Abstract
The movements of E-cadherin, epidermal growth factor receptor, and transferrin receptor in the plasma membrane of a cultured mouse keratinocyte cell line were studied using both single particle tracking (SPT; nanovid microscopy) and fluorescence photobleaching recovery (FPR). In the SPT technique, the receptor molecules are labeled with 40 nm-phi colloidal gold particles, and their movements are followed by video-enhanced differential interference contrast microscopy at a temporal resolution of 33 ms and at a nanometer-level spatial precision. The trajectories of the receptor molecules obtained by SPT were analyzed by developing a method that is based on the plot of the mean-square displacement against time. Four characteristic types of motion were observed: (a) stationary mode, in which the microscopic diffusion coefficient is less than 4.6 x 10(-12) cm2/s; (b) simple Brownian diffusion mode; (c) directed diffusion mode, in which unidirectional movements are superimposed on random motion; and (d) confined diffusion mode, in which particles undergoing Brownian diffusion (microscopic diffusion coefficient between 4.6 x 10(-12) and 1 x 10(-9) cm2/s) are confined within a limited area, probably by the membrane-associated cytoskeleton network. Comparison of these data obtained by SPT with those obtained by FPR suggests that the plasma membrane is compartmentalized into many small domains 300-600 nm in diameter (0.04-0.24 microns2 in area), in which receptor molecules are