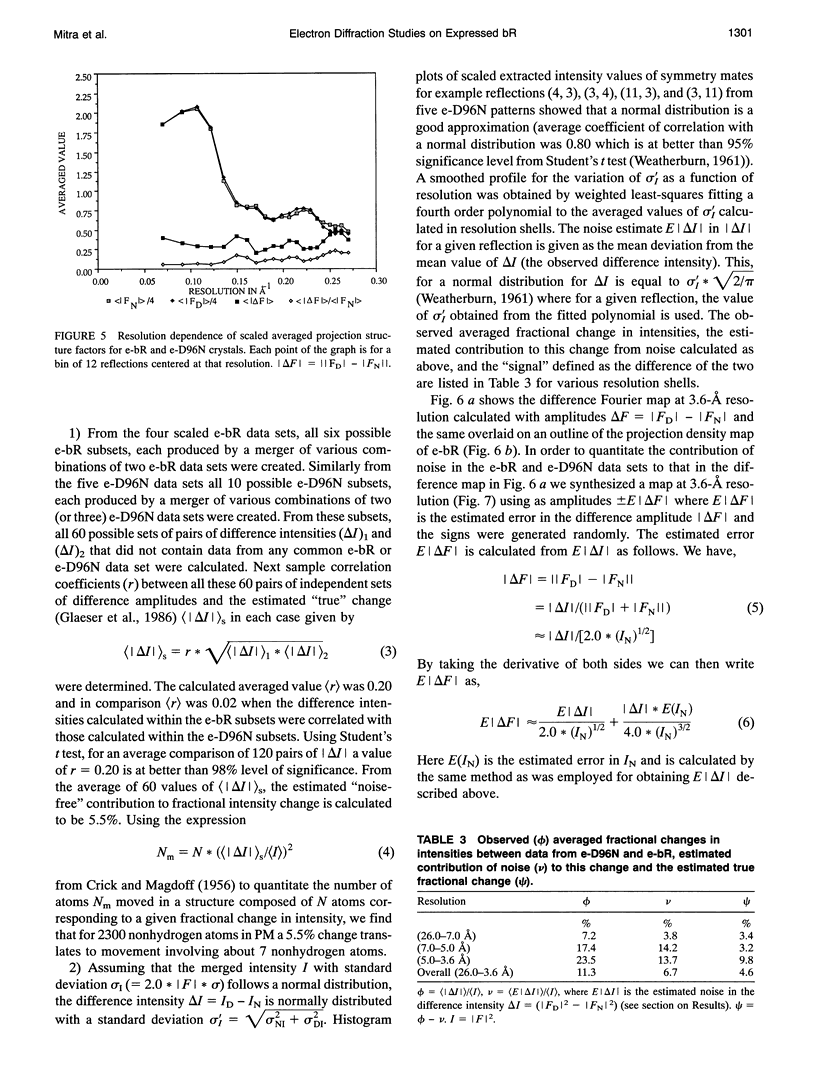
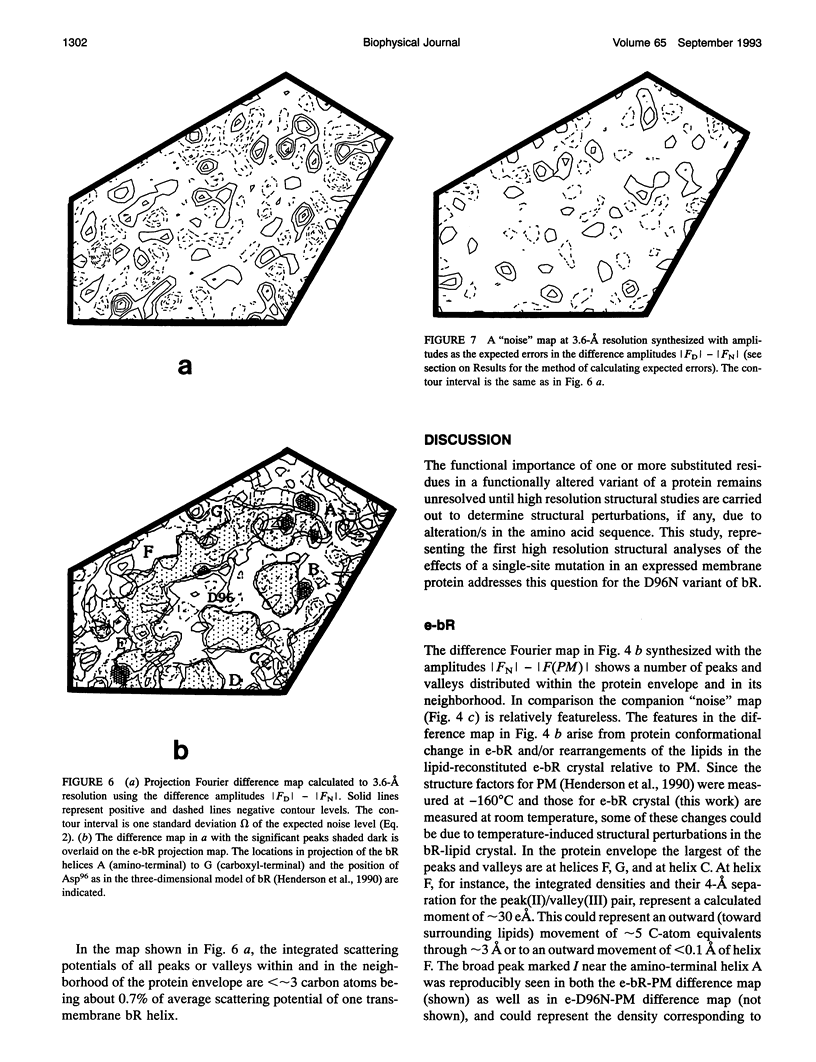
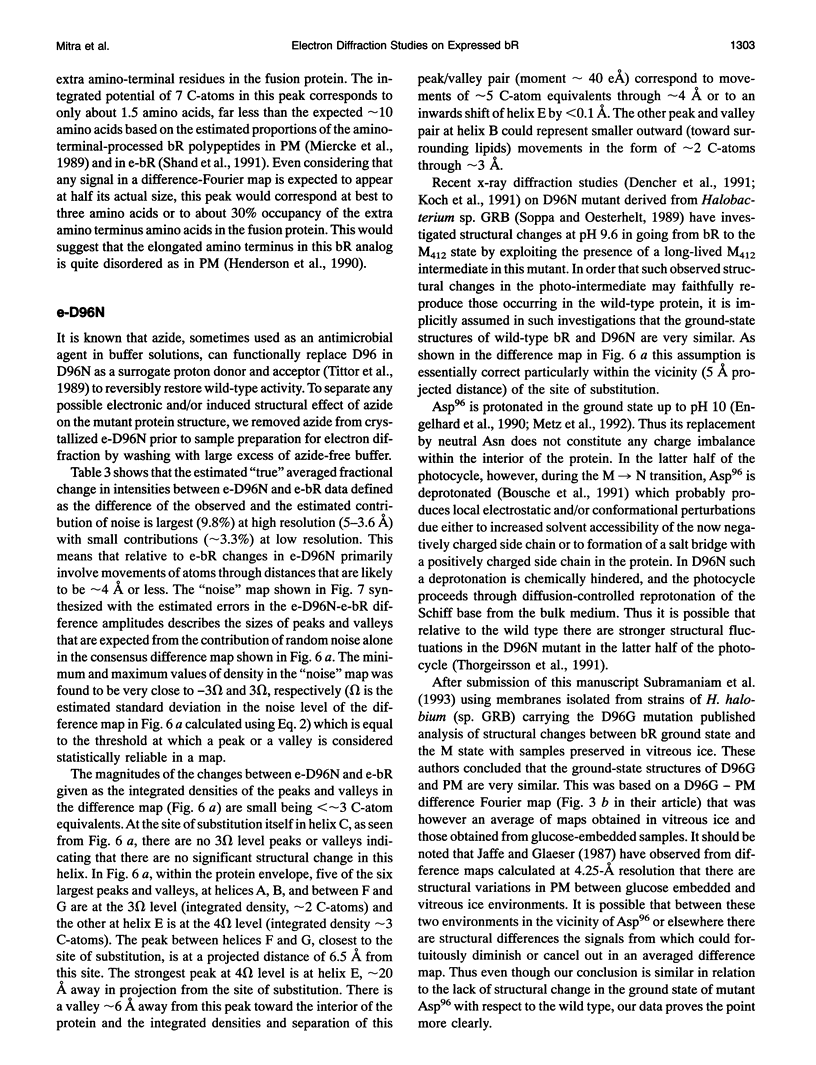
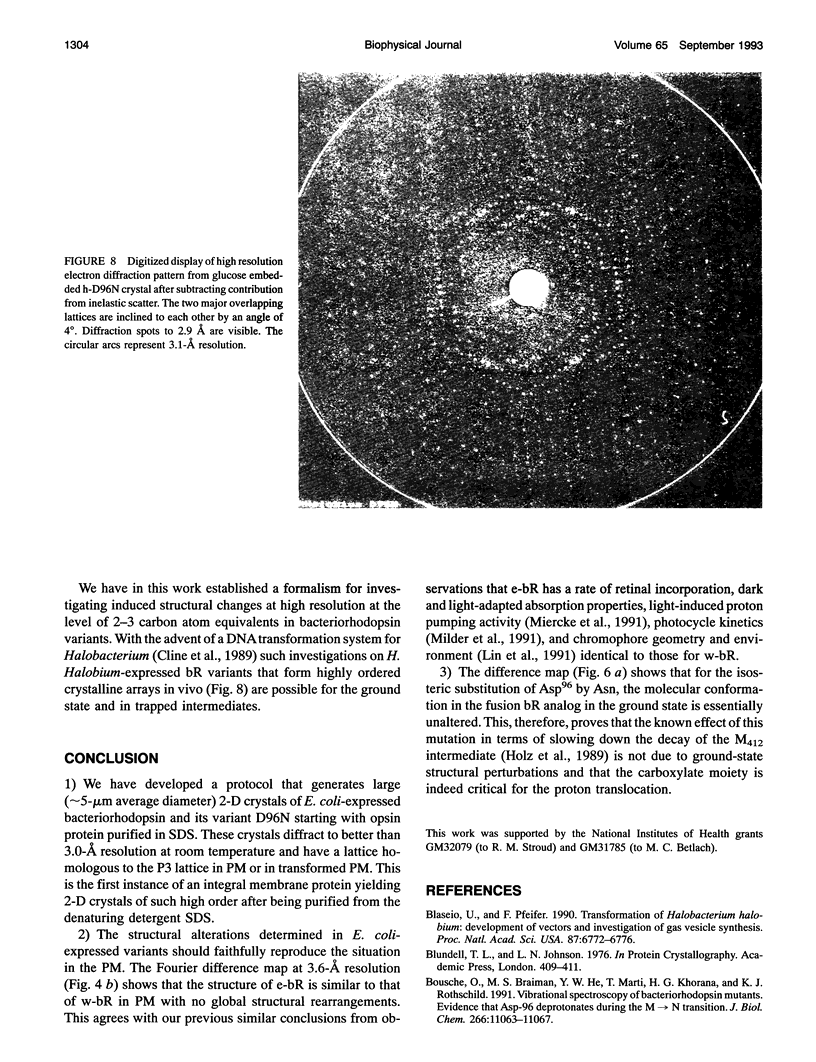
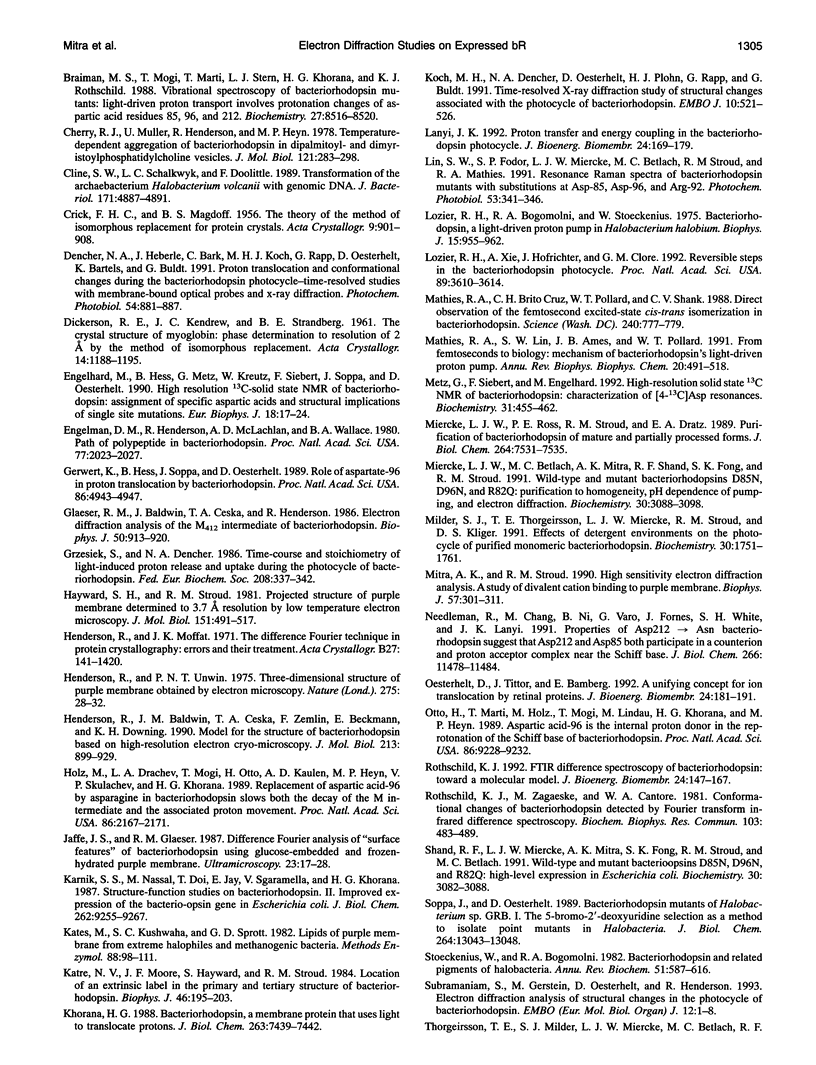
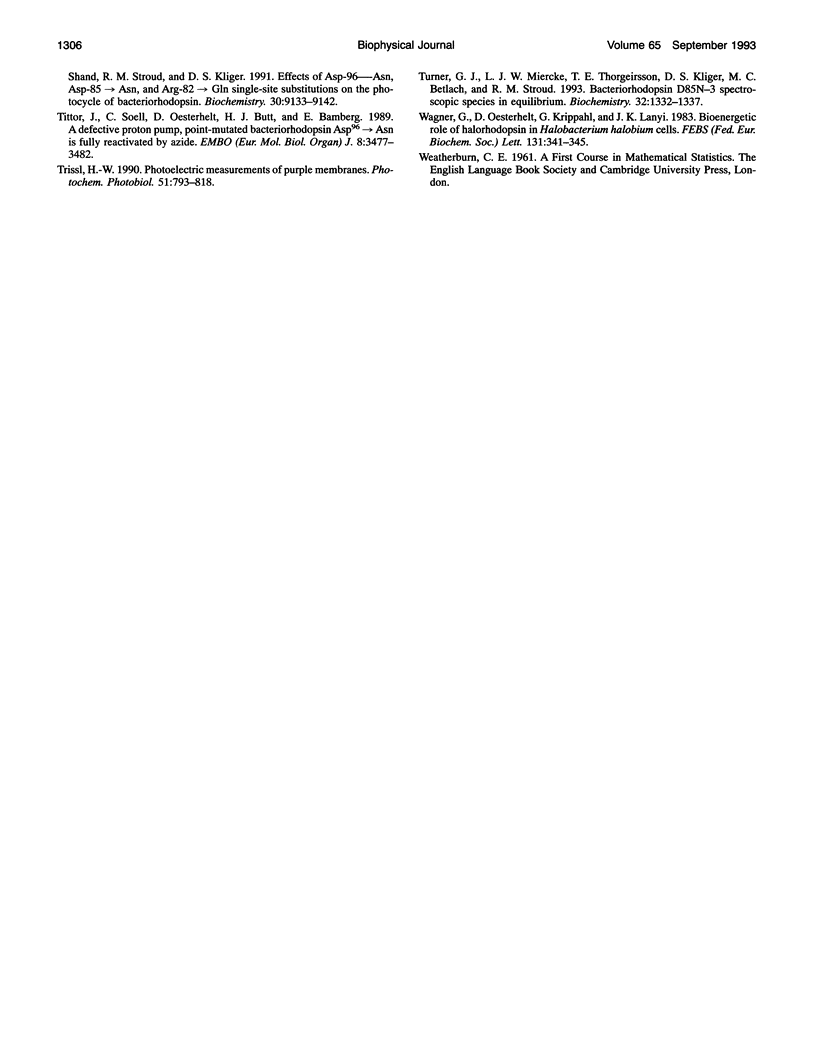
Images in this article
Selected References
These references are in PubMed. This may not be the complete list of references from this article.
- Blaseio U., Pfeifer F. Transformation of Halobacterium halobium: development of vectors and investigation of gas vesicle synthesis. Proc Natl Acad Sci U S A. 1990 Sep;87(17):6772–6776. doi: 10.1073/pnas.87.17.6772. [DOI] [PMC free article] [PubMed] [Google Scholar]
- Bousché O., Braiman M., He Y. W., Marti T., Khorana H. G., Rothschild K. J. Vibrational spectroscopy of bacteriorhodopsin mutants. Evidence that ASP-96 deprotonates during the M----N transition. J Biol Chem. 1991 Jun 15;266(17):11063–11067. [PubMed] [Google Scholar]
- Braiman M. S., Mogi T., Marti T., Stern L. J., Khorana H. G., Rothschild K. J. Vibrational spectroscopy of bacteriorhodopsin mutants: light-driven proton transport involves protonation changes of aspartic acid residues 85, 96, and 212. Biochemistry. 1988 Nov 15;27(23):8516–8520. doi: 10.1021/bi00423a002. [DOI] [PubMed] [Google Scholar]
