Abstract
Bolaform lipid vesicles were used to study the effect of physical constraints on membrane fusion. In these vesicles the membrane is organized in a single monolayer, because of the presence of covalent bonds in its middle plane. Therefore, the formation of fusion intermediates is subject to higher energy barriers and greater geometrical constraints than is usual in bilayer membranes. Bolaform lipids were extracted from the thermophilic archaeon Sulfolobus solfataricus. These lipids can be divided into two classes, the monosubstituted molecules, in which one of the polar heads is glycerol, and the bisubstituted molecules, endowed with two complex polar heads. The fusion process in vesicles composed of different mixtures of monosubstituted/bisubstituted molecules was studied by means of fluorescence techniques. Ca2+ or poly(ethylene glycol) was employed as a fusogenic agent. We found that fusion of such constrained membranes is still possible, provided that molecules able to mediate a structural rearrangement of the membrane are present. This condition is fulfilled by monosubstituted molecules, which are able to partition the glycerol headgroup in the apolar moiety. In addition, the presence of traces (approximately 5%) of the monopolar compound diphytanylglycerol is an important factor for fusion to occur. On the contrary, vesicles formed by bisubstituted molecules are unable to fuse, irrespective of the fusogen employed.
Full text
PDF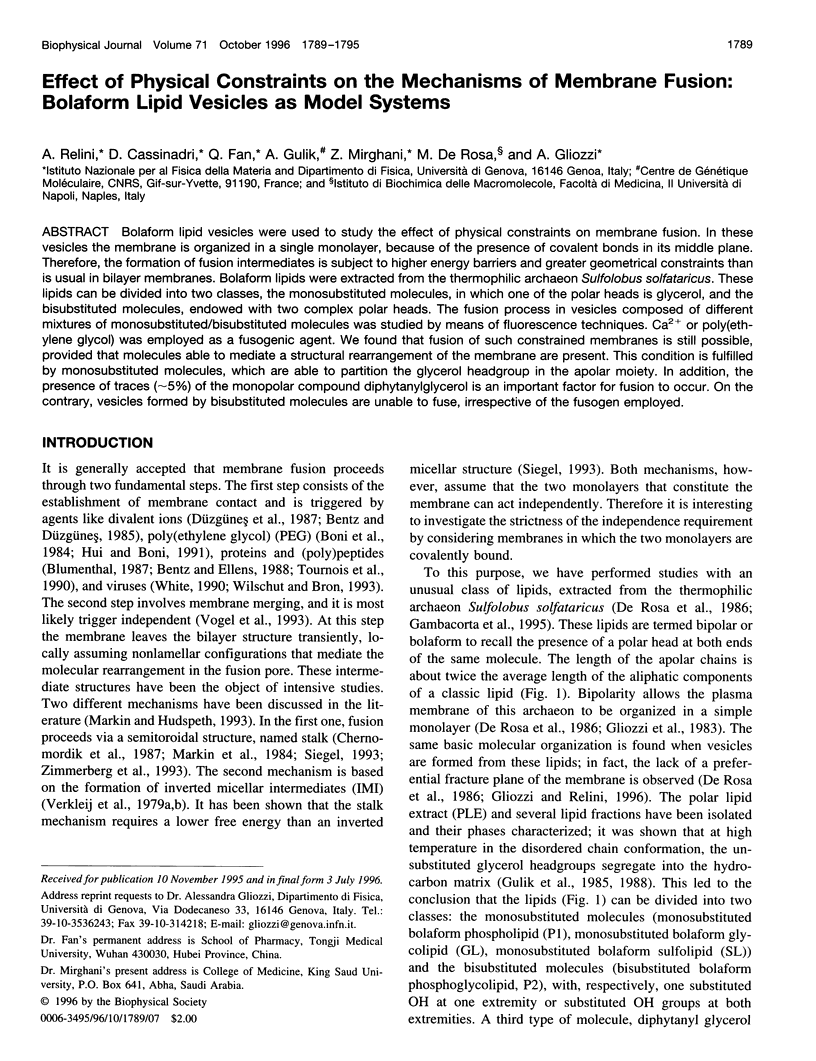
