Abstract
The effect of a nontoxic, nonionic block co-polymeric surface active agent, poloxamer 188, on electroporation of artificial lipid membranes made of azolectin, was investigated. Two different experimental protocols were used in our study: charge pulse and voltage clamp. For the charge pulse protocol, membranes were pulsed with a 10-micronsecond rectangular voltage waveform, after which membrane voltage decay was observed through an external 1-M omega resistance. For the voltage clamp protocol the membranes were pulsed with a waveform that consisted of an initial 10-microsecond rectangular phase, followed by a negative sloped ramp that decayed to zero in the subsequent 500 microseconds. Several parameters characterizing the electroporation process were measured and compared for the control membranes and membranes treated with 1.0 mM poloxamer 188. For both the charge pulse and voltage clamp experiments, the threshold voltage (amplitude of initial rectangular phase) and latency time (time elapsed between the end of rectangular phase and the onset of membrane electroporation) were measured. Membrane conductance (measured 200 microseconds after the initial rectangular phase) and rise time (tr; the time required for the porated membrane to reach a certain conductance value) were also determined for the voltage clamp experiments, and postelectroporation time constant (PE tau; the time constant for transmembrane voltage decay after onset of electroporation) for the charge pulse experiments. The charge pulse experiments were performed on 23 membranes with 10 control and 13 poloxamer-treated membranes, and voltage pulse experiments on 49 membranes with 26 control and 23 poloxamer-treated membranes. For both charge pulse and voltage