- Cherry R. J., Müller U. Temperature-dependent aggregation of bacteriorhodopsin in dipalmitoyl- and dimyristoylphosphatidylcholine vesicles. J Mol Biol. 1978 May 15;121(2):283–298. doi: 10.1016/s0022-2836(78)80010-2. [DOI] [PubMed] [Google Scholar]
- Engelhard M., Hess B., Metz G., Kreutz W., Siebert F., Soppa J., Oesterhelt D. High resolution 13C-solid state NMR of bacteriorhodopsin: assignment of specific aspartic acids and structural implications of single site mutations. Eur Biophys J. 1990;18(1):17–24. doi: 10.1007/BF00185416. [DOI] [PubMed] [Google Scholar]
- Engelman D. M., Henderson R., McLachlan A. D., Wallace B. A. Path of the polypeptide in bacteriorhodopsin. Proc Natl Acad Sci U S A. 1980 Apr;77(4):2023–2027. doi: 10.1073/pnas.77.4.2023. [DOI] [PMC free article] [PubMed] [Google Scholar]
- Gerwert K., Hess B., Soppa J., Oesterhelt D. Role of aspartate-96 in proton translocation by bacteriorhodopsin. Proc Natl Acad Sci U S A. 1989 Jul;86(13):4943–4947. doi: 10.1073/pnas.86.13.4943. [DOI] [PMC free article] [PubMed] [Google Scholar]
- Glaeser R. M., Baldwin J., Ceska T. A., Henderson R. Electron diffraction analysis of the M412 intermediate of bacteriorhodopsin. Biophys J. 1986 Nov;50(5):913–920. doi: 10.1016/S0006-3495(86)83532-9. [DOI] [PMC free article] [PubMed] [Google Scholar]
- Hayward S. B., Stroud R. M. Projected structure of purple membrane determined to 3.7 A resolution by low temperature electron microscopy. J Mol Biol. 1981 Sep 25;151(3):491–517. doi: 10.1016/0022-2836(81)90007-3. [DOI] [PubMed] [Google Scholar]
