Abstract
A quasi-elastic light-scattering (QELS) microscope spectrometer was used to study the dynamic properties of the membrane/cytoskeleton of individual human red blood cells (RBCs). QELS is a spectroscopic technique that measures intensity fluctuations of laser light scattered from a sample. The intensity fluctuations were analyzed using power spectra and the intensity autocorrelation function, g(2)(tau), which was approximated with a single exponential. The value of the correlation time, Tcorr, was used for comparing results. Motion of the RBC membrane/cytoskeleton was previously identified as the source of the QELS signal from the RBC (R. B. Tishler and F. D. Carlson, 1987. Biophys. J. 51:993-997), and additional data supporting that conclusion are presented. Similar results were obtained from anucleate mammalian RBCs that have structures similar to that of the human RBC, but not for morphologically distinct, nucleated RBCs. The effect of altering the physical properties of the cytoplasm and the membrane/cytoskeleton was also studied. Osmotically increasing the cytoplasmic viscosity led to significant increases in Tcorr. Increasing the membrane cholesterol content and increasing the intracellular calcium content both led to decreased deformability of the human RBC. In both cases, the modified cells with decreased deformability showed an increase in Tcorr, demonstrating that QELS could measure biochemically induced changes of the membrane/cytoskeleton. Physiological changes were measured in studies of age-separated RBC populations which showed that Tcorr was increased in the older, less deformable cells.
