Abstract
The binding of circulating cells to the vascular wall is a central process in inflammation, metastasis, and therapeutic cell delivery. Previous in vitro studies have identified the adhesion molecules on various circulating cells and the endothelium that govern the process under static conditions. Other studies have attempted to simulate in vivo conditions by subjecting adherent cells to shear stress as they interact with the endothelial cells in vitro. These experiments are generally performed with the cells suspended in Newtonian solutions. However, in vivo conditions are more complex because of the non-Newtonian flow of blood, which is a suspension consisting of 20-40% erythrocytes by volume. The forces imparted by the erythrocytes in the flow can contribute to the process of cell adhesion. A number of experimental and theoretical studies have suggested that the rheology of blood can influence the binding of circulating leukocytes by increasing the normal and axial forces on leukocytes or the frequency of their collision with the vessel wall, but there have been no systematic investigations of these phenomena to date. The present study quantifies the contribution of red blood cells (RBCs) in cell capture and adhesion to endothelial monolayers using a combination of mathematical modeling and in vitro studies. Mathematical modeling of the flow experiments suggested a physical mechanism involving RBC-induced leukocyte dispersion and/or increased normal adhesive contact. Flow chamber studies performed with and without RBCs in the suspending medium showed increases in wall collision and binding frequencies, and a decrease in rolling velocity in the presence of erythrocytes. Increased fluid viscosity alone did not influence the binding frequency, and the differences could not be attributed to large near-wall excesses of the lymphocytes. The results indicate that RBCs aid in the transport and initial engagement of lymphocytes to the vascular wall, modifying the existing paradigm for immune cell surveillance of the vascular endothelium by adding the erythrocyte as an essential contributor to this process.
Full text
PDF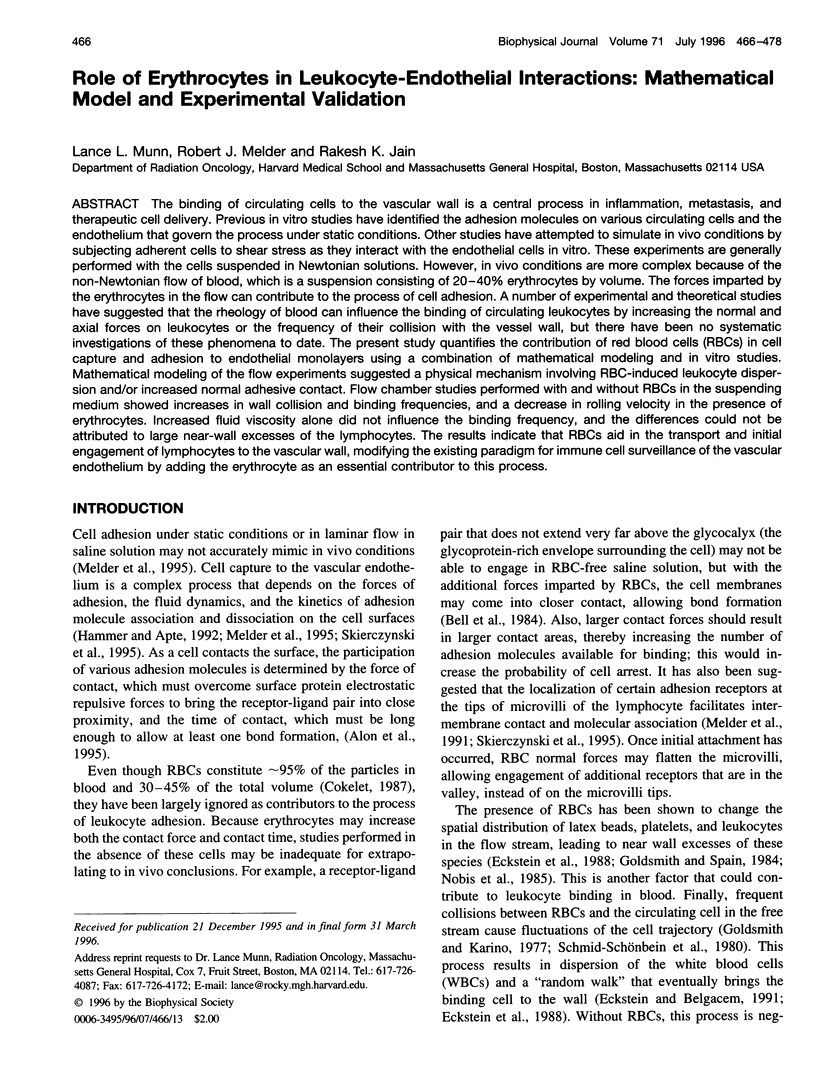
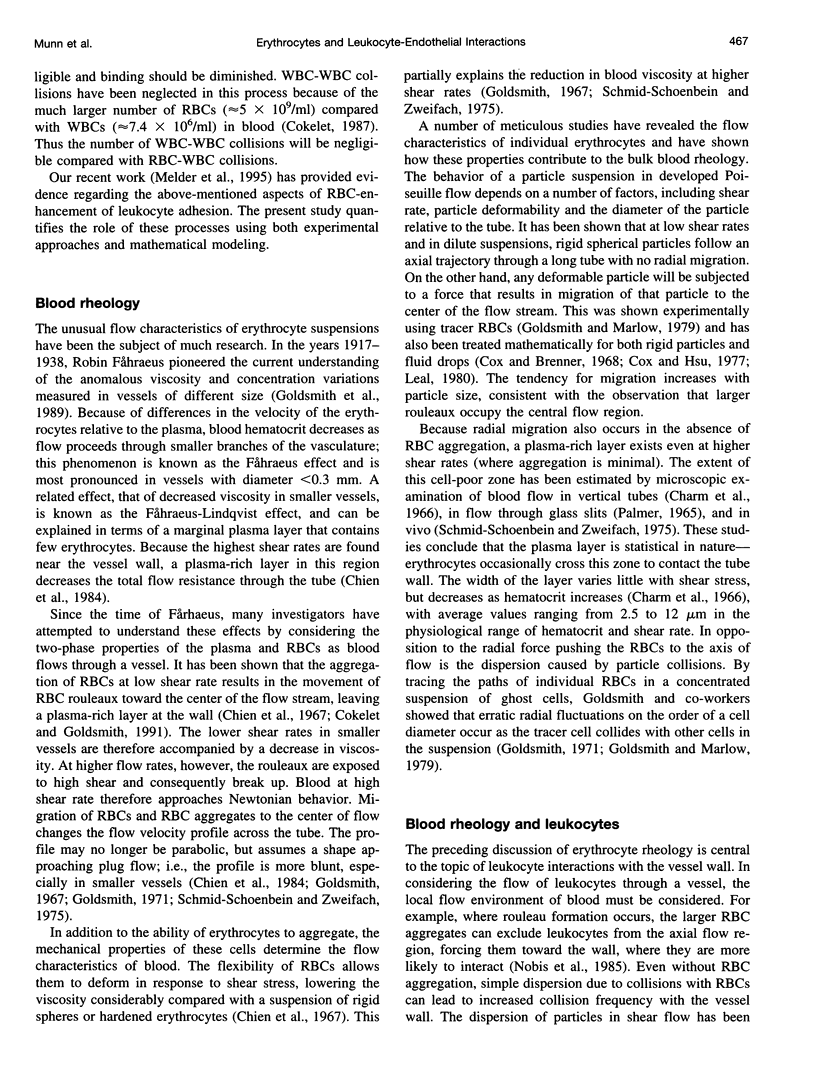
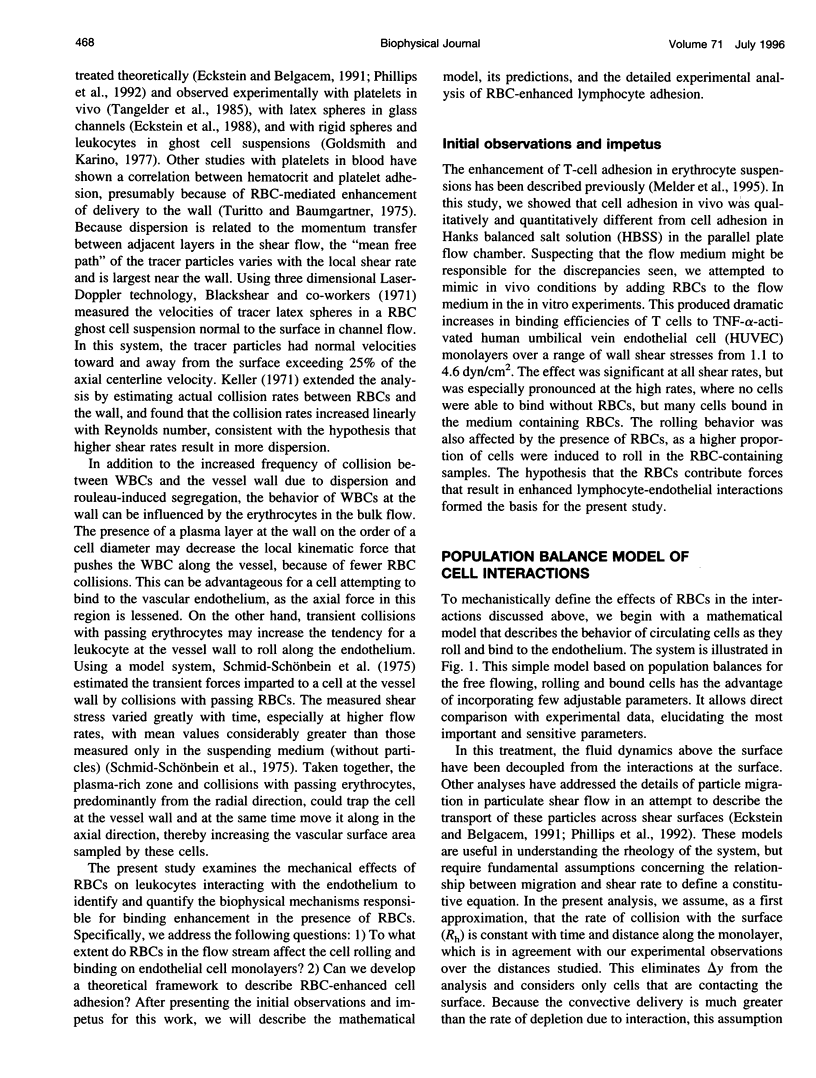
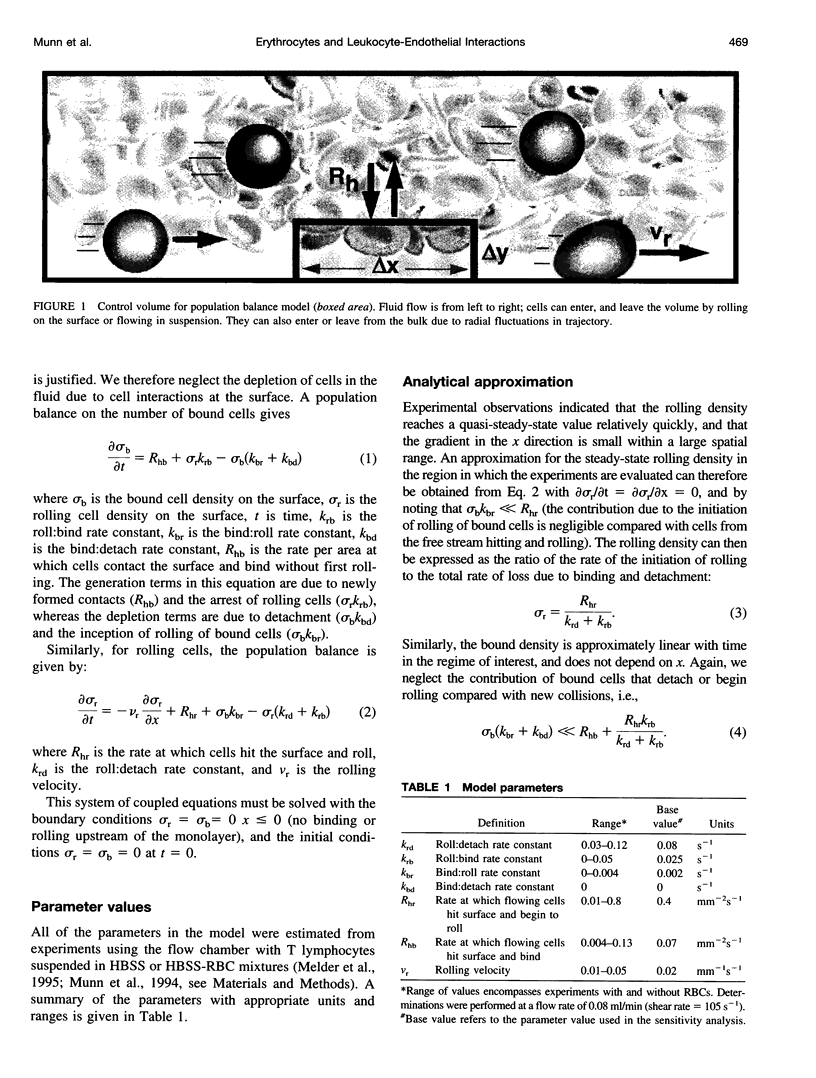
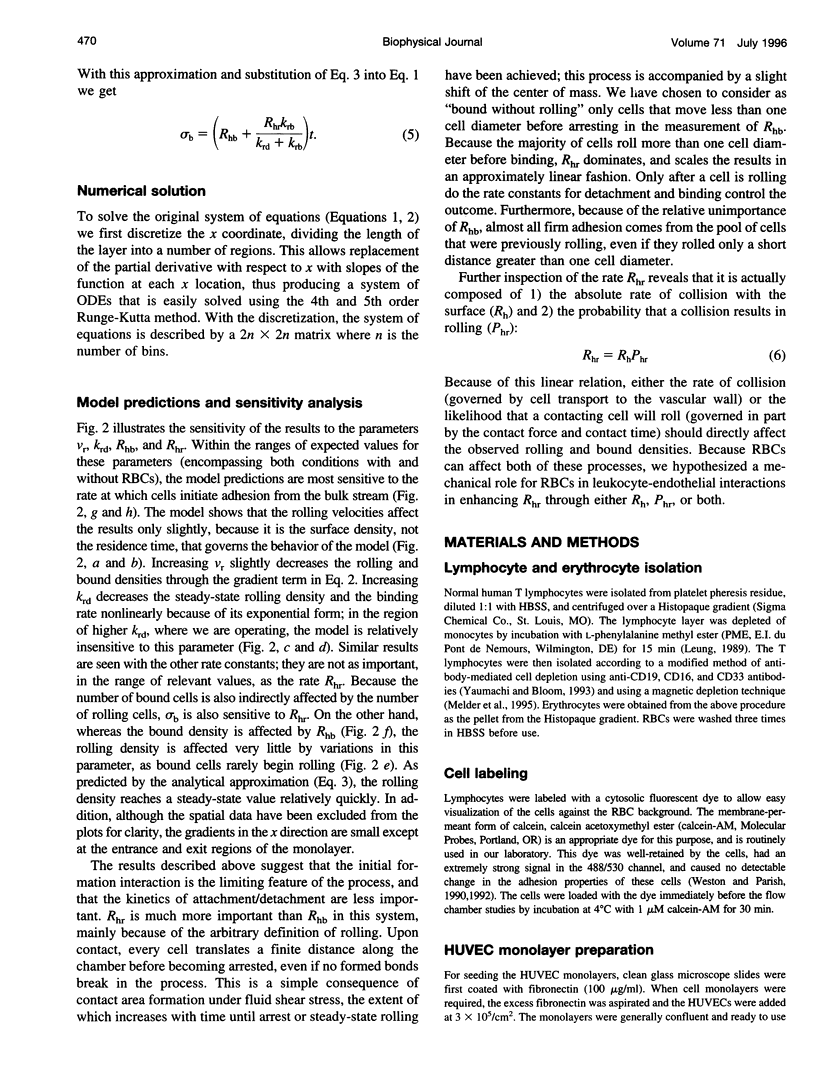