- Henderson R., Baldwin J. M., Ceska T. A., Zemlin F., Beckmann E., Downing K. H. Model for the structure of bacteriorhodopsin based on high-resolution electron cryo-microscopy. J Mol Biol. 1990 Jun 20;213(4):899–929. doi: 10.1016/S0022-2836(05)80271-2. [DOI] [PubMed] [Google Scholar]
- Henderson R., Unwin P. N. Three-dimensional model of purple membrane obtained by electron microscopy. Nature. 1975 Sep 4;257(5521):28–32. doi: 10.1038/257028a0. [DOI] [PubMed] [Google Scholar]
- Holz M., Drachev L. A., Mogi T., Otto H., Kaulen A. D., Heyn M. P., Skulachev V. P., Khorana H. G. Replacement of aspartic acid-96 by asparagine in bacteriorhodopsin slows both the decay of the M intermediate and the associated proton movement. Proc Natl Acad Sci U S A. 1989 Apr;86(7):2167–2171. doi: 10.1073/pnas.86.7.2167. [DOI] [PMC free article] [PubMed] [Google Scholar]
- Jaffe J. S., Glaeser R. M. Difference Fourier analysis of "surface features" of bacteriorhodopsin using glucose-embedded and frozen-hydrated purple membrane. Ultramicroscopy. 1987;23(1):17–28. doi: 10.1016/0304-3991(87)90223-3. [DOI] [PubMed] [Google Scholar]
