Abstract
The human Na(+)-glucose cotransporter (hSGLT1) has been shown to generate, in the absence of sugar, presteady-state currents in response to a change in potential, which could be fitted with single exponentials once the voltage had reached a new constant value. By the cut-open oocyte technique (voltage rising-speed approximately 1 mV/microsecond), phlorizin-sensitive transient currents could be detected with a higher time resolution during continuous intracellular perfusion. In the absence of sugar and internal Na+, and with 90 mM external Na+ concentration ([Na+]o), phlorizin-sensitive currents exhibited two relaxation time-constants: tau 1 increased from 2 to 10 ms when Vm decreased from +60 mV to -80 mV and remained at 10 ms for more negative Vm; tau 2 ranged from 0.4 to 0.8 ms in a weakly voltage-dependent manner. According to a previously proposed model, these two time constants could be accounted for by 1) Na+ crossing a fraction of the membrane electrical field to reach its binding site on the carrier and 2) conformational change of the free carrier. To test this hypothesis, the time constants were measured as [Na+]o was progressively reduced to 0 mM. At 30 and 10 mM external Na+, tau 1 reached the same plateau value of 10 ms but at more negative potentials (-120 and -160 mV, respectively). Contrary to the prediction of the model, two time constants continued to be detected in the bilateral absence of Na+ (at pH 8.0). Under these conditions, tau 1 continuously increased through the whole voltage range and did not reach the 10 ms level even when Vm had attained -200 mV while tau 2 remained in the range of 0.4-0.8 ms. These results indicate that 1) conformational change of the free carrier across the membrane must occur in more than one step and 2) Na+ binding/debinding is not responsible for either of the two observed exponential components of transient currents. By use of the simplest kinetic model accounting for the portion of the hSGLT1 transport cycle involving extracellular Na+ binding/debinding and the dual-step conformational change of the free carrier, tau 1 and tau 2 were fitted throughout the voltage range, and a few sets