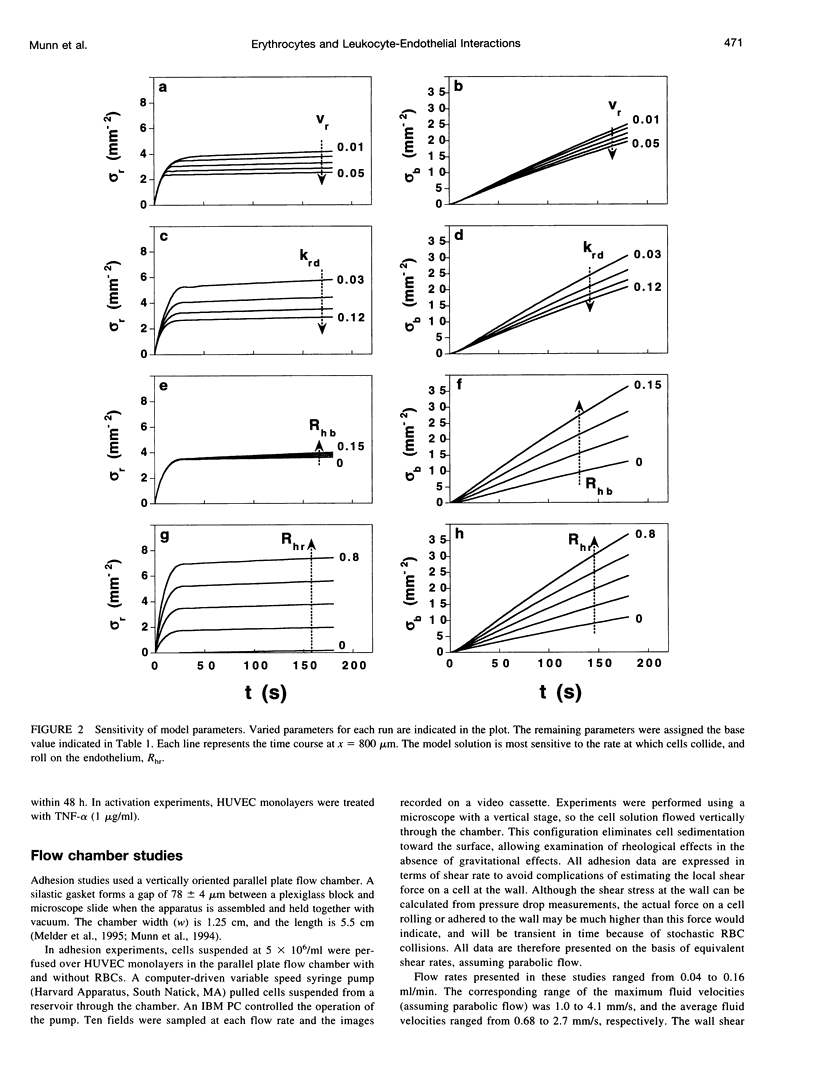
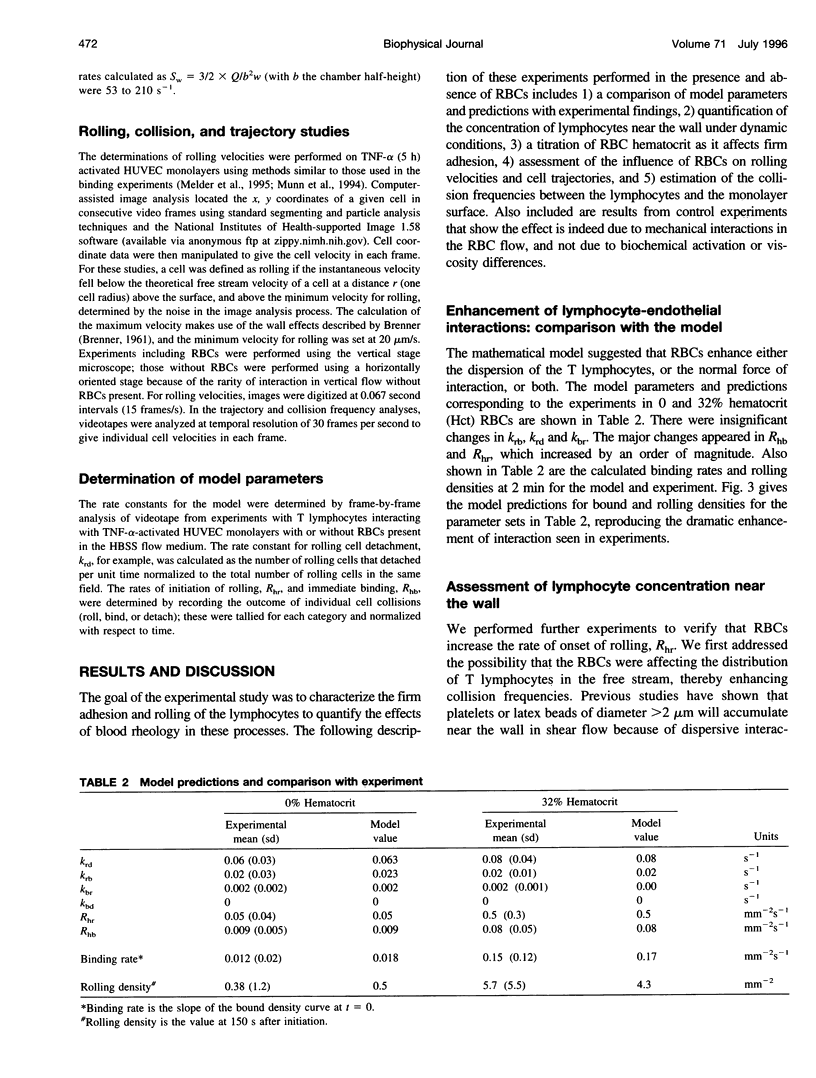
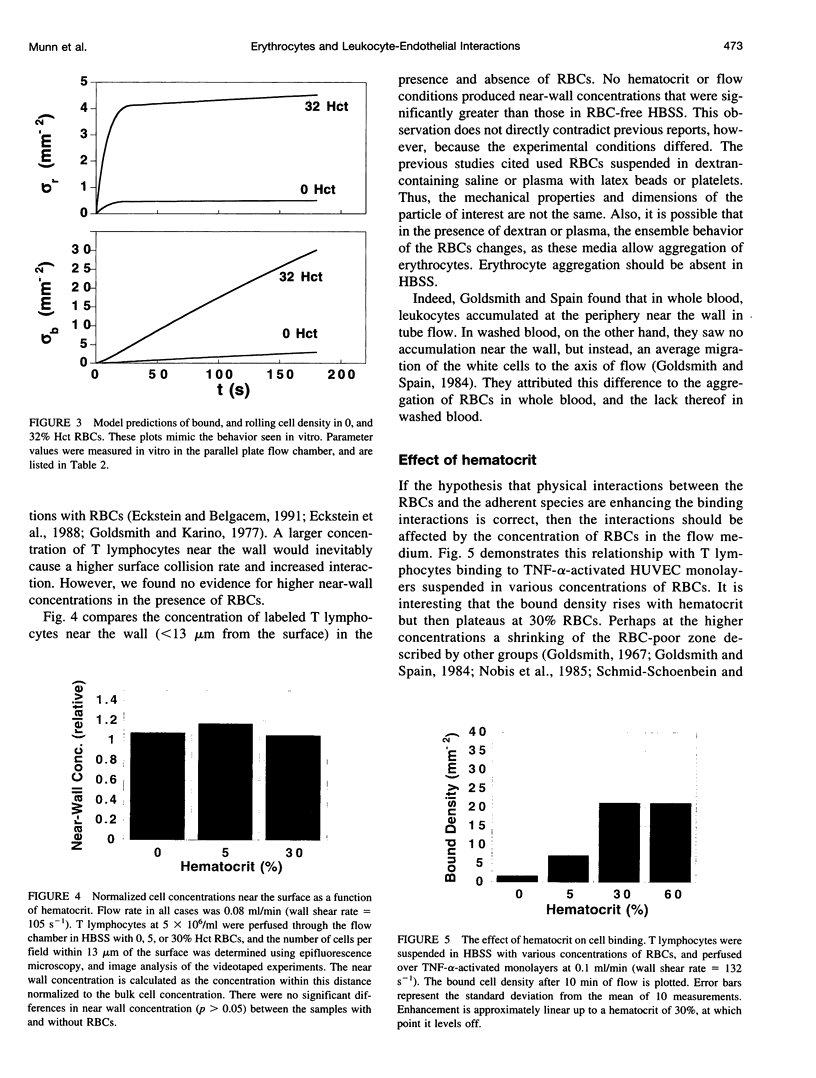
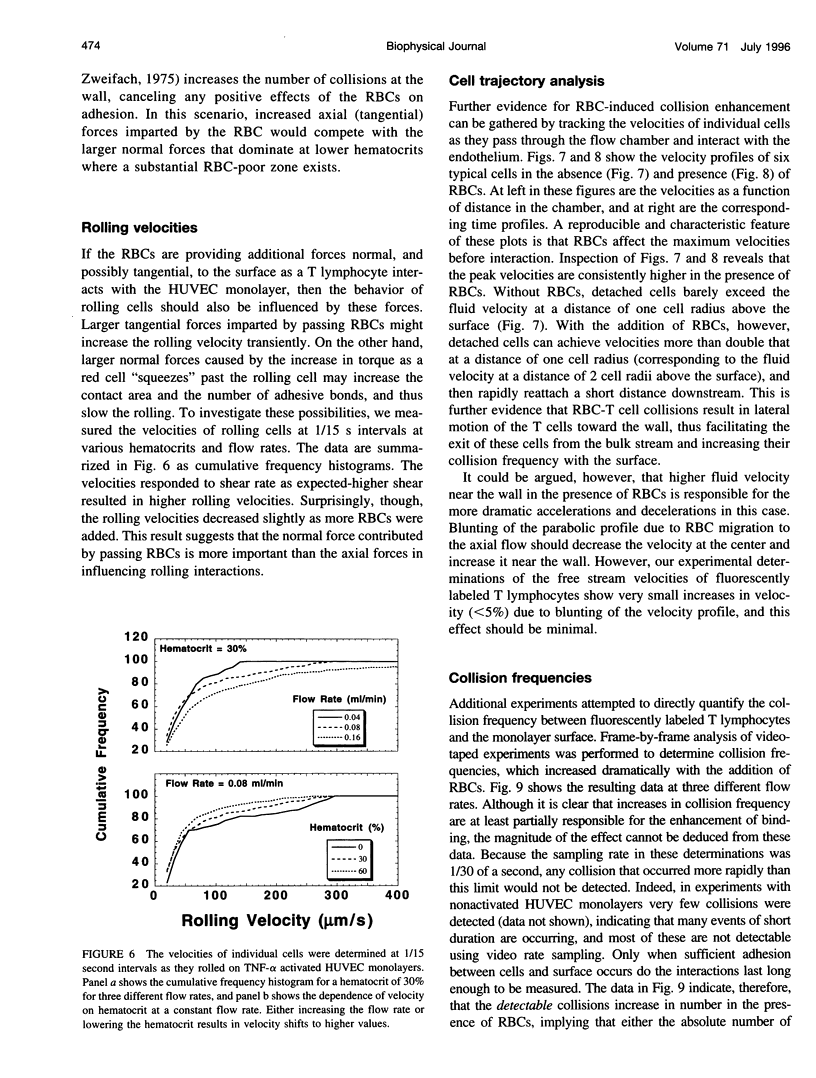
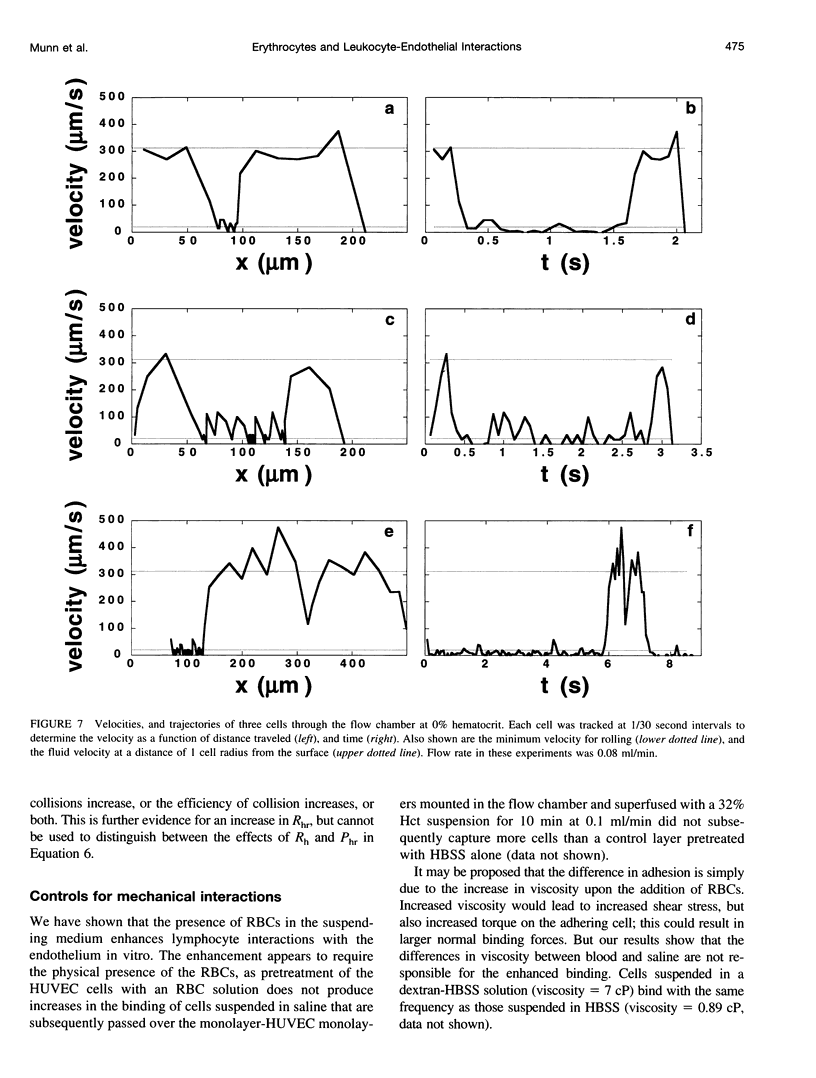
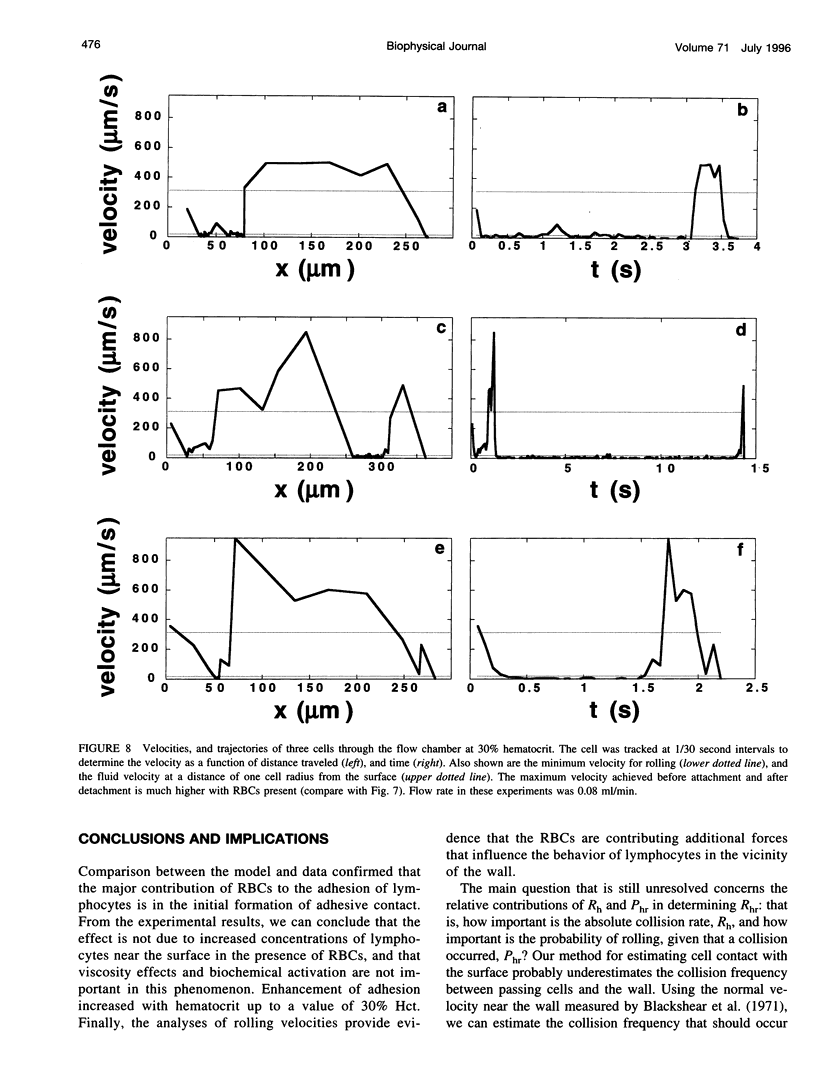
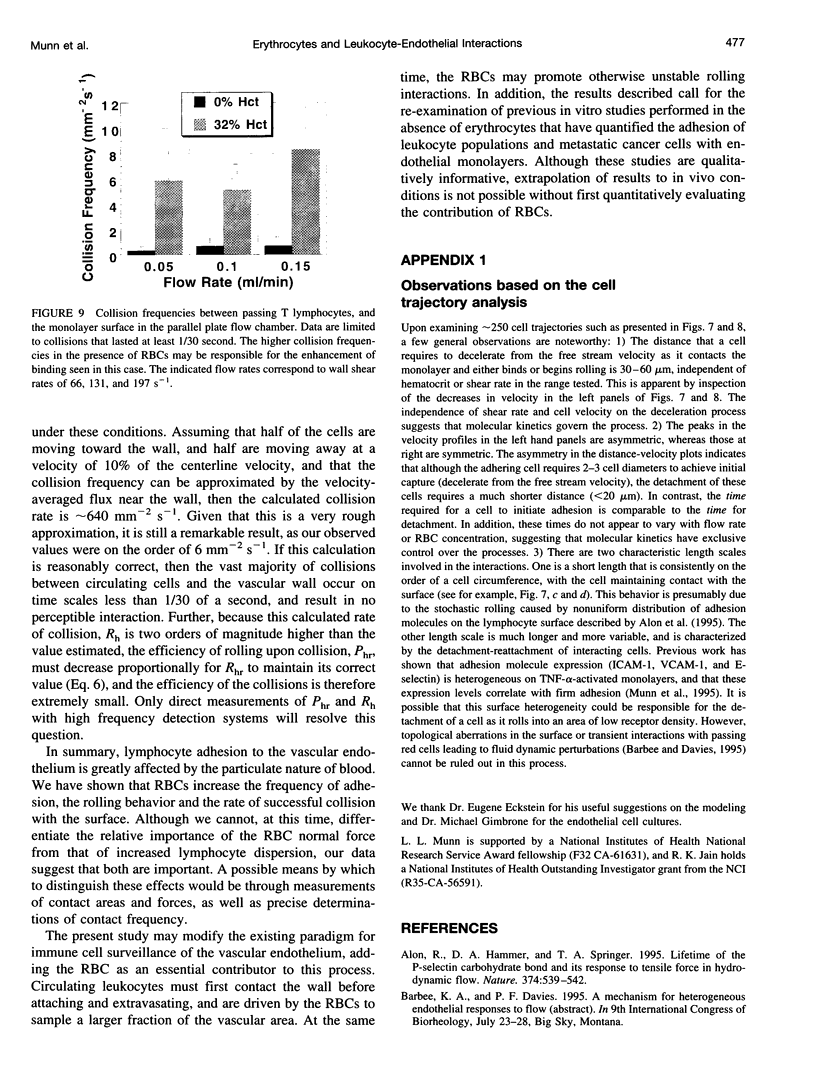
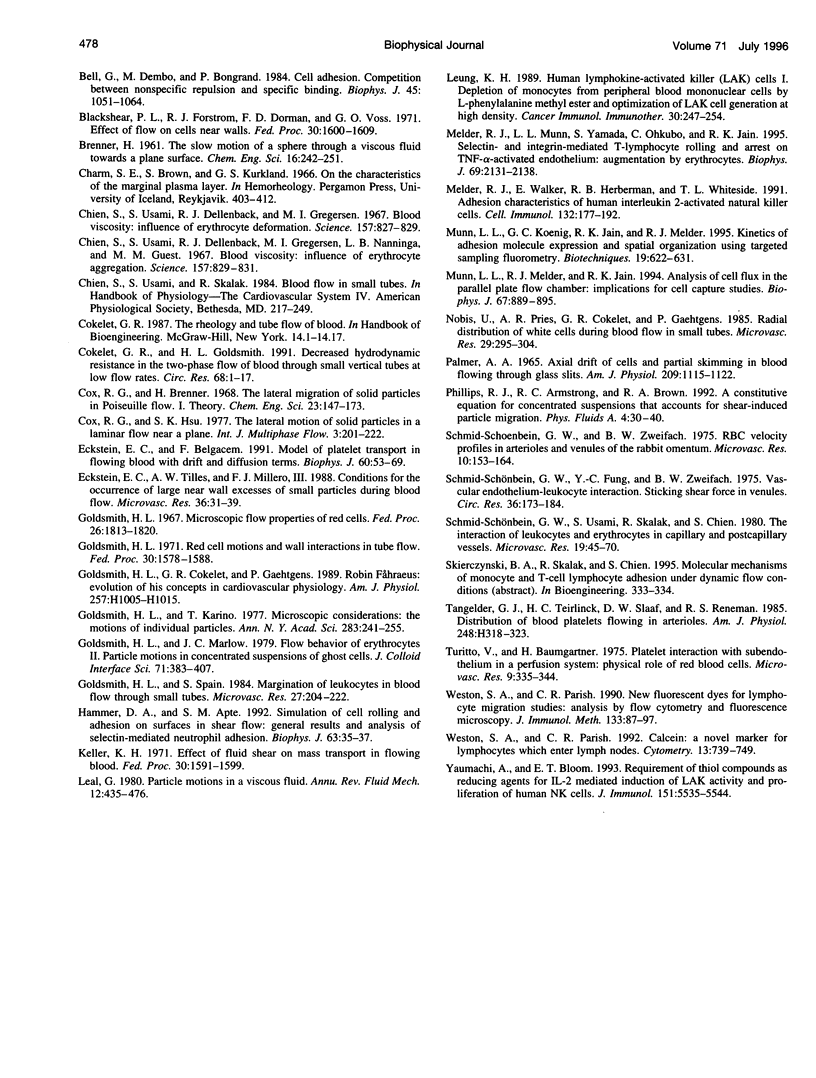
Images in this article
Selected References
These references are in PubMed. This may not be the complete list of references from this article.
- Alon R., Hammer D. A., Springer T. A. Lifetime of the P-selectin-carbohydrate bond and its response to tensile force in hydrodynamic flow. Nature. 1995 Apr 6;374(6522):539–542. doi: 10.1038/374539a0. [DOI] [PubMed] [Google Scholar]
- Bell G. I., Dembo M., Bongrand P. Cell adhesion. Competition between nonspecific repulsion and specific bonding. Biophys J. 1984 Jun;45(6):1051–1064. doi: 10.1016/S0006-3495(84)84252-6. [DOI] [PMC free article] [PubMed] [Google Scholar]
- Blackshear P. L., Jr, Forstrom R. J., Dorman F. D., Voss G. O. Effect of flow on cells near walls. Fed Proc. 1971 Sep-Oct;30(5):1600–1611. [PubMed] [Google Scholar]
