Abstract
The mechanism of interaction between cytochrome c and a solid-supported planar phosphatidylcholine membrane containing varying amounts of cardiolipin (0-20 mol%) has been studied over a wide range of protein concentrations (0-450 microM) and ionic strength conditions (10-150 mM), by direct measurement of protein binding using surface plasmon resonance (SPR) spectroscopy. The results demonstrate that cytochrome c binds to such phospholipid membranes in two distinct phases characterized by very different (approximately one order of magnitude) affinity constants. The second phase is dependent upon the prior occurrence of the first binding process. Although the binding affinities for both modes of binding are highly sensitive to both the cardiolipin concentration and the ionic strength of the buffer solution, indicating that electrostatic forces are involved in these processes, binding cannot be reversed by salt addition or by dilution. Furthermore, the final saturation levels of adsorbed protein are independent of ionic strength and cardiolipin concentration. These observations suggest that binding involves more than a simple electrostatic interaction. Invariance in the shapes of the SPR spectra indicates that no major structural transitions occur in the proteolipid membrane due to cytochrome c binding, i.e., the bilayer character of the lipid phase appears to be preserved during these interactions. Based on these results, a model of the lipid membrane-cytochrome c interaction is proposed that involves varying degrees of protein unfolding and subsequent binding to the membrane interior via hydrophobic forces.
Full text
PDF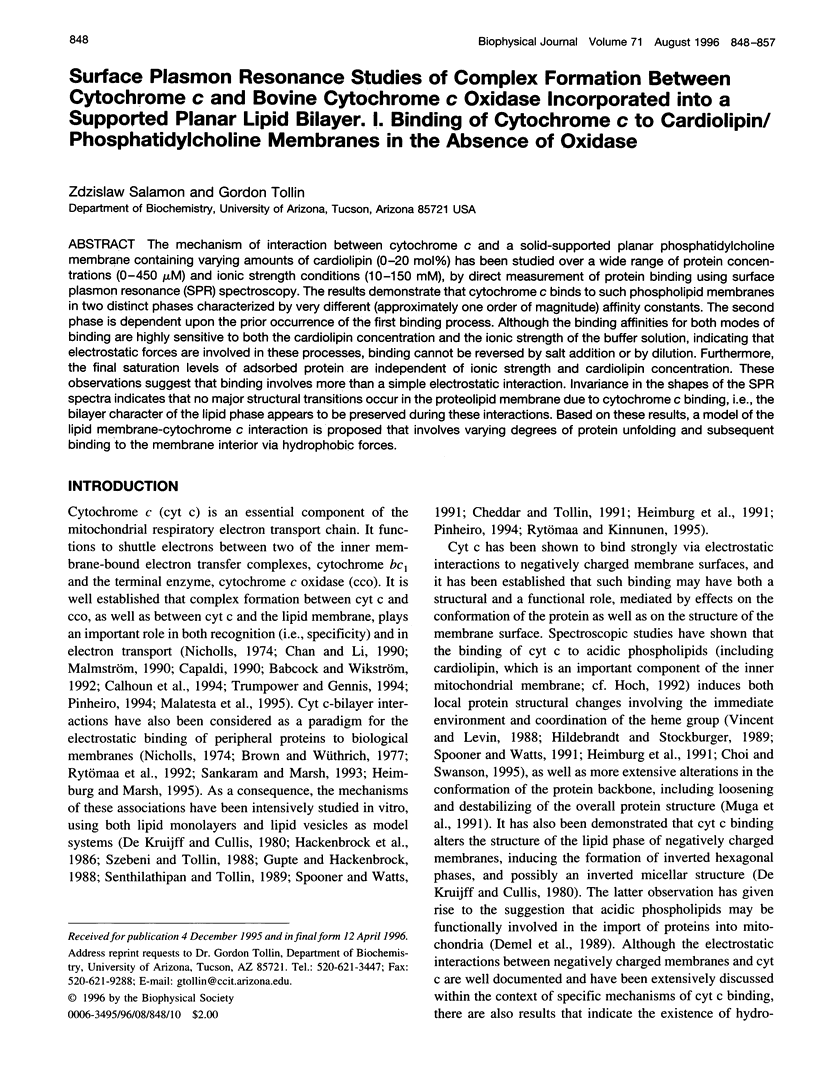
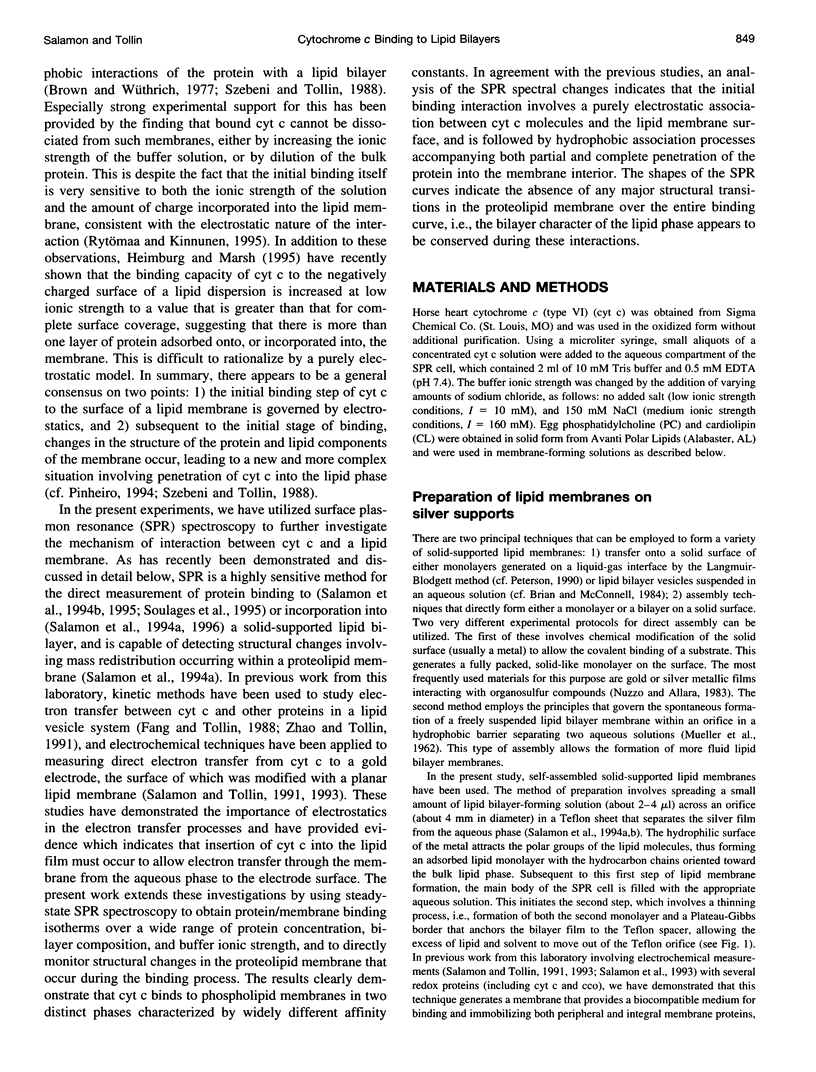
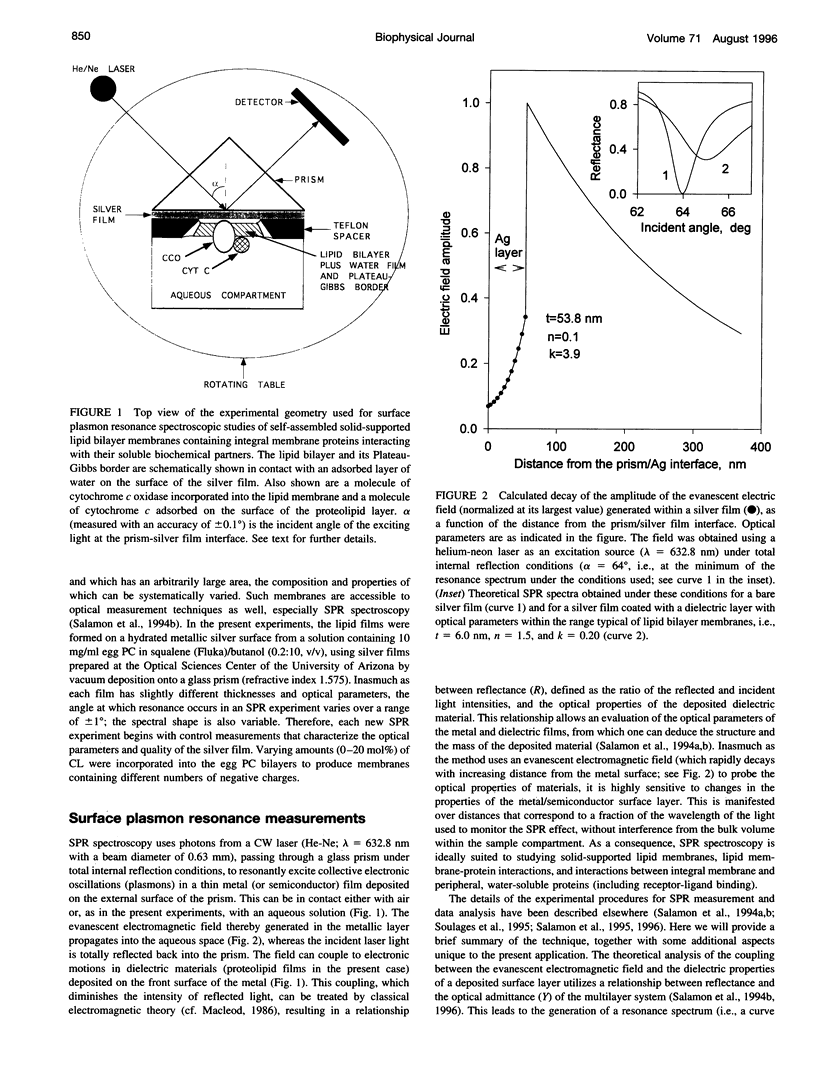
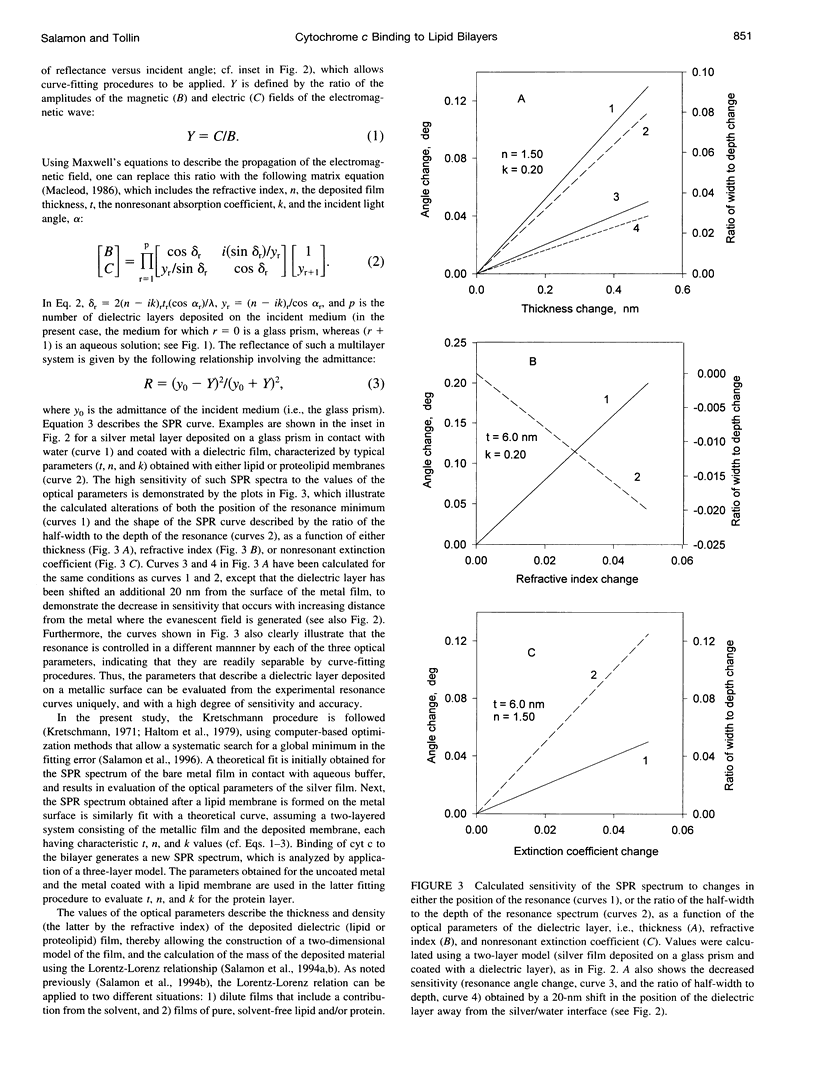