of parameters were found to reproduce the data satisfactorily. This study shows that 1) tau 1 and tau 2 correspond to two steps in the conformational change of the free carrier, 2) Na+ binding/debinding modulates the slow time constant (tau 1) and 3) a voltage-independent slow conformational change of the free carrier accounts for the observed plateau value of 10 ms.
Full text
PDF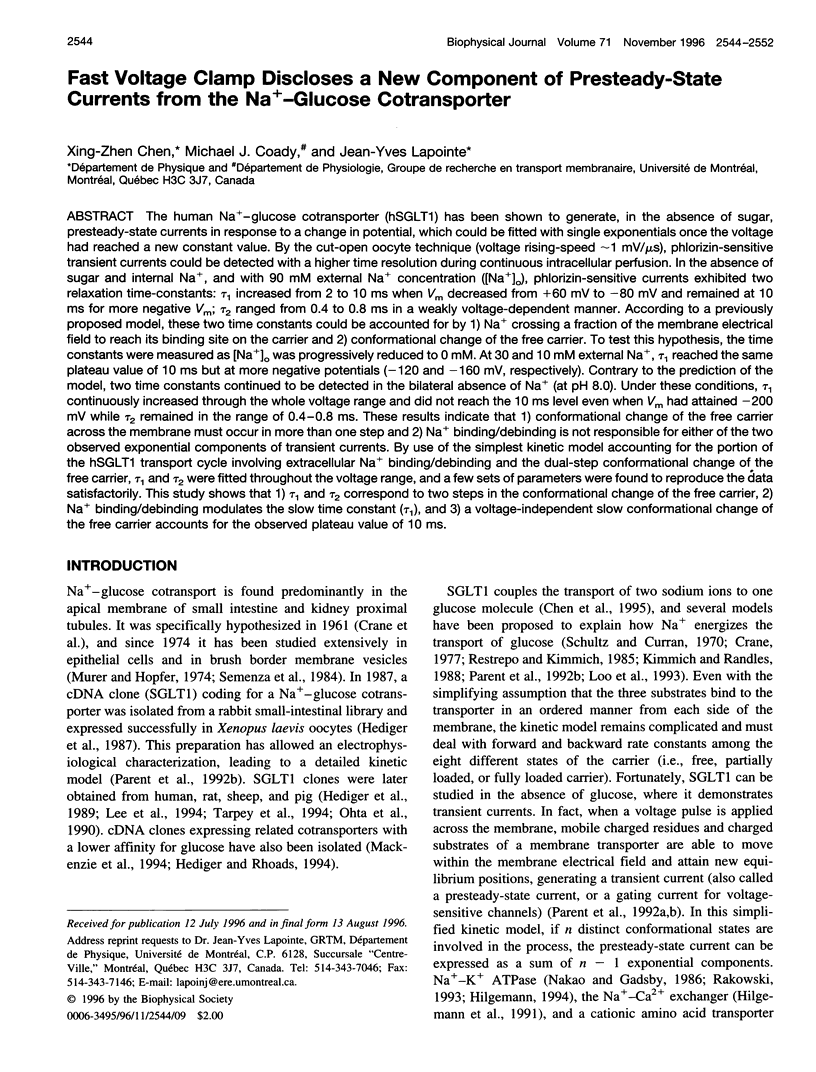
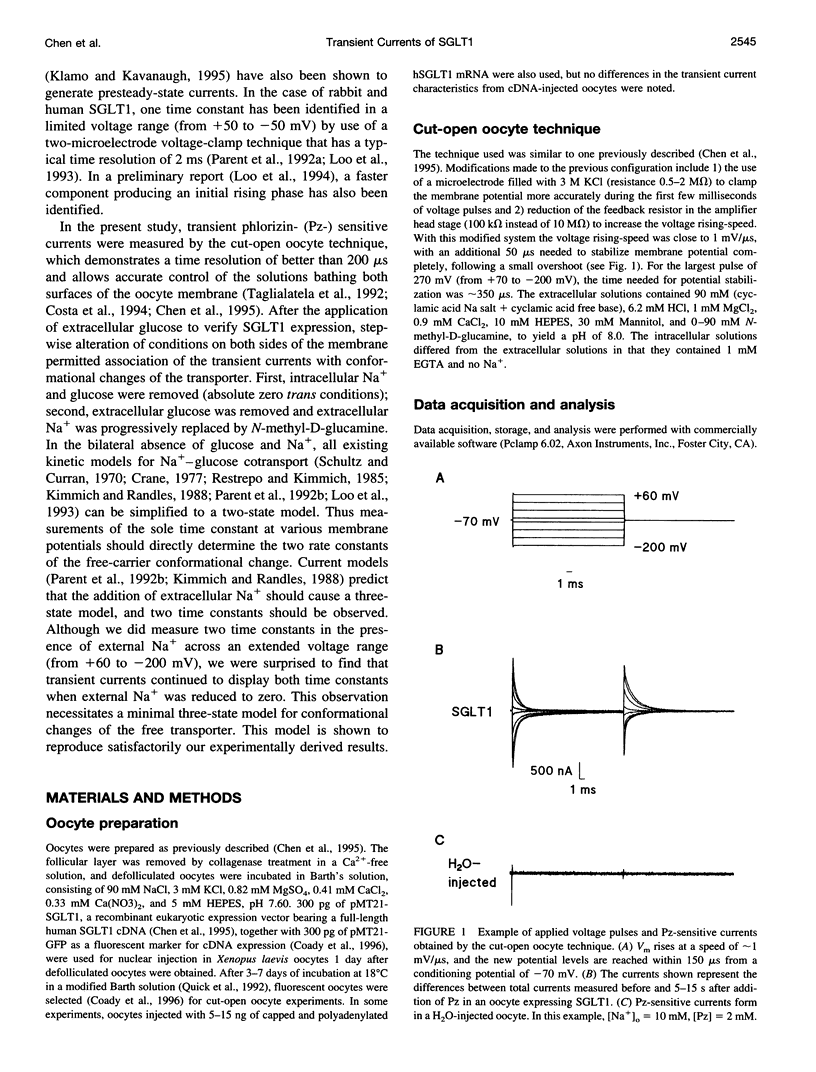
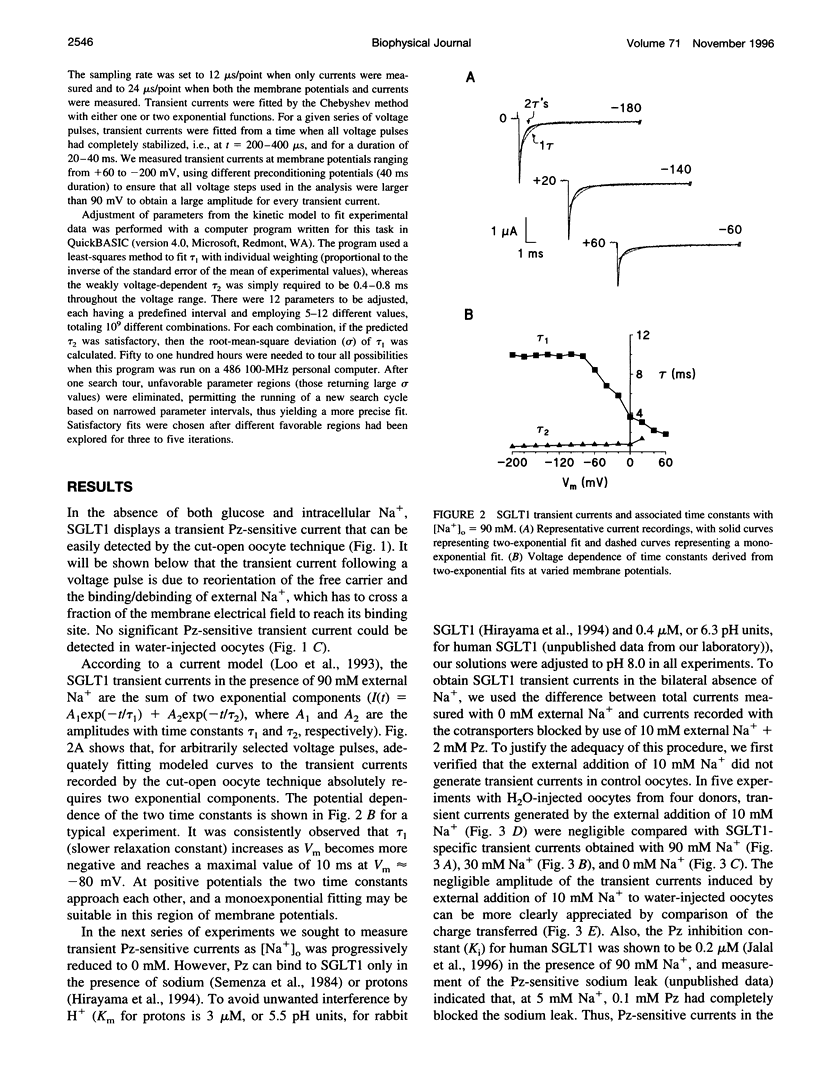
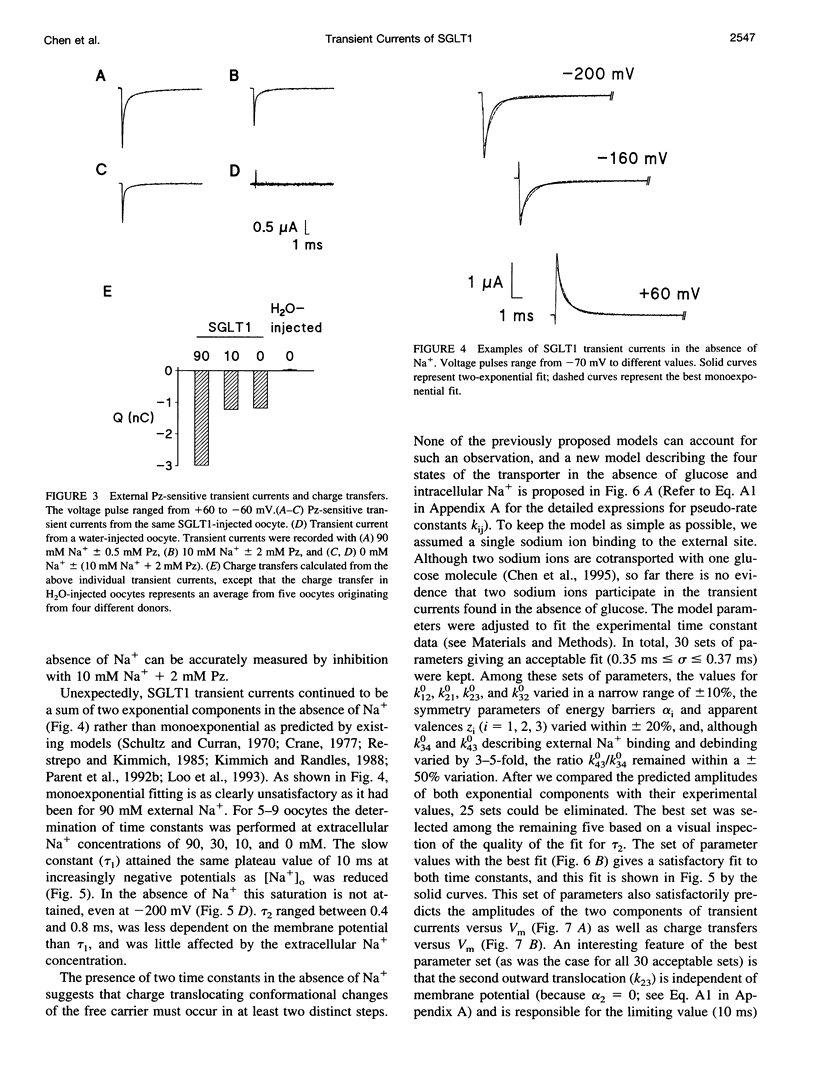
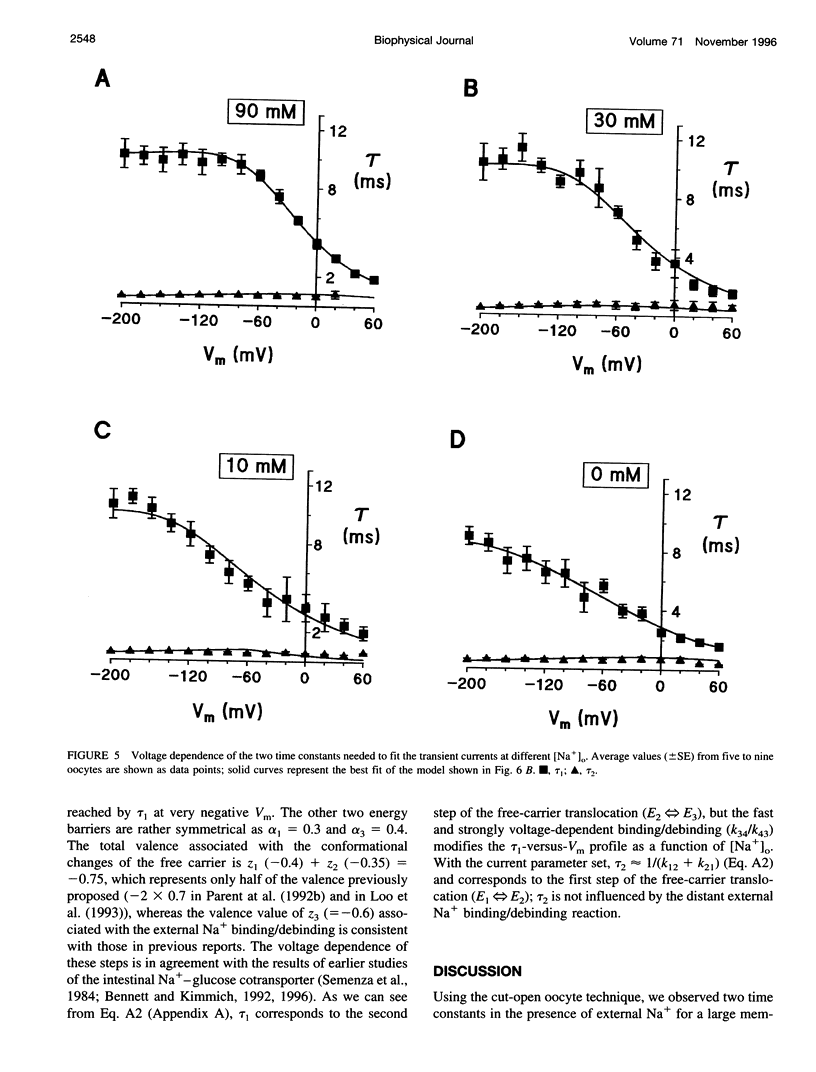
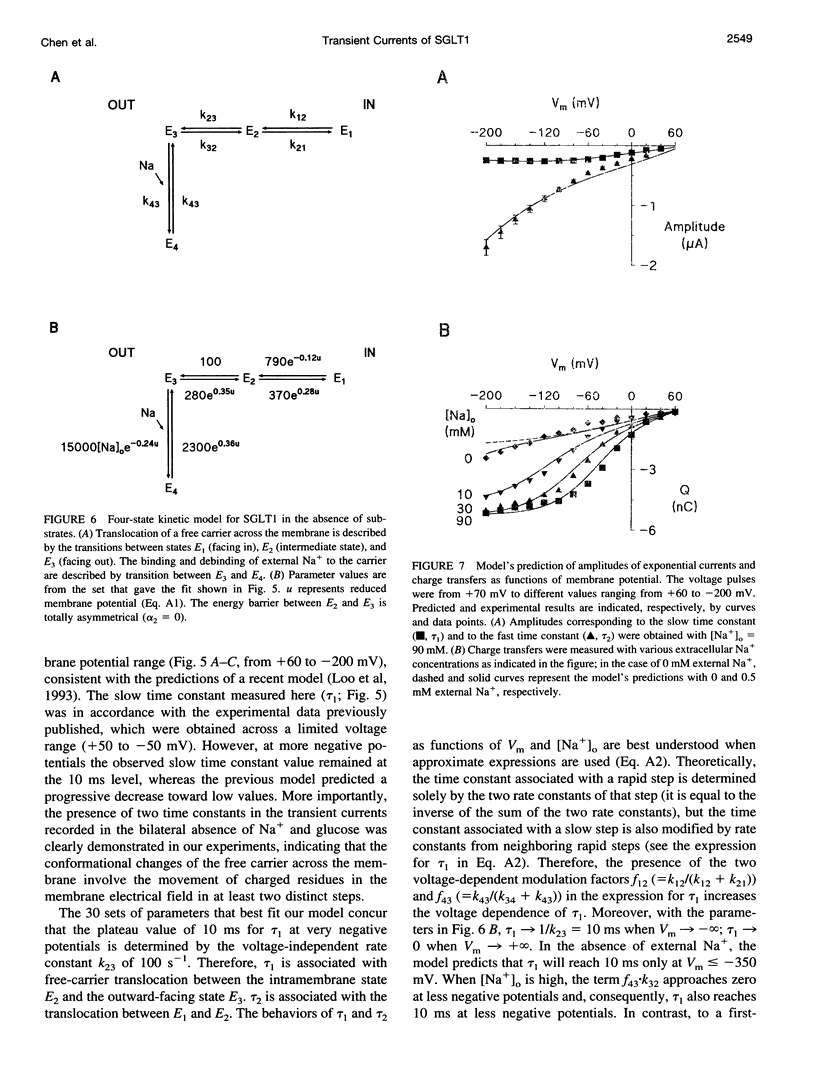
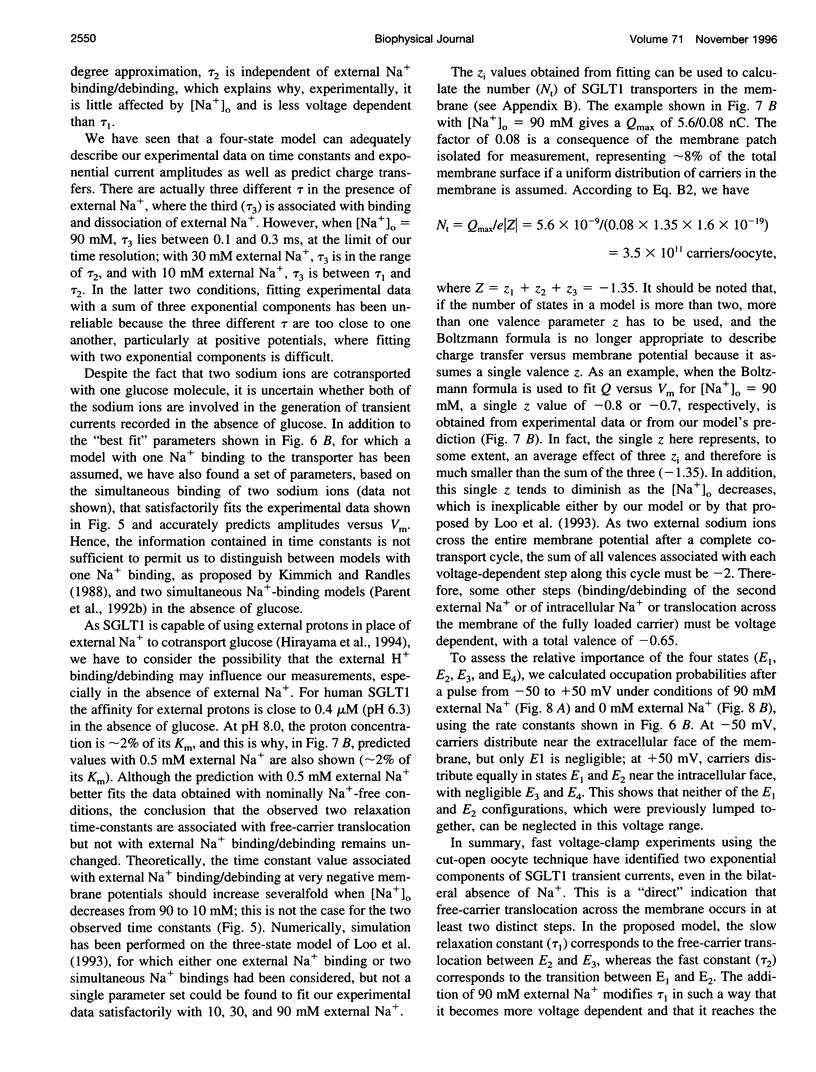
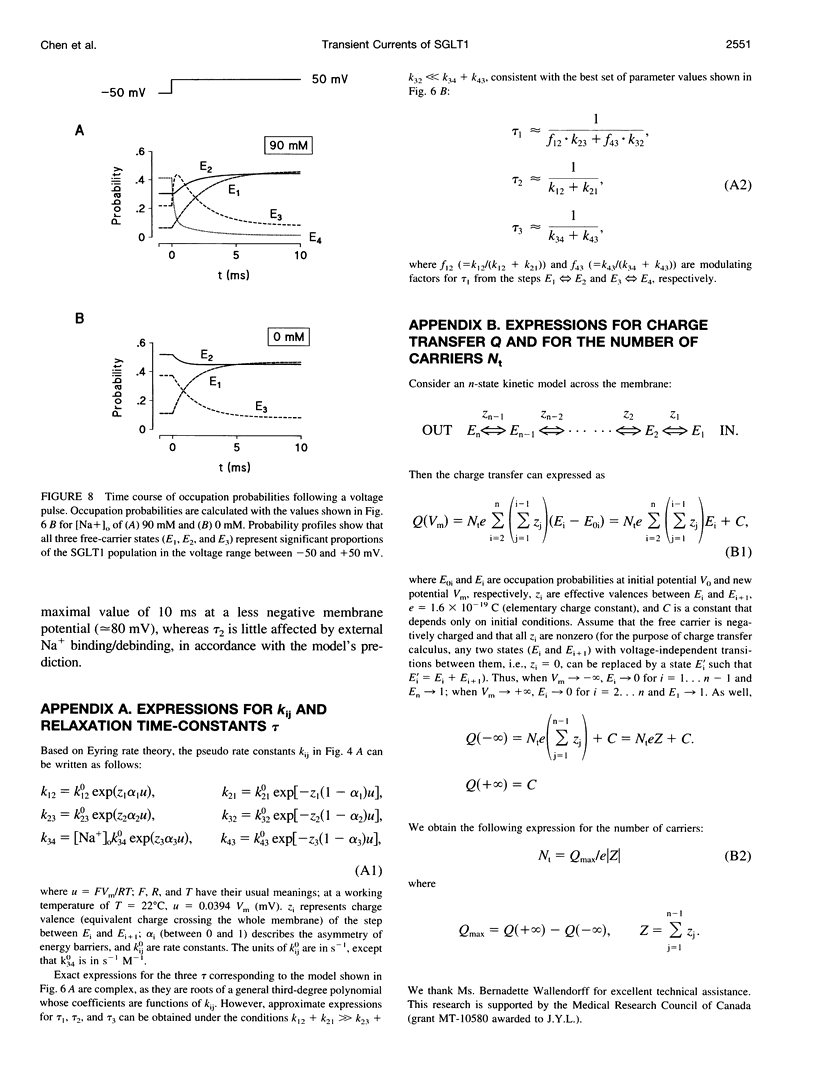
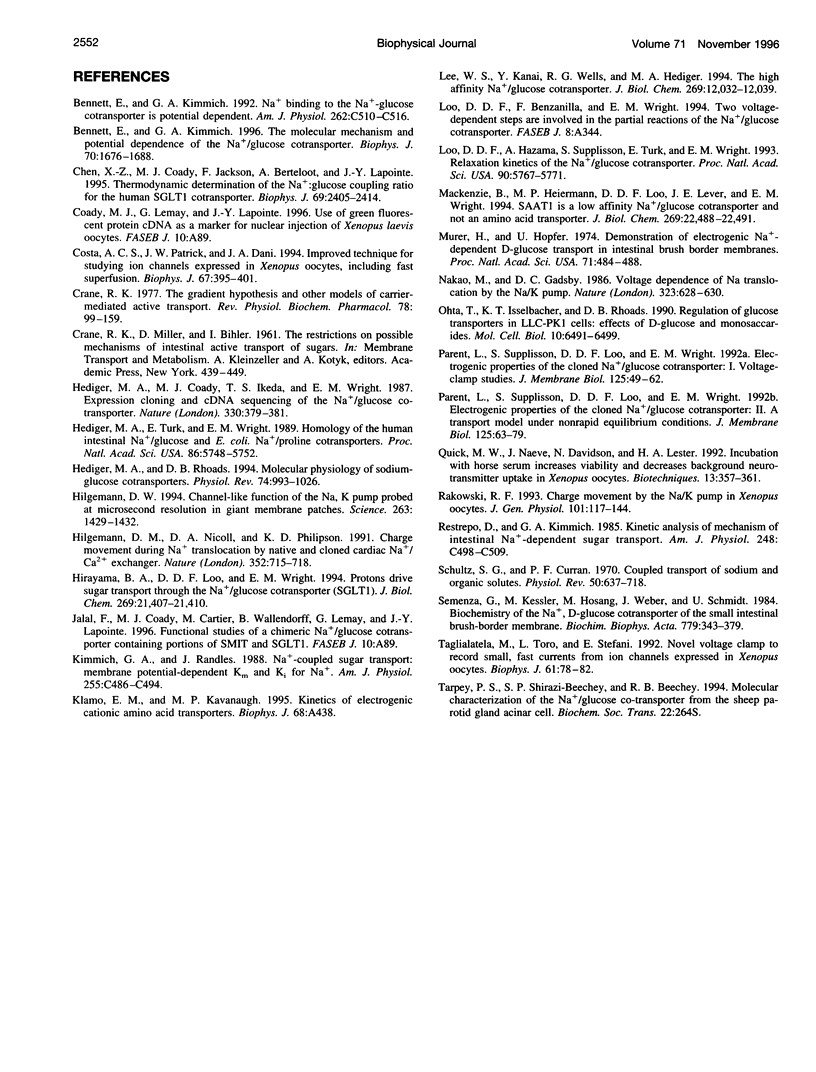
Images in this article
Selected References
These references are in PubMed. This may not be the complete list of references from this article.
- Bennett E., Kimmich G. A. Na+ binding to the Na(+)-glucose cotransporter is potential dependent. Am J Physiol. 1992 Feb;262(2 Pt 1):C510–C516. doi: 10.1152/ajpcell.1992.262.2.C510. [DOI] [PubMed] [Google Scholar]