- Chien S., Usami S., Dellenback R. J., Gregersen M. I. Blood viscosity: influence of erythrocyte deformation. Science. 1967 Aug 18;157(3790):827–829. doi: 10.1126/science.157.3790.827. [DOI] [PubMed] [Google Scholar]
- Chien S., Usami S., Dellenback R. J., Gregersen M. I., Nanninga L. B., Guest M. M. Blood viscosity: influence of erythrocyte aggregation. Science. 1967 Aug 18;157(3790):829–831. doi: 10.1126/science.157.3790.829. [DOI] [PubMed] [Google Scholar]
- Cokelet G. R., Goldsmith H. L. Decreased hydrodynamic resistance in the two-phase flow of blood through small vertical tubes at low flow rates. Circ Res. 1991 Jan;68(1):1–17. doi: 10.1161/01.res.68.1.1. [DOI] [PubMed] [Google Scholar]
- Eckstein E. C., Belgacem F. Model of platelet transport in flowing blood with drift and diffusion terms. Biophys J. 1991 Jul;60(1):53–69. doi: 10.1016/S0006-3495(91)82030-6. [DOI] [PMC free article] [PubMed] [Google Scholar]
- Eckstein E. C., Tilles A. W., Millero F. J., 3rd Conditions for the occurrence of large near-wall excesses of small particles during blood flow. Microvasc Res. 1988 Jul;36(1):31–39. doi: 10.1016/0026-2862(88)90036-2. [DOI] [PubMed] [Google Scholar]