Full text
PDF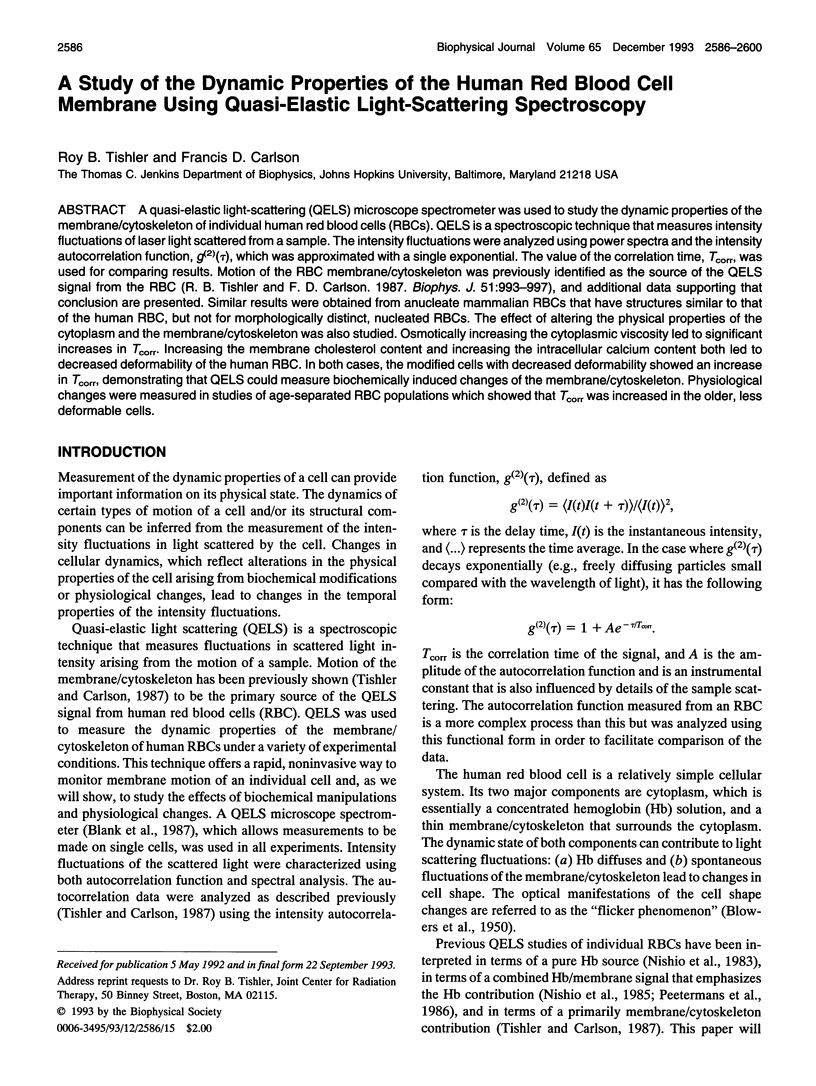
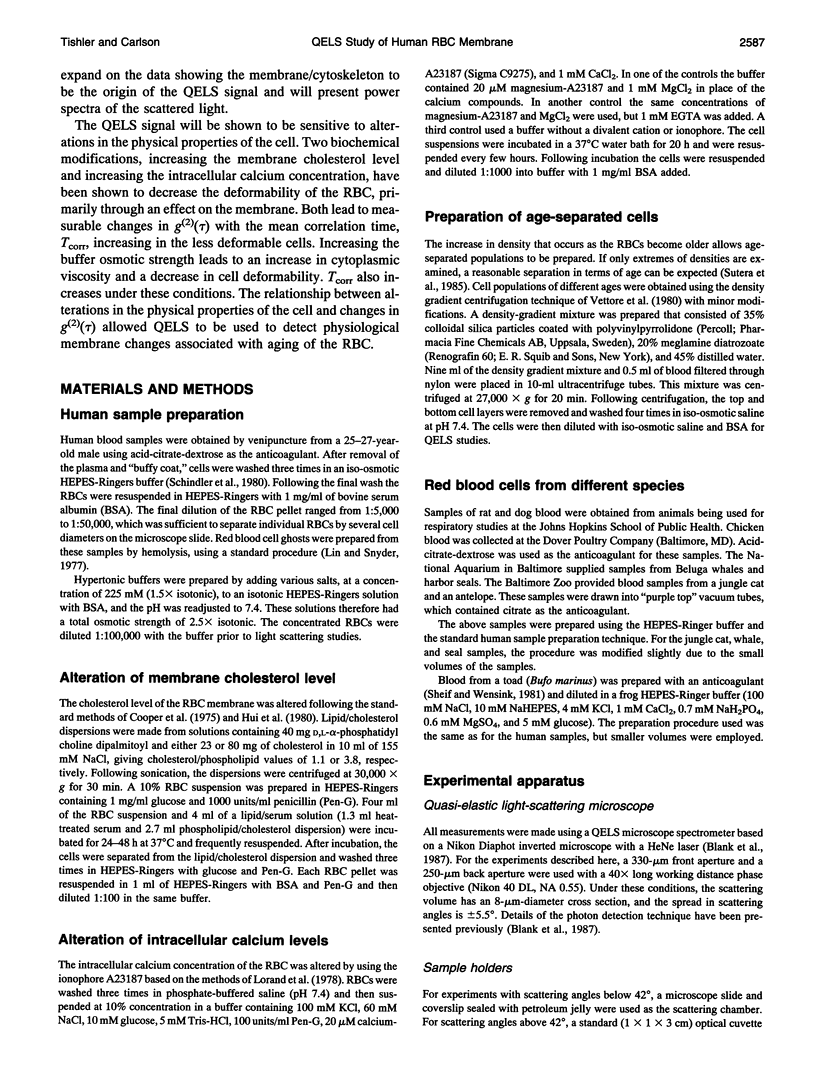
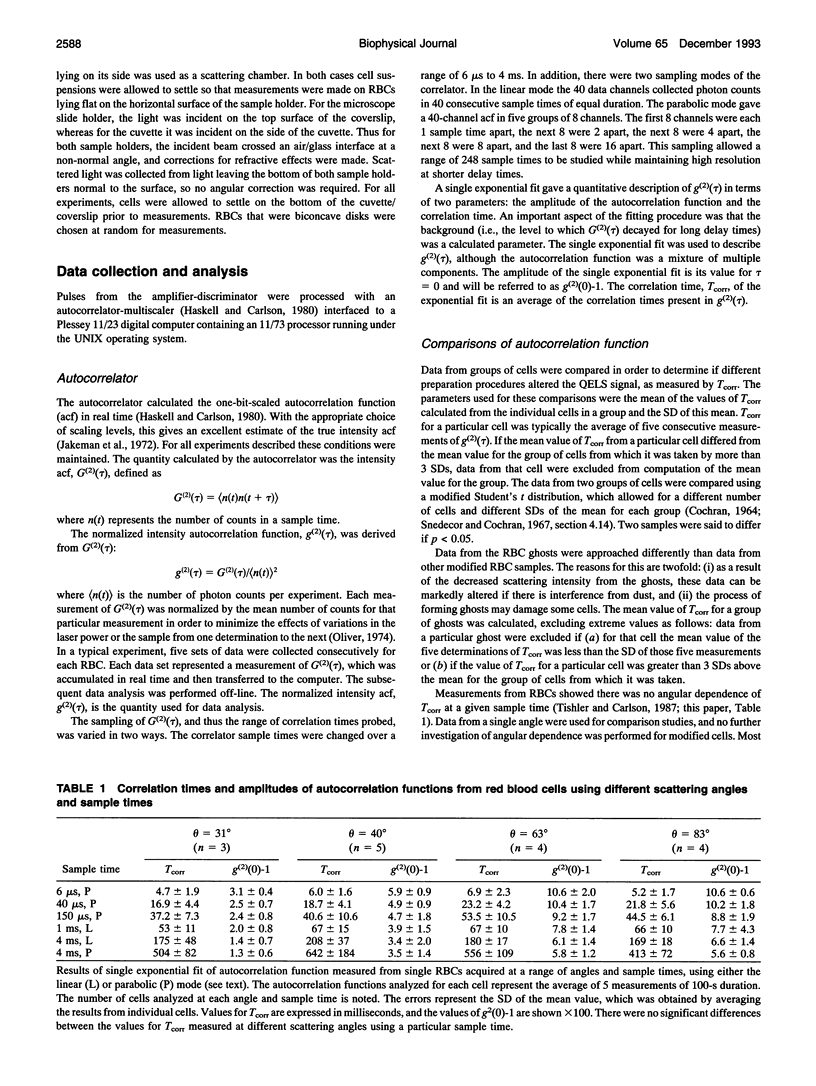
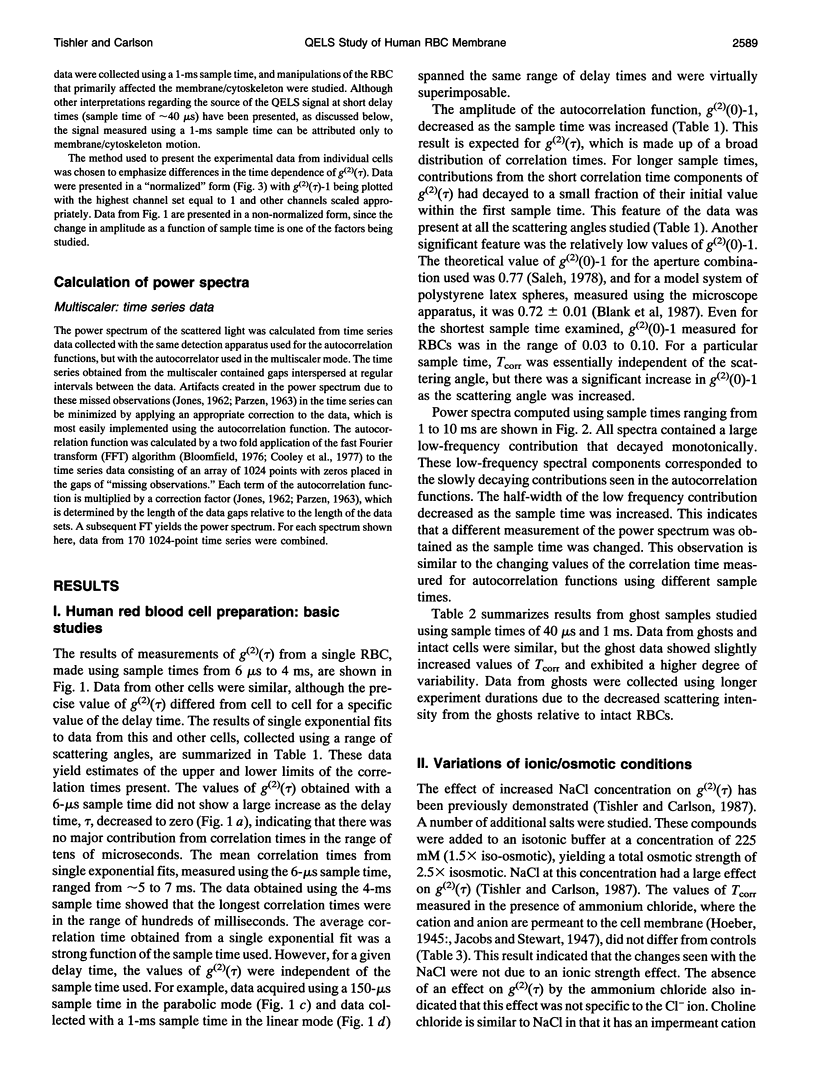
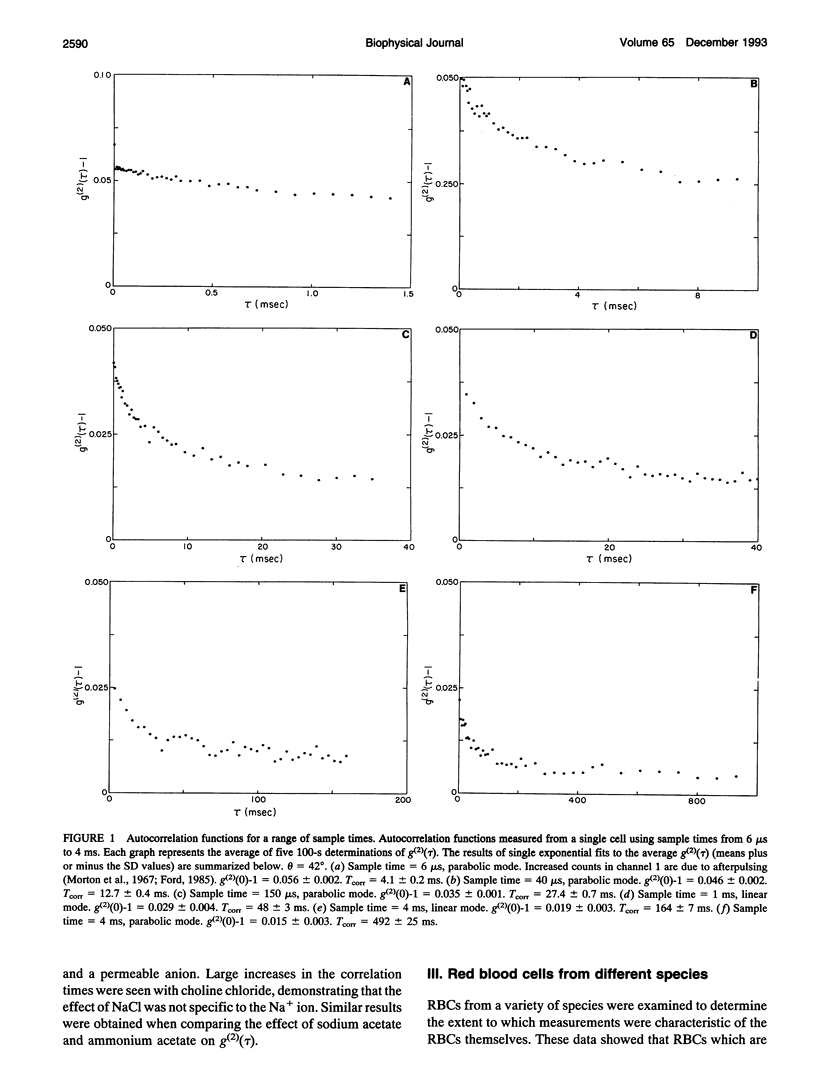
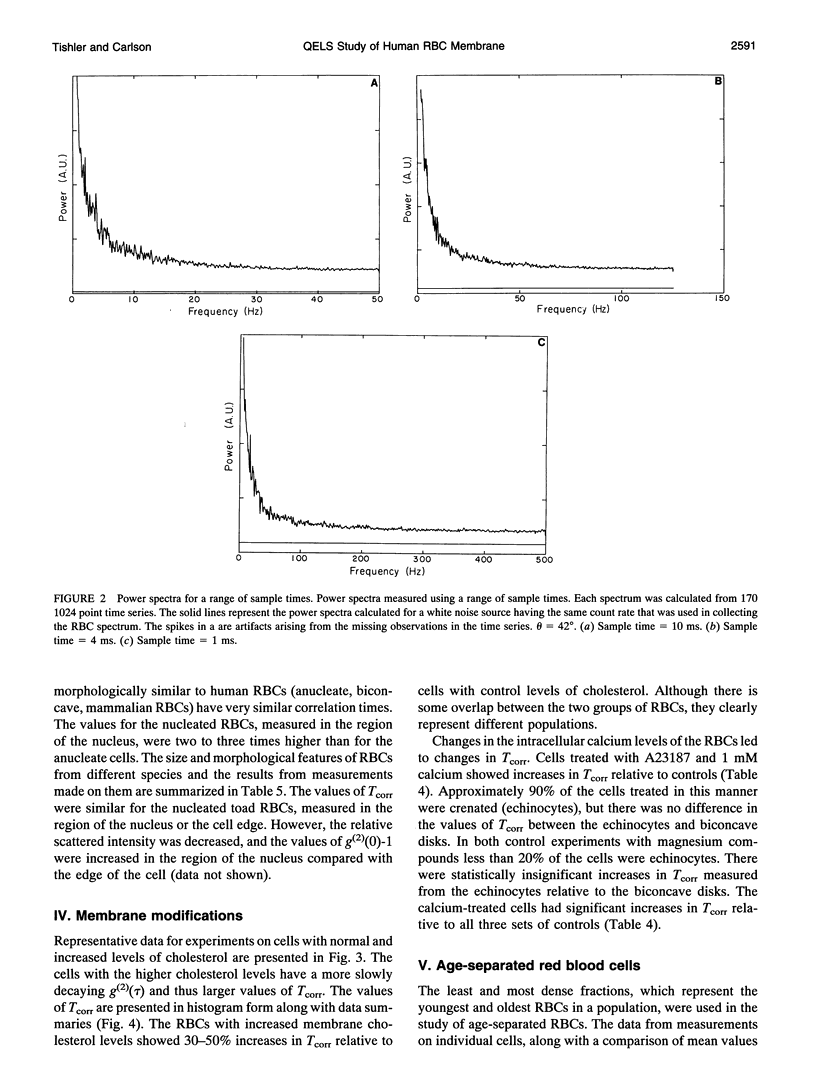
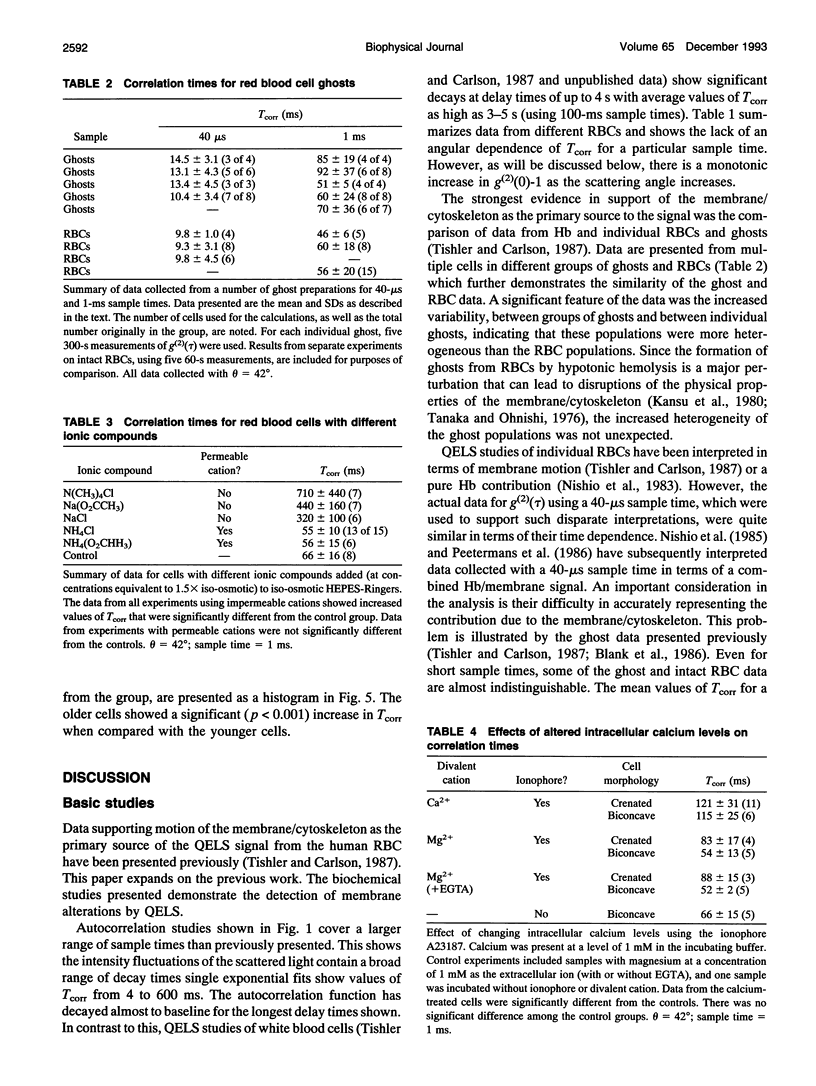
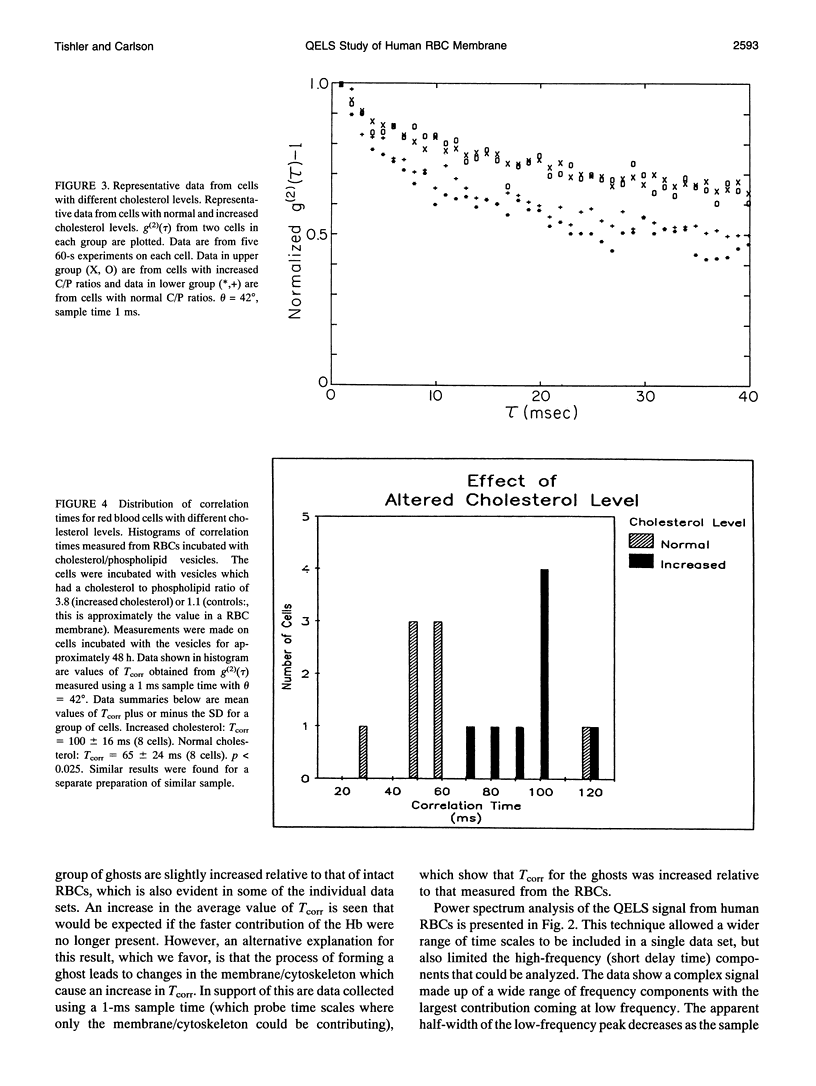