- Karnik S. S., Nassal M., Doi T., Jay E., Sgaramella V., Khorana H. G. Structure-function studies on bacteriorhodopsin. II. Improved expression of the bacterio-opsin gene in Escherichia coli. J Biol Chem. 1987 Jul 5;262(19):9255–9263. [PubMed] [Google Scholar]
- Katre N. V., Finer-Moore J., Stroud R. M., Hayward S. B. Location of an extrinsic label in the primary and tertiary structure of bacteriorhodopsin. Biophys J. 1984 Aug;46(2):195–203. doi: 10.1016/S0006-3495(84)84013-8. [DOI] [PMC free article] [PubMed] [Google Scholar]
- Khorana H. G. Bacteriorhodopsin, a membrane protein that uses light to translocate protons. J Biol Chem. 1988 Jun 5;263(16):7439–7442. [PubMed] [Google Scholar]
- Koch M. H., Dencher N. A., Oesterhelt D., Plöhn H. J., Rapp G., Büldt G. Time-resolved X-ray diffraction study of structural changes associated with the photocycle of bacteriorhodopsin. EMBO J. 1991 Mar;10(3):521–526. doi: 10.1002/j.1460-2075.1991.tb07978.x. [DOI] [PMC free article] [PubMed] [Google Scholar]
- Lanyi J. K. Proton transfer and energy coupling in the bacteriorhodopsin photocycle. J Bioenerg Biomembr. 1992 Apr;24(2):169–179. doi: 10.1007/BF00762675. [DOI] [PubMed] [Google Scholar]
- Lin S. W., Fodor S. P., Miercke L. J., Shand R. F., Betlach M. C., Stroud R. M., Mathies R. A. Resonance Raman spectra of bacteriorhodopsin mutants with substitutions at Asp-85, Asp-96, and Arg-82. Photochem Photobiol. 1991 Mar;53(3):341–346. doi: 10.1111/j.1751-1097.1991.tb03638.x. [DOI] [PubMed] [Google Scholar]