confined in the time scale of 3-30 s, and that the long-range diffusion observed by FPR can occur by successive movements of the receptors to adjacent compartments. Calcium-induced differentiation decreases the sum of the percentages of molecules in the directed diffusion and the stationary modes outside of the cell-cell contact regions on the cell surface (which is proposed to be the percentage of E-cadherin bound to the cytoskeleton/membrane-skeleton), from approximately 60% to 8% (low- and high-calcium mediums, respectively).
Full text
PDF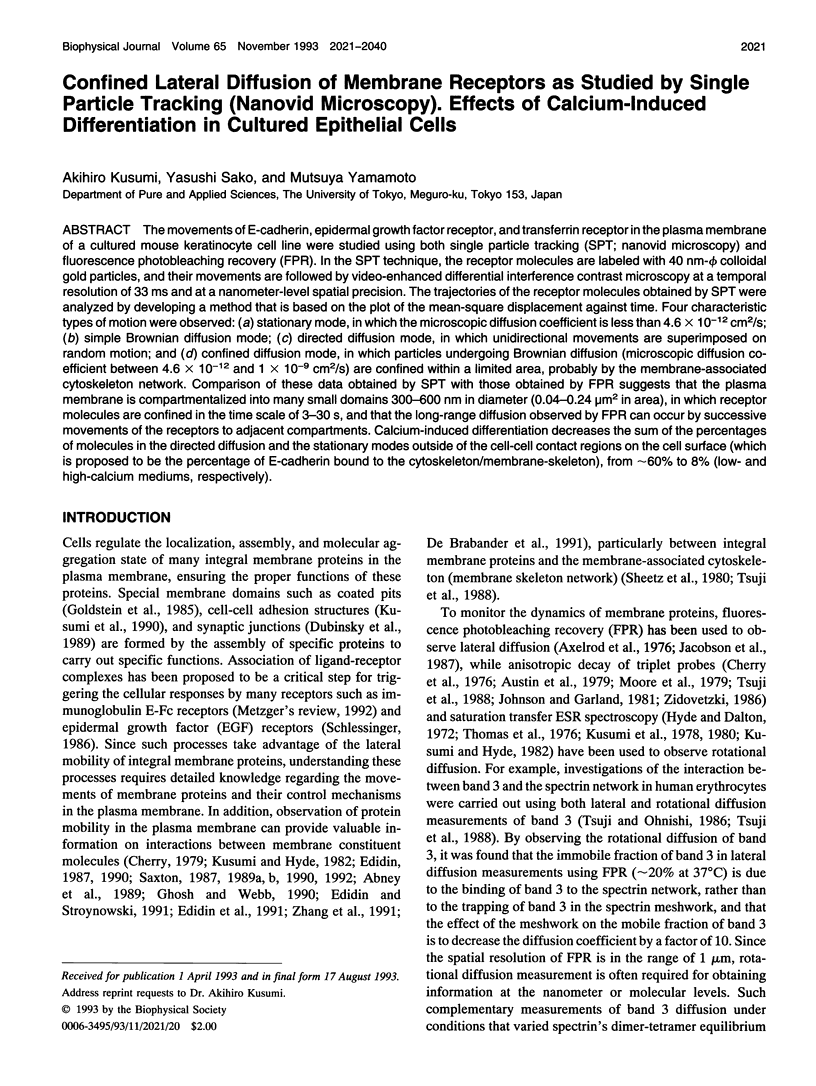
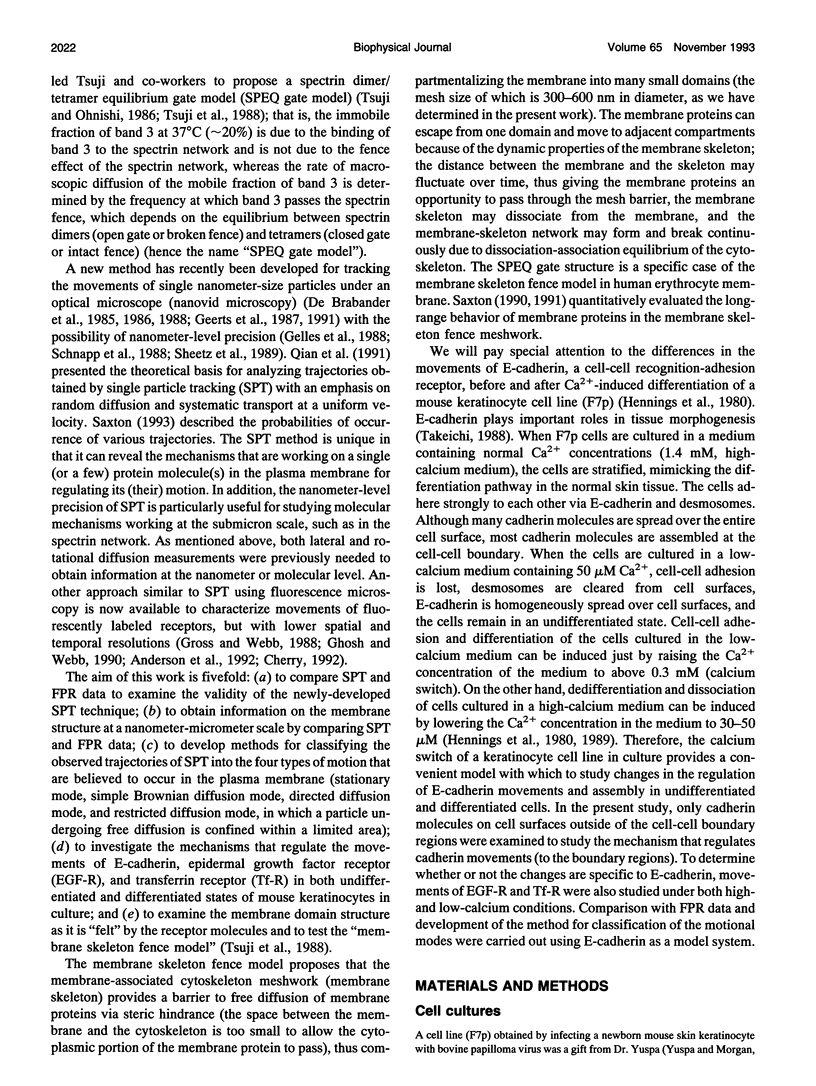
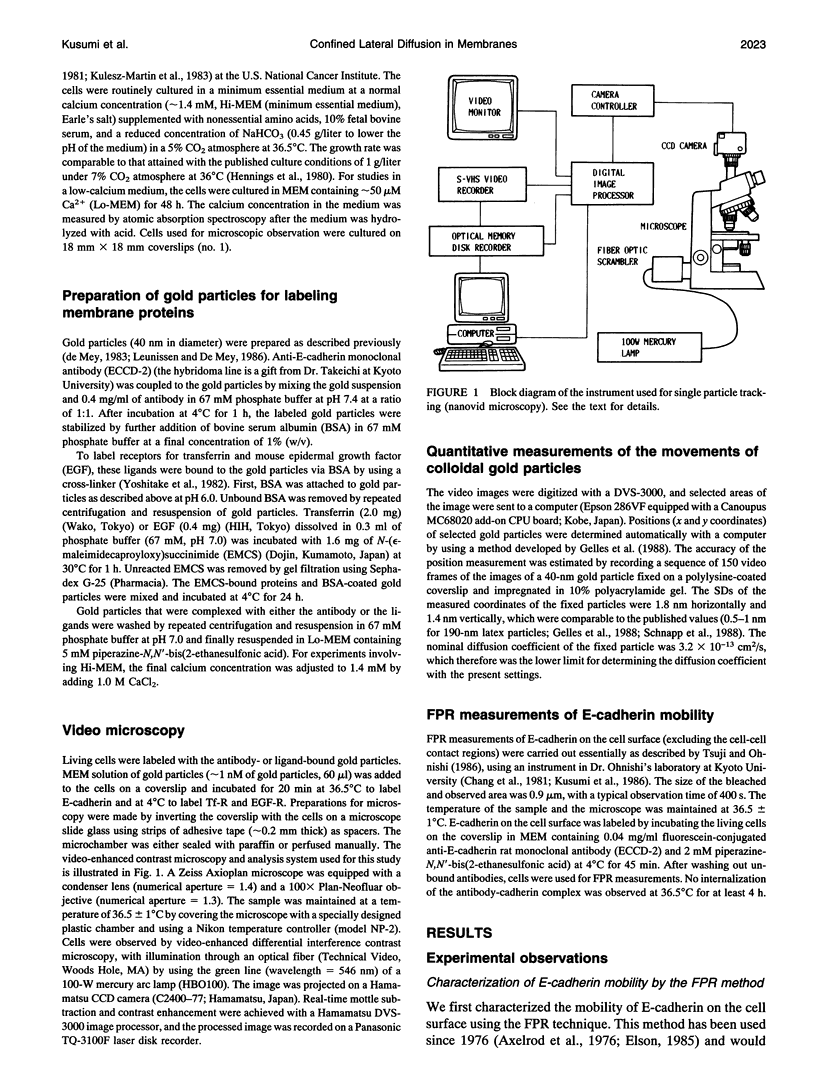
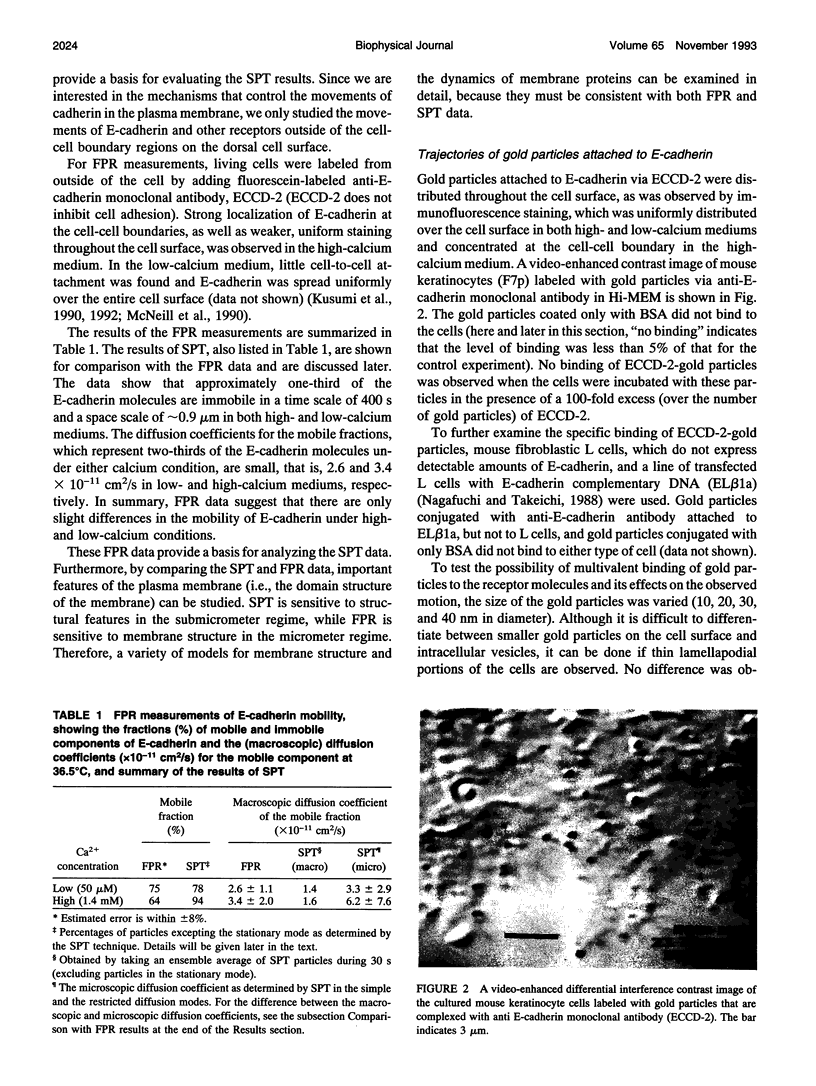
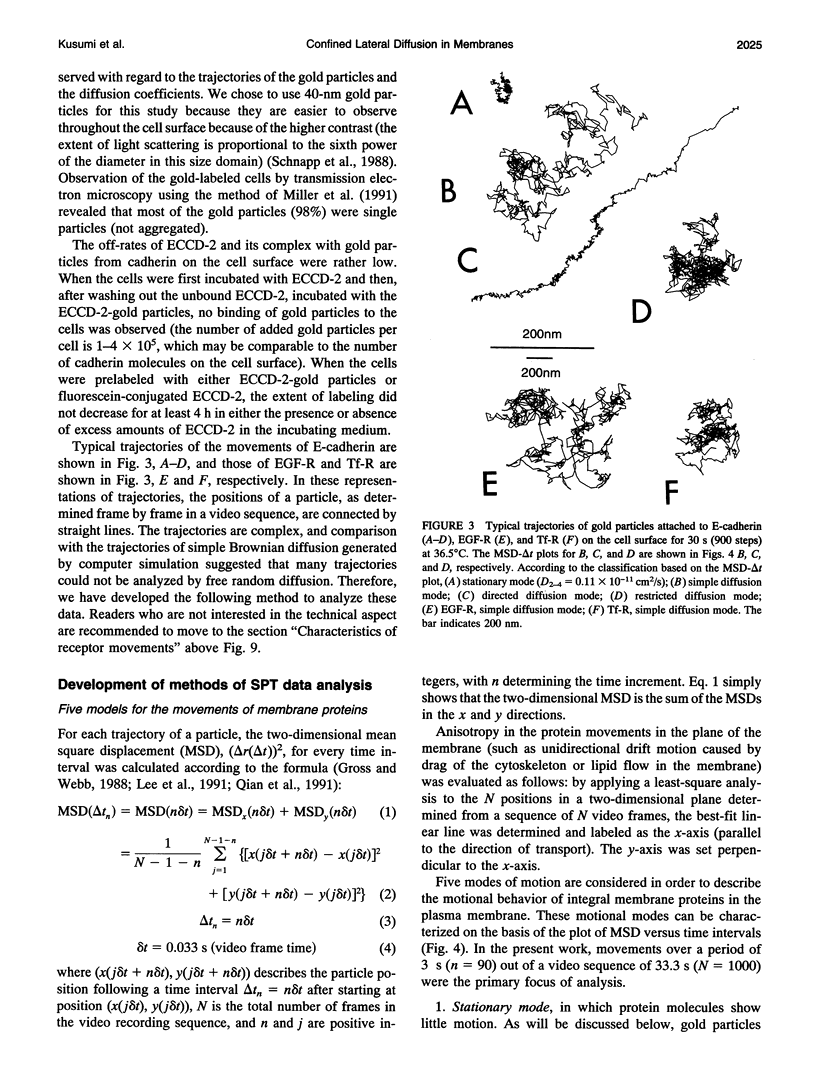
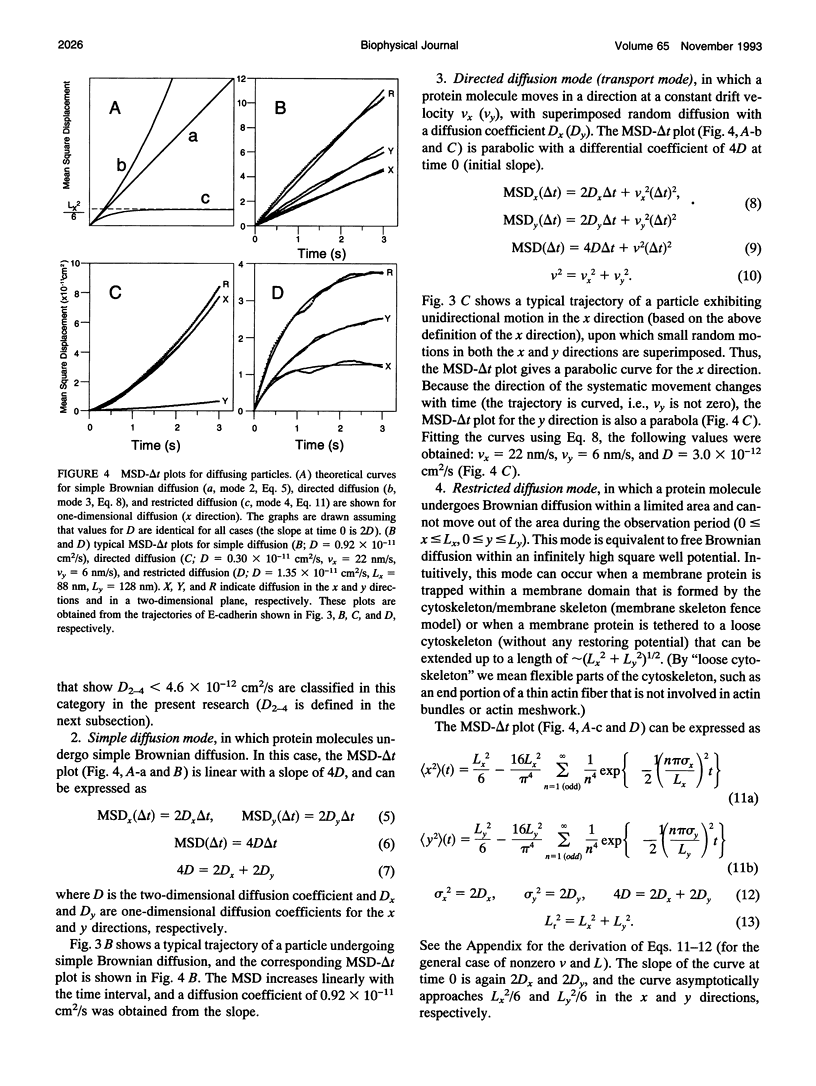
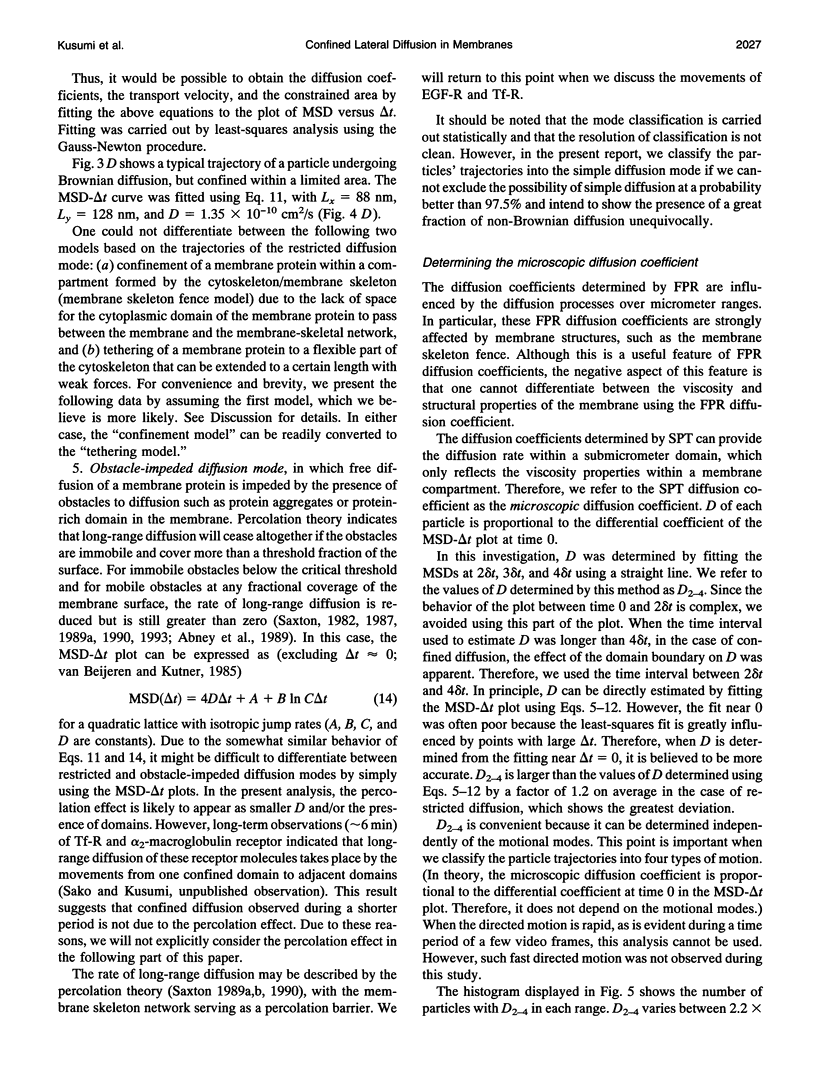
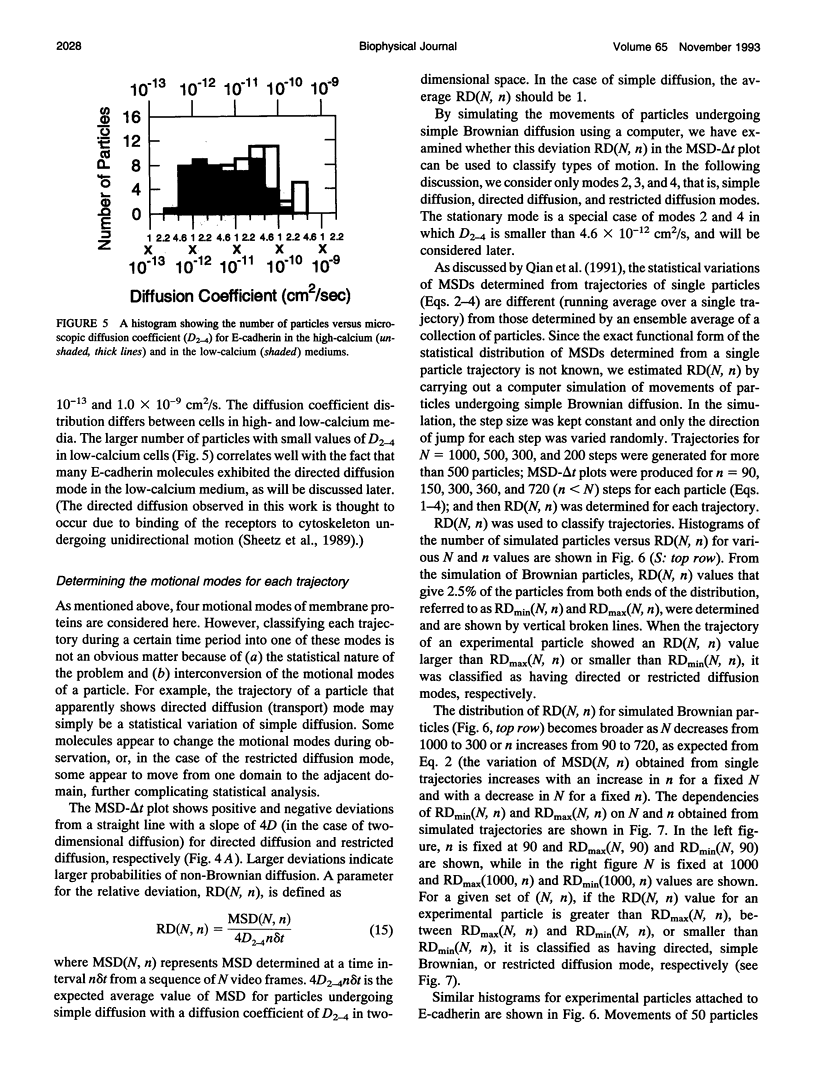
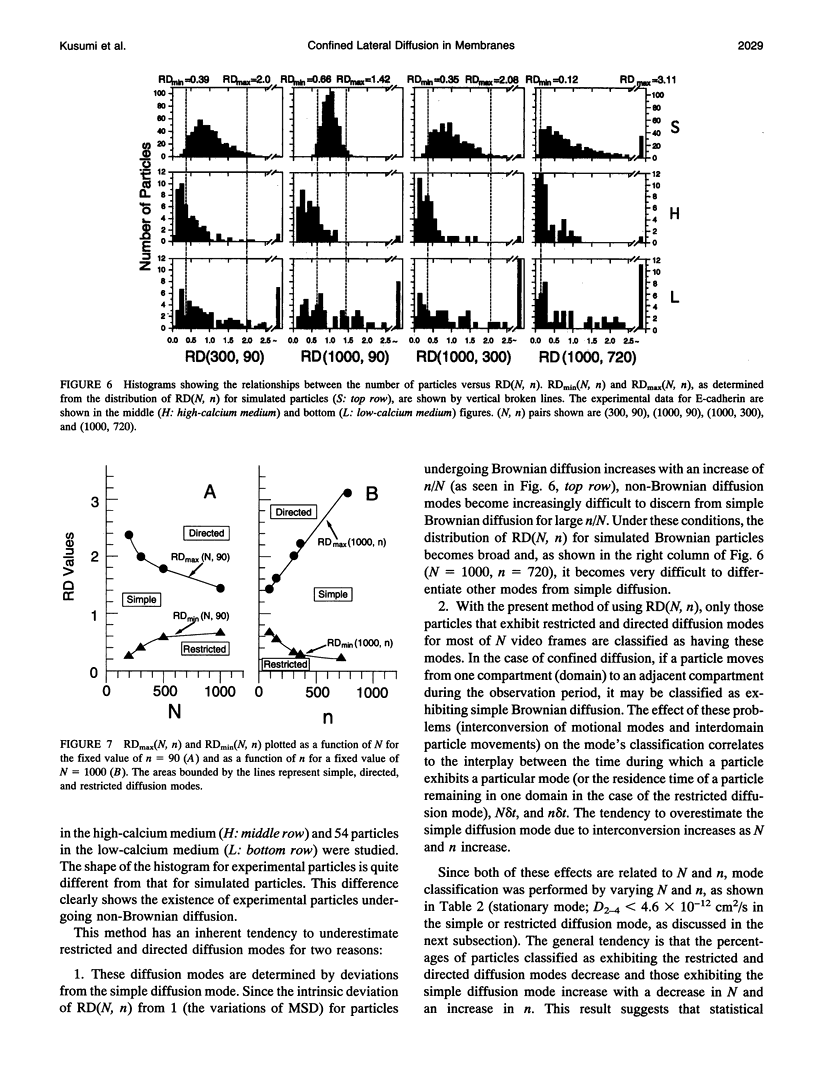
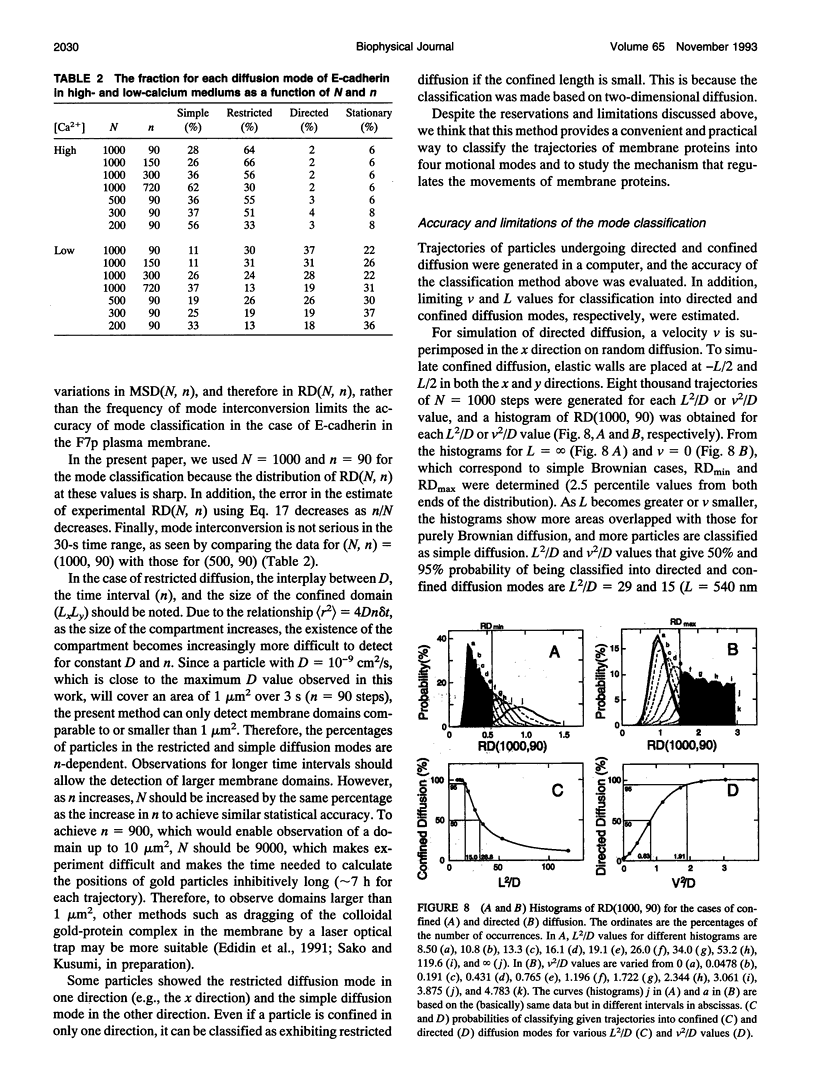
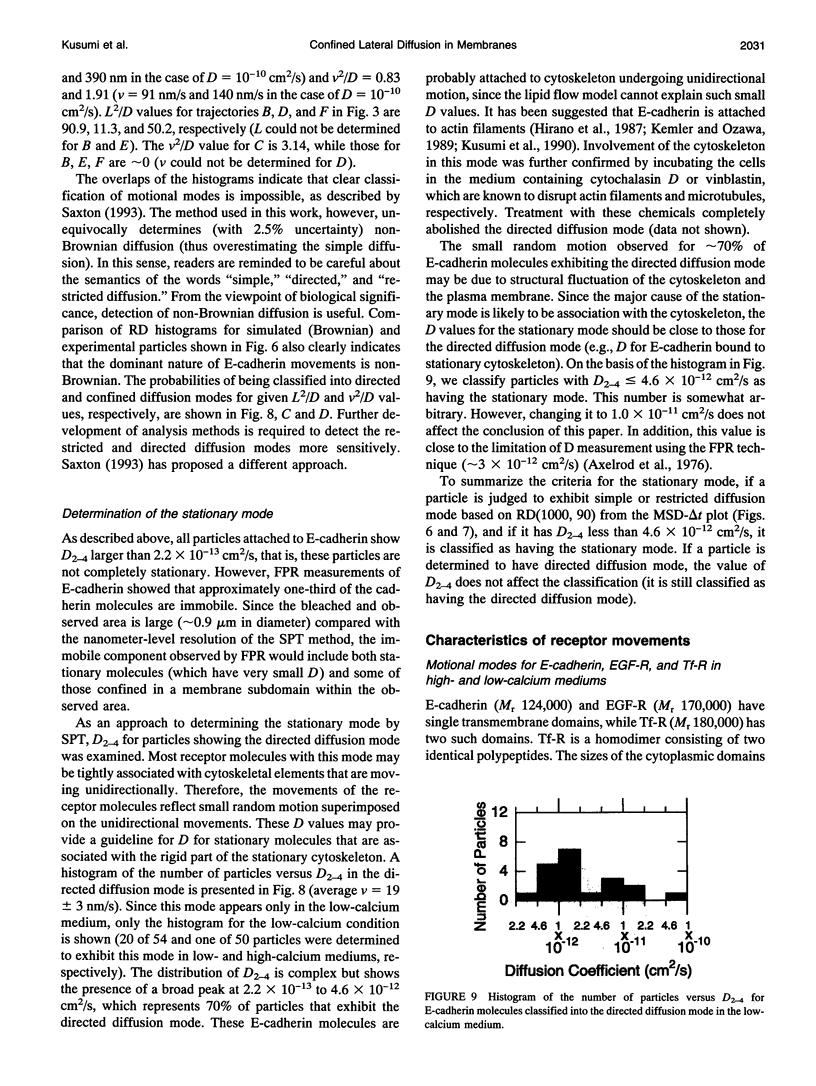
