Abstract
Azurin, a blue copper protein from the bacterial species Pseudomonas aeruginosa, contains a single tryptophan residue. Previous fluorescence measurements indicate that this residue is highly constrained and unusually inaccessible to water. In the apoprotein this residue also possesses a long-lived room-temperature phosphorescence (RTP), the nonexponential decay of which can be resolved into two major components associated with lifetimes of 417 and 592 ms, which likely originate from at least two conformations of the protein. The relative weights of these two decay components change with pH in good correlation with a change in protonation of His-35, which has been studied in Cu(II) azurin. Interestingly, the structural changes characterized in earlier work have little effect on the fluorescence decay and appear to occur away from the tryptophan residue. However, in the present work, the two RTP lifetimes suggest conformations with different structural rigidities in the vicinity of the tryptophan residue. The active conformation that predominates below a pH of 5.6 has the shorter lifetime and is less rigid. Phosphorescence decays of several metal derivatives of azurin were also measured and revealed strong similarities to that of apoazurin, indicating that the structural constraints upon the metal-binding site are imposed predominately by the protein.
Full text
PDF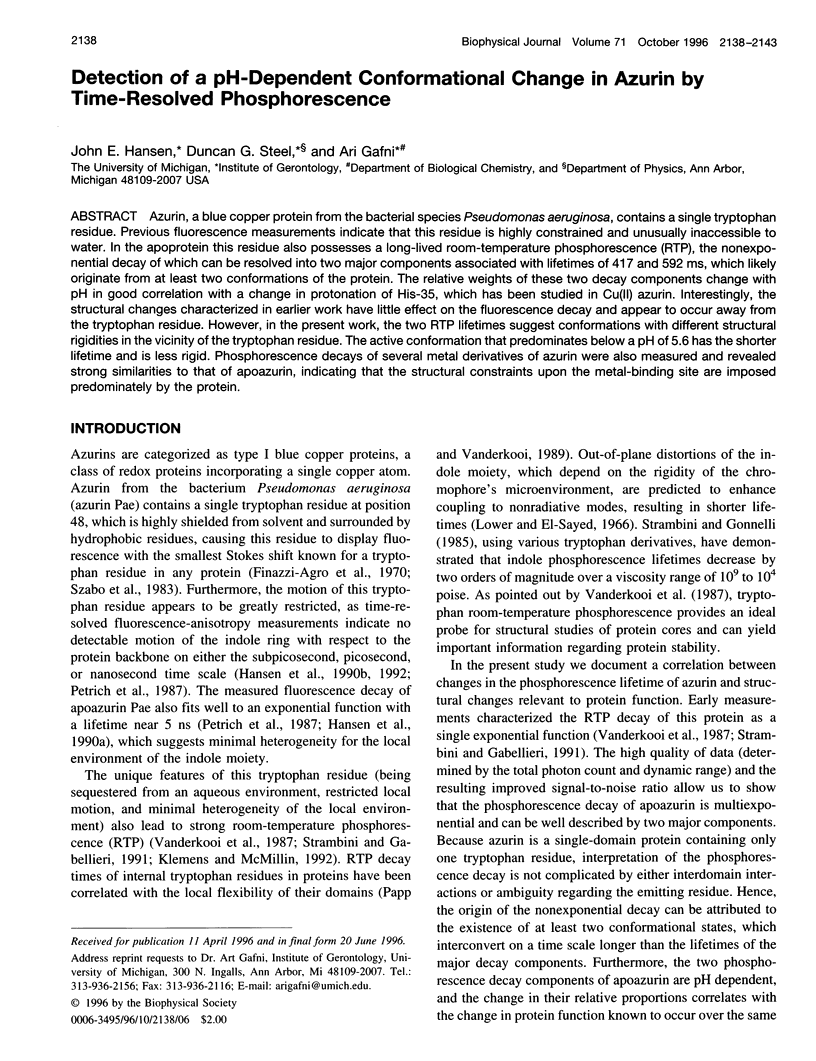
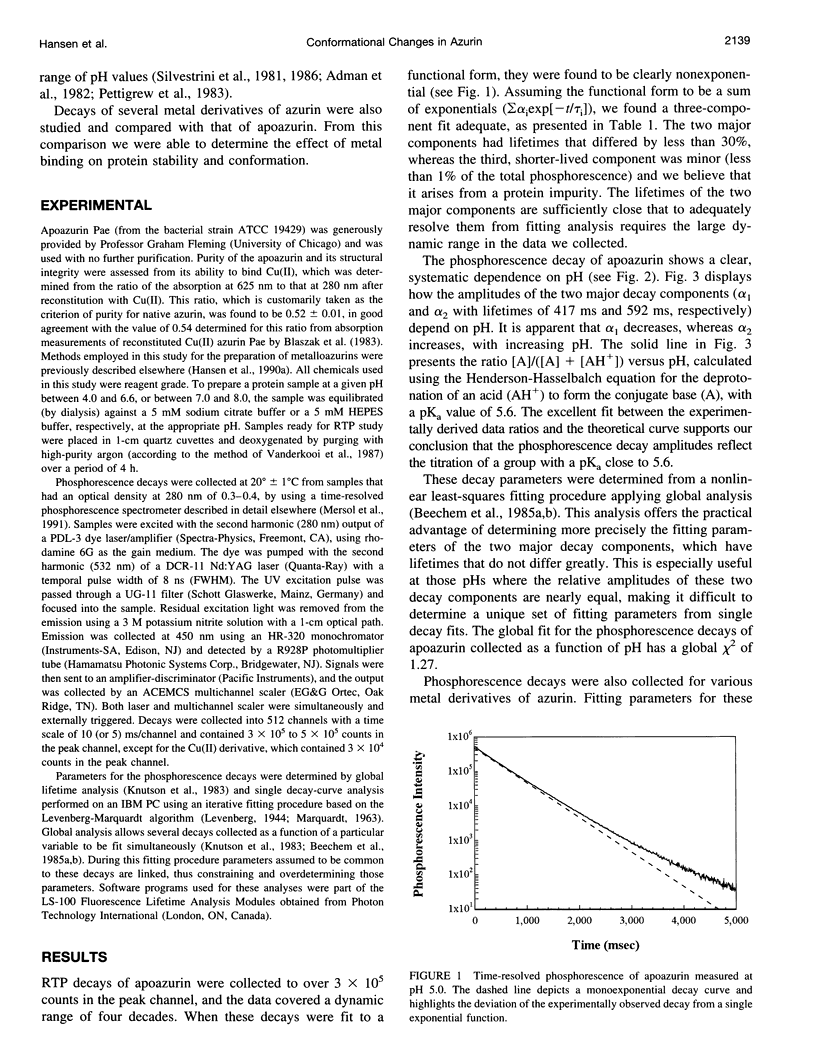
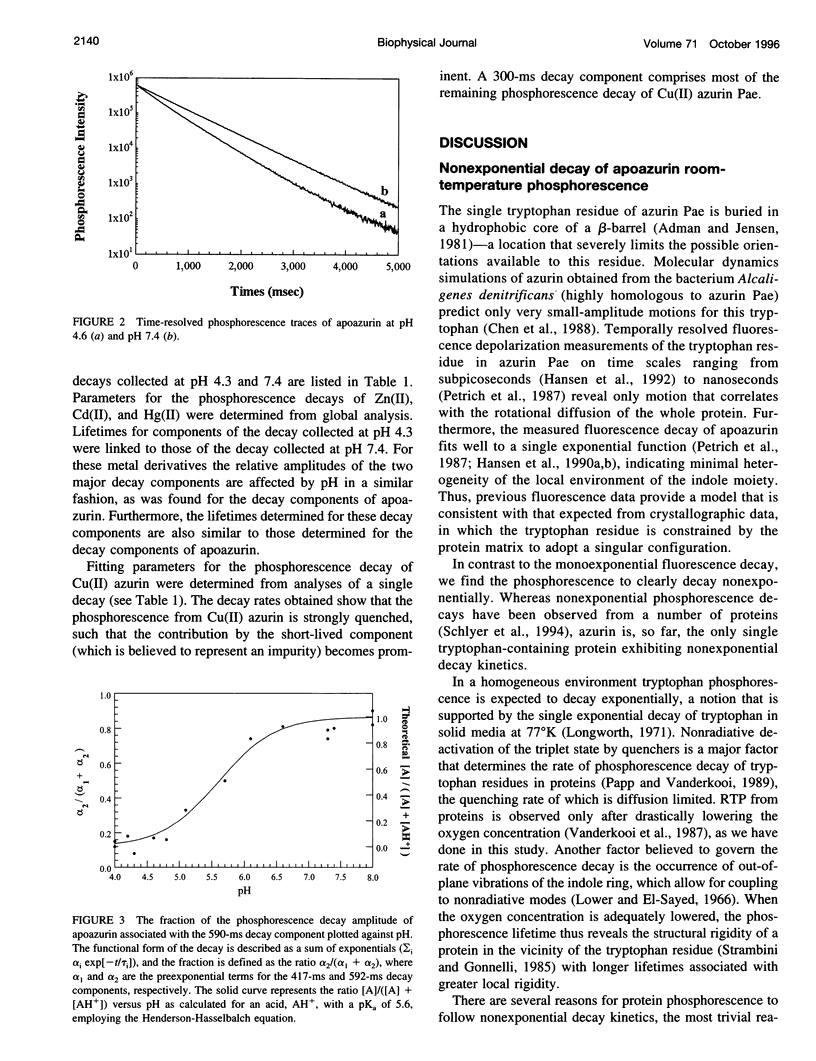
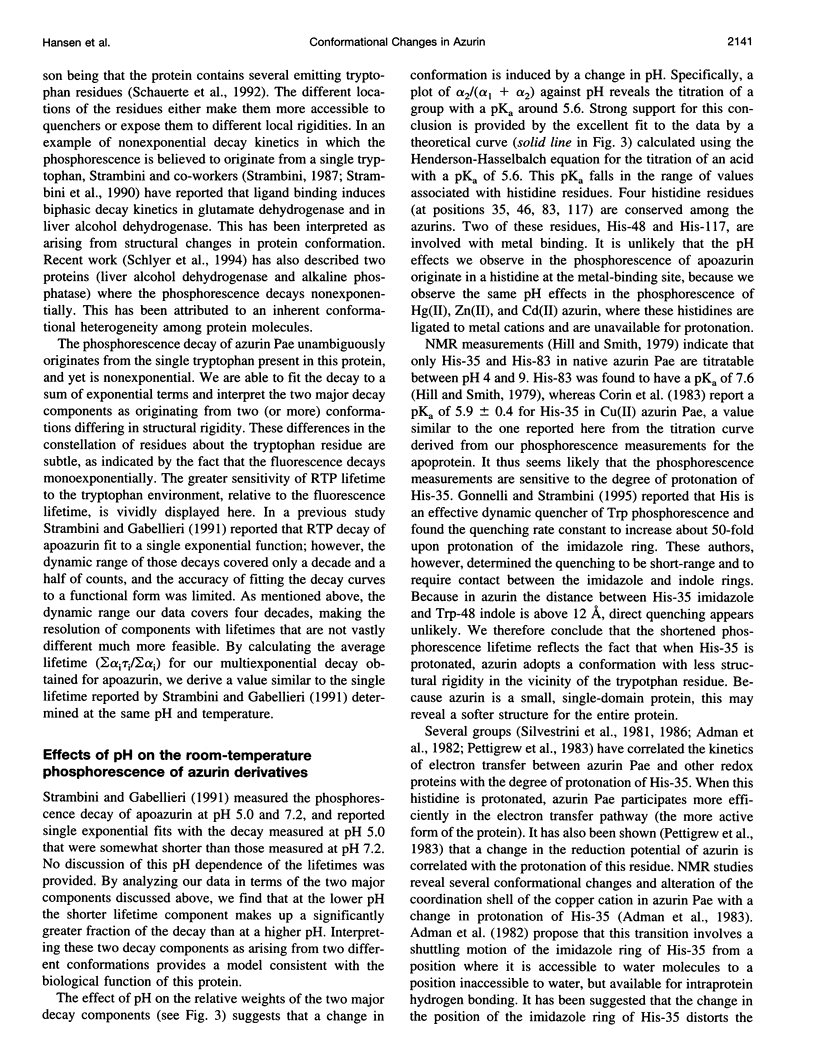
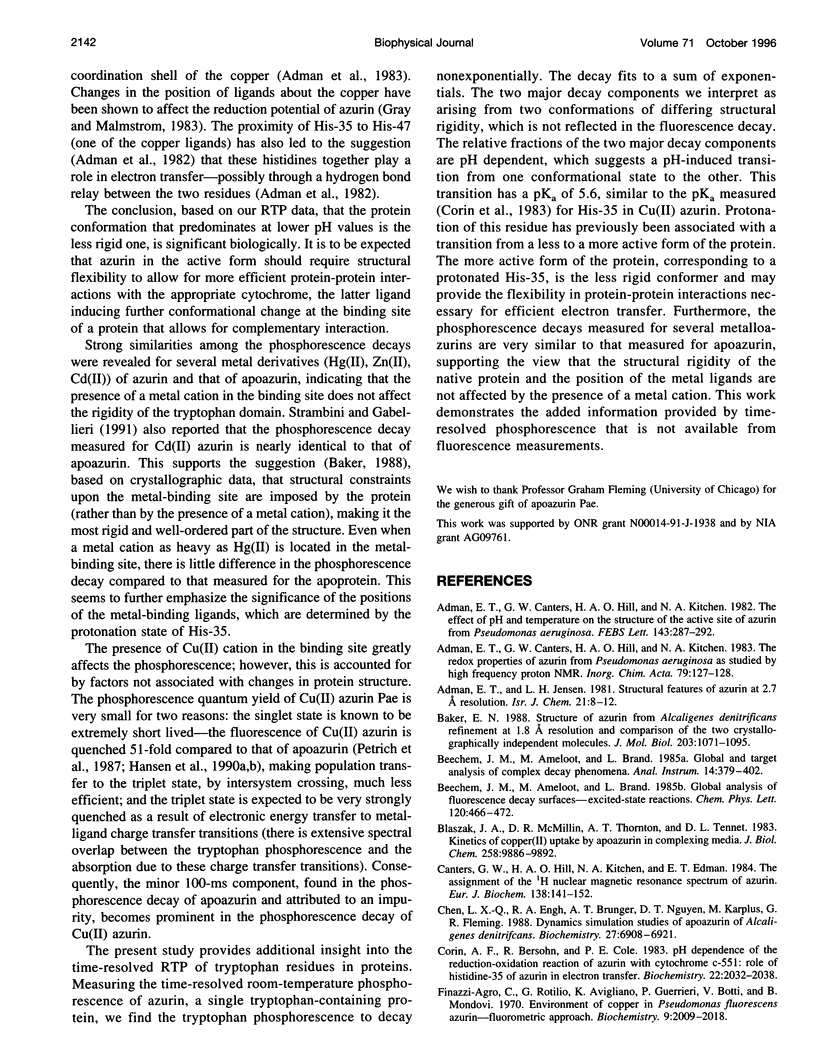
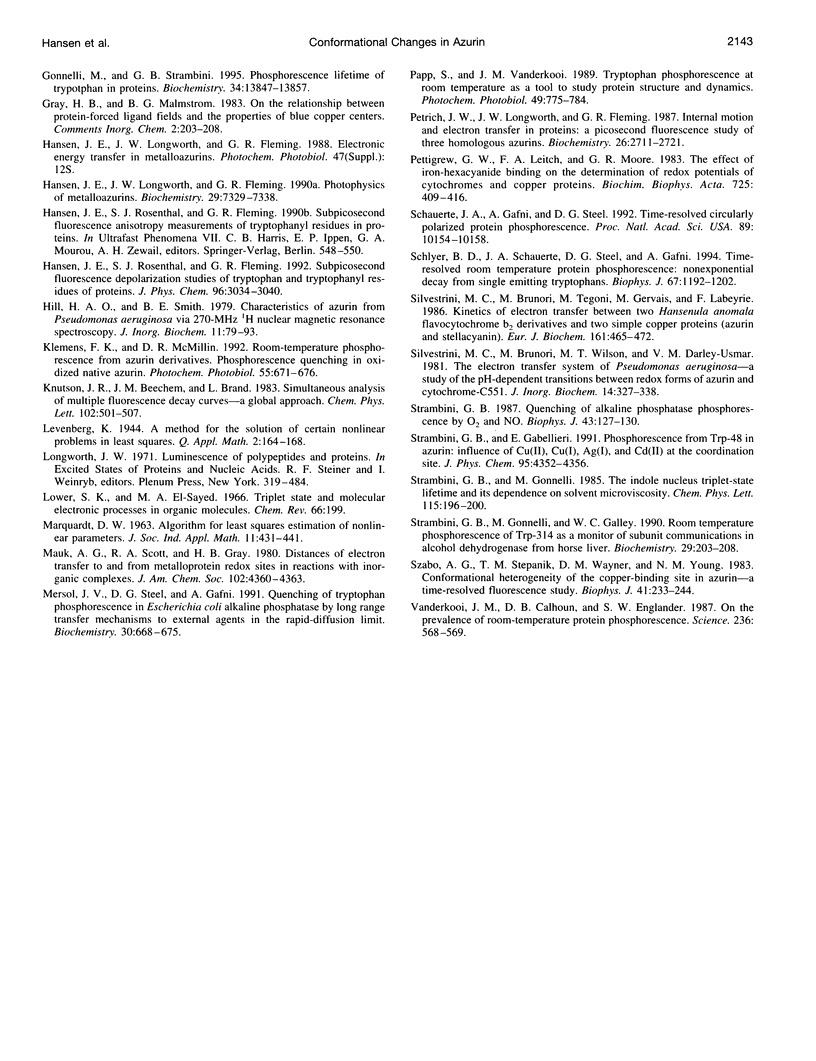
Selected References
These references are in PubMed. This may not be the complete list of references from this article.
- Adman E. T., Canters G. W., Hill H. A., Kitchen N. A. The effect of pH and temperature on the structure of the active site of azurin from Pseudomonas aeruginosa. FEBS Lett. 1982 Jul 5;143(2):287–292. doi: 10.1016/0014-5793(82)80118-x. [DOI] [PubMed] [Google Scholar]
- Baker E. N. Structure of azurin from Alcaligenes denitrificans refinement at 1.8 A resolution and comparison of the two crystallographically independent molecules. J Mol Biol. 1988 Oct 20;203(4):1071–1095. doi: 10.1016/0022-2836(88)90129-5. [DOI] [PubMed] [Google Scholar]
- Blaszak J. A., McMillin D. R., Thornton A. T., Tennent D. L. Kinetics of copper(II) uptake by apoazurin in complexing media. J Biol Chem. 1983 Aug 25;258(16):9886–9892. [PubMed] [Google Scholar]