- Lozier R. H., Bogomolni R. A., Stoeckenius W. Bacteriorhodopsin: a light-driven proton pump in Halobacterium Halobium. Biophys J. 1975 Sep;15(9):955–962. doi: 10.1016/S0006-3495(75)85875-9. [DOI] [PMC free article] [PubMed] [Google Scholar]
- Lozier R. H., Xie A., Hofrichter J., Clore G. M. Reversible steps in the bacteriorhodopsin photocycle. Proc Natl Acad Sci U S A. 1992 Apr 15;89(8):3610–3614. doi: 10.1073/pnas.89.8.3610. [DOI] [PMC free article] [PubMed] [Google Scholar]
- Mathies R. A., Brito Cruz C. H., Pollard W. T., Shank C. V. Direct observation of the femtosecond excited-state cis-trans isomerization in bacteriorhodopsin. Science. 1988 May 6;240(4853):777–779. doi: 10.1126/science.3363359. [DOI] [PubMed] [Google Scholar]
- Mathies R. A., Lin S. W., Ames J. B., Pollard W. T. From femtoseconds to biology: mechanism of bacteriorhodopsin's light-driven proton pump. Annu Rev Biophys Biophys Chem. 1991;20:491–518. doi: 10.1146/annurev.bb.20.060191.002423. [DOI] [PubMed] [Google Scholar]
- Metz G., Siebert F., Engelhard M. High-resolution solid state 13C NMR of bacteriorhodopsin: characterization of [4-13C]Asp resonances. Biochemistry. 1992 Jan 21;31(2):455–462. doi: 10.1021/bi00117a022. [DOI] [PubMed] [Google Scholar]