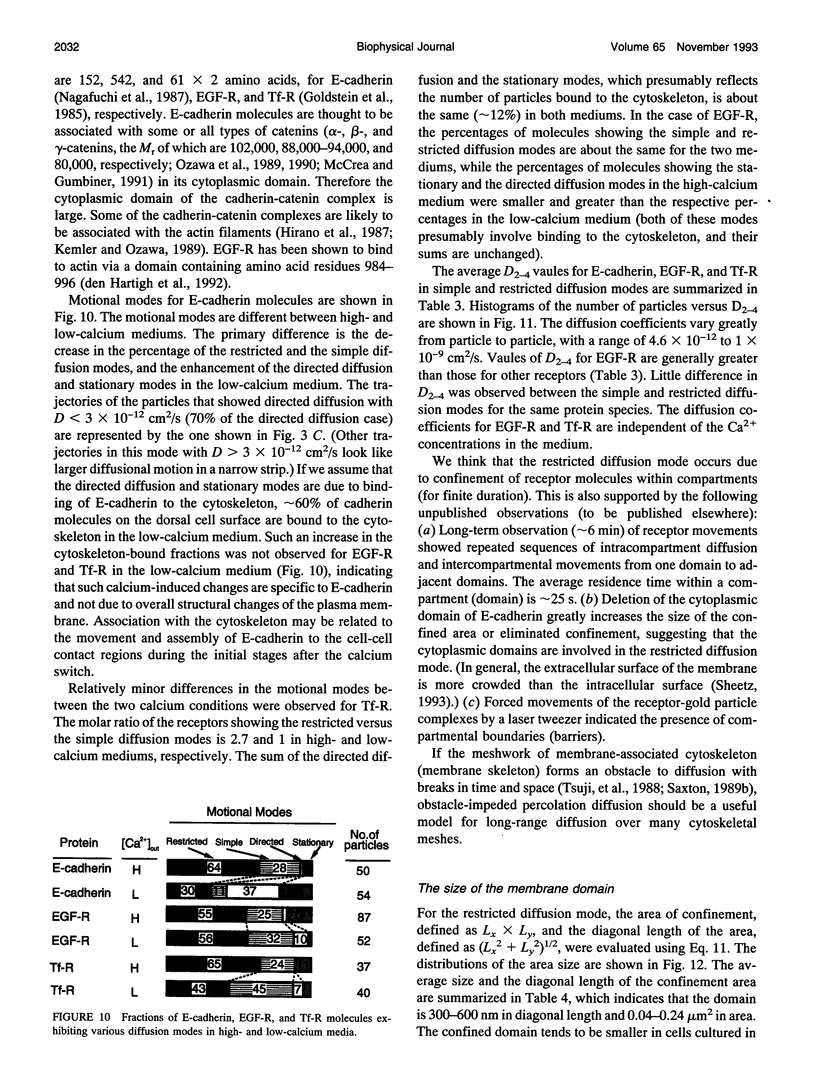
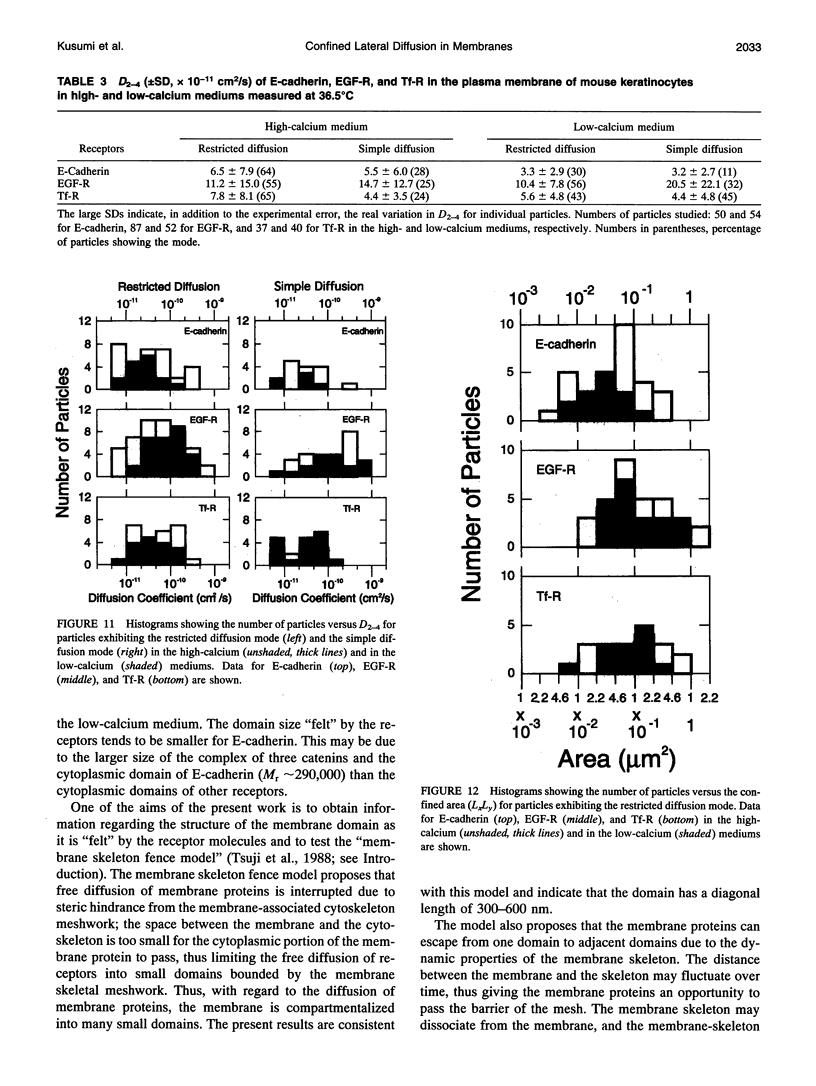
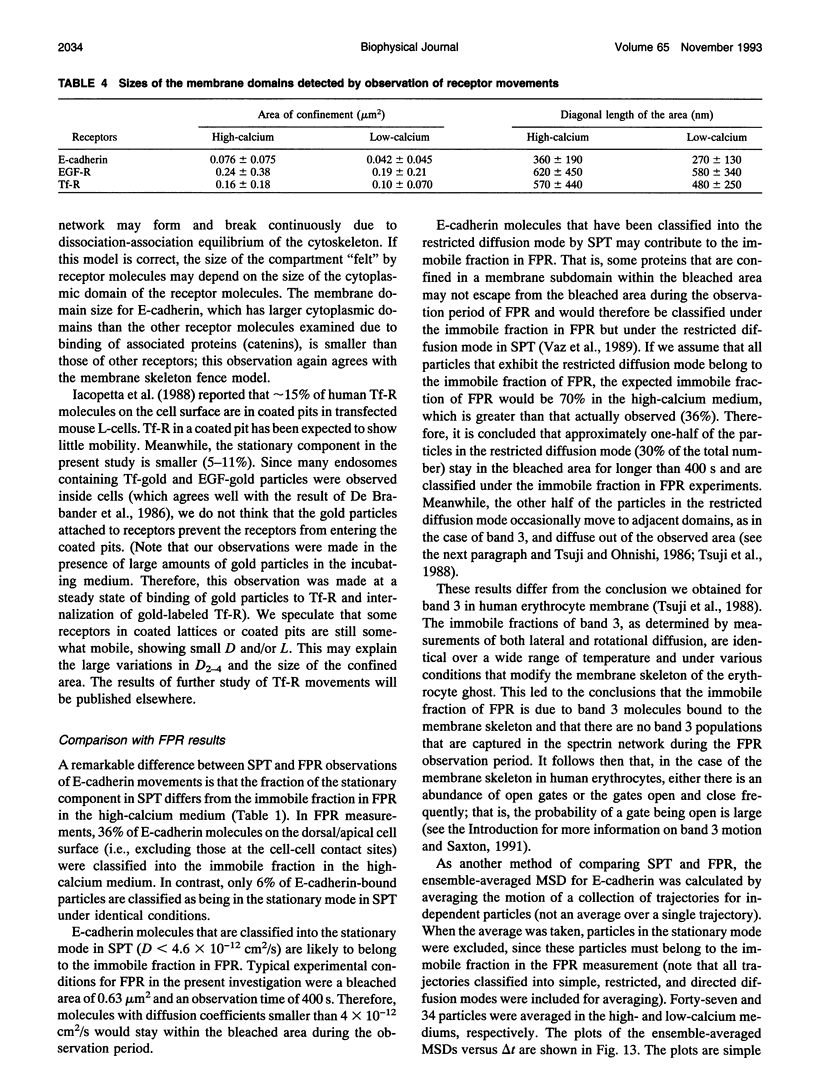
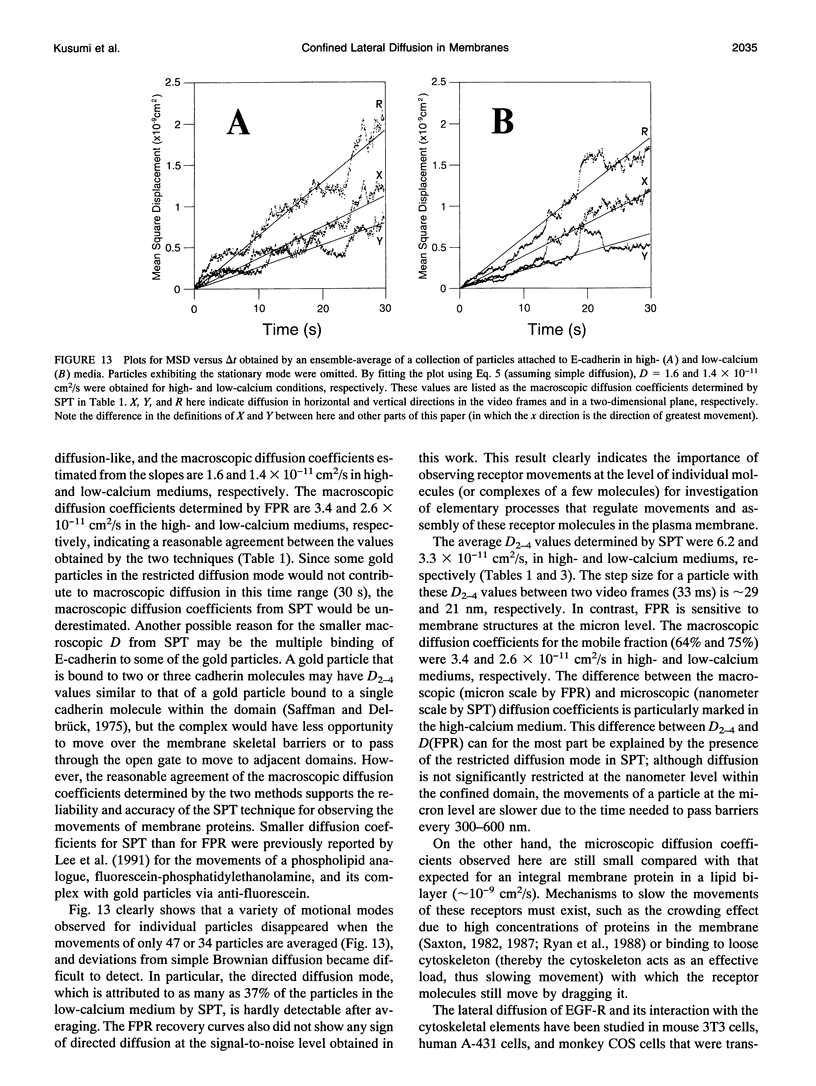
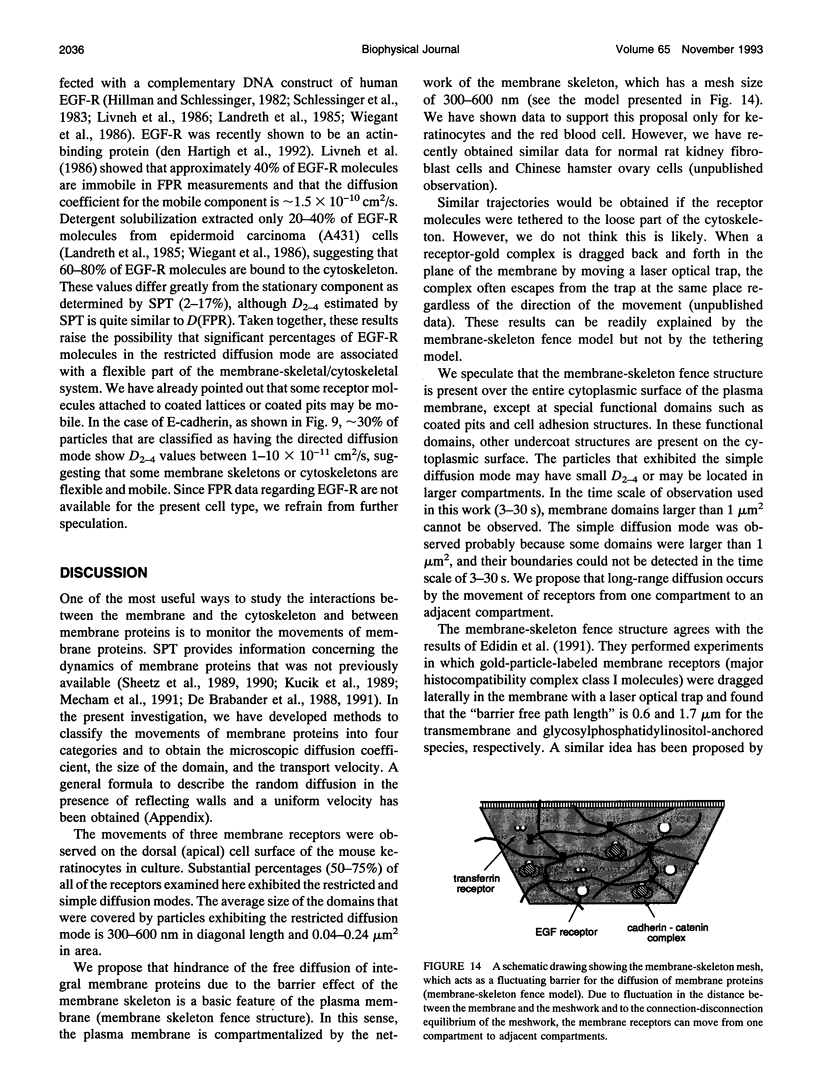
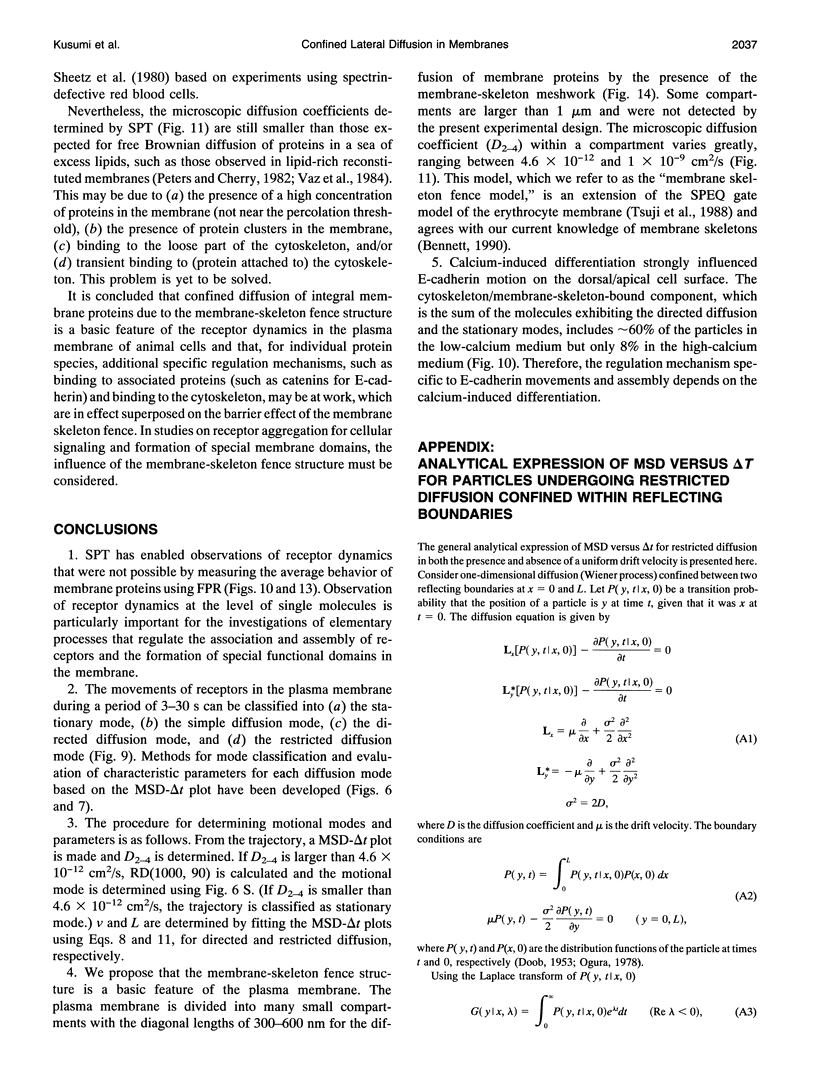
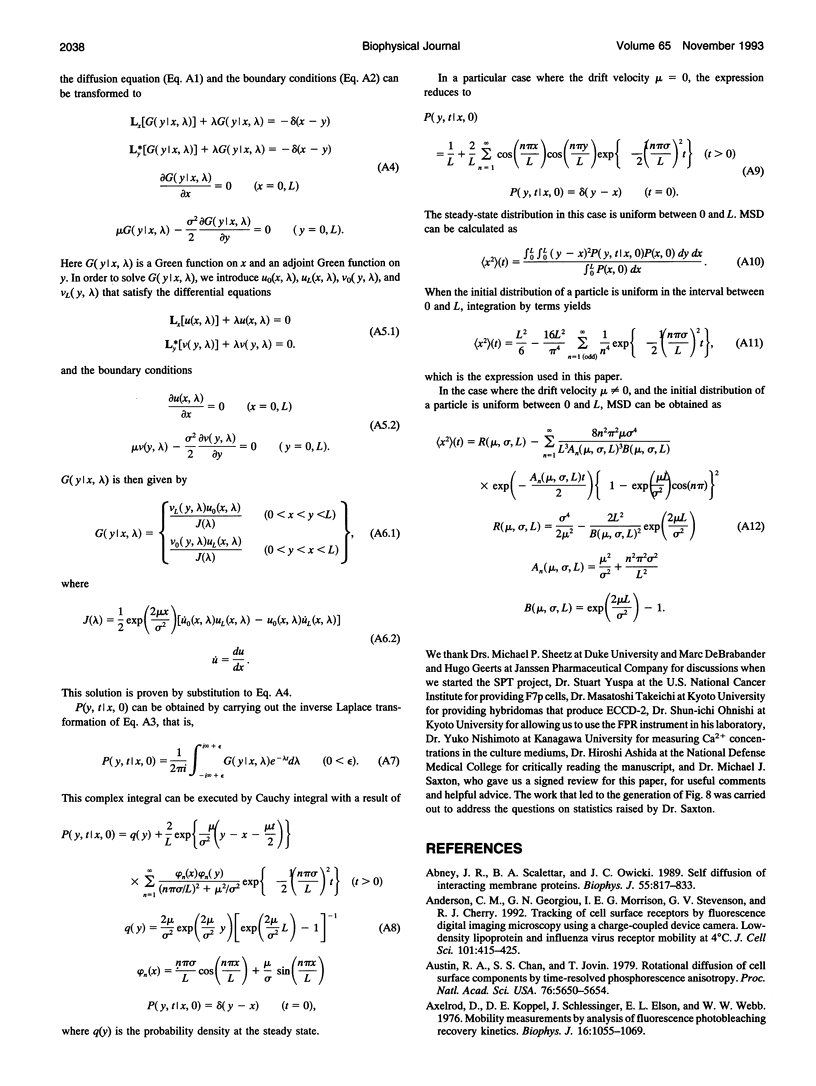
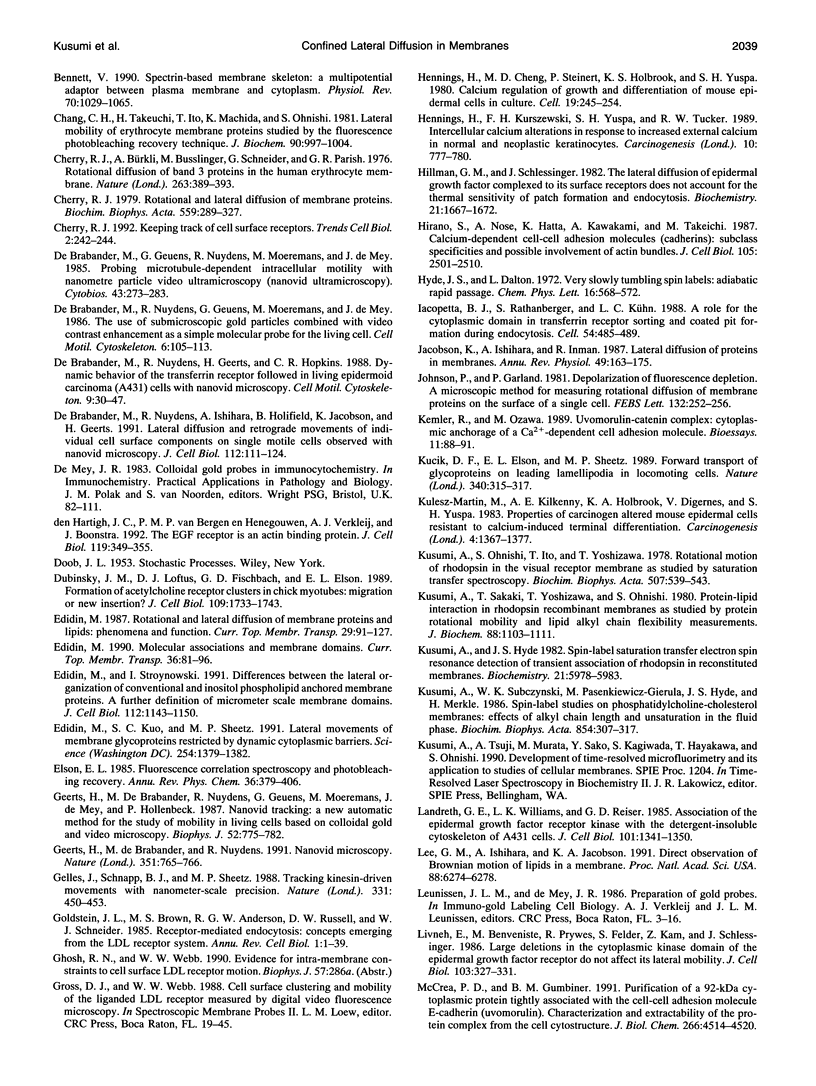
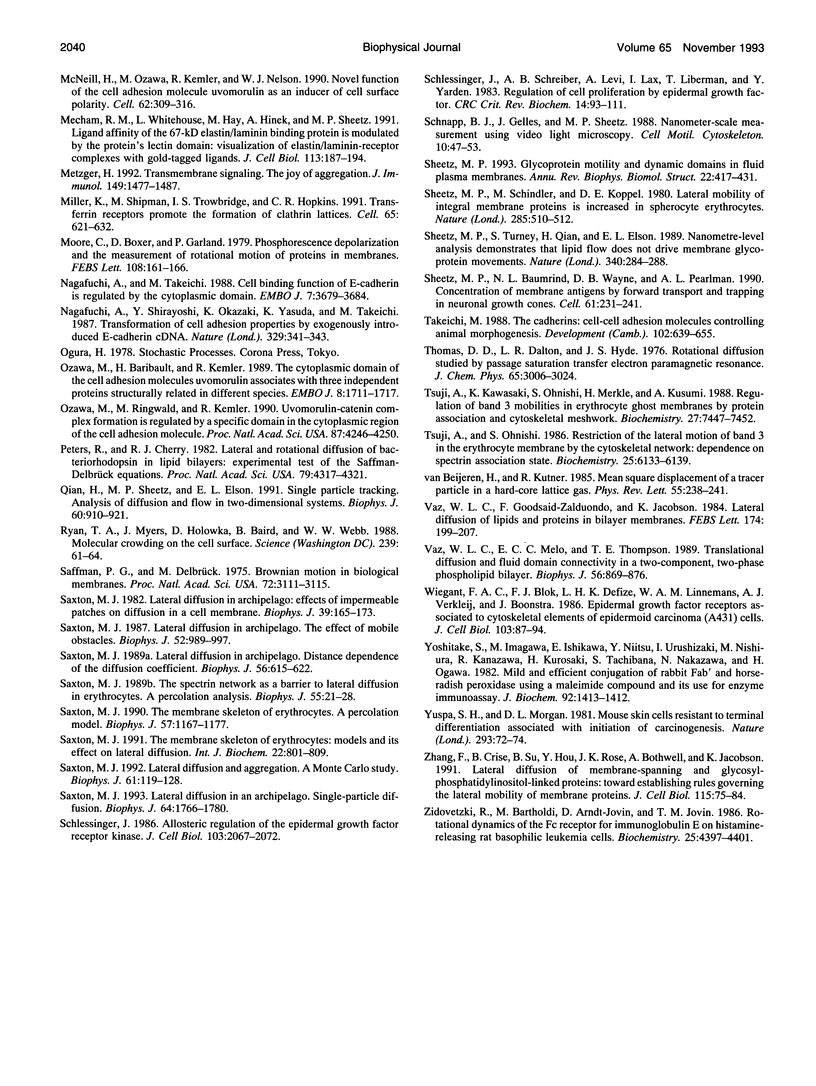
Images in this article
Selected References
These references are in PubMed. This may not be the complete list of references from this article.