- Canters G. W., Hill H. A., Kitchen N. A., Adman E. T. The assignment of the 1H nuclear magnetic resonance spectrum of azurin. An investigation of the 1H NMR spectrum of the blue copper protein, azurin, from Pseudomonas aeruginosa, with reference to the previously determined crystal structure. Eur J Biochem. 1984 Jan 2;138(1):141–152. doi: 10.1111/j.1432-1033.1984.tb07893.x. [DOI] [PubMed] [Google Scholar]
- Corin A. F., Bersohn R., Cole P. E. pH dependence of the reduction-oxidation reaction of azurin with cytochrome c-551: role of histidine-35 of azurin in electron transfer. Biochemistry. 1983 Apr 12;22(8):2032–2038. doi: 10.1021/bi00277a046. [DOI] [PubMed] [Google Scholar]
- Finazzi-Agrò A., Rotilio G., Avigliano L., Guerrieri P., Boffi V., Mondovì B. Environment of copper in Pseudomonas fluorescens azurin: fluorometric approach. Biochemistry. 1970 Apr 28;9(9):2009–2014. doi: 10.1021/bi00811a023. [DOI] [PubMed] [Google Scholar]
- Gonnelli M., Strambini G. B. Phosphorescence lifetime of tryptophan in proteins. Biochemistry. 1995 Oct 24;34(42):13847–13857. doi: 10.1021/bi00042a017. [DOI] [PubMed] [Google Scholar]
- Hansen J. E., Longworth J. W., Fleming G. R. Photophysics of metalloazurins. Biochemistry. 1990 Aug 7;29(31):7329–7338. doi: 10.1021/bi00483a024. [DOI] [PubMed] [Google Scholar]