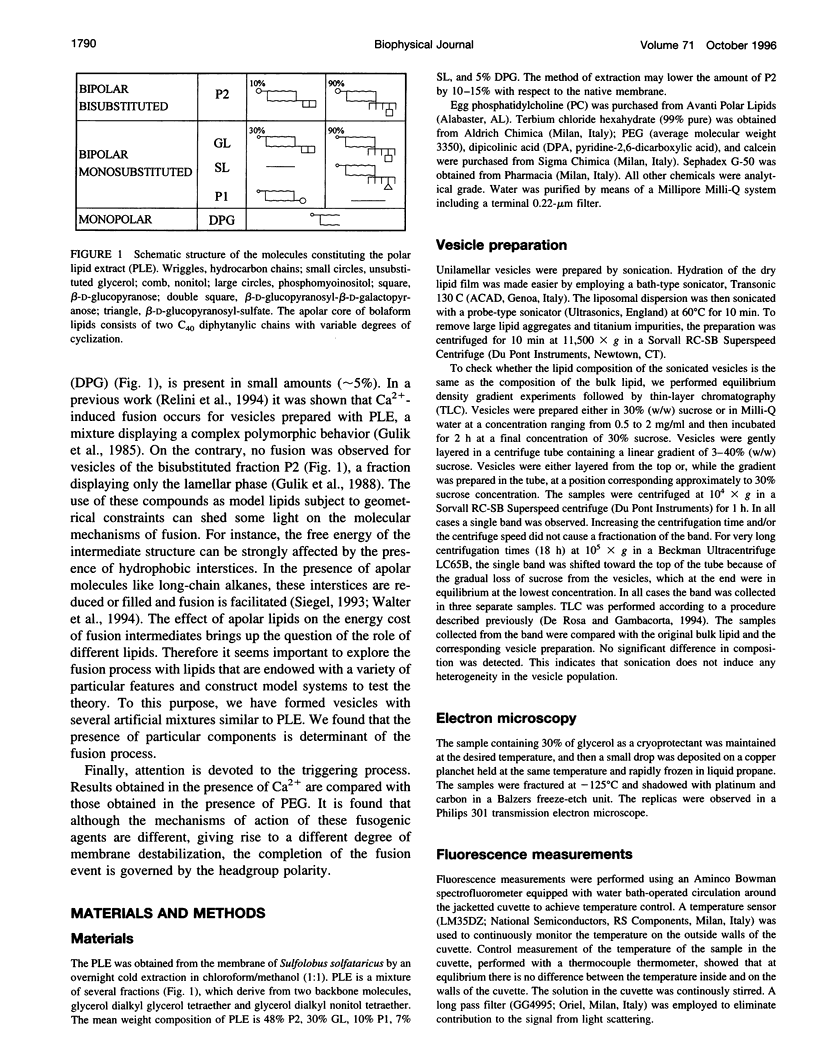
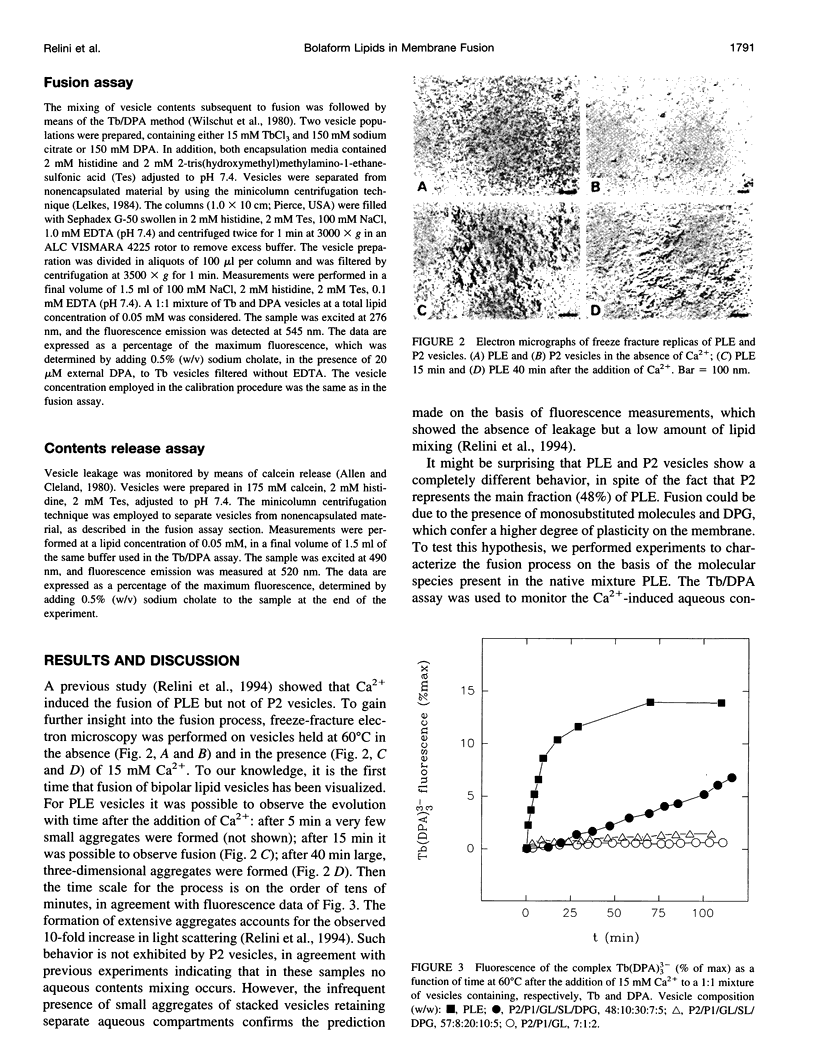
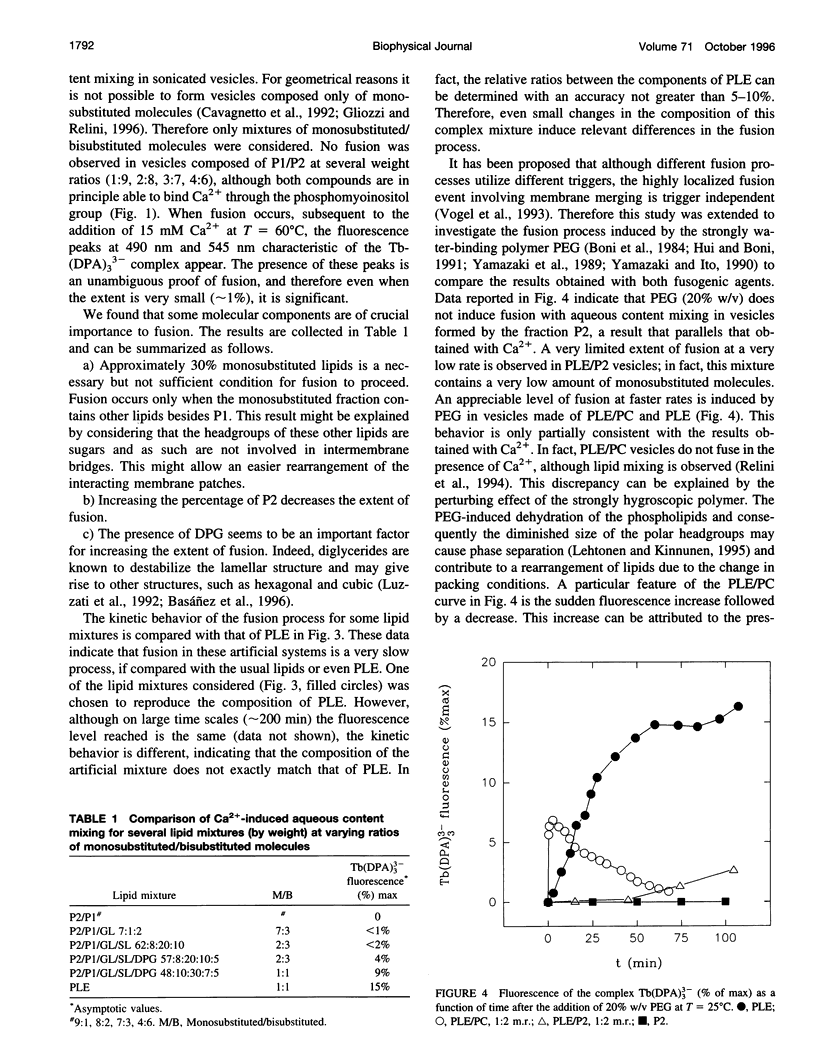
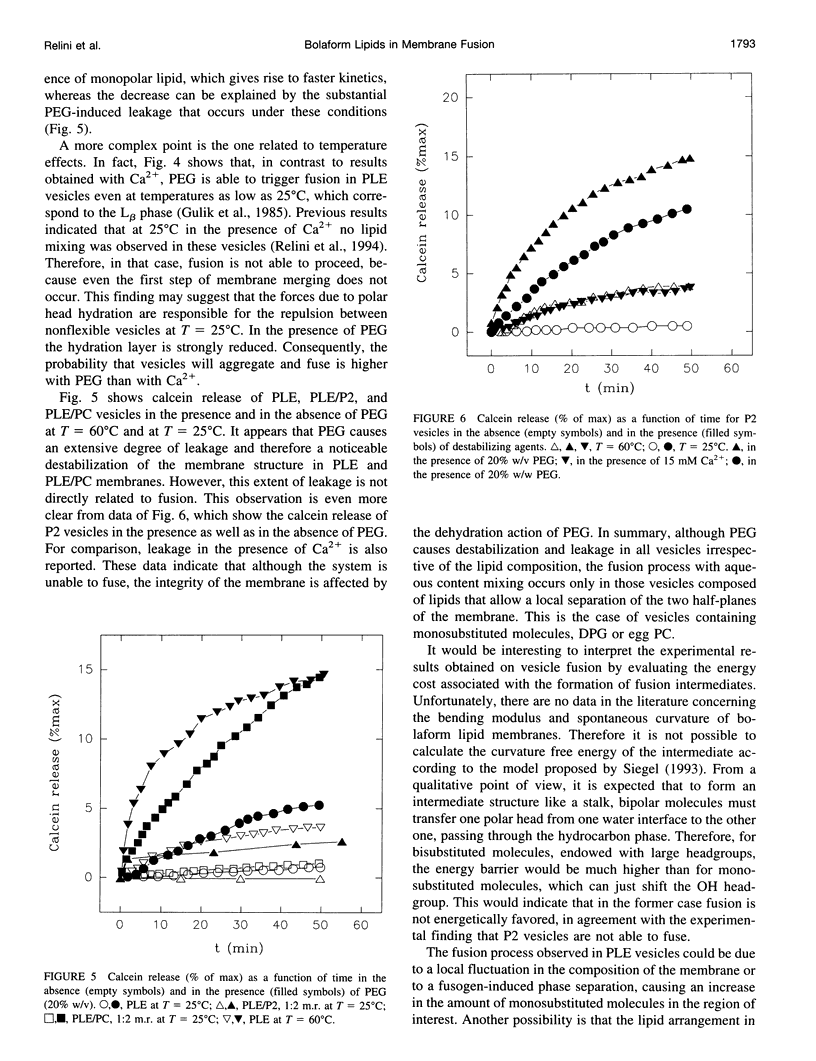
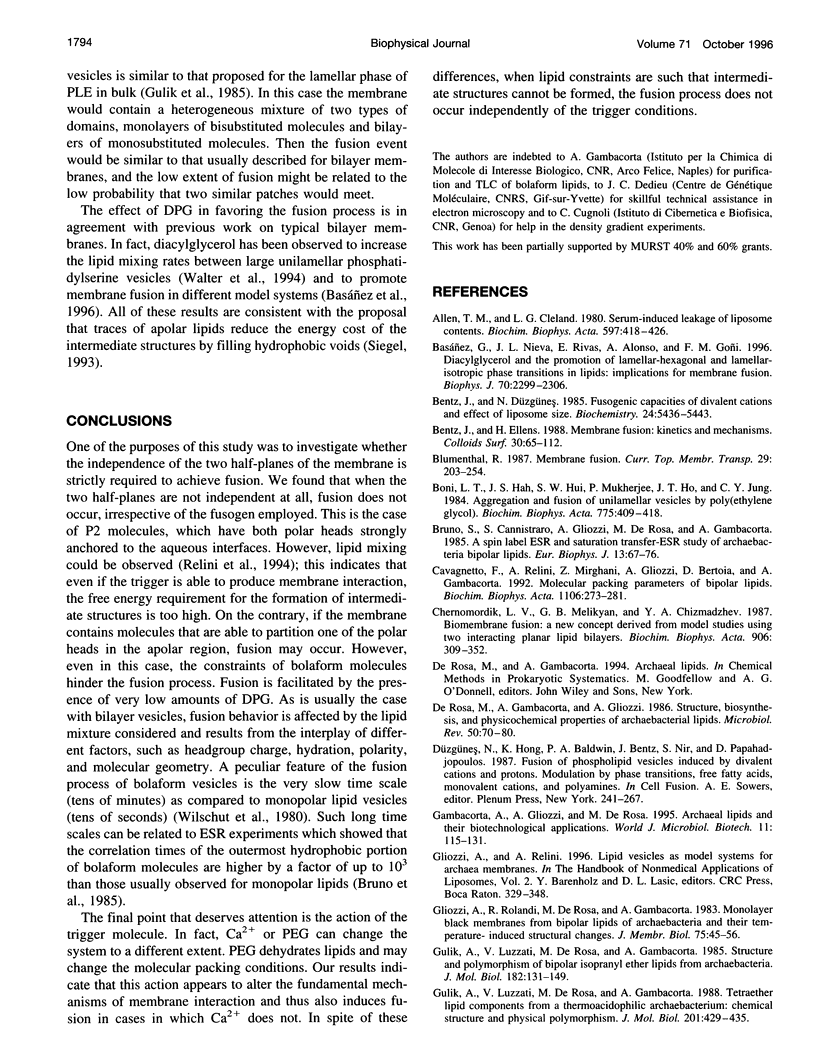
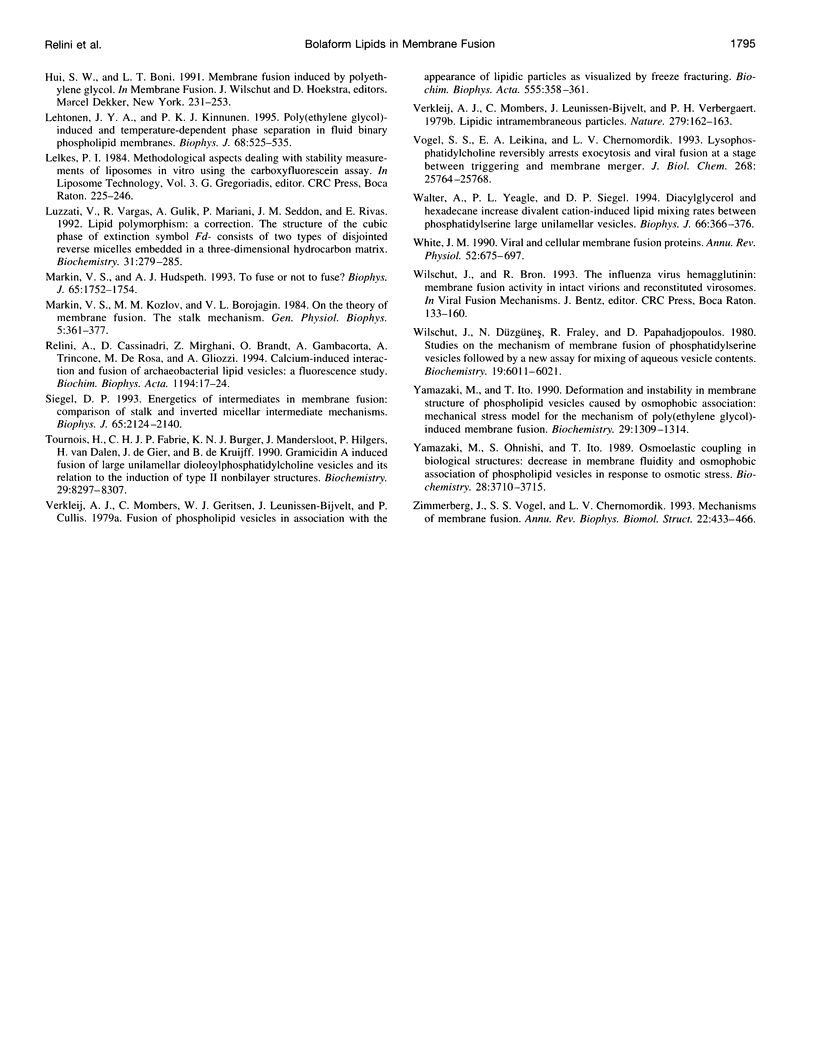
Images in this article
Selected References
These references are in PubMed. This may not be the complete list of references from this article.
