Abstract
Confocal imaging has revealed microdomains of intracellular free Ca2+ in turtle hair cells evoked by depolarizing pulses and has delineated factors affecting the growth and dissipation of such domains. However, imaging experiments have limited spatial and temporal resolution. To extend the range of the results we have developed a three-dimensional model of Ca2+ diffusion in a cylindrical hair cell, allowing part of the Ca2+ influx to occur over a small circular region (radius 0.125-1.0 micron) representing a high-density array of voltage-dependent channels. The model incorporated experimental information about the number of channels, the fixed and mobile Ca2+ buffers, and the Ca2+ extrusion mechanism. A feature of the calculations was the use of a variable grid size depending on the proximity to the Ca2+ channel cluster. The results agreed qualitatively with experimental data on the localization of the Ca2+ transients, although the experimental responses were smaller and slower, which is most likely due to temporal and spatial averaging in the imaging. The model made predictions about 1) the optimal Ca2+ channel number and density within a cluster, 2) the conditions to ensure independence of neighboring clusters, and 3) the influence of the Ca2+ buffers on the kinetics and localization of the microdomains. We suggest that an increase in the mobile Ca2+ buffer concentration in high-frequency hair cells (which possess a larger number of release sites) would allow lower amplitude and faster Ca2+ responses and promote functional independence of the sites.
Full text
PDF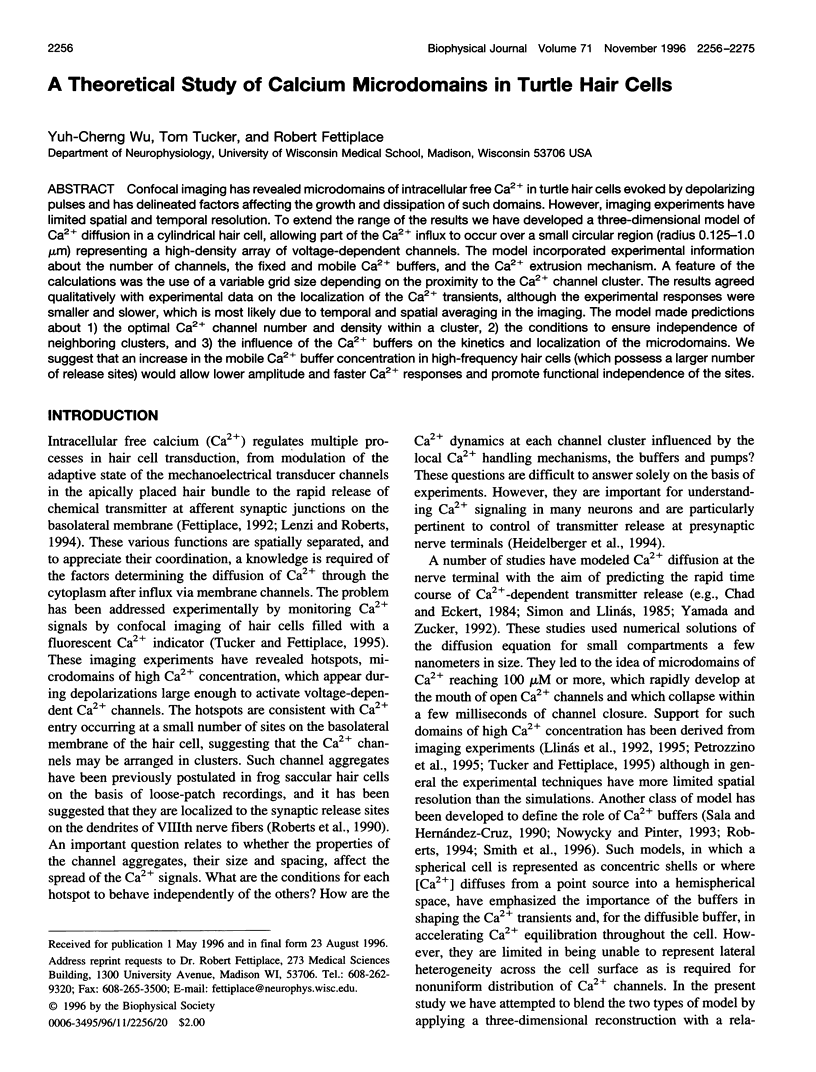
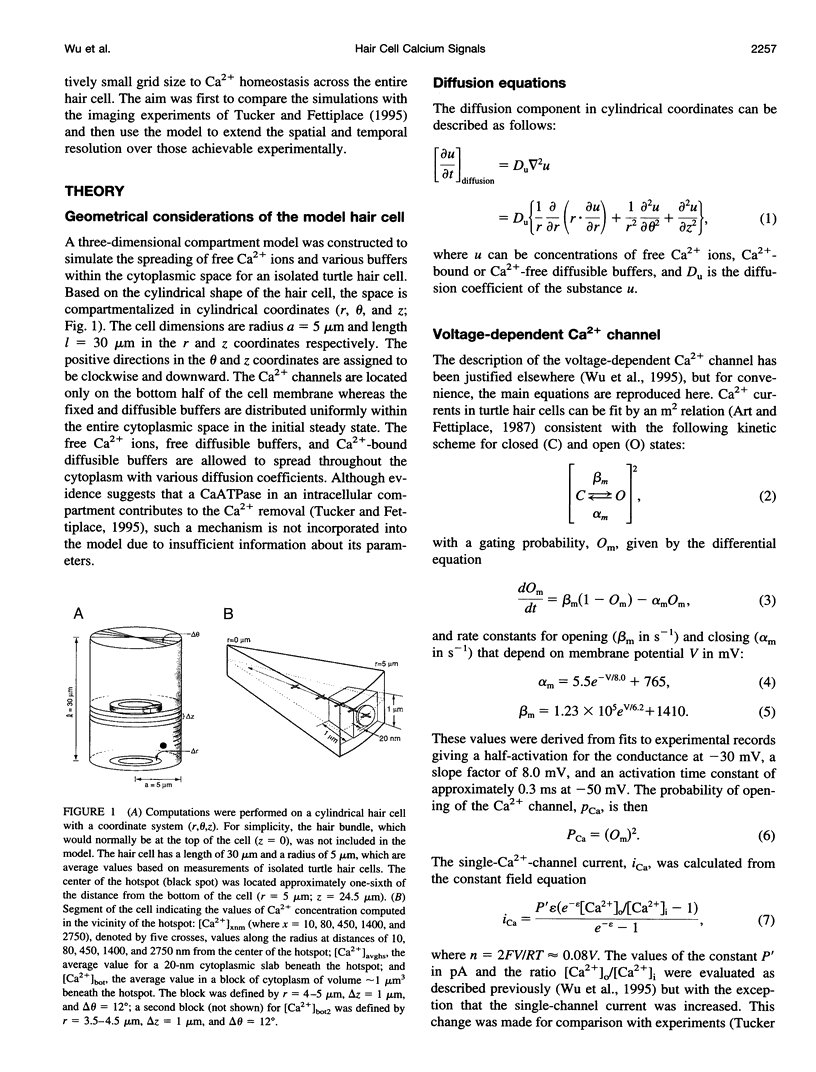
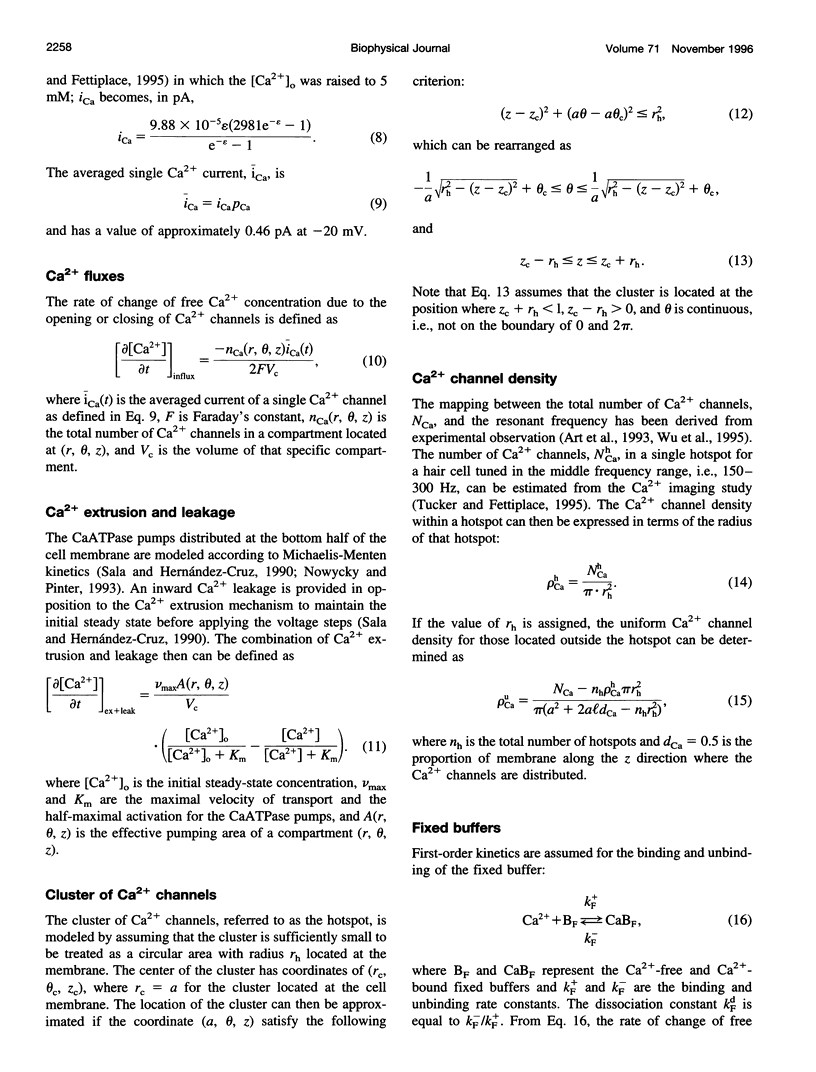
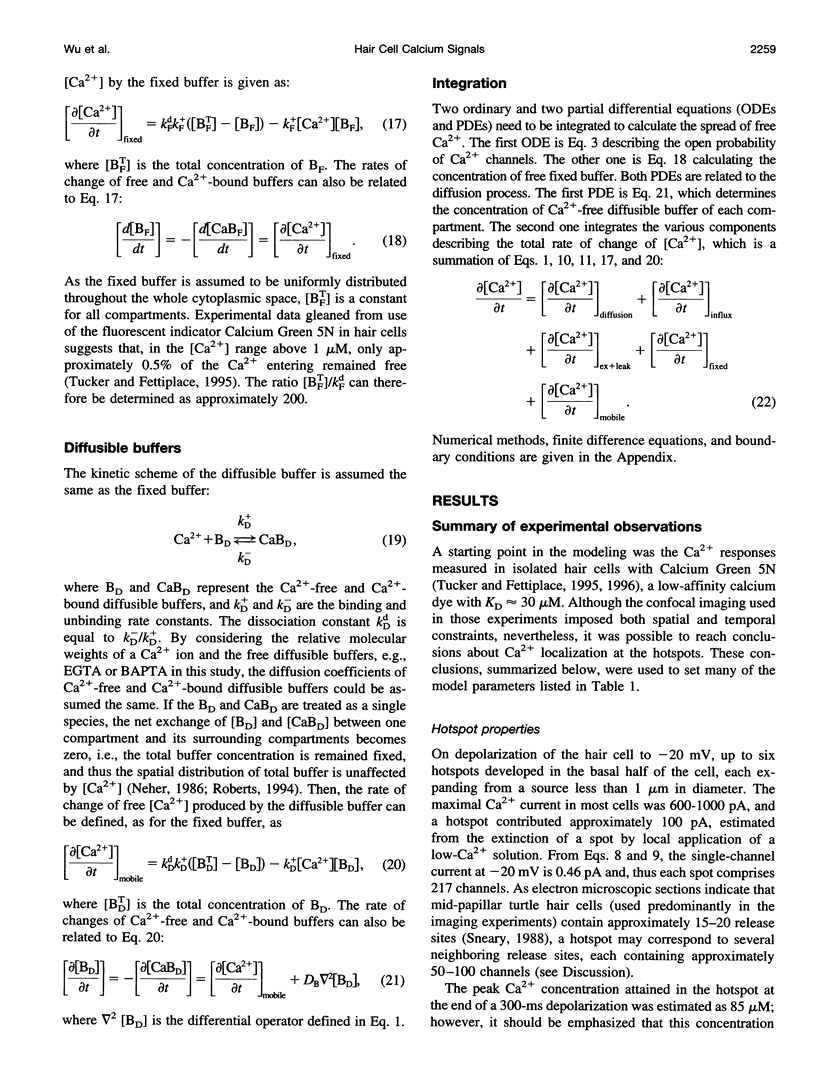
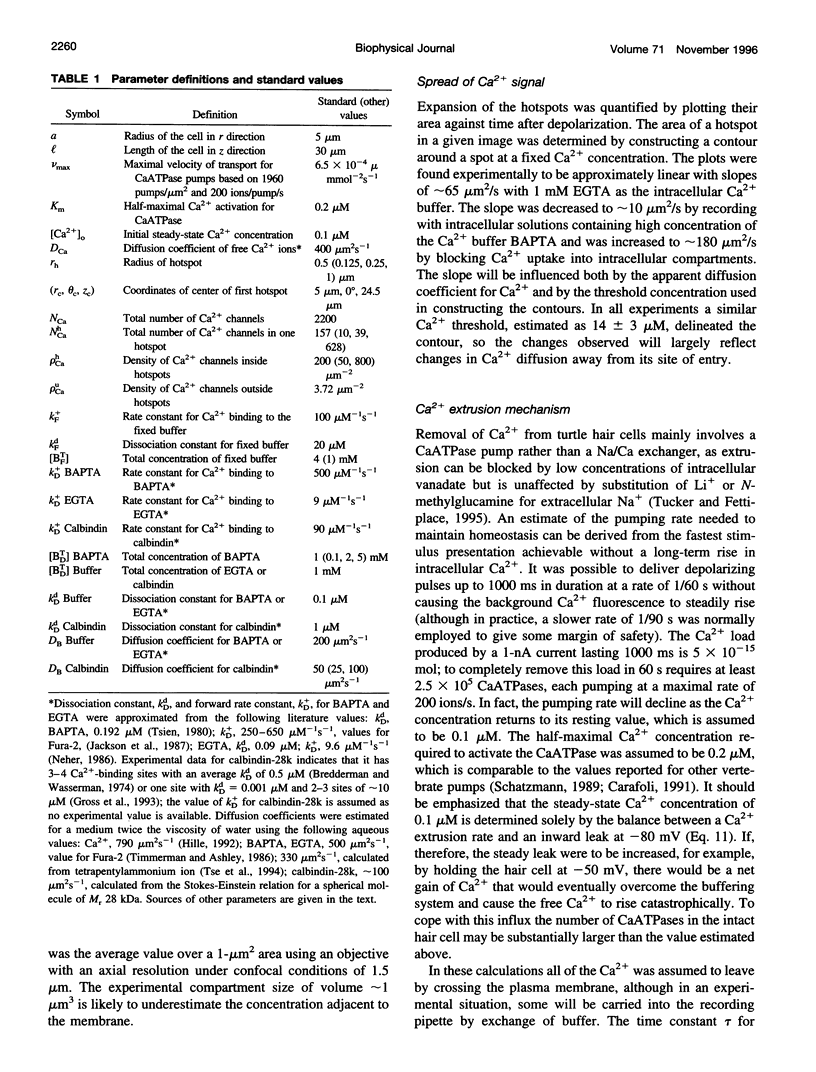
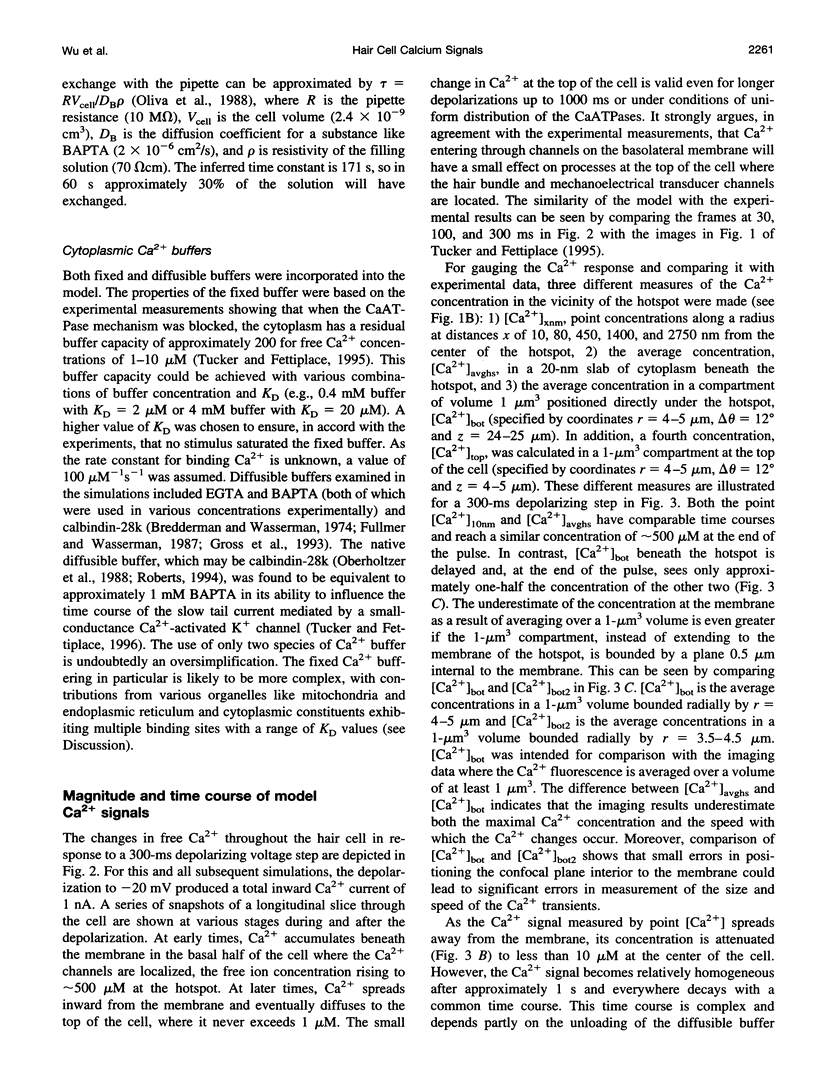