- Bennett E., Kimmich G. A. The molecular mechanism and potential dependence of the Na+/glucose cotransporter. Biophys J. 1996 Apr;70(4):1676–1688. doi: 10.1016/S0006-3495(96)79730-8. [DOI] [PMC free article] [PubMed] [Google Scholar]
- Chen X. Z., Coady M. J., Jackson F., Berteloot A., Lapointe J. Y. Thermodynamic determination of the Na+: glucose coupling ratio for the human SGLT1 cotransporter. Biophys J. 1995 Dec;69(6):2405–2414. doi: 10.1016/S0006-3495(95)80110-4. [DOI] [PMC free article] [PubMed] [Google Scholar]
- Costa A. C., Patrick J. W., Dani J. A. Improved technique for studying ion channels expressed in Xenopus oocytes, including fast superfusion. Biophys J. 1994 Jul;67(1):395–401. doi: 10.1016/S0006-3495(94)80494-1. [DOI] [PMC free article] [PubMed] [Google Scholar]
- Crane R. K. The gradient hypothesis and other models of carrier-mediated active transport. Rev Physiol Biochem Pharmacol. 1977;78:99–159. doi: 10.1007/BFb0027722. [DOI] [PubMed] [Google Scholar]
- Hediger M. A., Coady M. J., Ikeda T. S., Wright E. M. Expression cloning and cDNA sequencing of the Na+/glucose co-transporter. 1987 Nov 26-Dec 2Nature. 330(6146):379–381. doi: 10.1038/330379a0. [DOI] [PubMed] [Google Scholar]
- Hediger M. A., Rhoads D. B. Molecular physiology of sodium-glucose cotransporters. Physiol Rev. 1994 Oct;74(4):993–1026. doi: 10.1152/physrev.1994.74.4.993. [DOI] [PubMed] [Google Scholar]
- Hediger M. A., Turk E., Wright E. M. Homology of the human intestinal Na+/glucose and Escherichia coli Na+/proline cotransporters. Proc Natl Acad Sci U S A. 1989 Aug;86(15):5748–5752. doi: 10.1073/pnas.86.15.5748. [DOI] [PMC free article] [PubMed] [Google Scholar]
- Hilgemann D. W. Channel-like function of the Na,K pump probed at microsecond resolution in giant membrane patches. Science. 1994 Mar 11;263(5152):1429–1432. doi: 10.1126/science.8128223. [DOI] [PubMed] [Google Scholar]
- Hilgemann D. W., Nicoll D. A., Philipson K. D. Charge movement during Na+ translocation by native and cloned cardiac Na+/Ca2+ exchanger. Nature. 1991 Aug 22;352(6337):715–718. doi: 10.1038/352715a0. [DOI] [PubMed] [Google Scholar]