- Goldsmith H. L., Cokelet G. R., Gaehtgens P. Robin Fåhraeus: evolution of his concepts in cardiovascular physiology. Am J Physiol. 1989 Sep;257(3 Pt 2):H1005–H1015. doi: 10.1152/ajpheart.1989.257.3.H1005. [DOI] [PubMed] [Google Scholar]
- Goldsmith H. L. Microscopic flow properties of red cells. Fed Proc. 1967 Nov-Dec;26(6):1813–1820. [PubMed] [Google Scholar]
- Goldsmith H. L. Red cell motions and wall interactions in tube flow. Fed Proc. 1971 Sep-Oct;30(5):1578–1590. [PubMed] [Google Scholar]
- Goldsmith H. L., Spain S. Margination of leukocytes in blood flow through small tubes. Microvasc Res. 1984 Mar;27(2):204–222. doi: 10.1016/0026-2862(84)90054-2. [DOI] [PubMed] [Google Scholar]
- Hammer D. A., Apte S. M. Simulation of cell rolling and adhesion on surfaces in shear flow: general results and analysis of selectin-mediated neutrophil adhesion. Biophys J. 1992 Jul;63(1):35–57. doi: 10.1016/S0006-3495(92)81577-1. [DOI] [PMC free article] [PubMed] [Google Scholar]
- Keller K. H. Effect of fluid shear on mass transport in flowing blood. Fed Proc. 1971 Sep-Oct;30(5):1591–1599. [PubMed] [Google Scholar]