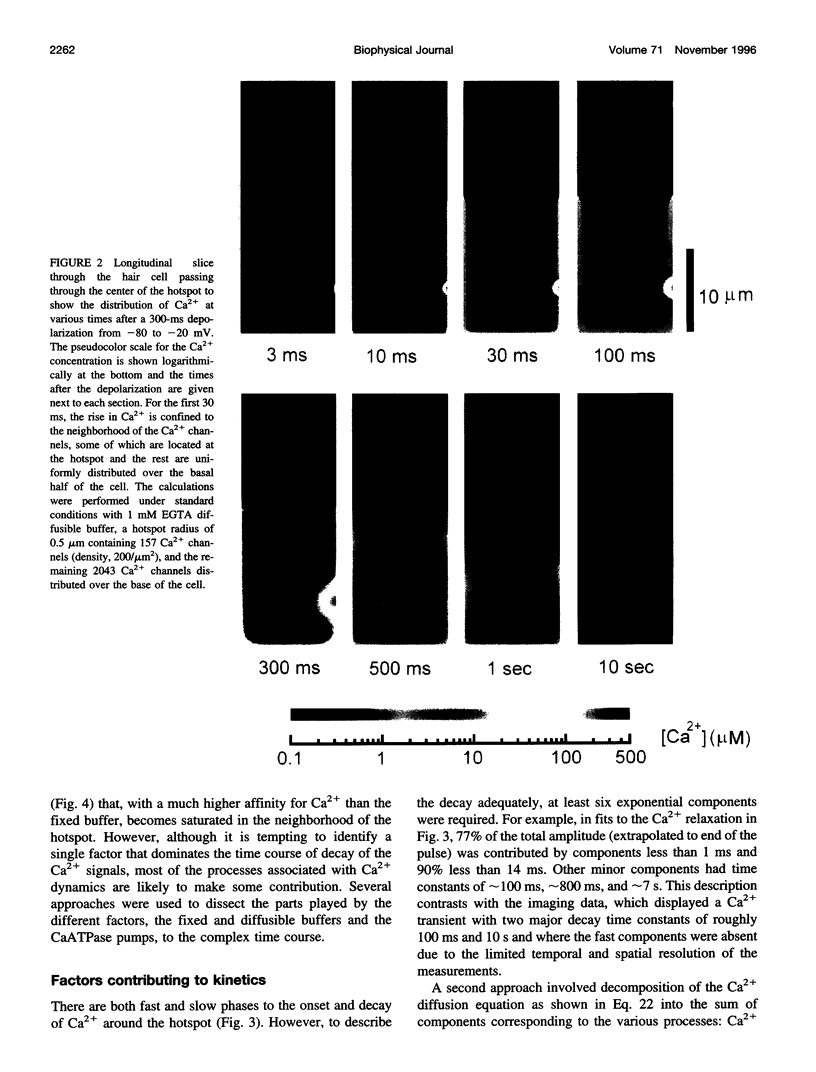
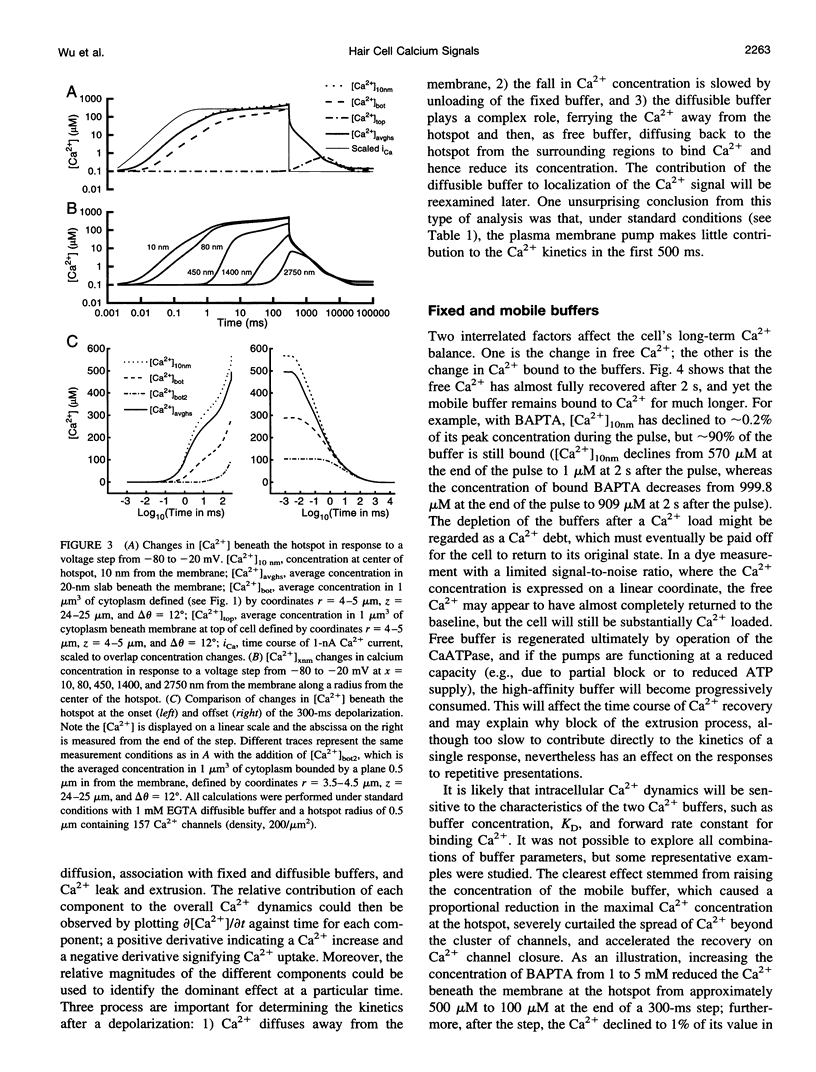
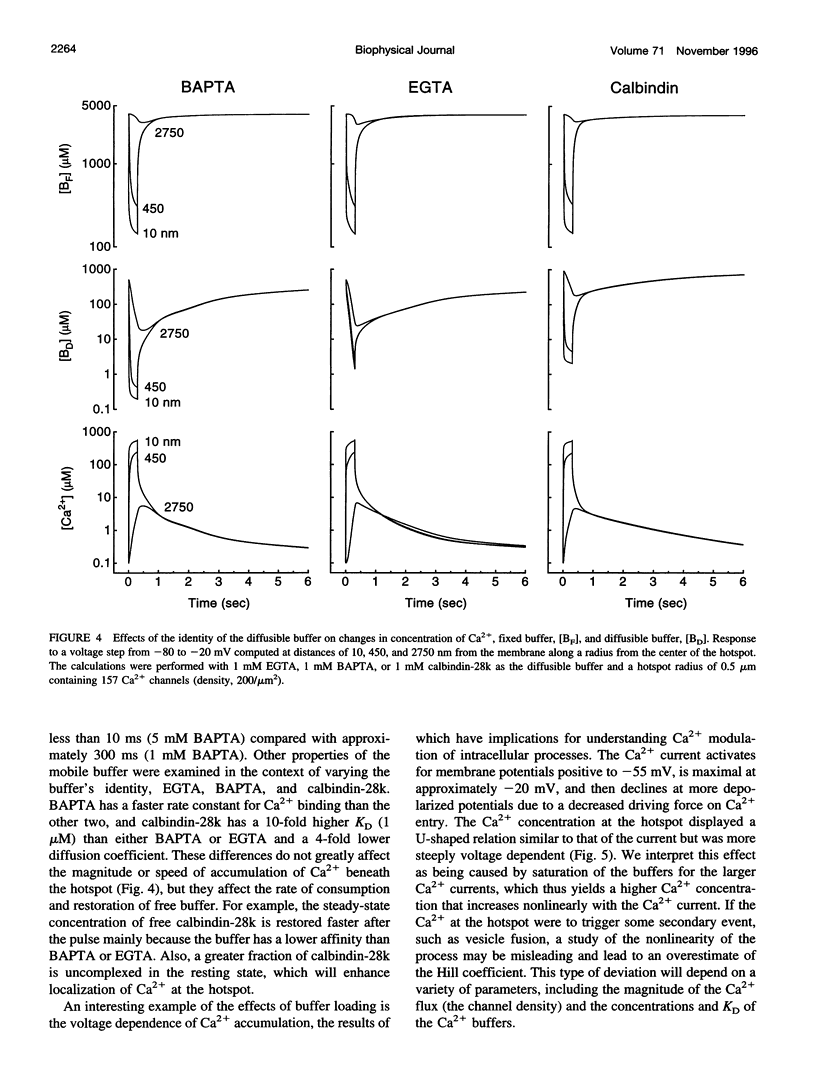
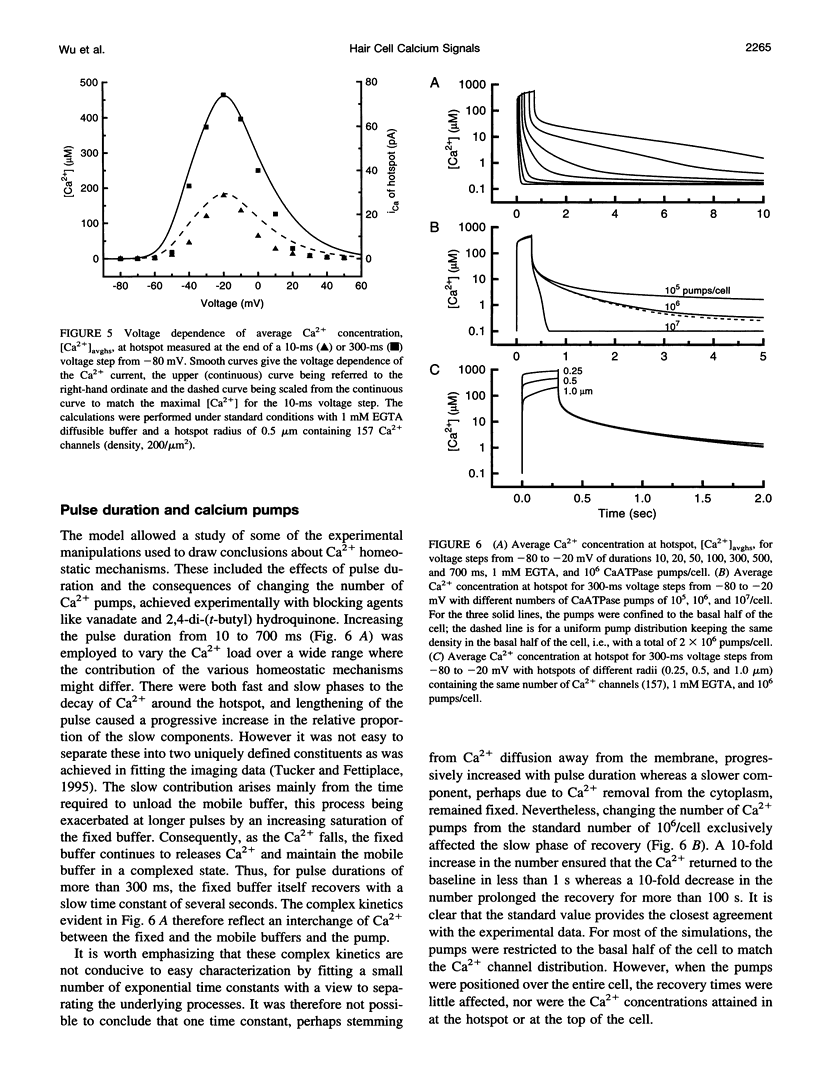
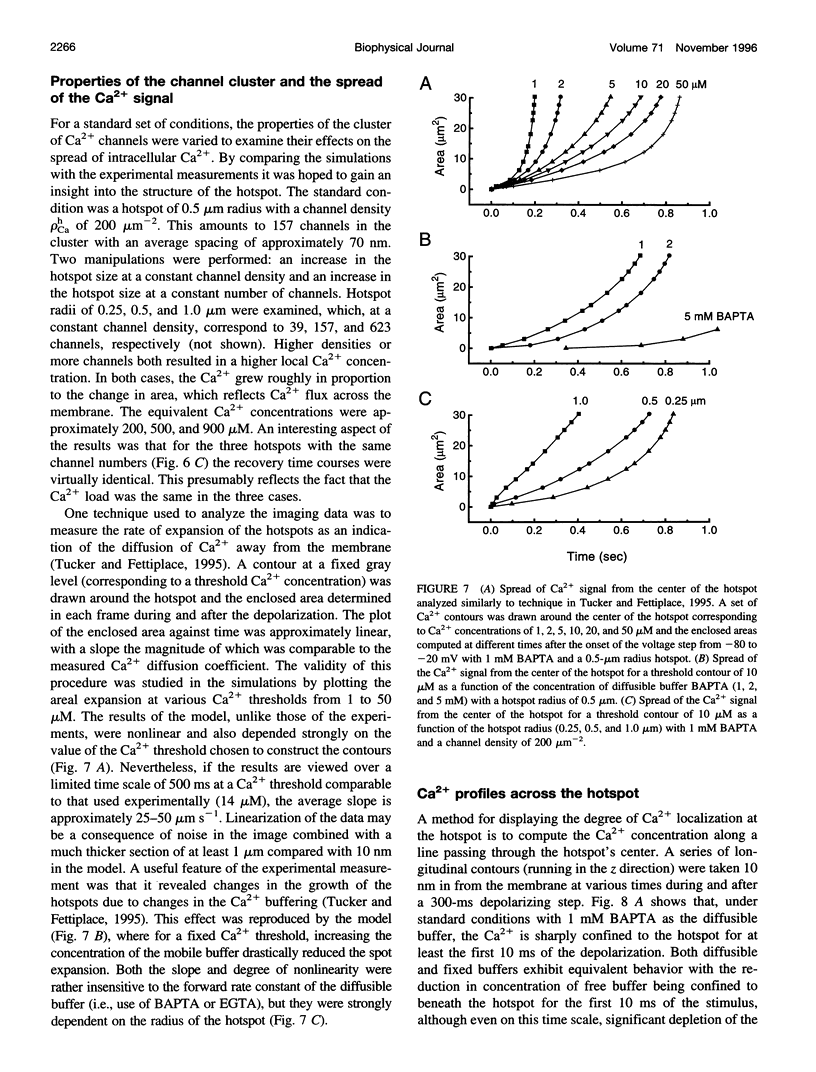
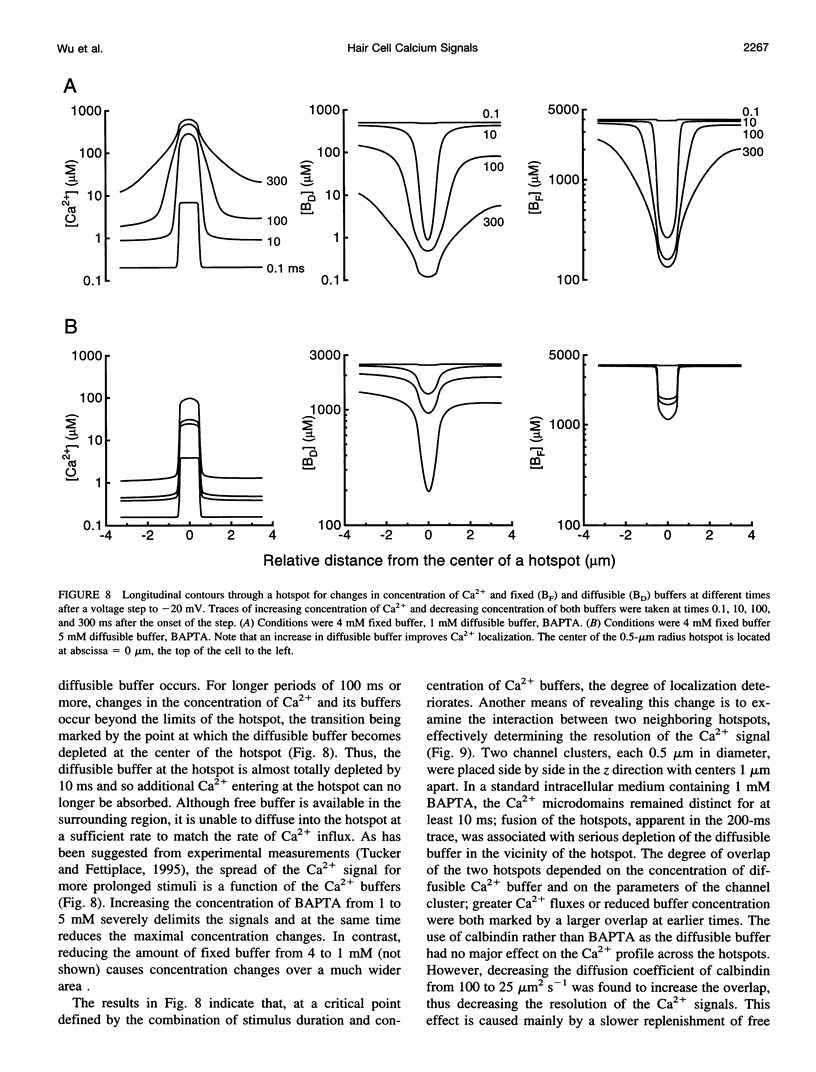
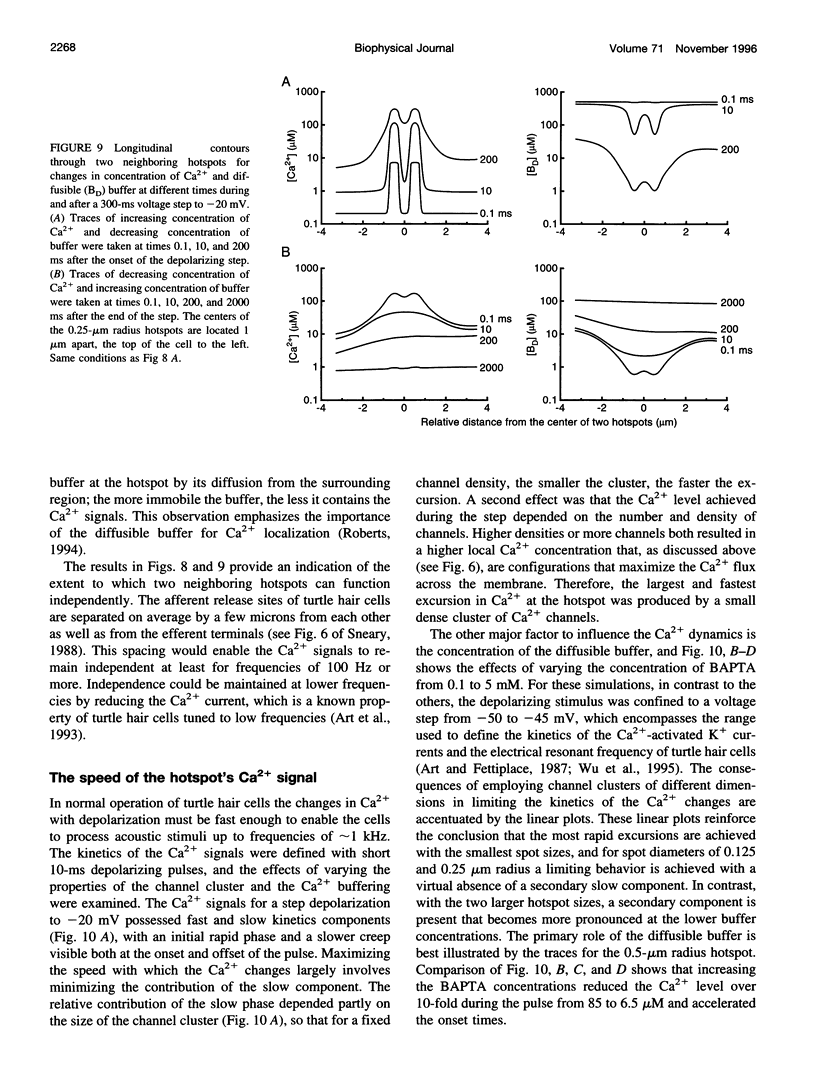
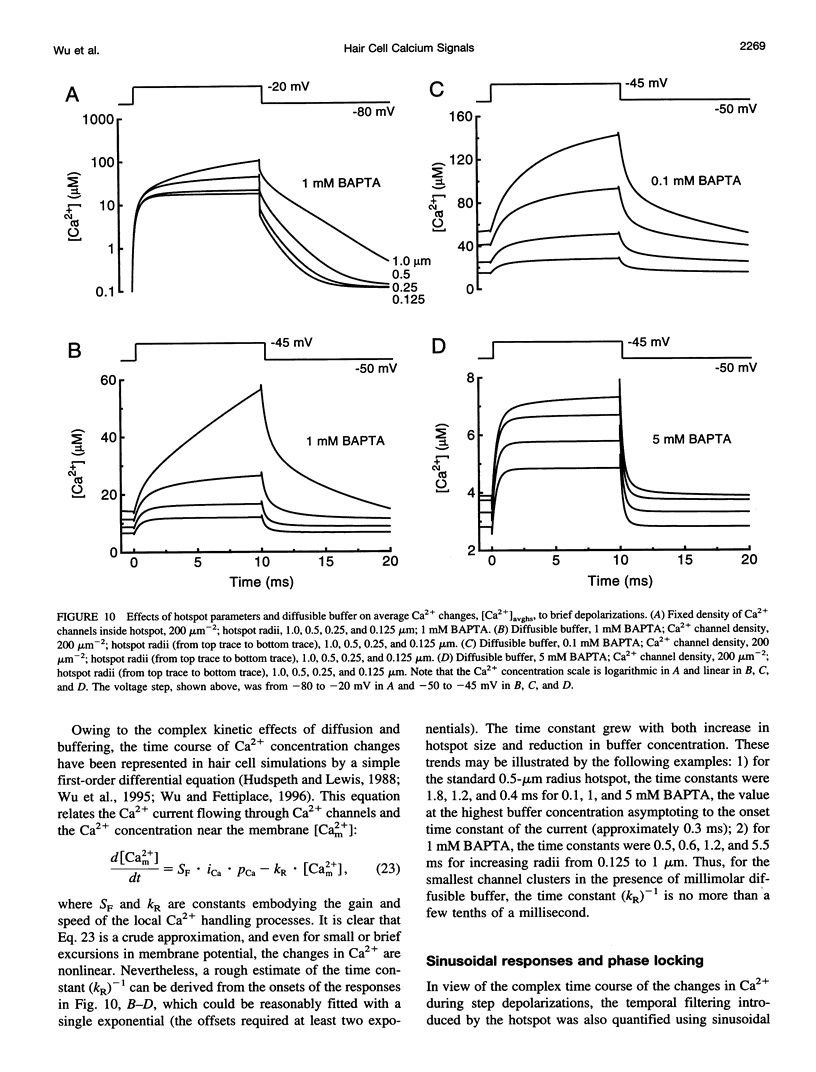
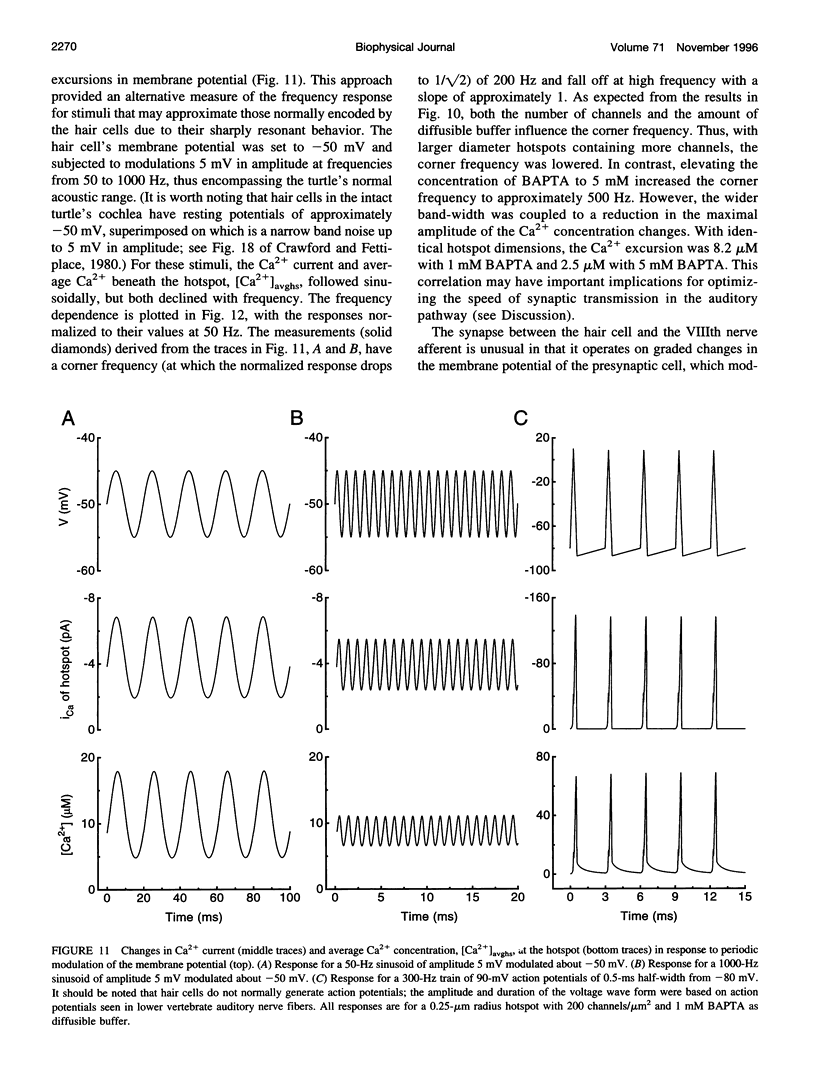