- Hansen T. B. Skadebehandlingen i Ringkøbing Amt. 3. Befolkningen og de alment praktiserende loegers holdninger og adfoerd i relation til skadebehandling. Ugeskr Laeger. 1992 Oct 26;154(44):3040–3044. [PubMed] [Google Scholar]
- Hill H. A., Smith B. E. Characteristics of azurin from Pseudomonas aeruginosa via 270-MHz 1H nuclear magnetic resonance spectroscopy. J Inorg Biochem. 1979 Oct;11(2):79–93. doi: 10.1016/s0162-0134(00)80174-9. [DOI] [PubMed] [Google Scholar]
- Klemens F. K., McMillin D. R. Room-temperature phosphorescence from azurin derivatives. Phosphorescence quenching in oxidized native azurin. Photochem Photobiol. 1992 May;55(5):671–676. doi: 10.1111/j.1751-1097.1992.tb08509.x. [DOI] [PubMed] [Google Scholar]
- Mersol J. V., Steel D. G., Gafni A. Quenching of tryptophan phosphorescence in Escherichia coli alkaline phosphatase by long-range transfer mechanisms to external agents in the rapid-diffusion limit. Biochemistry. 1991 Jan 22;30(3):668–675. doi: 10.1021/bi00217a012. [DOI] [PubMed] [Google Scholar]
- Papp S., Vanderkooi J. M. Tryptophan phosphorescence at room temperature as a tool to study protein structure and dynamics. Photochem Photobiol. 1989 Jun;49(6):775–784. doi: 10.1111/j.1751-1097.1989.tb05576.x. [DOI] [PubMed] [Google Scholar]