- Allen T. M., Cleland L. G. Serum-induced leakage of liposome contents. Biochim Biophys Acta. 1980 Apr 10;597(2):418–426. doi: 10.1016/0005-2736(80)90118-2. [DOI] [PubMed] [Google Scholar]
- Basanez G., Nieva J. L., Rivas E., Alonso A., Goni F. M. Diacylglycerol and the promotion of lamellar-hexagonal and lamellar-isotropic phase transitions in lipids: implications for membrane fusion. Biophys J. 1996 May;70(5):2299–2306. doi: 10.1016/S0006-3495(96)79795-3. [DOI] [PMC free article] [PubMed] [Google Scholar]
- Bentz J., Düzgüneş N. Fusogenic capacities of divalent cations and effect of liposome size. Biochemistry. 1985 Sep 24;24(20):5436–5443. doi: 10.1021/bi00341a023. [DOI] [PubMed] [Google Scholar]
- Boni L. T., Hah J. S., Hui S. W., Mukherjee P., Ho J. T., Jung C. Y. Aggregation and fusion of unilamellar vesicles by poly(ethylene glycol). Biochim Biophys Acta. 1984 Sep 5;775(3):409–418. doi: 10.1016/0005-2736(84)90198-6. [DOI] [PubMed] [Google Scholar]
- Cavagnetto F., Relini A., Mirghani Z., Gliozzi A., Bertoia D., Gambacorta A. Molecular packing parameters of bipolar lipids. Biochim Biophys Acta. 1992 May 21;1106(2):273–281. doi: 10.1016/0005-2736(92)90006-8. [DOI] [PubMed] [Google Scholar]
- Chernomordik L. V., Melikyan G. B., Chizmadzhev Y. A. Biomembrane fusion: a new concept derived from model studies using two interacting planar lipid bilayers. Biochim Biophys Acta. 1987 Oct 5;906(3):309–352. doi: 10.1016/0304-4157(87)90016-5. [DOI] [PubMed] [Google Scholar]
- De Rosa M., Gambacorta A., Gliozzi A. Structure, biosynthesis, and physicochemical properties of archaebacterial lipids. Microbiol Rev. 1986 Mar;50(1):70–80. doi: 10.1128/mr.50.1.70-80.1986. [DOI] [PMC free article] [PubMed] [Google Scholar]
- Gliozzi A., Rolandi R., De Rosa M., Gambacorta A. Monolayer black membranes from bipolar lipids of archaebacteria and their temperature-induced structural changes. J Membr Biol. 1983;75(1):45–56. doi: 10.1007/BF01870798. [DOI] [PubMed] [Google Scholar]
- Gulik A., Luzzati V., De Rosa M., Gambacorta A. Structure and polymorphism of bipolar isopranyl ether lipids from archaebacteria. J Mol Biol. 1985 Mar 5;182(1):131–149. doi: 10.1016/0022-2836(85)90032-4. [DOI] [PubMed] [Google Scholar]
- Gulik A., Luzzati V., DeRosa M., Gambacorta A. Tetraether lipid components from a thermoacidophilic archaebacterium. Chemical structure and physical polymorphism. J Mol Biol. 1988 May 20;201(2):429–435. doi: 10.1016/0022-2836(88)90149-0. [DOI] [PubMed] [Google Scholar]
- Lehtonen J. Y., Kinnunen P. K. Poly(ethylene glycol)-induced and temperature-dependent phase separation in fluid binary phospholipid membranes. Biophys J. 1995 Feb;68(2):525–535. doi: 10.1016/S0006-3495(95)80214-6. [DOI] [PMC free article] [PubMed] [Google Scholar]
- Luzzati V., Vargas R., Gulik A., Mariani P., Seddon J. M., Rivas E. Lipid polymorphism: a correction. The structure of the cubic phase of extinction symbol Fd-- consists of two types of disjointed reverse micelles embedded in a three-dimensional hydrocarbon matrix. Biochemistry. 1992 Jan 14;31(1):279–285. doi: 10.1021/bi00116a038. [DOI] [PubMed] [Google Scholar]
- Markin V. S., Hudspeth A. J. To fuse or not to fuse? Biophys J. 1993 Nov;65(5):1752–1754. doi: 10.1016/S0006-3495(93)81230-X. [DOI] [PMC free article] [PubMed] [Google Scholar]
- Markin V. S., Kozlov M. M., Borovjagin V. L. On the theory of membrane fusion. The stalk mechanism. Gen Physiol Biophys. 1984 Oct;3(5):361–377. [PubMed] [Google Scholar]