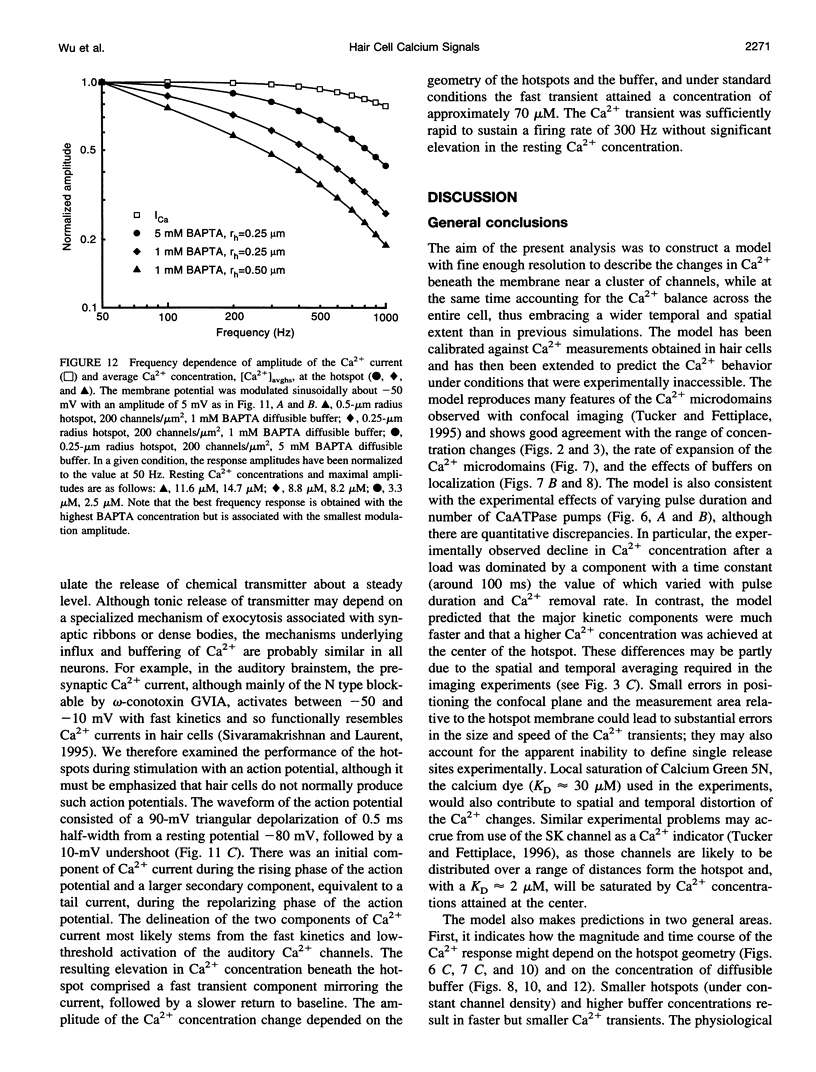
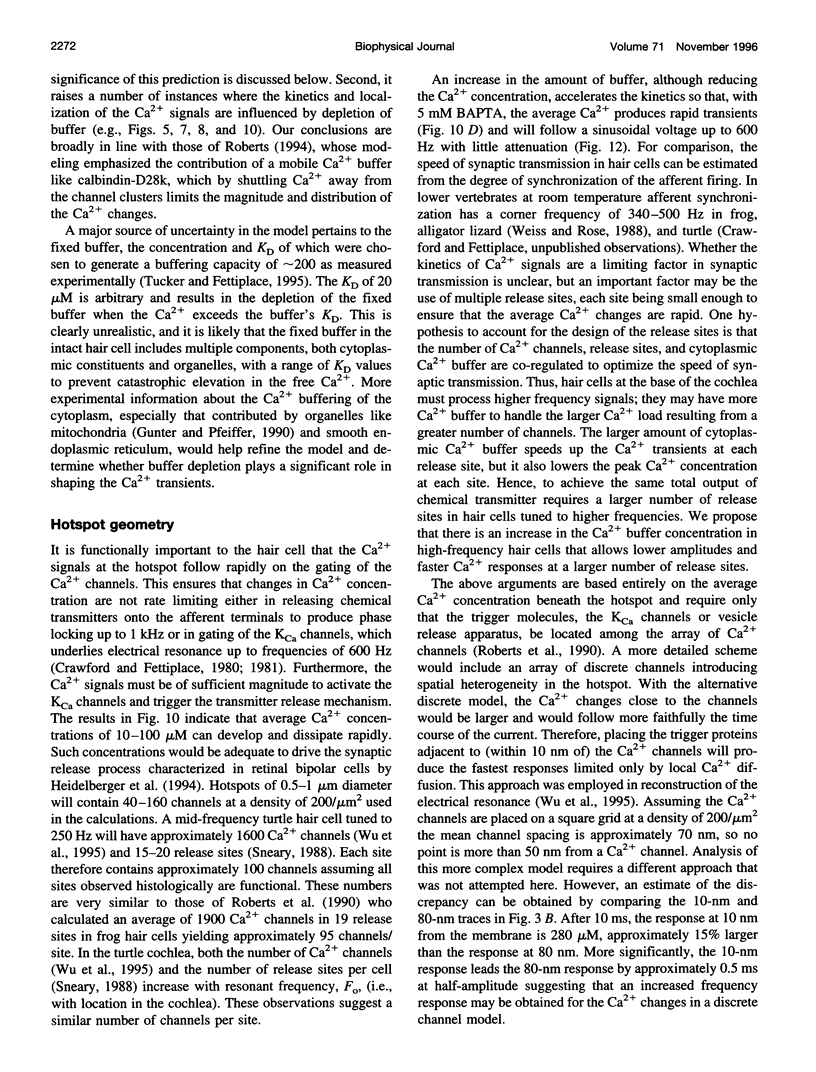
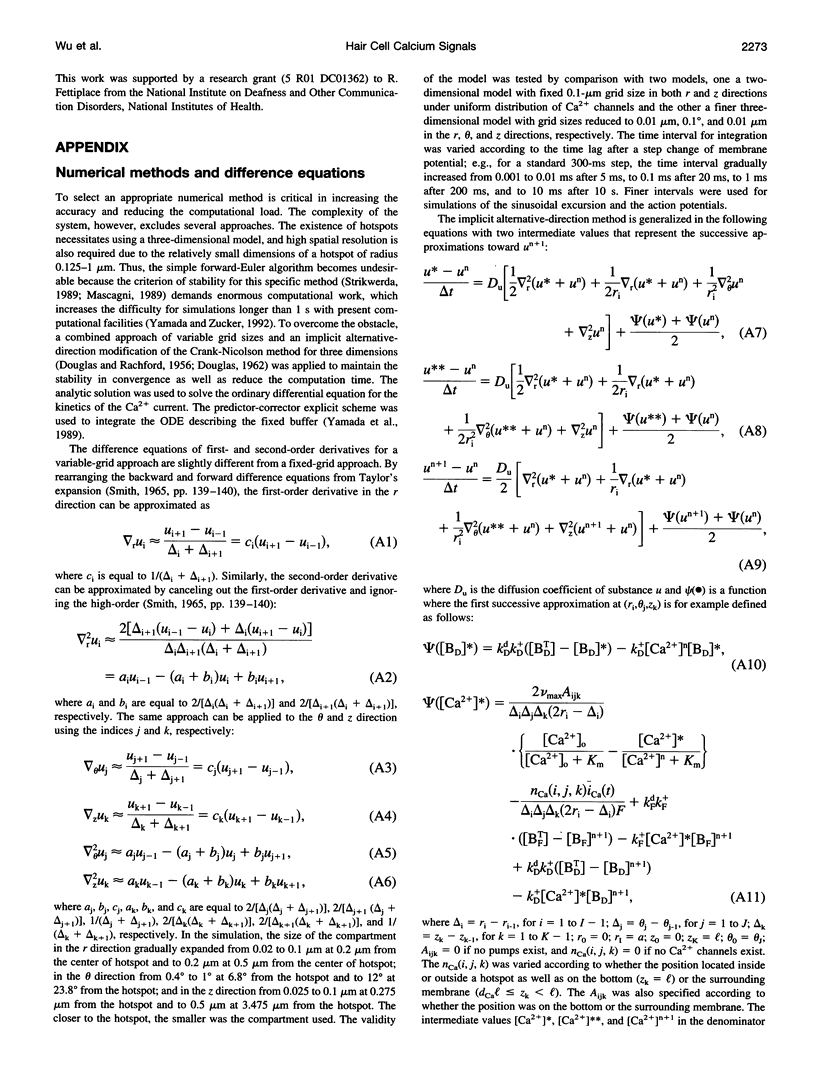
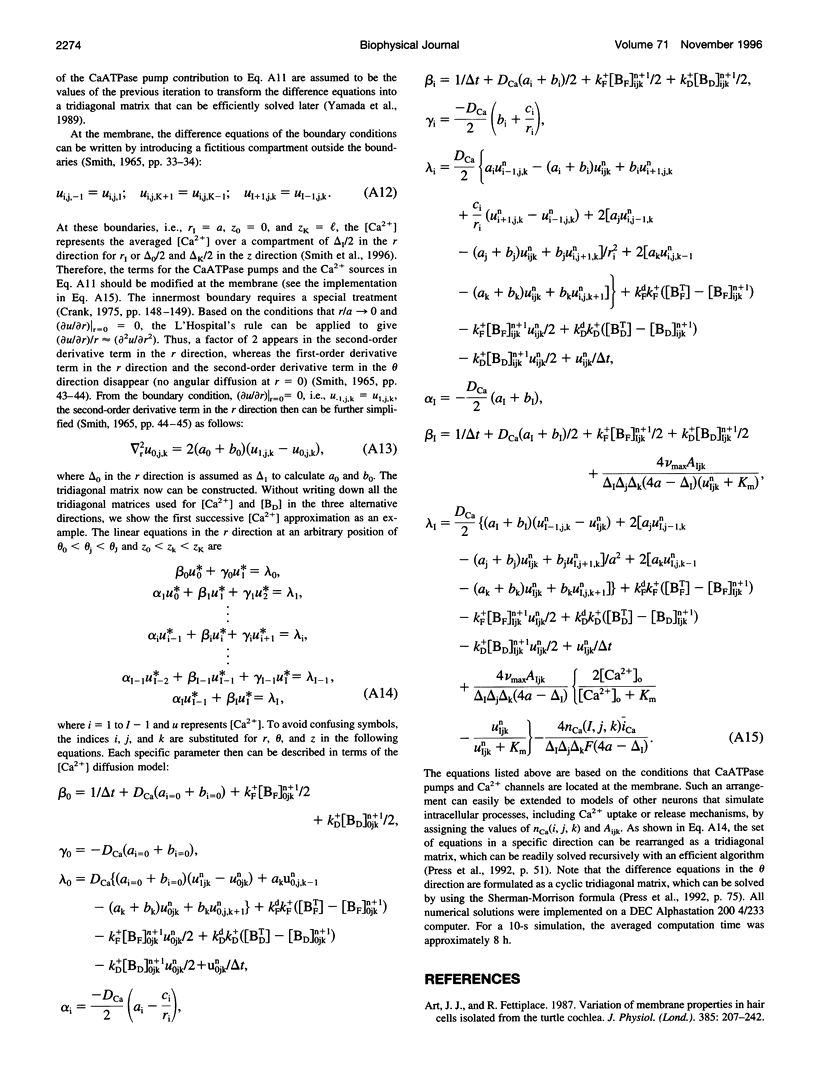
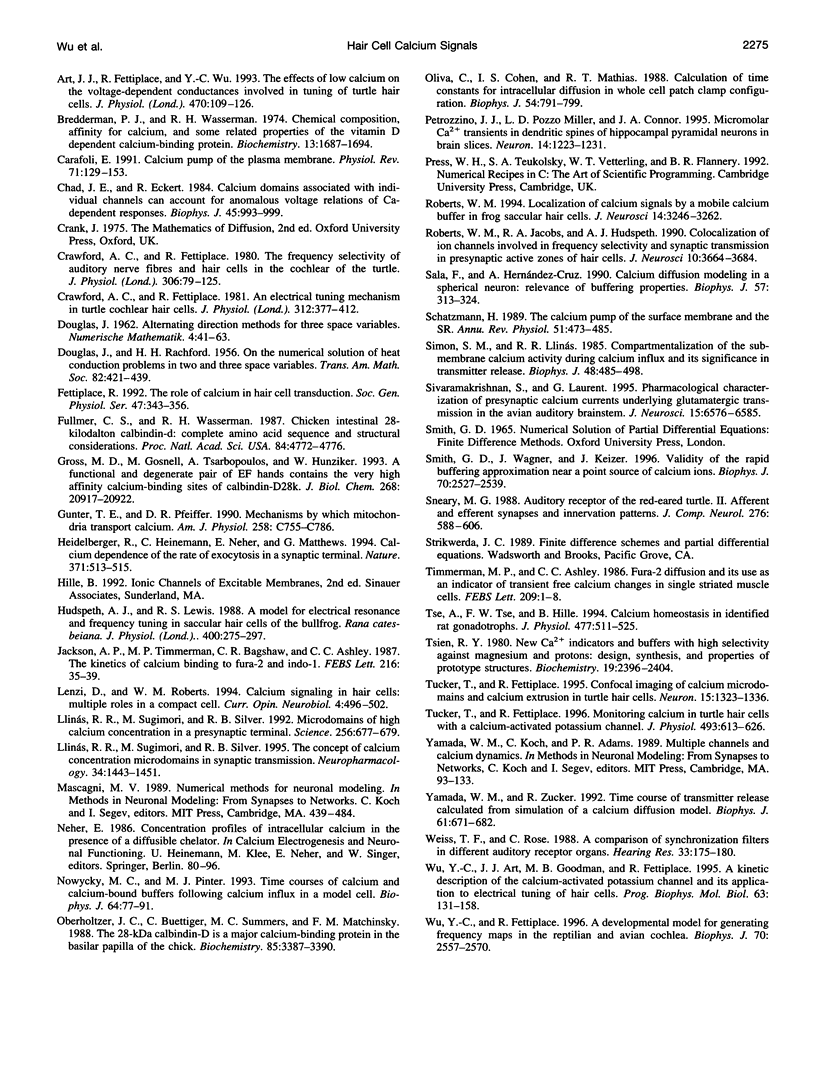
Images in this article
Selected References
These references are in PubMed. This may not be the complete list of references from this article.