- Miercke L. J., Betlach M. C., Mitra A. K., Shand R. F., Fong S. K., Stroud R. M. Wild-type and mutant bacteriorhodopsins D85N, D96N, and R82Q: purification to homogeneity, pH dependence of pumping, and electron diffraction. Biochemistry. 1991 Mar 26;30(12):3088–3098. doi: 10.1021/bi00226a016. [DOI] [PubMed] [Google Scholar]
- Miercke L. J., Ross P. E., Stroud R. M., Dratz E. A. Purification of bacteriorhodopsin and characterization of mature and partially processed forms. J Biol Chem. 1989 May 5;264(13):7531–7535. [PubMed] [Google Scholar]
- Milder S. J., Thorgeirsson T. E., Miercke L. J., Stroud R. M., Kliger D. S. Effects of detergent environments on the photocycle of purified monomeric bacteriorhodopsin. Biochemistry. 1991 Feb 19;30(7):1751–1761. doi: 10.1021/bi00221a004. [DOI] [PubMed] [Google Scholar]
- Mitra A. K., Stroud R. M. High sensitivity electron diffraction analysis. A study of divalent cation binding to purple membrane. Biophys J. 1990 Feb;57(2):301–311. doi: 10.1016/S0006-3495(90)82532-7. [DOI] [PMC free article] [PubMed] [Google Scholar]
- Needleman R., Chang M., Ni B., Váró G., Fornés J., White S. H., Lanyi J. K. Properties of Asp212----Asn bacteriorhodopsin suggest that Asp212 and Asp85 both participate in a counterion and proton acceptor complex near the Schiff base. J Biol Chem. 1991 Jun 25;266(18):11478–11484. [PubMed] [Google Scholar]