- Kimmich G. A., Randles J. Na+-coupled sugar transport: membrane potential-dependent Km and Ki for Na+. Am J Physiol. 1988 Oct;255(4 Pt 1):C486–C494. doi: 10.1152/ajpcell.1988.255.4.C486. [DOI] [PubMed] [Google Scholar]
- Loo D. D., Hazama A., Supplisson S., Turk E., Wright E. M. Relaxation kinetics of the Na+/glucose cotransporter. Proc Natl Acad Sci U S A. 1993 Jun 15;90(12):5767–5771. doi: 10.1073/pnas.90.12.5767. [DOI] [PMC free article] [PubMed] [Google Scholar]
- Murer H., Hopfer U. Demonstration of electrogenic Na+-dependent D-glucose transport in intestinal brush border membranes. Proc Natl Acad Sci U S A. 1974 Feb;71(2):484–488. doi: 10.1073/pnas.71.2.484. [DOI] [PMC free article] [PubMed] [Google Scholar]
- Nakao M., Gadsby D. C. Voltage dependence of Na translocation by the Na/K pump. Nature. 1986 Oct 16;323(6089):628–630. doi: 10.1038/323628a0. [DOI] [PubMed] [Google Scholar]
- Ohta T., Isselbacher K. J., Rhoads D. B. Regulation of glucose transporters in LLC-PK1 cells: effects of D-glucose and monosaccharides. Mol Cell Biol. 1990 Dec;10(12):6491–6499. doi: 10.1128/mcb.10.12.6491. [DOI] [PMC free article] [PubMed] [Google Scholar]