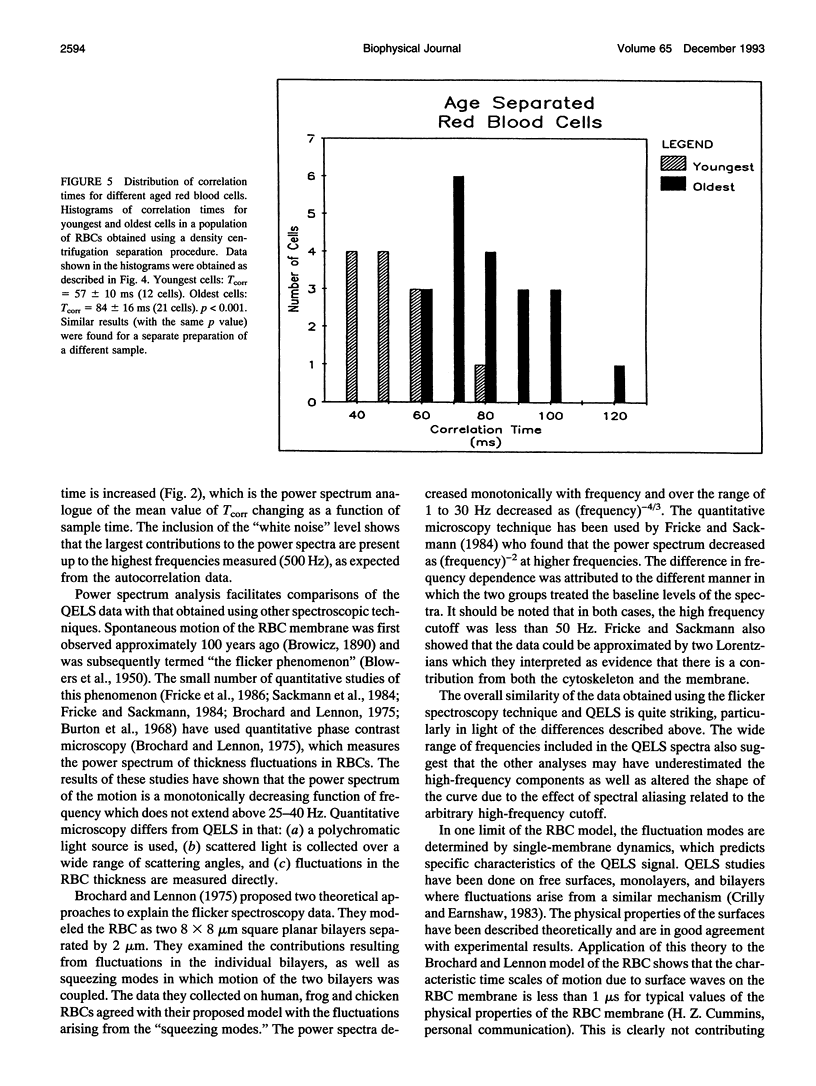
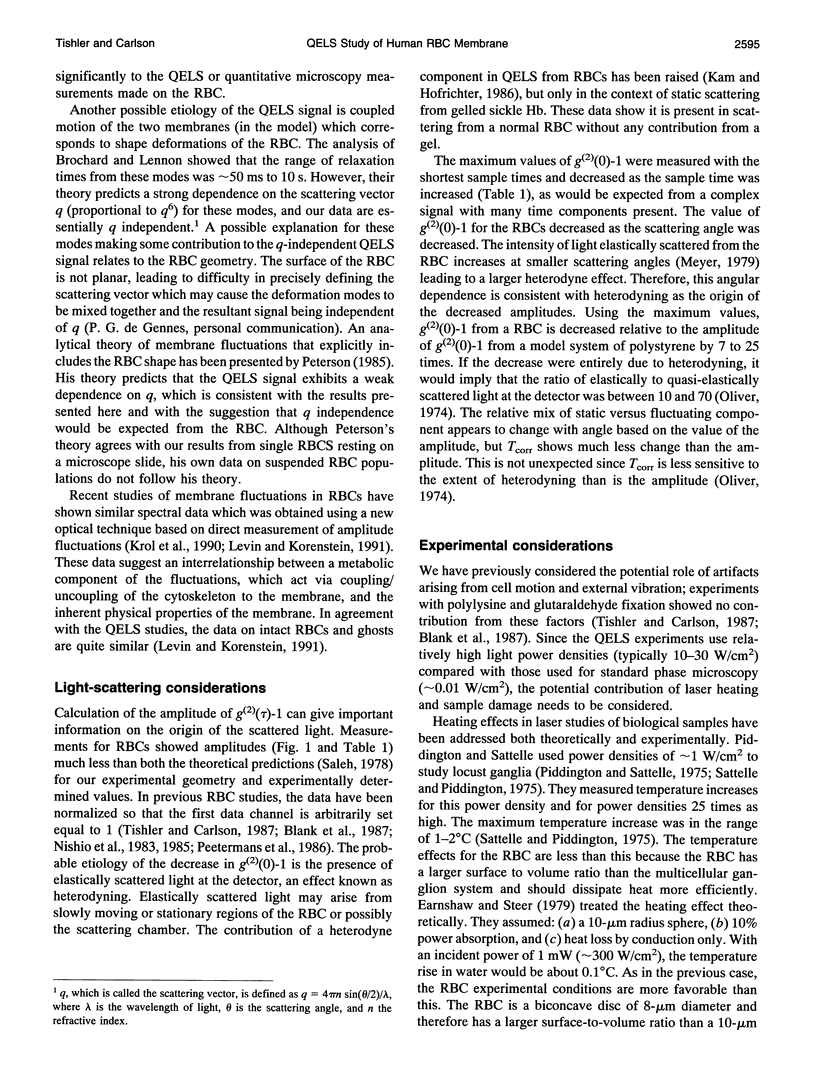
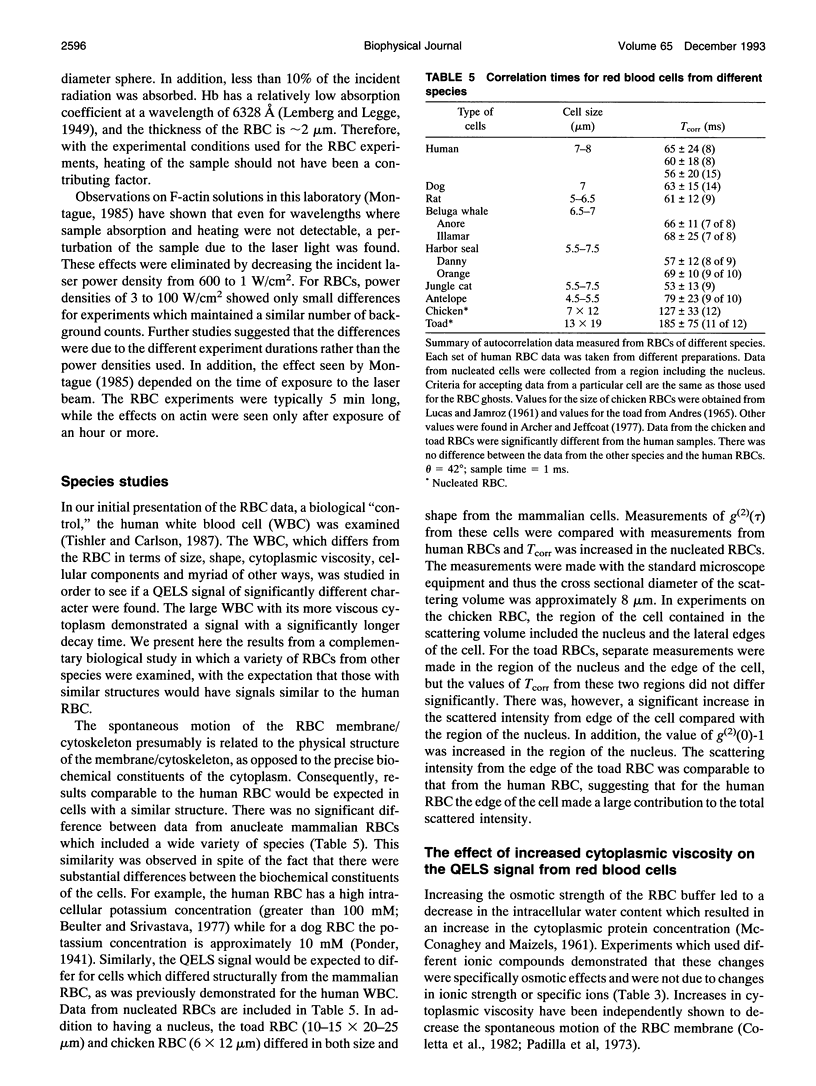
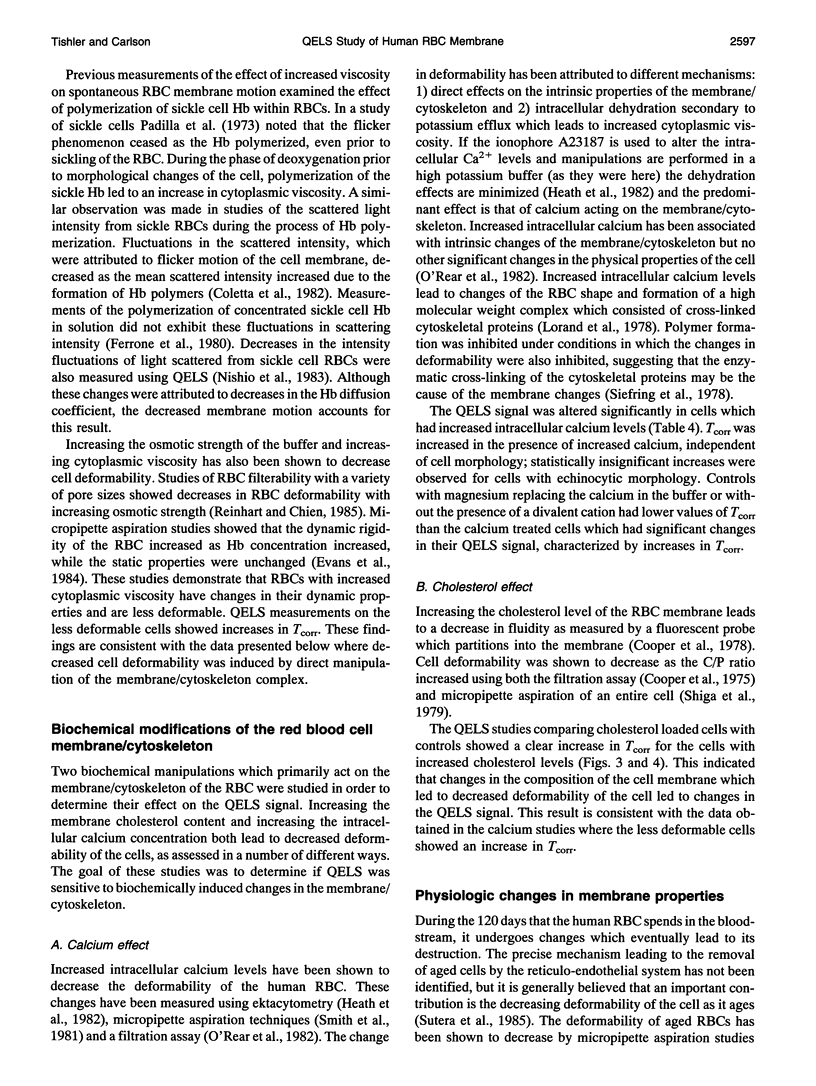
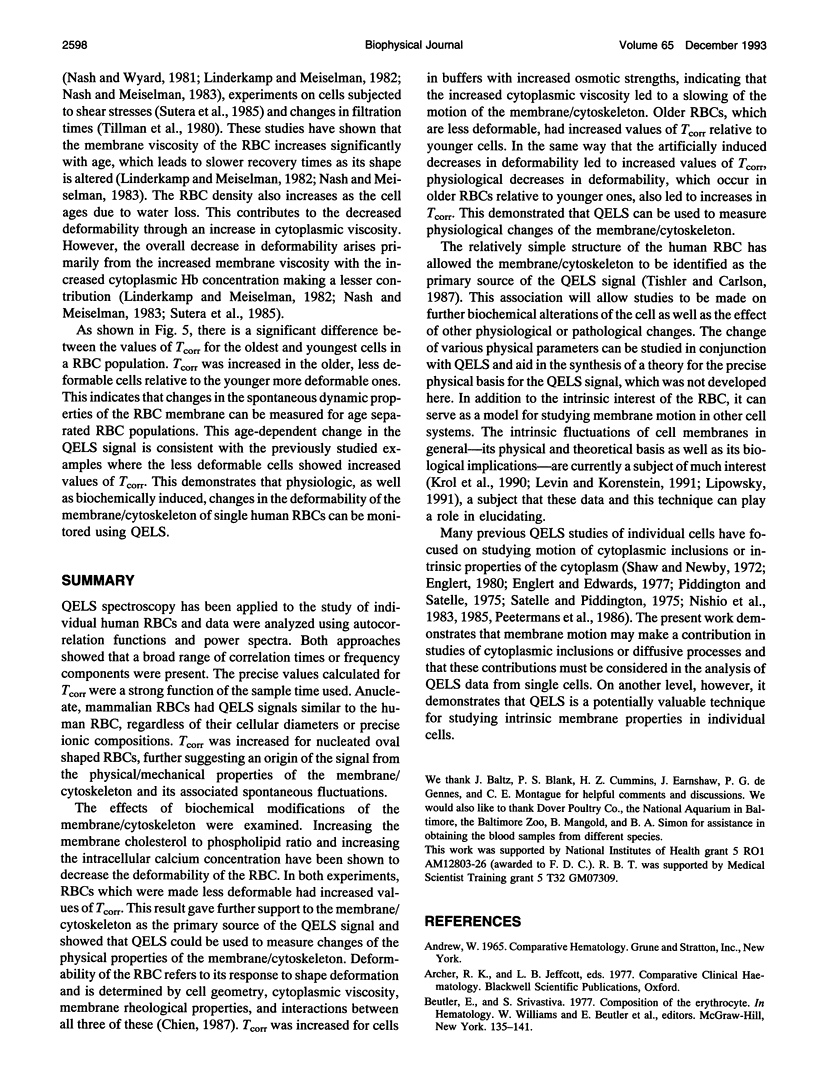
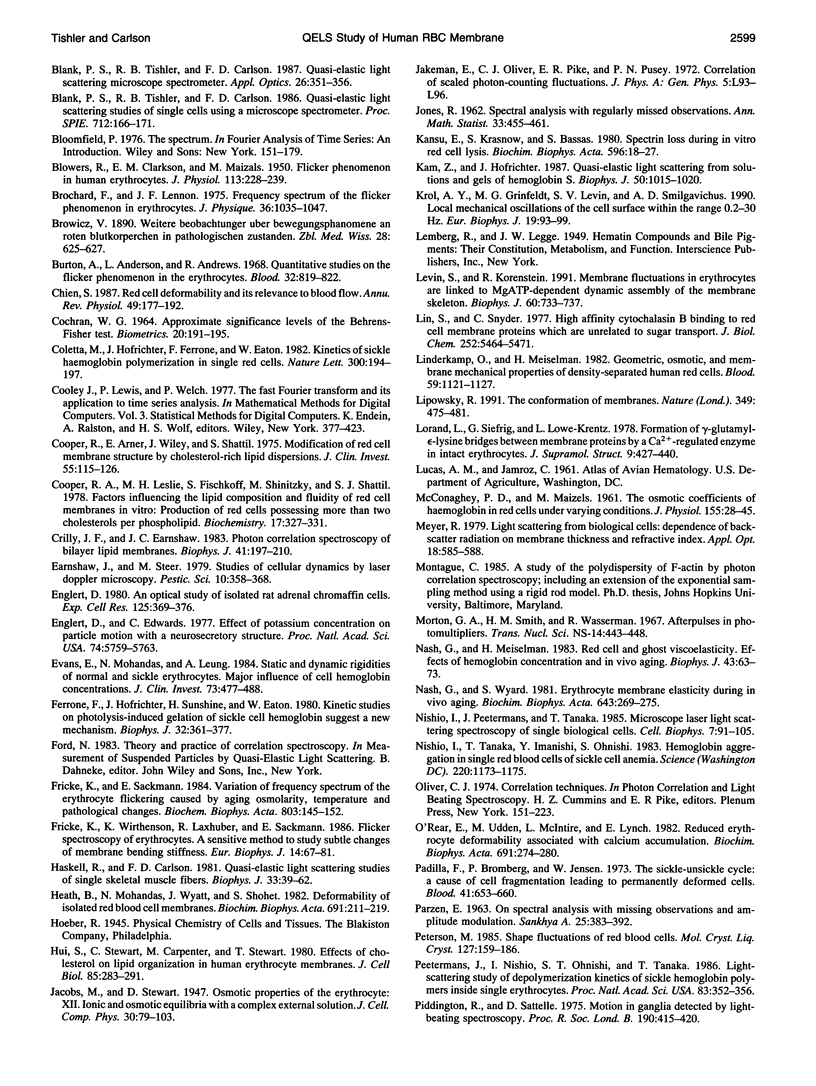
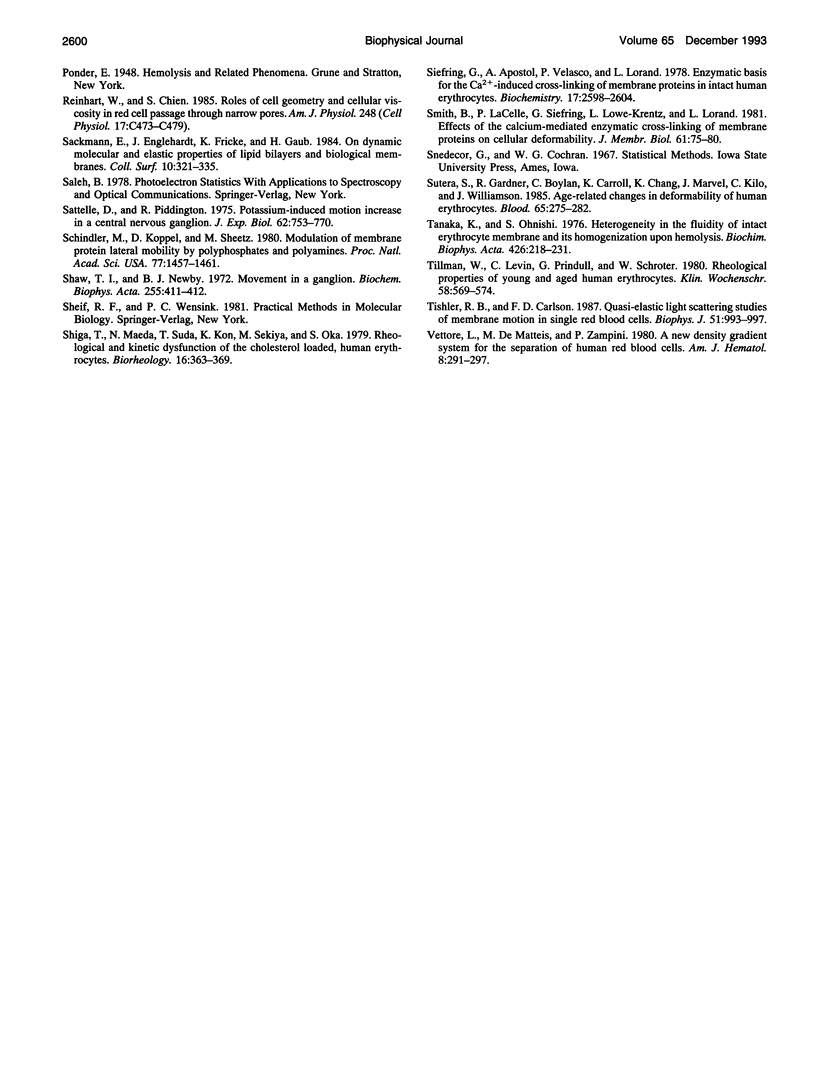
Selected References
These references are in PubMed. This may not be the complete list of references from this article.