- Oesterhelt D., Tittor J., Bamberg E. A unifying concept for ion translocation by retinal proteins. J Bioenerg Biomembr. 1992 Apr;24(2):181–191. doi: 10.1007/BF00762676. [DOI] [PubMed] [Google Scholar]
- Otto H., Marti T., Holz M., Mogi T., Lindau M., Khorana H. G., Heyn M. P. Aspartic acid-96 is the internal proton donor in the reprotonation of the Schiff base of bacteriorhodopsin. Proc Natl Acad Sci U S A. 1989 Dec;86(23):9228–9232. doi: 10.1073/pnas.86.23.9228. [DOI] [PMC free article] [PubMed] [Google Scholar]
- Rothschild K. J. FTIR difference spectroscopy of bacteriorhodopsin: toward a molecular model. J Bioenerg Biomembr. 1992 Apr;24(2):147–167. doi: 10.1007/BF00762674. [DOI] [PubMed] [Google Scholar]
- Rothschild K. J., Zagaeski M., Cantore W. A. Conformational changes of bacteriorhodopsin detected by Fourier transform infrared difference spectroscopy. Biochem Biophys Res Commun. 1981 Nov 30;103(2):483–489. doi: 10.1016/0006-291x(81)90478-2. [DOI] [PubMed] [Google Scholar]
- Shand R. F., Miercke L. J., Mitra A. K., Fong S. K., Stroud R. M., Betlach M. C. Wild-type and mutant bacterioopsins D85N, D96N, and R82Q: high-level expression in Escherichia coli. Biochemistry. 1991 Mar 26;30(12):3082–3088. doi: 10.1021/bi00226a015. [DOI] [PubMed] [Google Scholar]