- Relini A., Cassinadri D., Mirghani Z., Brandt O., Gambacorta A., Trincone A., De Rosa M., Gliozzi A. Calcium-induced interaction and fusion of archaeobacterial lipid vesicles: a fluorescence study. Biochim Biophys Acta. 1994 Aug 24;1194(1):17–24. doi: 10.1016/0005-2736(94)90198-8. [DOI] [PubMed] [Google Scholar]
- Siegel D. P. Energetics of intermediates in membrane fusion: comparison of stalk and inverted micellar intermediate mechanisms. Biophys J. 1993 Nov;65(5):2124–2140. doi: 10.1016/S0006-3495(93)81256-6. [DOI] [PMC free article] [PubMed] [Google Scholar]
- Tournois H., Fabrie C. H., Burger K. N., Mandersloot J., Hilgers P., van Dalen H., de Gier J., de Kruijff B. Gramicidin A induced fusion of large unilamellar dioleoylphosphatidylcholine vesicles and its relation to the induction of type II nonbilayer structures. Biochemistry. 1990 Sep 11;29(36):8297–8307. doi: 10.1021/bi00488a014. [DOI] [PubMed] [Google Scholar]
- Verkleij A. J., Mombers C., Gerritsen W. J., Leunissen-Bijvelt L., Cullis P. R. Fusion of phospholipid vesicles in association with the appearance of lipidic particles as visualized by freeze fracturing. Biochim Biophys Acta. 1979 Aug 7;555(2):358–361. doi: 10.1016/0005-2736(79)90175-5. [DOI] [PubMed] [Google Scholar]
- Verkleij A. J., Momvers C., Leunissen-Bijvelt J., Ververgaert P. H. Lipidic intramembranous particles. Nature. 1979 May 10;279(5709):162–163. doi: 10.1038/279162a0. [DOI] [PubMed] [Google Scholar]
- Vogel S. S., Leikina E. A., Chernomordik L. V. Lysophosphatidylcholine reversibly arrests exocytosis and viral fusion at a stage between triggering and membrane merger. J Biol Chem. 1993 Dec 5;268(34):25764–25768. [PubMed] [Google Scholar]
- Walter A., Yeagle P. L., Siegel D. P. Diacylglycerol and hexadecane increase divalent cation-induced lipid mixing rates between phosphatidylserine large unilamellar vesicles. Biophys J. 1994 Feb;66(2 Pt 1):366–376. doi: 10.1016/s0006-3495(94)80786-6. [DOI] [PMC free article] [PubMed] [Google Scholar]
- White J. M. Viral and cellular membrane fusion proteins. Annu Rev Physiol. 1990;52:675–697. doi: 10.1146/annurev.ph.52.030190.003331. [DOI] [PubMed] [Google Scholar]
- Wilschut J., Düzgüneş N., Fraley R., Papahadjopoulos D. Studies on the mechanism of membrane fusion: kinetics of calcium ion induced fusion of phosphatidylserine vesicles followed by a new assay for mixing of aqueous vesicle contents. Biochemistry. 1980 Dec 23;19(26):6011–6021. doi: 10.1021/bi00567a011. [DOI] [PubMed] [Google Scholar]
- Yamazaki M., Ito T. Deformation and instability in membrane structure of phospholipid vesicles caused by osmophobic association: mechanical stress model for the mechanism of poly(ethylene glycol)-induced membrane fusion. Biochemistry. 1990 Feb 6;29(5):1309–1314. doi: 10.1021/bi00457a029. [DOI] [PubMed] [Google Scholar]
- Yamazaki M., Ohnishi S., Ito T. Osmoelastic coupling in biological structures: decrease in membrane fluidity and osmophobic association of phospholipid vesicles in response to osmotic stress. Biochemistry. 1989 May 2;28(9):3710–3715. doi: 10.1021/bi00435a013. [DOI] [PubMed] [Google Scholar]
- Zimmerberg J., Vogel S. S., Chernomordik L. V. Mechanisms of membrane fusion. Annu Rev Biophys Biomol Struct. 1993;22:433–466. doi: 10.1146/annurev.bb.22.060193.002245. [DOI] [PubMed] [Google Scholar]