- Leung K. H. Human lymphokine-activated killer (LAK) cells. I. Depletion of monocytes from peripheral blood mononuclear cells by L-phenylalanine methyl ester: an optimization of LAK cell generation at high cell density. Cancer Immunol Immunother. 1989;30(4):247–253. doi: 10.1007/BF01665012. [DOI] [PMC free article] [PubMed] [Google Scholar]
- Melder R. J., Munn L. L., Yamada S., Ohkubo C., Jain R. K. Selectin- and integrin-mediated T-lymphocyte rolling and arrest on TNF-alpha-activated endothelium: augmentation by erythrocytes. Biophys J. 1995 Nov;69(5):2131–2138. doi: 10.1016/S0006-3495(95)80087-1. [DOI] [PMC free article] [PubMed] [Google Scholar]
- Melder R. J., Walker E., Herberman R. B., Whiteside T. L. Adhesion characteristics of human interleukin 2-activated natural killer cells. Cell Immunol. 1991 Jan;132(1):177–192. doi: 10.1016/0008-8749(91)90017-6. [DOI] [PubMed] [Google Scholar]
- Munn L. L., Koenig G. C., Jain R. K., Melder R. J. Kinetics of adhesion molecule expression and spatial organization using targeted sampling fluorometry. Biotechniques. 1995 Oct;19(4):622-6, 628-31. [PubMed] [Google Scholar]
- Munn L. L., Melder R. J., Jain R. K. Analysis of cell flux in the parallel plate flow chamber: implications for cell capture studies. Biophys J. 1994 Aug;67(2):889–895. doi: 10.1016/S0006-3495(94)80550-8. [DOI] [PMC free article] [PubMed] [Google Scholar]