- Petrich J. W., Longworth J. W., Fleming G. R. Internal motion and electron transfer in proteins: a picosecond fluorescence study of three homologous azurins. Biochemistry. 1987 May 19;26(10):2711–2722. doi: 10.1021/bi00384a010. [DOI] [PubMed] [Google Scholar]
- Pettigrew G. W., Leitch F. A., Moore G. R. The effect of iron-hexacyanide binding on the determination of redox potentials of cytochromes and copper proteins. Biochim Biophys Acta. 1983 Dec 30;725(3):409–416. doi: 10.1016/0005-2728(83)90181-0. [DOI] [PubMed] [Google Scholar]
- Schauerte J. A., Steel D. G., Gafni A. Time-resolved circularly polarized protein phosphorescence. Proc Natl Acad Sci U S A. 1992 Nov 1;89(21):10154–10158. doi: 10.1073/pnas.89.21.10154. [DOI] [PMC free article] [PubMed] [Google Scholar]
- Schlyer B. D., Schauerte J. A., Steel D. G., Gafni A. Time-resolved room temperature protein phosphorescence: nonexponential decay from single emitting tryptophans. Biophys J. 1994 Sep;67(3):1192–1202. doi: 10.1016/S0006-3495(94)80588-0. [DOI] [PMC free article] [PubMed] [Google Scholar]