- Soppa J., Oesterhelt D. Bacteriorhodopsin mutants of Halobacterium sp. GRB. I. The 5-bromo-2'-deoxyuridine selection as a method to isolate point mutants in halobacteria. J Biol Chem. 1989 Aug 5;264(22):13043–13048. [PubMed] [Google Scholar]
- Stoeckenius W., Bogomolni R. A. Bacteriorhodopsin and related pigments of halobacteria. Annu Rev Biochem. 1982;51:587–616. doi: 10.1146/annurev.bi.51.070182.003103. [DOI] [PubMed] [Google Scholar]
- Thorgeirsson T. E., Milder S. J., Miercke L. J., Betlach M. C., Shand R. F., Stroud R. M., Kliger D. S. Effects of Asp-96----Asn, Asp-85----Asn, and Arg-82----Gln single-site substitutions on the photocycle of bacteriorhodopsin. Biochemistry. 1991 Sep 24;30(38):9133–9142. doi: 10.1021/bi00102a003. [DOI] [PubMed] [Google Scholar]
- Tittor J., Soell C., Oesterhelt D., Butt H. J., Bamberg E. A defective proton pump, point-mutated bacteriorhodopsin Asp96----Asn is fully reactivated by azide. EMBO J. 1989 Nov;8(11):3477–3482. doi: 10.1002/j.1460-2075.1989.tb08512.x. [DOI] [PMC free article] [PubMed] [Google Scholar]
- Trissl H. W. Photoelectric measurements of purple membranes. Photochem Photobiol. 1990 Jun;51(6):793–818. [PubMed] [Google Scholar]
- Turner G. J., Miercke L. J., Thorgeirsson T. E., Kliger D. S., Betlach M. C., Stroud R. M. Bacteriorhodopsin D85N: three spectroscopic species in equilibrium. Biochemistry. 1993 Feb 9;32(5):1332–1337. doi: 10.1021/bi00056a019. [DOI] [PubMed] [Google Scholar]