- Nobis U., Pries A. R., Cokelet G. R., Gaehtgens P. Radial distribution of white cells during blood flow in small tubes. Microvasc Res. 1985 May;29(3):295–304. doi: 10.1016/0026-2862(85)90020-2. [DOI] [PubMed] [Google Scholar]
- Palmer A. A. Axial drift of cells and partial plasma skimming in blood flowing through glass slits. Am J Physiol. 1965 Dec;209(6):1115–1122. doi: 10.1152/ajplegacy.1965.209.6.1115. [DOI] [PubMed] [Google Scholar]
- Schmid-Schoenbein G. W., Fung Y. C., Zweifach B. W. Vascular endothelium-leukocyte interaction; sticking shear force in venules. Circ Res. 1975 Jan;36(1):173–184. doi: 10.1161/01.res.36.1.173. [DOI] [PubMed] [Google Scholar]
- Schmid-Schoenbein G. W., Zweifach B. W. RBC velocity profiles in arterioles and venules of the rabbit omentum. Microvasc Res. 1975 Sep;10(2):153–164. doi: 10.1016/0026-2862(75)90003-5. [DOI] [PubMed] [Google Scholar]
- Schmid-Schönbein G. W., Usami S., Skalak R., Chien S. The interaction of leukocytes and erythrocytes in capillary and postcapillary vessels. Microvasc Res. 1980 Jan;19(1):45–70. doi: 10.1016/0026-2862(80)90083-7. [DOI] [PubMed] [Google Scholar]