- Silvestrini M. C., Brunori M., Tegoni M., Gervais M., Labeyrie F. Kinetics of electron transfer between two Hansenula anomala flavocytochrome b2 derivatives and two simple copper proteins (azurin and stellacyanin). Eur J Biochem. 1986 Dec 1;161(2):465–472. doi: 10.1111/j.1432-1033.1986.tb10467.x. [DOI] [PubMed] [Google Scholar]
- Strambini G. B., Gonnelli M., Galley W. C. Room temperature phosphorescence of Trp-314 as a monitor of subunit communications in alcohol dehydrogenase from horse liver. Biochemistry. 1990 Jan 9;29(1):203–208. doi: 10.1021/bi00453a028. [DOI] [PubMed] [Google Scholar]
- Strambini G. B. Singular oxygen effects on the room-temperature phosphorescence of alcohol dehydrogenase from horse liver. Biophys J. 1983 Jul;43(1):127–130. doi: 10.1016/S0006-3495(83)84331-8. [DOI] [PMC free article] [PubMed] [Google Scholar]
- Szabo A. G., Stepanik T. M., Wayner D. M., Young N. M. Conformational heterogeneity of the copper binding site in azurin. A time-resolved fluorescence study. Biophys J. 1983 Mar;41(3):233–244. doi: 10.1016/S0006-3495(83)84433-6. [DOI] [PMC free article] [PubMed] [Google Scholar]
- Vanderkooi J. M., Calhoun D. B., Englander S. W. On the prevalence of room-temperature protein phosphorescence. Science. 1987 May 1;236(4801):568–569. doi: 10.1126/science.3576185. [DOI] [PMC free article] [PubMed] [Google Scholar]