- Art J. J., Fettiplace R. Variation of membrane properties in hair cells isolated from the turtle cochlea. J Physiol. 1987 Apr;385:207–242. doi: 10.1113/jphysiol.1987.sp016492. [DOI] [PMC free article] [PubMed] [Google Scholar]
- Art J. J., Fettiplace R., Wu Y. C. The effects of low calcium on the voltage-dependent conductances involved in tuning of turtle hair cells. J Physiol. 1993 Oct;470:109–126. doi: 10.1113/jphysiol.1993.sp019850. [DOI] [PMC free article] [PubMed] [Google Scholar]
- Bredderman P. J., Wasserman R. H. Chemical composition, affinity for calcium, and some related properties of the vitamin D dependent calcium-binding protein. Biochemistry. 1974 Apr 9;13(8):1687–1694. doi: 10.1021/bi00705a021. [DOI] [PubMed] [Google Scholar]
- Carafoli E. Calcium pump of the plasma membrane. Physiol Rev. 1991 Jan;71(1):129–153. doi: 10.1152/physrev.1991.71.1.129. [DOI] [PubMed] [Google Scholar]
- Chad J. E., Eckert R. Calcium domains associated with individual channels can account for anomalous voltage relations of CA-dependent responses. Biophys J. 1984 May;45(5):993–999. doi: 10.1016/S0006-3495(84)84244-7. [DOI] [PMC free article] [PubMed] [Google Scholar]
- Crawford A. C., Fettiplace R. An electrical tuning mechanism in turtle cochlear hair cells. J Physiol. 1981 Mar;312:377–412. doi: 10.1113/jphysiol.1981.sp013634. [DOI] [PMC free article] [PubMed] [Google Scholar]
- Crawford A. C., Fettiplace R. The frequency selectivity of auditory nerve fibres and hair cells in the cochlea of the turtle. J Physiol. 1980 Sep;306:79–125. doi: 10.1113/jphysiol.1980.sp013387. [DOI] [PMC free article] [PubMed] [Google Scholar]
- Fettiplace R. The role of calcium in hair cell transduction. Soc Gen Physiol Ser. 1992;47:343–356. [PubMed] [Google Scholar]
- Fullmer C. S., Wasserman R. H. Chicken intestinal 28-kilodalton calbindin-D: complete amino acid sequence and structural considerations. Proc Natl Acad Sci U S A. 1987 Jul;84(14):4772–4776. doi: 10.1073/pnas.84.14.4772. [DOI] [PMC free article] [PubMed] [Google Scholar]
- Gross M. D., Gosnell M., Tsarbopoulos A., Hunziker W. A functional and degenerate pair of EF hands contains the very high affinity calcium-binding site of calbindin-D28K. J Biol Chem. 1993 Oct 5;268(28):20917–20922. [PubMed] [Google Scholar]
- Gunter T. E., Pfeiffer D. R. Mechanisms by which mitochondria transport calcium. Am J Physiol. 1990 May;258(5 Pt 1):C755–C786. doi: 10.1152/ajpcell.1990.258.5.C755. [DOI] [PubMed] [Google Scholar]
- Heidelberger R., Heinemann C., Neher E., Matthews G. Calcium dependence of the rate of exocytosis in a synaptic terminal. Nature. 1994 Oct 6;371(6497):513–515. doi: 10.1038/371513a0. [DOI] [PubMed] [Google Scholar]
- Hudspeth A. J., Lewis R. S. A model for electrical resonance and frequency tuning in saccular hair cells of the bull-frog, Rana catesbeiana. J Physiol. 1988 Jun;400:275–297. doi: 10.1113/jphysiol.1988.sp017120. [DOI] [PMC free article] [PubMed] [Google Scholar]
- Jackson A. P., Timmerman M. P., Bagshaw C. R., Ashley C. C. The kinetics of calcium binding to fura-2 and indo-1. FEBS Lett. 1987 May 25;216(1):35–39. doi: 10.1016/0014-5793(87)80752-4. [DOI] [PubMed] [Google Scholar]
- Lenzi D., Roberts W. M. Calcium signalling in hair cells: multiple roles in a compact cell. Curr Opin Neurobiol. 1994 Aug;4(4):496–502. doi: 10.1016/0959-4388(94)90049-3. [DOI] [PubMed] [Google Scholar]
- Llinás R., Sugimori M., Silver R. B. Microdomains of high calcium concentration in a presynaptic terminal. Science. 1992 May 1;256(5057):677–679. doi: 10.1126/science.1350109. [DOI] [PubMed] [Google Scholar]
- Llinás R., Sugimori M., Silver R. B. The concept of calcium concentration microdomains in synaptic transmission. Neuropharmacology. 1995 Nov;34(11):1443–1451. doi: 10.1016/0028-3908(95)00150-5. [DOI] [PubMed] [Google Scholar]
- Nowycky M. C., Pinter M. J. Time courses of calcium and calcium-bound buffers following calcium influx in a model cell. Biophys J. 1993 Jan;64(1):77–91. doi: 10.1016/S0006-3495(93)81342-0. [DOI] [PMC free article] [PubMed] [Google Scholar]
- Oberholtzer J. C., Buettger C., Summers M. C., Matschinsky F. M. The 28-kDa calbindin-D is a major calcium-binding protein in the basilar papilla of the chick. Proc Natl Acad Sci U S A. 1988 May;85(10):3387–3390. doi: 10.1073/pnas.85.10.3387. [DOI] [PMC free article] [PubMed] [Google Scholar]
- Oliva C., Cohen I. S., Mathias R. T. Calculation of time constants for intracellular diffusion in whole cell patch clamp configuration. Biophys J. 1988 Nov;54(5):791–799. doi: 10.1016/S0006-3495(88)83017-0. [DOI] [PMC free article] [PubMed] [Google Scholar]
- Petrozzino J. J., Pozzo Miller L. D., Connor J. A. Micromolar Ca2+ transients in dendritic spines of hippocampal pyramidal neurons in brain slice. Neuron. 1995 Jun;14(6):1223–1231. doi: 10.1016/0896-6273(95)90269-4. [DOI] [PubMed] [Google Scholar]
- Roberts W. M., Jacobs R. A., Hudspeth A. J. Colocalization of ion channels involved in frequency selectivity and synaptic transmission at presynaptic active zones of hair cells. J Neurosci. 1990 Nov;10(11):3664–3684. doi: 10.1523/JNEUROSCI.10-11-03664.1990. [DOI] [PMC free article] [PubMed] [Google Scholar]
- Roberts W. M. Localization of calcium signals by a mobile calcium buffer in frog saccular hair cells. J Neurosci. 1994 May;14(5 Pt 2):3246–3262. doi: 10.1523/JNEUROSCI.14-05-03246.1994. [DOI] [PMC free article] [PubMed] [Google Scholar]
- Sala F., Hernández-Cruz A. Calcium diffusion modeling in a spherical neuron. Relevance of buffering properties. Biophys J. 1990 Feb;57(2):313–324. doi: 10.1016/S0006-3495(90)82533-9. [DOI] [PMC free article] [PubMed] [Google Scholar]
- Schatzmann H. J. The calcium pump of the surface membrane and of the sarcoplasmic reticulum. Annu Rev Physiol. 1989;51:473–485. doi: 10.1146/annurev.ph.51.030189.002353. [DOI] [PubMed] [Google Scholar]
- Simon S. M., Llinás R. R. Compartmentalization of the submembrane calcium activity during calcium influx and its significance in transmitter release. Biophys J. 1985 Sep;48(3):485–498. doi: 10.1016/S0006-3495(85)83804-2. [DOI] [PMC free article] [PubMed] [Google Scholar]
- Sivaramakrishnan S., Laurent G. Pharmacological characterization of presynaptic calcium currents underlying glutamatergic transmission in the avian auditory brainstem. J Neurosci. 1995 Oct;15(10):6576–6585. doi: 10.1523/JNEUROSCI.15-10-06576.1995. [DOI] [PMC free article] [PubMed] [Google Scholar]
- Smith G. D., Wagner J., Keizer J. Validity of the rapid buffering approximation near a point source of calcium ions. Biophys J. 1996 Jun;70(6):2527–2539. doi: 10.1016/S0006-3495(96)79824-7. [DOI] [PMC free article] [PubMed] [Google Scholar]
- Sneary M. G. Auditory receptor of the red-eared turtle: II. Afferent and efferent synapses and innervation patterns. J Comp Neurol. 1988 Oct 22;276(4):588–606. doi: 10.1002/cne.902760411. [DOI] [PubMed] [Google Scholar]
- Timmerman M. P., Ashley C. C. Fura-2 diffusion and its use as an indicator of transient free calcium changes in single striated muscle cells. FEBS Lett. 1986 Dec 1;209(1):1–8. doi: 10.1016/0014-5793(86)81073-0. [DOI] [PubMed] [Google Scholar]
- Tse A., Tse F. W., Hille B. Calcium homeostasis in identified rat gonadotrophs. J Physiol. 1994 Jun 15;477(Pt 3):511–525. doi: 10.1113/jphysiol.1994.sp020212. [DOI] [PMC free article] [PubMed] [Google Scholar]
- Tsien R. Y. New calcium indicators and buffers with high selectivity against magnesium and protons: design, synthesis, and properties of prototype structures. Biochemistry. 1980 May 27;19(11):2396–2404. doi: 10.1021/bi00552a018. [DOI] [PubMed] [Google Scholar]
- Tucker T. R., Fettiplace R. Monitoring calcium in turtle hair cells with a calcium-activated potassium channel. J Physiol. 1996 Aug 1;494(Pt 3):613–626. doi: 10.1113/jphysiol.1996.sp021519. [DOI] [PMC free article] [PubMed] [Google Scholar]
- Tucker T., Fettiplace R. Confocal imaging of calcium microdomains and calcium extrusion in turtle hair cells. Neuron. 1995 Dec;15(6):1323–1335. doi: 10.1016/0896-6273(95)90011-x. [DOI] [PubMed] [Google Scholar]
- Weiss T. F., Rose C. A comparison of synchronization filters in different auditory receptor organs. Hear Res. 1988 May;33(2):175–179. doi: 10.1016/0378-5955(88)90030-5. [DOI] [PubMed] [Google Scholar]
- Wu Y. C., Art J. J., Goodman M. B., Fettiplace R. A kinetic description of the calcium-activated potassium channel and its application to electrical tuning of hair cells. Prog Biophys Mol Biol. 1995;63(2):131–158. doi: 10.1016/0079-6107(95)00002-5. [DOI] [PubMed] [Google Scholar]
- Wu Y. C., Fettiplace R. A developmental model for generating frequency maps in the reptilian and avian cochleas. Biophys J. 1996 Jun;70(6):2557–2570. doi: 10.1016/S0006-3495(96)79827-2. [DOI] [PMC free article] [PubMed] [Google Scholar]
- Yamada W. M., Zucker R. S. Time course of transmitter release calculated from simulations of a calcium diffusion model. Biophys J. 1992 Mar;61(3):671–682. doi: 10.1016/S0006-3495(92)81872-6. [DOI] [PMC free article] [PubMed] [Google Scholar]