- Tangelder G. J., Teirlinck H. C., Slaaf D. W., Reneman R. S. Distribution of blood platelets flowing in arterioles. Am J Physiol. 1985 Mar;248(3 Pt 2):H318–H323. doi: 10.1152/ajpheart.1985.248.3.H318. [DOI] [PubMed] [Google Scholar]
- Turitto V. T., Baumgartner H. R. Platelet interaction with subendothelium in a perfusion system: physical role of red blood cells. Microvasc Res. 1975 May;9(3):335–344. doi: 10.1016/0026-2862(75)90070-9. [DOI] [PubMed] [Google Scholar]
- Weston S. A., Parish C. R. Calcein: a novel marker for lymphocytes which enter lymph nodes. Cytometry. 1992;13(7):739–749. doi: 10.1002/cyto.990130710. [DOI] [PubMed] [Google Scholar]
- Weston S. A., Parish C. R. New fluorescent dyes for lymphocyte migration studies. Analysis by flow cytometry and fluorescence microscopy. J Immunol Methods. 1990 Oct 4;133(1):87–97. doi: 10.1016/0022-1759(90)90322-m. [DOI] [PubMed] [Google Scholar]
- Yamauchi A., Bloom E. T. Requirement of thiol compounds as reducing agents for IL-2-mediated induction of LAK activity and proliferation of human NK cells. J Immunol. 1993 Nov 15;151(10):5535–5544. [PubMed] [Google Scholar]