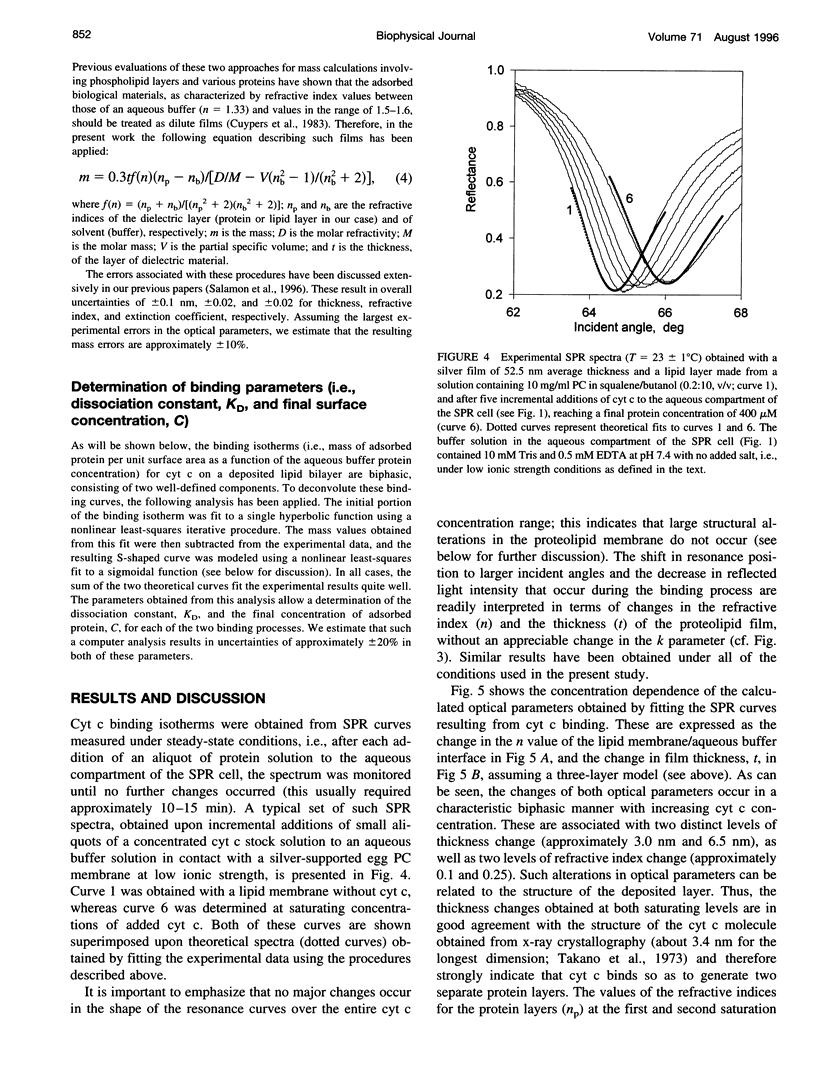
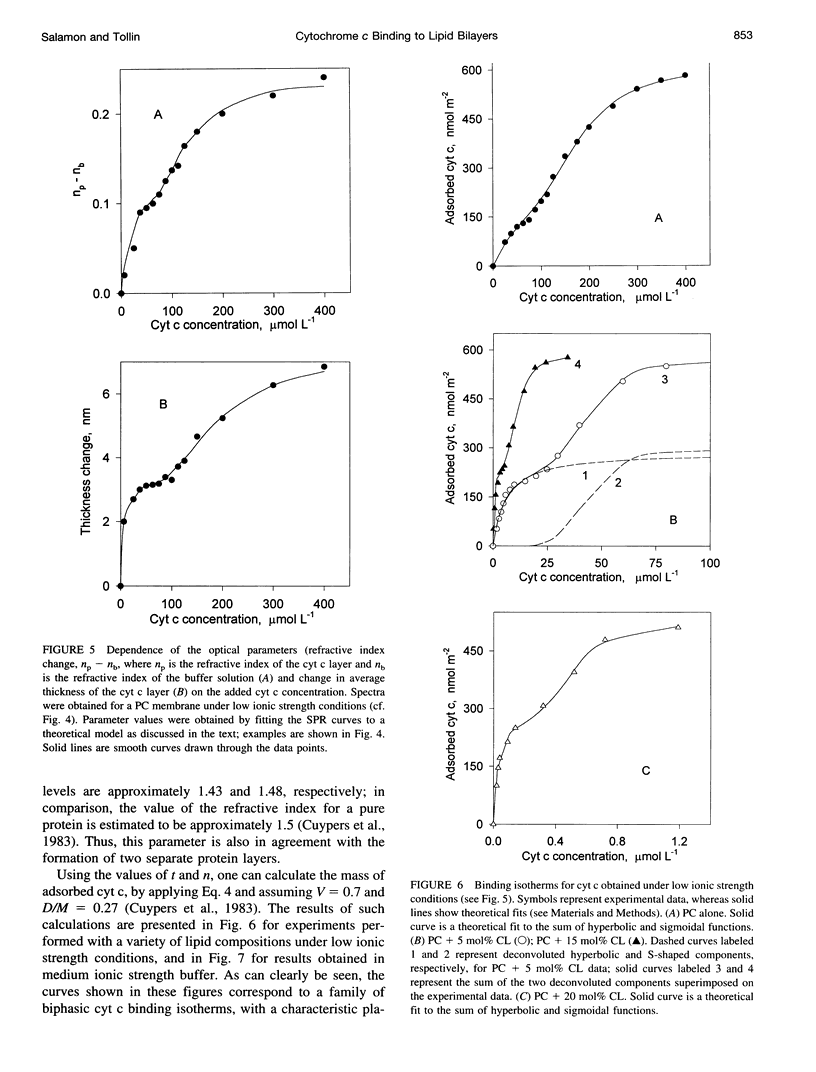
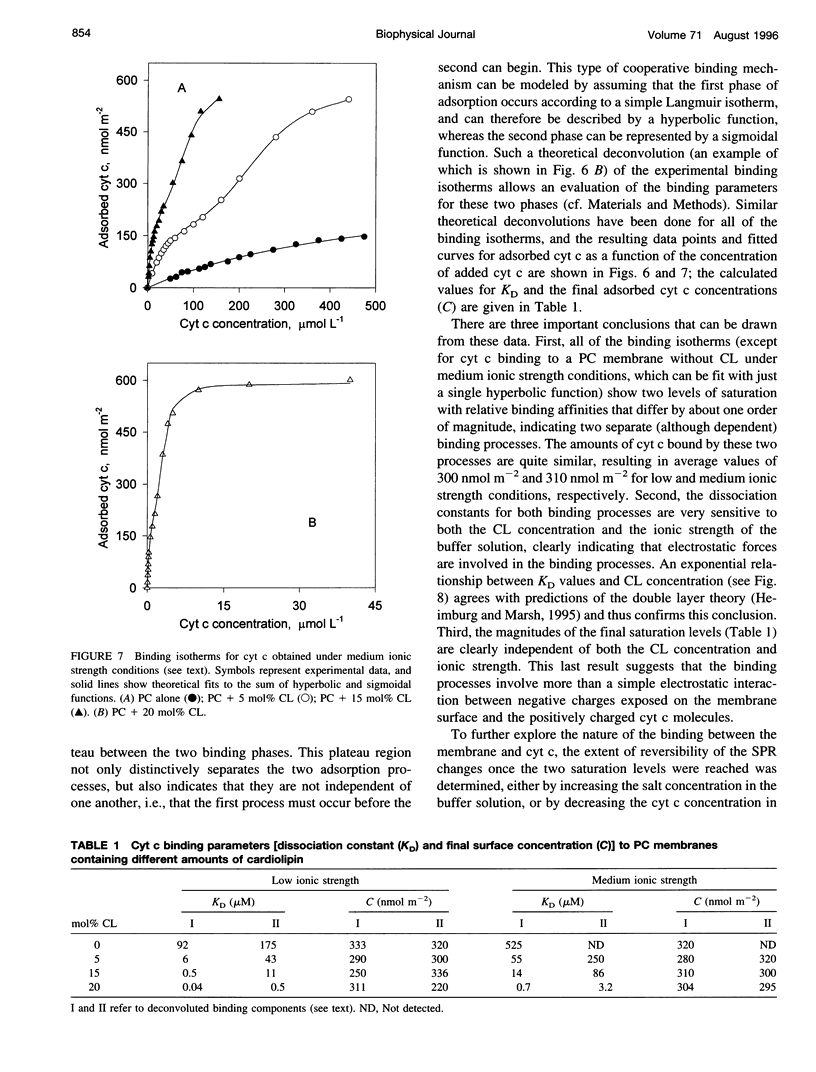
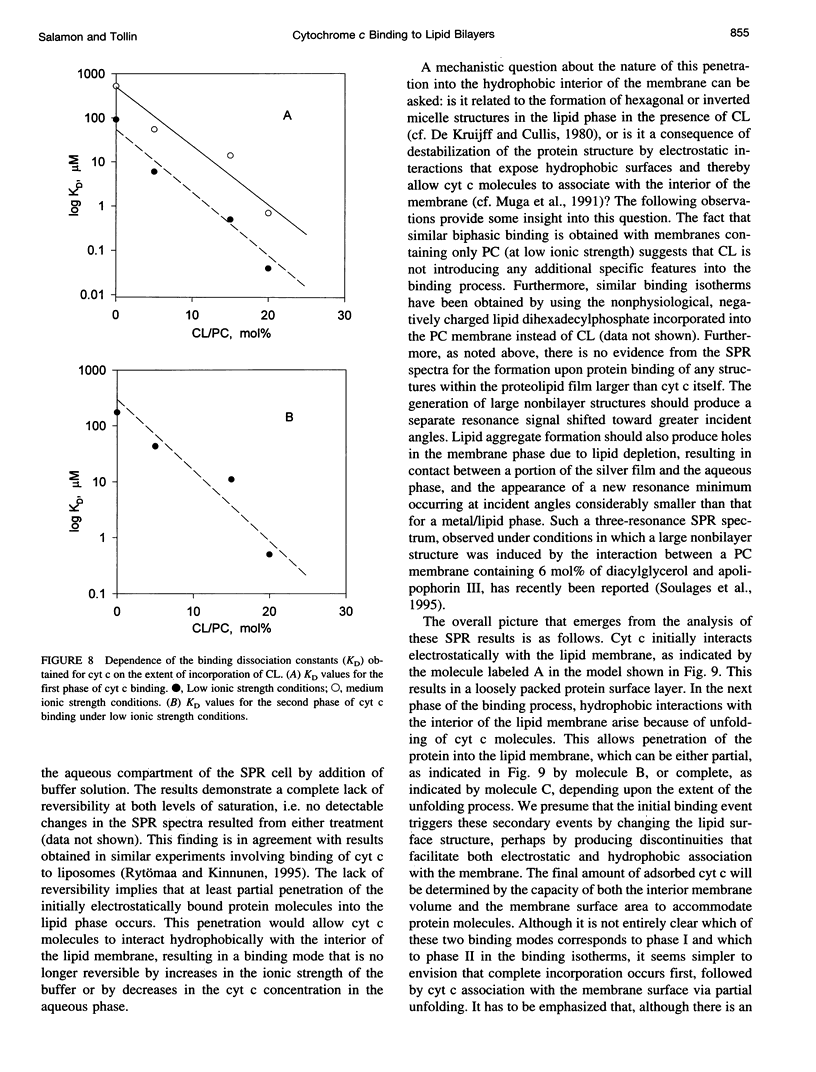
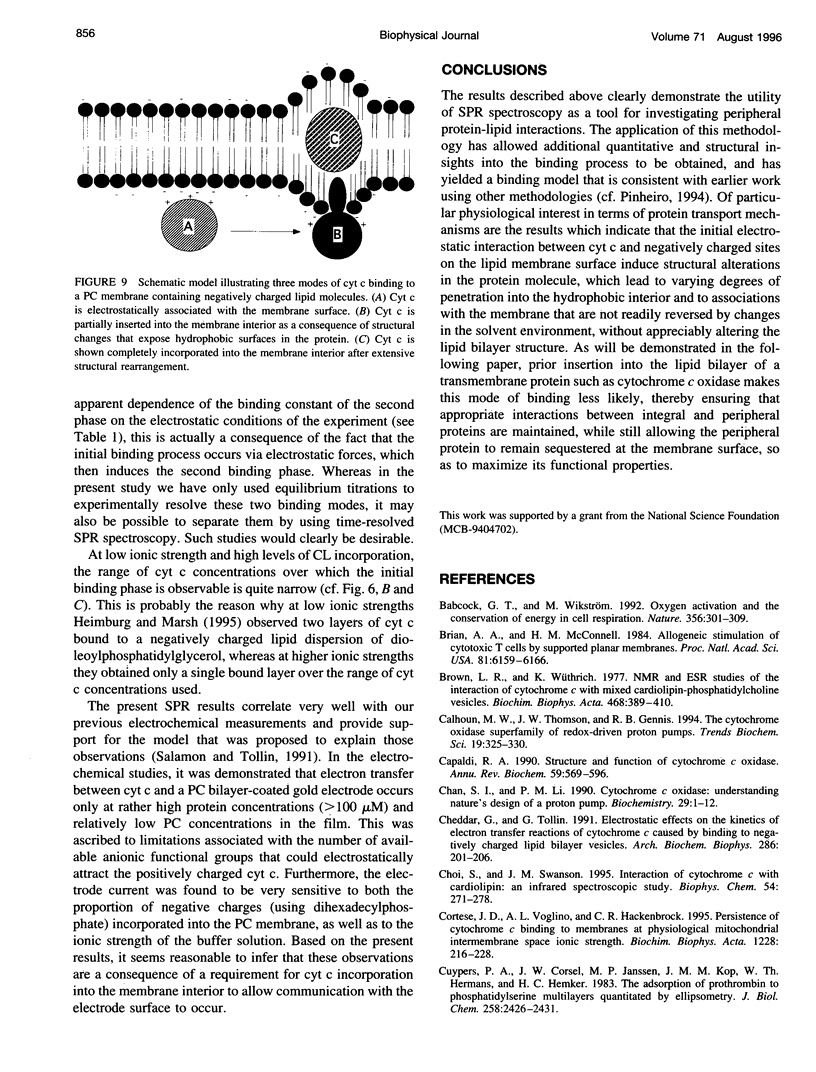
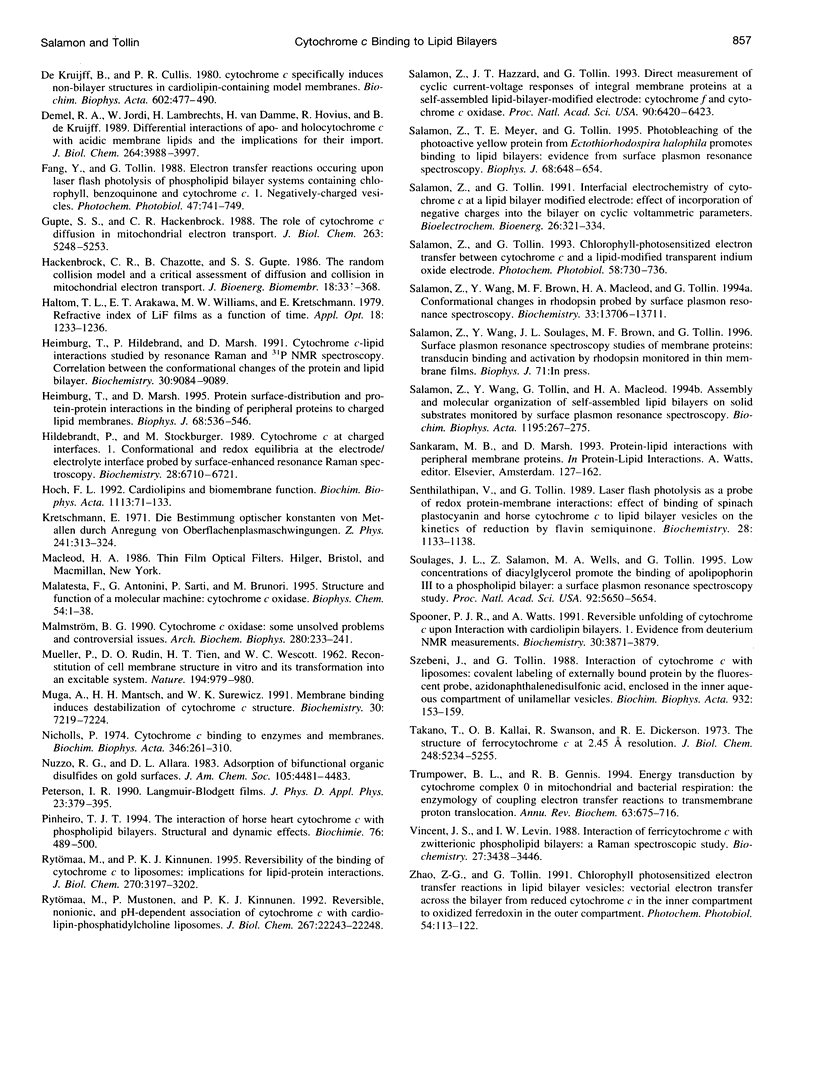
Images in this article
Selected References
These references are in PubMed. This may not be the complete list of references from this article.