- BLOWERS R., CLARKSON E. M., MAIZELS M. Flicker phenomenon in human erythrocytes. J Physiol. 1951 Apr;113(2-3):228–239. doi: 10.1113/jphysiol.1951.sp004568. [DOI] [PMC free article] [PubMed] [Google Scholar]
- Burton A. L., Anderson W. L., Andrews R. V. Quantitative studies on the flicker phenomenon in the erythrocytes. Blood. 1968 Nov;32(5):819–822. [PubMed] [Google Scholar]
- Chien S. Red cell deformability and its relevance to blood flow. Annu Rev Physiol. 1987;49:177–192. doi: 10.1146/annurev.ph.49.030187.001141. [DOI] [PubMed] [Google Scholar]
- Coletta M., Hofrichter J., Ferrone F. A., Eaton W. A. Kinetics of sickle haemoglobin polymerization in single red cells. Nature. 1982 Nov 11;300(5888):194–197. doi: 10.1038/300194a0. [DOI] [PubMed] [Google Scholar]
- Cooper R. A., Arner E. C., Wiley J. S., Shattil S. J. Modification of red cell membrane structure by cholesterol-rich lipid dispersions. A model for the primary spur cell defect. J Clin Invest. 1975 Jan;55(1):115–126. doi: 10.1172/JCI107901. [DOI] [PMC free article] [PubMed] [Google Scholar]
- Cooper R. A., Leslie M. H., Fischkoff S., Shinitzky M., Shattil S. J. Factors influencing the lipid composition and fluidity of red cell membranes in vitro: production of red cells possessing more than two cholesterols per phospholipid. Biochemistry. 1978 Jan 24;17(2):327–331. doi: 10.1021/bi00595a021. [DOI] [PubMed] [Google Scholar]
- Crilly J. F., Earnshaw J. C. Photon correlation spectroscopy of bilayer lipid membranes. Biophys J. 1983 Feb;41(2):197–210. doi: 10.1016/S0006-3495(83)84420-8. [DOI] [PMC free article] [PubMed] [Google Scholar]
- Englert D. F. An optical study of isolated rat adrenal chromaffin cells. Exp Cell Res. 1980 Feb;125(2):369–376. doi: 10.1016/0014-4827(80)90131-7. [DOI] [PubMed] [Google Scholar]
- Englert D., Edwards C. Effect of increased potassium concentrations on particle motion within a neurosecretory structure. Proc Natl Acad Sci U S A. 1977 Dec;74(12):5759–5763. doi: 10.1073/pnas.74.12.5759. [DOI] [PMC free article] [PubMed] [Google Scholar]
- Evans E., Mohandas N., Leung A. Static and dynamic rigidities of normal and sickle erythrocytes. Major influence of cell hemoglobin concentration. J Clin Invest. 1984 Feb;73(2):477–488. doi: 10.1172/JCI111234. [DOI] [PMC free article] [PubMed] [Google Scholar]
- Ferrone F. A., Hofrichter J., Sunshine H. R., Eaton W. A. Kinetic studies on photolysis-induced gelation of sickle cell hemoglobin suggest a new mechanism. Biophys J. 1980 Oct;32(1):361–380. doi: 10.1016/S0006-3495(80)84962-9. [DOI] [PMC free article] [PubMed] [Google Scholar]
- Fricke K., Sackmann E. Variation of frequency spectrum of the erythrocyte flickering caused by aging, osmolarity, temperature and pathological changes. Biochim Biophys Acta. 1984 Mar 23;803(3):145–152. doi: 10.1016/0167-4889(84)90004-1. [DOI] [PubMed] [Google Scholar]
- Fricke K., Wirthensohn K., Laxhuber R., Sackmann E. Flicker spectroscopy of erythrocytes. A sensitive method to study subtle changes of membrane bending stiffness. Eur Biophys J. 1986;14(2):67–81. doi: 10.1007/BF00263063. [DOI] [PubMed] [Google Scholar]
- Haskell R. C., Carlson F. D. Quasi-elastic light-scattering studies of single skeletal muscle fibers. Biophys J. 1981 Jan;33(1):39–62. doi: 10.1016/S0006-3495(81)84871-0. [DOI] [PMC free article] [PubMed] [Google Scholar]
- Heath B. P., Mohandas N., Wyatt J. L., Shohet S. B. Deformability of isolated red blood cell membranes. Biochim Biophys Acta. 1982 Oct 7;691(2):211–219. doi: 10.1016/0005-2736(82)90409-6. [DOI] [PubMed] [Google Scholar]
- Hui S. W., Stewart C. M., Carpenter M. P., Stewart T. P. Effects of cholesterol on lipid organization in human erythrocyte membrane. J Cell Biol. 1980 May;85(2):283–291. doi: 10.1083/jcb.85.2.283. [DOI] [PMC free article] [PubMed] [Google Scholar]
- Kam Z., Hofrichter J. Quasi-elastic laser light scattering from solutions and gels of hemoglobin S. Biophys J. 1986 Nov;50(5):1015–1020. doi: 10.1016/S0006-3495(86)83544-5. [DOI] [PMC free article] [PubMed] [Google Scholar]
- Kansu E., Krasnow S. H., Ballas S. K. Spectrin loss during in vitro red cell lysis. Biochim Biophys Acta. 1980 Feb 15;596(1):18–27. doi: 10.1016/0005-2736(80)90167-4. [DOI] [PubMed] [Google Scholar]
- Krol AYu, Grinfeldt M. G., Levin S. V., Smilgavichus A. D. Local mechanical oscillations of the cell surface within the range 0.2-30 Hz. Eur Biophys J. 1990;19(2):93–99. doi: 10.1007/BF00185092. [DOI] [PubMed] [Google Scholar]
- Levin S., Korenstein R. Membrane fluctuations in erythrocytes are linked to MgATP-dependent dynamic assembly of the membrane skeleton. Biophys J. 1991 Sep;60(3):733–737. doi: 10.1016/S0006-3495(91)82104-X. [DOI] [PMC free article] [PubMed] [Google Scholar]
- Lin S., Snyder C. E., Jr High affinity cytochalasin B binding to red cell membrane proteins which are unrelated to sugar transport. J Biol Chem. 1977 Aug 10;252(15):5464–5471. [PubMed] [Google Scholar]
- Linderkamp O., Meiselman H. J. Geometric, osmotic, and membrane mechanical properties of density-separated human red cells. Blood. 1982 Jun;59(6):1121–1127. [PubMed] [Google Scholar]