- Abney J. R., Scalettar B. A., Owicki J. C. Self diffusion of interacting membrane proteins. Biophys J. 1989 May;55(5):817–833. doi: 10.1016/S0006-3495(89)82882-6. [DOI] [PMC free article] [PubMed] [Google Scholar]
- Anderson C. M., Georgiou G. N., Morrison I. E., Stevenson G. V., Cherry R. J. Tracking of cell surface receptors by fluorescence digital imaging microscopy using a charge-coupled device camera. Low-density lipoprotein and influenza virus receptor mobility at 4 degrees C. J Cell Sci. 1992 Feb;101(Pt 2):415–425. doi: 10.1242/jcs.101.2.415. [DOI] [PubMed] [Google Scholar]
- Austin R. H., Chan S. S., Jovin T. M. Rotational diffusion of cell surface components by time-resolved phosphorescence anisotropy. Proc Natl Acad Sci U S A. 1979 Nov;76(11):5650–5654. doi: 10.1073/pnas.76.11.5650. [DOI] [PMC free article] [PubMed] [Google Scholar]
- Axelrod D., Koppel D. E., Schlessinger J., Elson E., Webb W. W. Mobility measurement by analysis of fluorescence photobleaching recovery kinetics. Biophys J. 1976 Sep;16(9):1055–1069. doi: 10.1016/S0006-3495(76)85755-4. [DOI] [PMC free article] [PubMed] [Google Scholar]
- Bennett V. Spectrin-based membrane skeleton: a multipotential adaptor between plasma membrane and cytoplasm. Physiol Rev. 1990 Oct;70(4):1029–1065. doi: 10.1152/physrev.1990.70.4.1029. [DOI] [PubMed] [Google Scholar]
- Chang C. H., Takeuchi H., Ito T., Machida K., Ohnishi S. Lateral mobility of erythrocyte membrane proteins studied by the fluorescence photobleaching recovery technique. J Biochem. 1981 Oct;90(4):997–1004. doi: 10.1093/oxfordjournals.jbchem.a133586. [DOI] [PubMed] [Google Scholar]
- Cherry R. J., Bürkli A., Busslinger M., Schneider G., Parish G. R. Rotational diffusion of band 3 proteins in the human erythrocyte membrane. Nature. 1976 Sep 30;263(5576):389–393. doi: 10.1038/263389a0. [DOI] [PubMed] [Google Scholar]
- Cherry R. J. Keeping track of cell surface receptor. Trends Cell Biol. 1992 Aug;2(8):242–244. doi: 10.1016/0962-8924(92)90312-b. [DOI] [PubMed] [Google Scholar]
- Cherry R. J. Rotational and lateral diffusion of membrane proteins. Biochim Biophys Acta. 1979 Dec 20;559(4):289–327. doi: 10.1016/0304-4157(79)90009-1. [DOI] [PubMed] [Google Scholar]
- De Brabander M., Geuens G., Nuydens R., Moeremans M., De Mey J. Probing microtubule-dependent intracellular motility with nanometre particle video ultramicroscopy (nanovid ultramicroscopy). Cytobios. 1985;43(174S):273–283. [PubMed] [Google Scholar]
- De Brabander M., Nuydens R., Geerts H., Hopkins C. R. Dynamic behavior of the transferrin receptor followed in living epidermoid carcinoma (A431) cells with nanovid microscopy. Cell Motil Cytoskeleton. 1988;9(1):30–47. doi: 10.1002/cm.970090105. [DOI] [PubMed] [Google Scholar]
- De Brabander M., Nuydens R., Geuens G., Moeremans M., De Mey J. The use of submicroscopic gold particles combined with video contrast enhancement as a simple molecular probe for the living cell. Cell Motil Cytoskeleton. 1986;6(2):105–113. doi: 10.1002/cm.970060207. [DOI] [PubMed] [Google Scholar]
- Dubinsky J. M., Loftus D. J., Fischbach G. D., Elson E. L. Formation of acetylcholine receptor clusters in chick myotubes: migration or new insertion? J Cell Biol. 1989 Oct;109(4 Pt 1):1733–1743. doi: 10.1083/jcb.109.4.1733. [DOI] [PMC free article] [PubMed] [Google Scholar]
- Edidin M., Kuo S. C., Sheetz M. P. Lateral movements of membrane glycoproteins restricted by dynamic cytoplasmic barriers. Science. 1991 Nov 29;254(5036):1379–1382. doi: 10.1126/science.1835798. [DOI] [PubMed] [Google Scholar]
- Edidin M., Stroynowski I. Differences between the lateral organization of conventional and inositol phospholipid-anchored membrane proteins. A further definition of micrometer scale membrane domains. J Cell Biol. 1991 Mar;112(6):1143–1150. doi: 10.1083/jcb.112.6.1143. [DOI] [PMC free article] [PubMed] [Google Scholar]
- Geerts H., De Brabander M., Nuydens R., Geuens S., Moeremans M., De Mey J., Hollenbeck P. Nanovid tracking: a new automatic method for the study of mobility in living cells based on colloidal gold and video microscopy. Biophys J. 1987 Nov;52(5):775–782. doi: 10.1016/S0006-3495(87)83271-X. [DOI] [PMC free article] [PubMed] [Google Scholar]
- Geerts H., de Brabander M., Nuydens R. Nanovid microscopy. Nature. 1991 Jun 27;351(6329):765–766. doi: 10.1038/351765a0. [DOI] [PubMed] [Google Scholar]
- Gelles J., Schnapp B. J., Sheetz M. P. Tracking kinesin-driven movements with nanometre-scale precision. Nature. 1988 Feb 4;331(6155):450–453. doi: 10.1038/331450a0. [DOI] [PubMed] [Google Scholar]
- Goldstein J. L., Brown M. S., Anderson R. G., Russell D. W., Schneider W. J. Receptor-mediated endocytosis: concepts emerging from the LDL receptor system. Annu Rev Cell Biol. 1985;1:1–39. doi: 10.1146/annurev.cb.01.110185.000245. [DOI] [PubMed] [Google Scholar]
- Hennings H., Kruszewski F. H., Yuspa S. H., Tucker R. W. Intracellular calcium alterations in response to increased external calcium in normal and neoplastic keratinocytes. Carcinogenesis. 1989 Apr;10(4):777–780. doi: 10.1093/carcin/10.4.777. [DOI] [PubMed] [Google Scholar]
- Hennings H., Michael D., Cheng C., Steinert P., Holbrook K., Yuspa S. H. Calcium regulation of growth and differentiation of mouse epidermal cells in culture. Cell. 1980 Jan;19(1):245–254. doi: 10.1016/0092-8674(80)90406-7. [DOI] [PubMed] [Google Scholar]
- Hillman G. M., Schlessinger J. Lateral diffusion of epidermal growth factor complexed to its surface receptors does not account for the thermal sensitivity of patch formation and endocytosis. Biochemistry. 1982 Mar 30;21(7):1667–1672. doi: 10.1021/bi00536a030. [DOI] [PubMed] [Google Scholar]
- Hirano S., Nose A., Hatta K., Kawakami A., Takeichi M. Calcium-dependent cell-cell adhesion molecules (cadherins): subclass specificities and possible involvement of actin bundles. J Cell Biol. 1987 Dec;105(6 Pt 1):2501–2510. doi: 10.1083/jcb.105.6.2501. [DOI] [PMC free article] [PubMed] [Google Scholar]
- Iacopetta B. J., Rothenberger S., Kühn L. C. A role for the cytoplasmic domain in transferrin receptor sorting and coated pit formation during endocytosis. Cell. 1988 Aug 12;54(4):485–489. doi: 10.1016/0092-8674(88)90069-4. [DOI] [PubMed] [Google Scholar]
- Jacobson K., Ishihara A., Inman R. Lateral diffusion of proteins in membranes. Annu Rev Physiol. 1987;49:163–175. doi: 10.1146/annurev.ph.49.030187.001115. [DOI] [PubMed] [Google Scholar]
- Johnson P., Garland P. B. Depolarization of fluorescence depletion. A microscopic method for measuring rotational diffusion of membrane proteins on the surface of a single cell. FEBS Lett. 1981 Sep 28;132(2):252–256. doi: 10.1016/0014-5793(81)81172-6. [DOI] [PubMed] [Google Scholar]
- Kemler R., Ozawa M. Uvomorulin-catenin complex: cytoplasmic anchorage of a Ca2+-dependent cell adhesion molecule. Bioessays. 1989 Oct;11(4):88–91. doi: 10.1002/bies.950110403. [DOI] [PubMed] [Google Scholar]
- Kucik D. F., Elson E. L., Sheetz M. P. Forward transport of glycoproteins on leading lamellipodia in locomoting cells. Nature. 1989 Jul 27;340(6231):315–317. doi: 10.1038/340315a0. [DOI] [PubMed] [Google Scholar]
- Kulesz-Martin M., Kilkenny A. E., Holbrook K. A., Digernes V., Yuspa S. H. Properties of carcinogen altered mouse epidermal cells resistant to calcium-induced terminal differentiation. Carcinogenesis. 1983 Nov;4(11):1367–1377. doi: 10.1093/carcin/4.11.1367. [DOI] [PubMed] [Google Scholar]
- Kusumi A., Hyde J. S. Spin-label saturation-transfer electron spin resonance detection of transient association of rhodopsin in reconstituted membranes. Biochemistry. 1982 Nov 9;21(23):5978–5983. doi: 10.1021/bi00266a039. [DOI] [PubMed] [Google Scholar]
- Kusumi A., Sakaki T., Yoshizawa T., Ohnishi S. Protein-lipid interaction in rhodopsin recombinant membranes as studied by protein rotational mobility and lipid alkyl chain flexibility measurements. J Biochem. 1980 Oct;88(4):1103–1111. doi: 10.1093/oxfordjournals.jbchem.a133063. [DOI] [PubMed] [Google Scholar]
- Kusumi A., Subczynski W. K., Pasenkiewicz-Gierula M., Hyde J. S., Merkle H. Spin-label studies on phosphatidylcholine-cholesterol membranes: effects of alkyl chain length and unsaturation in the fluid phase. Biochim Biophys Acta. 1986 Jan 29;854(2):307–317. doi: 10.1016/0005-2736(86)90124-0. [DOI] [PubMed] [Google Scholar]
- Landreth G. E., Williams L. K., Rieser G. D. Association of the epidermal growth factor receptor kinase with the detergent-insoluble cytoskeleton of A431 cells. J Cell Biol. 1985 Oct;101(4):1341–1350. doi: 10.1083/jcb.101.4.1341. [DOI] [PMC free article] [PubMed] [Google Scholar]
- Lee G. M., Ishihara A., Jacobson K. A. Direct observation of brownian motion of lipids in a membrane. Proc Natl Acad Sci U S A. 1991 Jul 15;88(14):6274–6278. doi: 10.1073/pnas.88.14.6274. [DOI] [PMC free article] [PubMed] [Google Scholar]
- Livneh E., Benveniste M., Prywes R., Felder S., Kam Z., Schlessinger J. Large deletions in the cytoplasmic kinase domain of the epidermal growth factor receptor do not affect its laternal mobility. J Cell Biol. 1986 Aug;103(2):327–331. doi: 10.1083/jcb.103.2.327. [DOI] [PMC free article] [PubMed] [Google Scholar]
- McCrea P. D., Gumbiner B. M. Purification of a 92-kDa cytoplasmic protein tightly associated with the cell-cell adhesion molecule E-cadherin (uvomorulin). Characterization and extractability of the protein complex from the cell cytostructure. J Biol Chem. 1991 Mar 5;266(7):4514–4520. [PubMed] [Google Scholar]
- McNeill H., Ozawa M., Kemler R., Nelson W. J. Novel function of the cell adhesion molecule uvomorulin as an inducer of cell surface polarity. Cell. 1990 Jul 27;62(2):309–316. doi: 10.1016/0092-8674(90)90368-o. [DOI] [PubMed] [Google Scholar]