- Babcock G. T., Wikström M. Oxygen activation and the conservation of energy in cell respiration. Nature. 1992 Mar 26;356(6367):301–309. doi: 10.1038/356301a0. [DOI] [PubMed] [Google Scholar]
- Brian A. A., McConnell H. M. Allogeneic stimulation of cytotoxic T cells by supported planar membranes. Proc Natl Acad Sci U S A. 1984 Oct;81(19):6159–6163. doi: 10.1073/pnas.81.19.6159. [DOI] [PMC free article] [PubMed] [Google Scholar]
- Brown L. R., Wüthrich K. NMR and ESR studies of the interactions of cytochrome c with mixed cardiolipin-phosphatidylcholine vesicles. Biochim Biophys Acta. 1977 Aug 1;468(3):389–410. doi: 10.1016/0005-2736(77)90290-5. [DOI] [PubMed] [Google Scholar]
- Calhoun M. W., Thomas J. W., Gennis R. B. The cytochrome oxidase superfamily of redox-driven proton pumps. Trends Biochem Sci. 1994 Aug;19(8):325–330. doi: 10.1016/0968-0004(94)90071-x. [DOI] [PubMed] [Google Scholar]
- Capaldi R. A. Structure and function of cytochrome c oxidase. Annu Rev Biochem. 1990;59:569–596. doi: 10.1146/annurev.bi.59.070190.003033. [DOI] [PubMed] [Google Scholar]
- Chan S. I., Li P. M. Cytochrome c oxidase: understanding nature's design of a proton pump. Biochemistry. 1990 Jan 9;29(1):1–12. doi: 10.1021/bi00453a001. [DOI] [PubMed] [Google Scholar]
- Cheddar G., Tollin G. Electrostatic effects on the kinetics of electron transfer reactions of cytochrome c caused by binding to negatively charged lipid bilayer vesicles. Arch Biochem Biophys. 1991 Apr;286(1):201–206. doi: 10.1016/0003-9861(91)90028-h. [DOI] [PubMed] [Google Scholar]
- Choi S., Swanson J. M. Interaction of cytochrome c with cardiolipin: an infrared spectroscopic study. Biophys Chem. 1995 May;54(3):271–278. doi: 10.1016/0301-4622(94)00151-9. [DOI] [PubMed] [Google Scholar]
- Cortese J. D., Voglino A. L., Hackenbrock C. R. Persistence of cytochrome c binding to membranes at physiological mitochondrial intermembrane space ionic strength. Biochim Biophys Acta. 1995 Mar 14;1228(2-3):216–228. doi: 10.1016/0005-2728(94)00178-8. [DOI] [PubMed] [Google Scholar]
- Cuypers P. A., Corsel J. W., Janssen M. P., Kop J. M., Hermens W. T., Hemker H. C. The adsorption of prothrombin to phosphatidylserine multilayers quantitated by ellipsometry. J Biol Chem. 1983 Feb 25;258(4):2426–2431. [PubMed] [Google Scholar]
- Demel R. A., Jordi W., Lambrechts H., van Damme H., Hovius R., de Kruijff B. Differential interactions of apo- and holocytochrome c with acidic membrane lipids in model systems and the implications for their import into mitochondria. J Biol Chem. 1989 Mar 5;264(7):3988–3997. [PubMed] [Google Scholar]
- Gupte S. S., Hackenbrock C. R. The role of cytochrome c diffusion in mitochondrial electron transport. J Biol Chem. 1988 Apr 15;263(11):5248–5253. [PubMed] [Google Scholar]
- Hackenbrock C. R., Chazotte B., Gupte S. S. The random collision model and a critical assessment of diffusion and collision in mitochondrial electron transport. J Bioenerg Biomembr. 1986 Oct;18(5):331–368. doi: 10.1007/BF00743010. [DOI] [PubMed] [Google Scholar]
- Heimburg T., Hildebrandt P., Marsh D. Cytochrome c-lipid interactions studied by resonance Raman and 31P NMR spectroscopy. Correlation between the conformational changes of the protein and the lipid bilayer. Biochemistry. 1991 Sep 17;30(37):9084–9089. doi: 10.1021/bi00101a025. [DOI] [PubMed] [Google Scholar]
- Heimburg T., Marsh D. Protein surface-distribution and protein-protein interactions in the binding of peripheral proteins to charged lipid membranes. Biophys J. 1995 Feb;68(2):536–546. doi: 10.1016/S0006-3495(95)80215-8. [DOI] [PMC free article] [PubMed] [Google Scholar]
- Hildebrandt P., Stockburger M. Cytochrome c at charged interfaces. 1. Conformational and redox equilibria at the electrode/electrolyte interface probed by surface-enhanced resonance Raman spectroscopy. Biochemistry. 1989 Aug 8;28(16):6710–6721. doi: 10.1021/bi00442a026. [DOI] [PubMed] [Google Scholar]
- Hoch F. L. Cardiolipins and biomembrane function. Biochim Biophys Acta. 1992 Mar 26;1113(1):71–133. doi: 10.1016/0304-4157(92)90035-9. [DOI] [PubMed] [Google Scholar]
- MUELLER P., RUDIN D. O., TIEN H. T., WESCOTT W. C. Reconstitution of cell membrane structure in vitro and its transformation into an excitable system. Nature. 1962 Jun 9;194:979–980. doi: 10.1038/194979a0. [DOI] [PubMed] [Google Scholar]
- Malatesta F., Antonini G., Sarti P., Brunori M. Structure and function of a molecular machine: cytochrome c oxidase. Biophys Chem. 1995 Mar;54(1):1–33. doi: 10.1016/0301-4622(94)00117-3. [DOI] [PubMed] [Google Scholar]
- Malmström B. G. Cytochrome oxidase: some unsolved problems and controversial issues. Arch Biochem Biophys. 1990 Aug 1;280(2):233–241. doi: 10.1016/0003-9861(90)90325-s. [DOI] [PubMed] [Google Scholar]