- Parent L., Supplisson S., Loo D. D., Wright E. M. Electrogenic properties of the cloned Na+/glucose cotransporter: I. Voltage-clamp studies. J Membr Biol. 1992 Jan;125(1):49–62. doi: 10.1007/BF00235797. [DOI] [PubMed] [Google Scholar]
- Parent L., Supplisson S., Loo D. D., Wright E. M. Electrogenic properties of the cloned Na+/glucose cotransporter: II. A transport model under nonrapid equilibrium conditions. J Membr Biol. 1992 Jan;125(1):63–79. doi: 10.1007/BF00235798. [DOI] [PubMed] [Google Scholar]
- Quick M. W., Naeve J., Davidson N., Lester H. A. Incubation with horse serum increases viability and decreases background neurotransmitter uptake in Xenopus oocytes. Biotechniques. 1992 Sep;13(3):357–361. [PubMed] [Google Scholar]
- Rakowski R. F. Charge movement by the Na/K pump in Xenopus oocytes. J Gen Physiol. 1993 Jan;101(1):117–144. doi: 10.1085/jgp.101.1.117. [DOI] [PMC free article] [PubMed] [Google Scholar]
- Restrepo D., Kimmich G. A. Kinetic analysis of mechanism of intestinal Na+-dependent sugar transport. Am J Physiol. 1985 May;248(5 Pt 1):C498–C509. doi: 10.1152/ajpcell.1985.248.5.C498. [DOI] [PubMed] [Google Scholar]
- Schultz S. G., Curran P. F. Coupled transport of sodium and organic solutes. Physiol Rev. 1970 Oct;50(4):637–718. doi: 10.1152/physrev.1970.50.4.637. [DOI] [PubMed] [Google Scholar]
- Semenza G., Kessler M., Hosang M., Weber J., Schmidt U. Biochemistry of the Na+, D-glucose cotransporter of the small-intestinal brush-border membrane. The state of the art in 1984. Biochim Biophys Acta. 1984 Sep 3;779(3):343–379. doi: 10.1016/0304-4157(84)90016-9. [DOI] [PubMed] [Google Scholar]
- Taglialatela M., Toro L., Stefani E. Novel voltage clamp to record small, fast currents from ion channels expressed in Xenopus oocytes. Biophys J. 1992 Jan;61(1):78–82. doi: 10.1016/S0006-3495(92)81817-9. [DOI] [PMC free article] [PubMed] [Google Scholar]
- Tarpey P. S., Shirazi-Beechey S. P., Beechey R. B. Molecular characterisation of the Na+/glucose co-transporter from the sheep parotid gland acinar cell. Biochem Soc Trans. 1994 Aug;22(3):264S–264S. doi: 10.1042/bst022264s. [DOI] [PubMed] [Google Scholar]