- Mecham R. P., Whitehouse L., Hay M., Hinek A., Sheetz M. P. Ligand affinity of the 67-kD elastin/laminin binding protein is modulated by the protein's lectin domain: visualization of elastin/laminin-receptor complexes with gold-tagged ligands. J Cell Biol. 1991 Apr;113(1):187–194. doi: 10.1083/jcb.113.1.187. [DOI] [PMC free article] [PubMed] [Google Scholar]
- Metzger H. Transmembrane signaling: the joy of aggregation. J Immunol. 1992 Sep 1;149(5):1477–1487. [PubMed] [Google Scholar]
- Miller K., Shipman M., Trowbridge I. S., Hopkins C. R. Transferrin receptors promote the formation of clathrin lattices. Cell. 1991 May 17;65(4):621–632. doi: 10.1016/0092-8674(91)90094-f. [DOI] [PubMed] [Google Scholar]
- Moore C., Boxer D., Garland P. Phosphorescence depolarization and the measurement of rotational motion of proteins in membranes. FEBS Lett. 1979 Dec 1;108(1):161–166. doi: 10.1016/0014-5793(79)81200-4. [DOI] [PubMed] [Google Scholar]
- Nagafuchi A., Shirayoshi Y., Okazaki K., Yasuda K., Takeichi M. Transformation of cell adhesion properties by exogenously introduced E-cadherin cDNA. Nature. 1987 Sep 24;329(6137):341–343. doi: 10.1038/329341a0. [DOI] [PubMed] [Google Scholar]
- Nagafuchi A., Takeichi M. Cell binding function of E-cadherin is regulated by the cytoplasmic domain. EMBO J. 1988 Dec 1;7(12):3679–3684. doi: 10.1002/j.1460-2075.1988.tb03249.x. [DOI] [PMC free article] [PubMed] [Google Scholar]
- Ozawa M., Baribault H., Kemler R. The cytoplasmic domain of the cell adhesion molecule uvomorulin associates with three independent proteins structurally related in different species. EMBO J. 1989 Jun;8(6):1711–1717. doi: 10.1002/j.1460-2075.1989.tb03563.x. [DOI] [PMC free article] [PubMed] [Google Scholar]
- Ozawa M., Ringwald M., Kemler R. Uvomorulin-catenin complex formation is regulated by a specific domain in the cytoplasmic region of the cell adhesion molecule. Proc Natl Acad Sci U S A. 1990 Jun;87(11):4246–4250. doi: 10.1073/pnas.87.11.4246. [DOI] [PMC free article] [PubMed] [Google Scholar]
- Peters R., Cherry R. J. Lateral and rotational diffusion of bacteriorhodopsin in lipid bilayers: experimental test of the Saffman-Delbrück equations. Proc Natl Acad Sci U S A. 1982 Jul;79(14):4317–4321. doi: 10.1073/pnas.79.14.4317. [DOI] [PMC free article] [PubMed] [Google Scholar]
- Qian H., Sheetz M. P., Elson E. L. Single particle tracking. Analysis of diffusion and flow in two-dimensional systems. Biophys J. 1991 Oct;60(4):910–921. doi: 10.1016/S0006-3495(91)82125-7. [DOI] [PMC free article] [PubMed] [Google Scholar]
- Ryan T. A., Myers J., Holowka D., Baird B., Webb W. W. Molecular crowding on the cell surface. Science. 1988 Jan 1;239(4835):61–64. doi: 10.1126/science.2962287. [DOI] [PubMed] [Google Scholar]
- Saffman P. G., Delbrück M. Brownian motion in biological membranes. Proc Natl Acad Sci U S A. 1975 Aug;72(8):3111–3113. doi: 10.1073/pnas.72.8.3111. [DOI] [PMC free article] [PubMed] [Google Scholar]
- Saxton M. J. Lateral diffusion and aggregation. A Monte Carlo study. Biophys J. 1992 Jan;61(1):119–128. doi: 10.1016/S0006-3495(92)81821-0. [DOI] [PMC free article] [PubMed] [Google Scholar]
- Saxton M. J. Lateral diffusion in an archipelago. Distance dependence of the diffusion coefficient. Biophys J. 1989 Sep;56(3):615–622. doi: 10.1016/S0006-3495(89)82708-0. [DOI] [PMC free article] [PubMed] [Google Scholar]
- Saxton M. J. Lateral diffusion in an archipelago. Effects of impermeable patches on diffusion in a cell membrane. Biophys J. 1982 Aug;39(2):165–173. doi: 10.1016/S0006-3495(82)84504-9. [DOI] [PMC free article] [PubMed] [Google Scholar]
- Saxton M. J. Lateral diffusion in an archipelago. Single-particle diffusion. Biophys J. 1993 Jun;64(6):1766–1780. doi: 10.1016/S0006-3495(93)81548-0. [DOI] [PMC free article] [PubMed] [Google Scholar]
- Saxton M. J. Lateral diffusion in an archipelago. The effect of mobile obstacles. Biophys J. 1987 Dec;52(6):989–997. doi: 10.1016/S0006-3495(87)83291-5. [DOI] [PMC free article] [PubMed] [Google Scholar]
- Saxton M. J. The membrane skeleton of erythrocytes. A percolation model. Biophys J. 1990 Jun;57(6):1167–1177. doi: 10.1016/S0006-3495(90)82636-9. [DOI] [PMC free article] [PubMed] [Google Scholar]
- Saxton M. J. The membrane skeleton of erythrocytes: models of its effect on lateral diffusion. Int J Biochem. 1990;22(8):801–809. doi: 10.1016/0020-711x(90)90283-9. [DOI] [PubMed] [Google Scholar]
- Saxton M. J. The spectrin network as a barrier to lateral diffusion in erythrocytes. A percolation analysis. Biophys J. 1989 Jan;55(1):21–28. doi: 10.1016/S0006-3495(89)82776-6. [DOI] [PMC free article] [PubMed] [Google Scholar]
- Schlessinger J. Allosteric regulation of the epidermal growth factor receptor kinase. J Cell Biol. 1986 Dec;103(6 Pt 1):2067–2072. doi: 10.1083/jcb.103.6.2067. [DOI] [PMC free article] [PubMed] [Google Scholar]
- Schlessinger J., Schreiber A. B., Levi A., Lax I., Libermann T., Yarden Y. Regulation of cell proliferation by epidermal growth factor. CRC Crit Rev Biochem. 1983;14(2):93–111. doi: 10.3109/10409238309102791. [DOI] [PubMed] [Google Scholar]
- Schnapp B. J., Gelles J., Sheetz M. P. Nanometer-scale measurements using video light microscopy. Cell Motil Cytoskeleton. 1988;10(1-2):47–53. doi: 10.1002/cm.970100109. [DOI] [PubMed] [Google Scholar]
- Sheetz M. P., Baumrind N. L., Wayne D. B., Pearlman A. L. Concentration of membrane antigens by forward transport and trapping in neuronal growth cones. Cell. 1990 Apr 20;61(2):231–241. doi: 10.1016/0092-8674(90)90804-n. [DOI] [PubMed] [Google Scholar]
- Sheetz M. P. Glycoprotein motility and dynamic domains in fluid plasma membranes. Annu Rev Biophys Biomol Struct. 1993;22:417–431. doi: 10.1146/annurev.bb.22.060193.002221. [DOI] [PubMed] [Google Scholar]
- Sheetz M. P., Schindler M., Koppel D. E. Lateral mobility of integral membrane proteins is increased in spherocytic erythrocytes. Nature. 1980 Jun 12;285(5765):510–511. doi: 10.1038/285510a0. [DOI] [PubMed] [Google Scholar]
- Sheetz M. P., Turney S., Qian H., Elson E. L. Nanometre-level analysis demonstrates that lipid flow does not drive membrane glycoprotein movements. Nature. 1989 Jul 27;340(6231):284–288. doi: 10.1038/340284a0. [DOI] [PubMed] [Google Scholar]
- Takeichi M. The cadherins: cell-cell adhesion molecules controlling animal morphogenesis. Development. 1988 Apr;102(4):639–655. doi: 10.1242/dev.102.4.639. [DOI] [PubMed] [Google Scholar]
- Tsuji A., Kawasaki K., Ohnishi S., Merkle H., Kusumi A. Regulation of band 3 mobilities in erythrocyte ghost membranes by protein association and cytoskeletal meshwork. Biochemistry. 1988 Sep 20;27(19):7447–7452. doi: 10.1021/bi00419a041. [DOI] [PubMed] [Google Scholar]
- Tsuji A., Ohnishi S. Restriction of the lateral motion of band 3 in the erythrocyte membrane by the cytoskeletal network: dependence on spectrin association state. Biochemistry. 1986 Oct 7;25(20):6133–6139. doi: 10.1021/bi00368a045. [DOI] [PubMed] [Google Scholar]
- Vaz W. L., Melo E. C., Thompson T. E. Translational diffusion and fluid domain connectivity in a two-component, two-phase phospholipid bilayer. Biophys J. 1989 Nov;56(5):869–876. doi: 10.1016/S0006-3495(89)82733-X. [DOI] [PMC free article] [PubMed] [Google Scholar]
- Wiegant F. A., Blok F. J., Defize L. H., Linnemans W. A., Verkley A. J., Boonstra J. Epidermal growth factor receptors associated to cytoskeletal elements of epidermoid carcinoma (A431) cells. J Cell Biol. 1986 Jul;103(1):87–94. doi: 10.1083/jcb.103.1.87. [DOI] [PMC free article] [PubMed] [Google Scholar]
- Yoshitake S., Imagawa M., Ishikawa E., Niitsu Y., Urushizaki I., Nishiura M., Kanazawa R., Kurosaki H., Tachibana S., Nakazawa N. Mild and efficient conjugation of rabbit Fab' and horseradish peroxidase using a maleimide compound and its use for enzyme immunoassay. J Biochem. 1982 Nov;92(5):1413–1424. doi: 10.1093/oxfordjournals.jbchem.a134065. [DOI] [PubMed] [Google Scholar]
- Yuspa S. H., Morgan D. L. Mouse skin cells resistant to terminal differentiation associated with initiation of carcinogenesis. Nature. 1981 Sep 3;293(5827):72–74. doi: 10.1038/293072a0. [DOI] [PubMed] [Google Scholar]
- Zhang F., Crise B., Su B., Hou Y., Rose J. K., Bothwell A., Jacobson K. Lateral diffusion of membrane-spanning and glycosylphosphatidylinositol-linked proteins: toward establishing rules governing the lateral mobility of membrane proteins. J Cell Biol. 1991 Oct;115(1):75–84. doi: 10.1083/jcb.115.1.75. [DOI] [PMC free article] [PubMed] [Google Scholar]
- Zidovetzki R., Bartholdi M., Arndt-Jovin D., Jovin T. M. Rotational dynamics of the Fc receptor for immunoglobulin E on histamine-releasing rat basophilic leukemia cells. Biochemistry. 1986 Jul 29;25(15):4397–4401. doi: 10.1021/bi00363a033. [DOI] [PubMed] [Google Scholar]
- de Brabander M., Nuydens R., Ishihara A., Holifield B., Jacobson K., Geerts H. Lateral diffusion and retrograde movements of individual cell surface components on single motile cells observed with Nanovid microscopy. J Cell Biol. 1991 Jan;112(1):111–124. doi: 10.1083/jcb.112.1.111. [DOI] [PMC free article] [PubMed] [Google Scholar]
- den Hartigh J. C., van Bergen en Henegouwen P. M., Verkleij A. J., Boonstra J. The EGF receptor is an actin-binding protein. J Cell Biol. 1992 Oct;119(2):349–355. doi: 10.1083/jcb.119.2.349. [DOI] [PMC free article] [PubMed] [Google Scholar]
- van Beijeren H, Kutner R. Mean square displacement of a tracer particle in a hard-core lattice gas. Phys Rev Lett. 1985 Jul 8;55(2):238–241. doi: 10.1103/PhysRevLett.55.238. [DOI] [PubMed] [Google Scholar]