- Muga A., Mantsch H. H., Surewicz W. K. Membrane binding induces destabilization of cytochrome c structure. Biochemistry. 1991 Jul 23;30(29):7219–7224. doi: 10.1021/bi00243a025. [DOI] [PubMed] [Google Scholar]
- Nicholls P. Cytochrome c binding to enzymes and membranes. Biochim Biophys Acta. 1974 Dec 30;346(3-4):261–310. doi: 10.1016/0304-4173(74)90003-2. [DOI] [PubMed] [Google Scholar]
- Pinheiro T. J. The interaction of horse heart cytochrome c with phospholipid bilayers. Structural and dynamic effects. Biochimie. 1994;76(6):489–500. doi: 10.1016/0300-9084(94)90173-2. [DOI] [PubMed] [Google Scholar]
- Rytömaa M., Kinnunen P. K. Reversibility of the binding of cytochrome c to liposomes. Implications for lipid-protein interactions. J Biol Chem. 1995 Feb 17;270(7):3197–3202. doi: 10.1074/jbc.270.7.3197. [DOI] [PubMed] [Google Scholar]
- Rytömaa M., Mustonen P., Kinnunen P. K. Reversible, nonionic, and pH-dependent association of cytochrome c with cardiolipin-phosphatidylcholine liposomes. J Biol Chem. 1992 Nov 5;267(31):22243–22248. [PubMed] [Google Scholar]
- Salamon Z., Hazzard J. T., Tollin G. Direct measurement of cyclic current-voltage responses of integral membrane proteins at a self-assembled lipid-bilayer-modified electrode: cytochrome f and cytochrome c oxidase. Proc Natl Acad Sci U S A. 1993 Jul 15;90(14):6420–6423. doi: 10.1073/pnas.90.14.6420. [DOI] [PMC free article] [PubMed] [Google Scholar]
- Salamon Z., Meyer T. E., Tollin G. Photobleaching of the photoactive yellow protein from Ectothiorhodospira halophila promotes binding to lipid bilayers: evidence from surface plasmon resonance spectroscopy. Biophys J. 1995 Feb;68(2):648–654. doi: 10.1016/S0006-3495(95)80225-0. [DOI] [PMC free article] [PubMed] [Google Scholar]
- Salamon Z., Wang Y., Brown M. F., Macleod H. A., Tollin G. Conformational changes in rhodopsin probed by surface plasmon resonance spectroscopy. Biochemistry. 1994 Nov 22;33(46):13706–13711. doi: 10.1021/bi00250a022. [DOI] [PubMed] [Google Scholar]
- Salamon Z., Wang Y., Tollin G., Macleod H. A. Assembly and molecular organization of self-assembled lipid bilayers on solid substrates monitored by surface plasmon resonance spectroscopy. Biochim Biophys Acta. 1994 Nov 2;1195(2):267–275. doi: 10.1016/0005-2736(94)90266-6. [DOI] [PubMed] [Google Scholar]
- Senthilathipan V., Tollin G. Laser flash photolysis as a probe of redox protein-membrane interactions: effect of binding of spinach plastocyanin and horse cytochrome c to lipid bilayer vesicles on the kinetics of reduction by flavin semiquinone. Biochemistry. 1989 Feb 7;28(3):1133–1138. doi: 10.1021/bi00429a030. [DOI] [PubMed] [Google Scholar]
- Soulages J. L., Salamon Z., Wells M. A., Tollin G. Low concentrations of diacylglycerol promote the binding of apolipophorin III to a phospholipid bilayer: a surface plasmon resonance spectroscopy study. Proc Natl Acad Sci U S A. 1995 Jun 6;92(12):5650–5654. doi: 10.1073/pnas.92.12.5650. [DOI] [PMC free article] [PubMed] [Google Scholar]
- Spooner P. J., Watts A. Reversible unfolding of cytochrome c upon interaction with cardiolipin bilayers. 1. Evidence from deuterium NMR measurements. Biochemistry. 1991 Apr 23;30(16):3871–3879. doi: 10.1021/bi00230a010. [DOI] [PubMed] [Google Scholar]
- Szebeni J., Tollin G. Interaction of cytochrome c with liposomes: covalent labeling of externally bound protein by the fluorescent probe, azidonaphthalenedisulfonic acid, enclosed in the inner aqueous compartment of unilamellar vesicles. Biochim Biophys Acta. 1988 Jan 20;932(1):153–159. doi: 10.1016/0005-2728(88)90150-8. [DOI] [PubMed] [Google Scholar]
- Takano T., Kallai O. B., Swanson R., Dickerson R. E. The structure of ferrocytochrome c at 2.45 A resolution. J Biol Chem. 1973 Aug 10;248(15):5234–5255. [PubMed] [Google Scholar]
- Trumpower B. L., Gennis R. B. Energy transduction by cytochrome complexes in mitochondrial and bacterial respiration: the enzymology of coupling electron transfer reactions to transmembrane proton translocation. Annu Rev Biochem. 1994;63:675–716. doi: 10.1146/annurev.bi.63.070194.003331. [DOI] [PubMed] [Google Scholar]
- Vincent J. S., Levin I. W. Interaction of ferricytochrome c with zwitterionic phospholipid bilayers: a Raman spectroscopic study. Biochemistry. 1988 May 3;27(9):3438–3446. doi: 10.1021/bi00409a047. [DOI] [PubMed] [Google Scholar]
- de Kruijff B., Cullis P. R. Cytochrome c specifically induces non-bilayer structures in cardiolipin-containing model membranes. Biochim Biophys Acta. 1980 Nov 18;602(3):477–490. doi: 10.1016/0005-2736(80)90327-2. [DOI] [PubMed] [Google Scholar]