- Lipowsky R. The conformation of membranes. Nature. 1991 Feb 7;349(6309):475–481. doi: 10.1038/349475a0. [DOI] [PubMed] [Google Scholar]
- Lorand L., Siefring G. E., Jr, Lowe-Krentz L. Formation of gamma-glutamyl-epsilon-lysine bridges between membrane proteins by a Ca2+-regulated enzyme in intact erythrocytes. J Supramol Struct. 1978;9(3):427–440. doi: 10.1002/jss.400090313. [DOI] [PubMed] [Google Scholar]
- MCCONAGHEY P. D., MAIZELS M. The osmotic coefficients of haemoglobin in red cells under varying conditions. J Physiol. 1961 Jan;155:28–45. doi: 10.1113/jphysiol.1961.sp006611. [DOI] [PMC free article] [PubMed] [Google Scholar]
- Nash G. B., Meiselman H. J. Red cell and ghost viscoelasticity. Effects of hemoglobin concentration and in vivo aging. Biophys J. 1983 Jul;43(1):63–73. doi: 10.1016/S0006-3495(83)84324-0. [DOI] [PMC free article] [PubMed] [Google Scholar]
- Nash G. B., Wyard S. J. Erythrocyte membrane elasticity during in vivo ageing. Biochim Biophys Acta. 1981 May 6;643(2):269–275. doi: 10.1016/0005-2736(81)90072-9. [DOI] [PubMed] [Google Scholar]
- Nishio I., Peetermans J., Tanaka T. Microscope laser light scattering spectroscopy of single biological cells. Cell Biophys. 1985 Jun;7(2):91–105. doi: 10.1007/BF02784485. [DOI] [PubMed] [Google Scholar]
- Nishio I., Tanaka T., Sun S. T., Imanishi Y., Ohnishi S. T. Hemoglobin aggregation in single red blood cells of sickle cell anemia. Science. 1983 Jun 10;220(4602):1173–1175. doi: 10.1126/science.6857241. [DOI] [PubMed] [Google Scholar]
- O'Rear E. A., Udden M. M., McIntire L. V., Lynch E. C. Reduced erythrocyte deformability associated with calcium accumulation. Biochim Biophys Acta. 1982 Oct 7;691(2):274–280. doi: 10.1016/0005-2736(82)90416-3. [DOI] [PubMed] [Google Scholar]
- Padilla F., Bromberg P. A., Jensen W. N. The sickle-unsickle cycle: a cause of cell fragmentation leading to permanently deformed cells. Blood. 1973 May;41(5):653–660. [PubMed] [Google Scholar]
- Peetermans J., Nishio I., Ohnishi S. T., Tanaka T. Light-scattering study of depolymerization kinetics of sickle hemoglobin polymers inside single erythrocytes. Proc Natl Acad Sci U S A. 1986 Jan;83(2):352–356. doi: 10.1073/pnas.83.2.352. [DOI] [PMC free article] [PubMed] [Google Scholar]
- Piddington R. W., Sattelle D. B. Motion in nerve ganglia detected by light-beating spectroscopy. Proc R Soc Lond B Biol Sci. 1975 Sep 23;190(1101):415–420. doi: 10.1098/rspb.1975.0103. [DOI] [PubMed] [Google Scholar]
- Reinhart W. H., Chien S. Roles of cell geometry and cellular viscosity in red cell passage through narrow pores. Am J Physiol. 1985 May;248(5 Pt 1):C473–C479. doi: 10.1152/ajpcell.1985.248.5.C473. [DOI] [PubMed] [Google Scholar]
- Sattelle D. B., Piddington R. W. Potassium-induced motion increase in a central nervous ganglion. J Exp Biol. 1975 Jun;62(3):753–770. doi: 10.1242/jeb.62.3.753. [DOI] [PubMed] [Google Scholar]
- Schindler M., Koppel D. E., Sheetz M. P. Modulation of membrane protein lateral mobility by polyphosphates and polyamines. Proc Natl Acad Sci U S A. 1980 Mar;77(3):1457–1461. doi: 10.1073/pnas.77.3.1457. [DOI] [PMC free article] [PubMed] [Google Scholar]
- Shaw T. I., Newby B. J. Movement in a ganglion. Biochim Biophys Acta. 1972 Jan 17;255(1):411–412. doi: 10.1016/0005-2736(72)90042-9. [DOI] [PubMed] [Google Scholar]
- Shiga T., Maeda N., Suda T., Kon K., Sekiya M., Oka S. Rheological and kinetic dysfunctions of the cholesterol-loaded, human erythrocytes. Biorheology. 1979;16(4-5):363–369. doi: 10.3233/bir-1979-164-511. [DOI] [PubMed] [Google Scholar]
- Siefring G. E., Jr, Apostol A. B., Velasco P. T., Lorand L. Enzymatic basis for the Ca2+-induced cross-linking of membrane proteins in intact human erythrocytes. Biochemistry. 1978 Jun 27;17(13):2598–2604. doi: 10.1021/bi00606a022. [DOI] [PubMed] [Google Scholar]
- Smith B. D., La Celle P. L., Siefring G. E., Jr, Lowe-Krentz L., Lorand L. Effects of the calcium-mediated enzymatic cross-linking of membrane proteins on cellular deformability. J Membr Biol. 1981;61(2):75–80. doi: 10.1007/BF02007633. [DOI] [PubMed] [Google Scholar]
- Sutera S. P., Gardner R. A., Boylan C. W., Carroll G. L., Chang K. C., Marvel J. S., Kilo C., Gonen B., Williamson J. R. Age-related changes in deformability of human erythrocytes. Blood. 1985 Feb;65(2):275–282. [PubMed] [Google Scholar]
- Tanaka K. I., Ohnishi S. Heterogeneity in the fluidity of intact erythrocyte membrane and its homogenization upon hemolysis. Biochim Biophys Acta. 1976 Mar 5;426(2):218–231. doi: 10.1016/0005-2736(76)90333-3. [DOI] [PubMed] [Google Scholar]
- Tillmann W., Levin C., Prindull G., Schröter W. Rheological properties of young and aged human erythrocytes. Klin Wochenschr. 1980 Jun 2;58(11):569–574. doi: 10.1007/BF01477168. [DOI] [PubMed] [Google Scholar]
- Tishler R. B., Carlson F. D. Quasi-elastic light scattering studies of membrane motion in single red blood cells. Biophys J. 1987 Jun;51(6):993–997. doi: 10.1016/S0006-3495(87)83428-8. [DOI] [PMC free article] [PubMed] [Google Scholar]
- Vettore L., De Matteis M. C., Zampini P. A new density gradient system for the separation of human red blood cells. Am J Hematol. 1980;8(3):291–297. doi: 10.1002/ajh.2830080307. [DOI] [PubMed] [Google Scholar]