clamp experiments, poloxamer 188-treated membranes exhibited a statistically higher threshold voltage (p = 0.1 and p = 0.06, respectively), and longer latency time (p = 0.04 and p = 0.05, respectively). Also, poloxamer 188-treated membranes were found to have a relatively lower conductance (p = 0.001), longer time required for the porated membrane to reach a certain conductance value (p = 0.05), and longer postelectroporation time constant (p = 0.005). Furthermore, addition of poloxamer 188 was found to reduce the membrane capacitance by approximately 4-8% in 5 min. These findings suggest that poloxamer 188 adsorbs into the lipid bilayers, thereby decreasing their susceptibility to electroporation.
Full text
PDF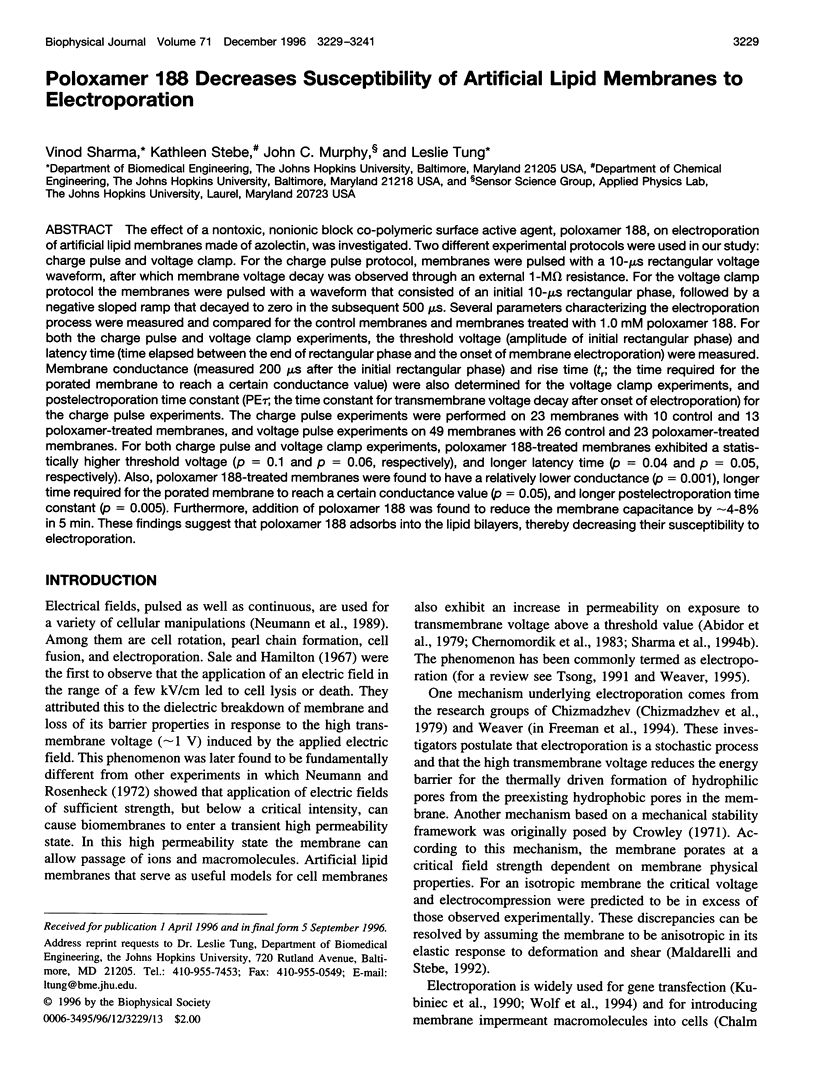
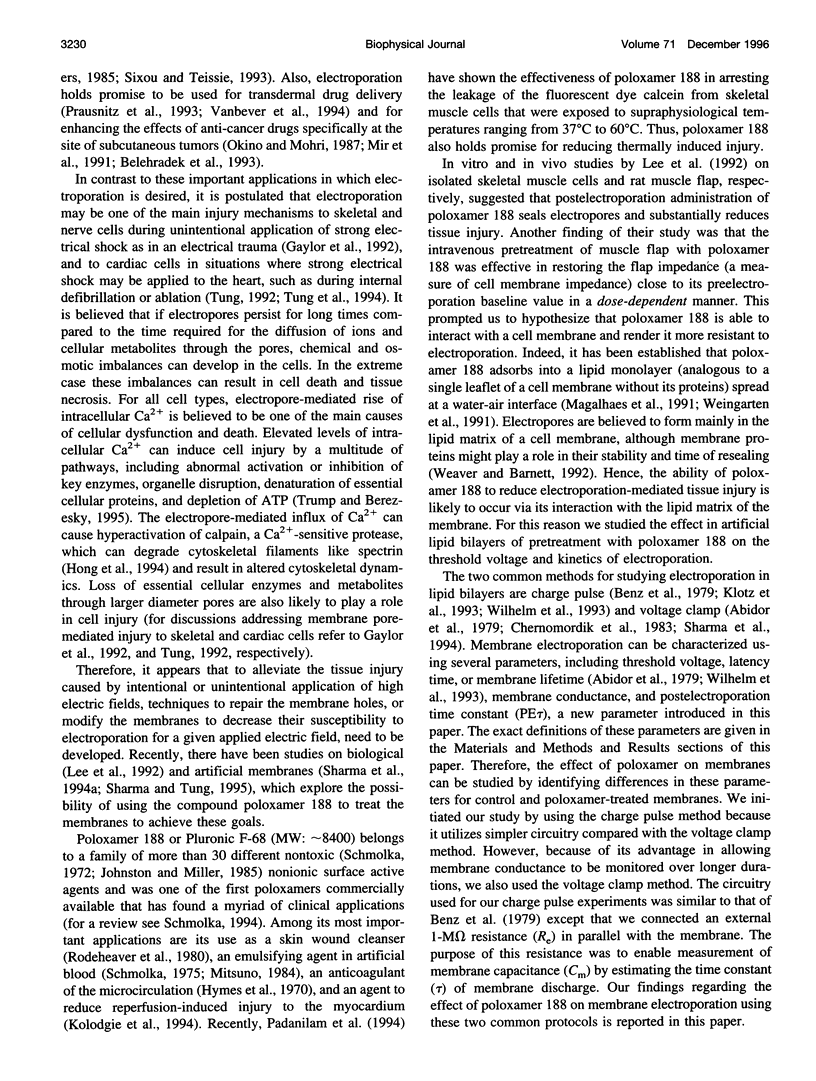
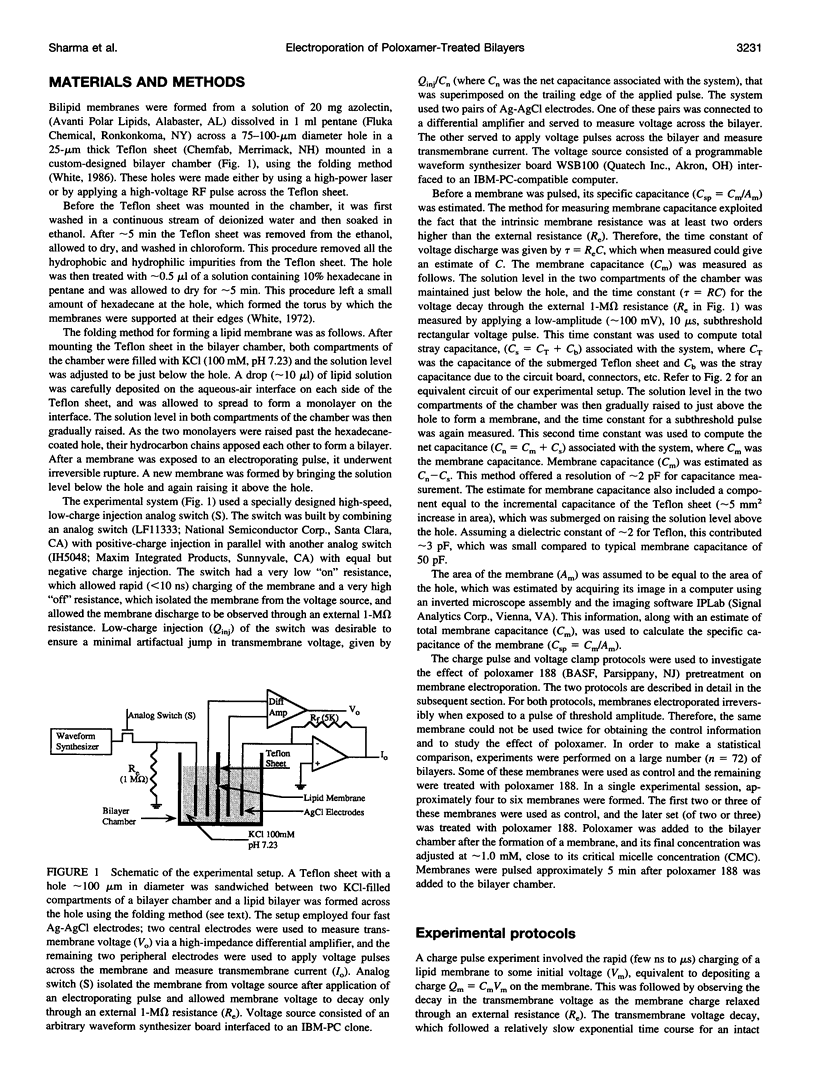
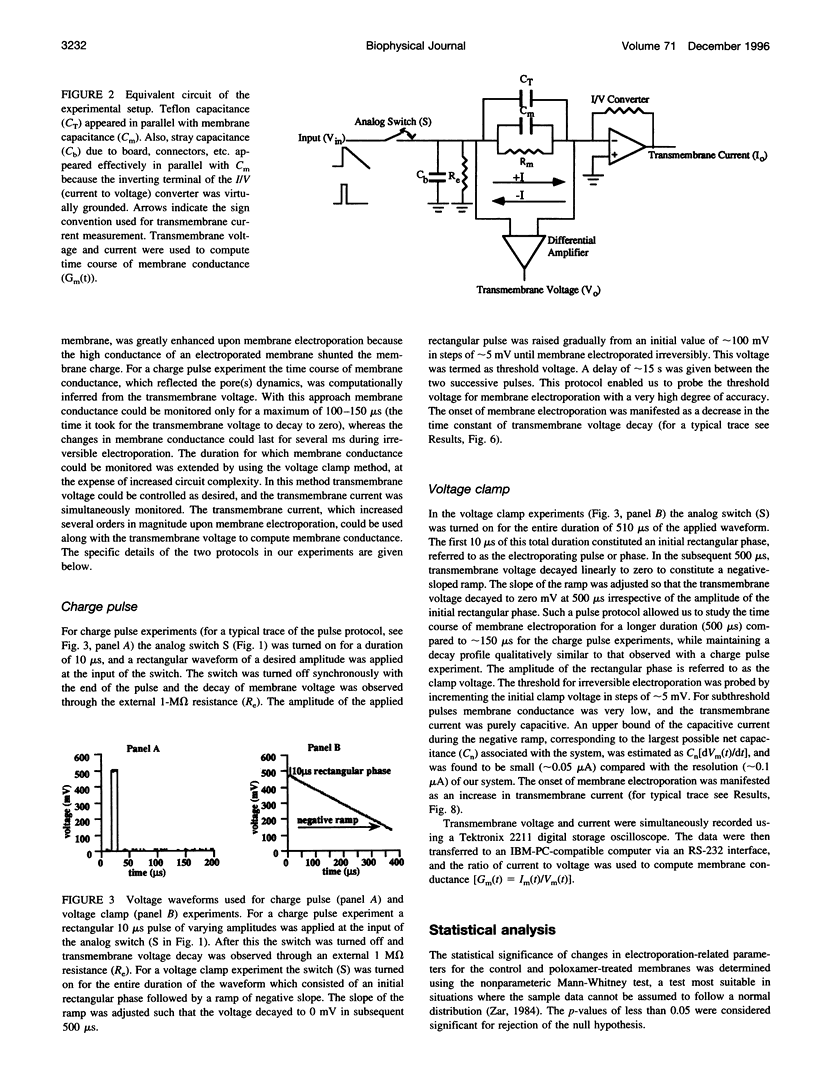
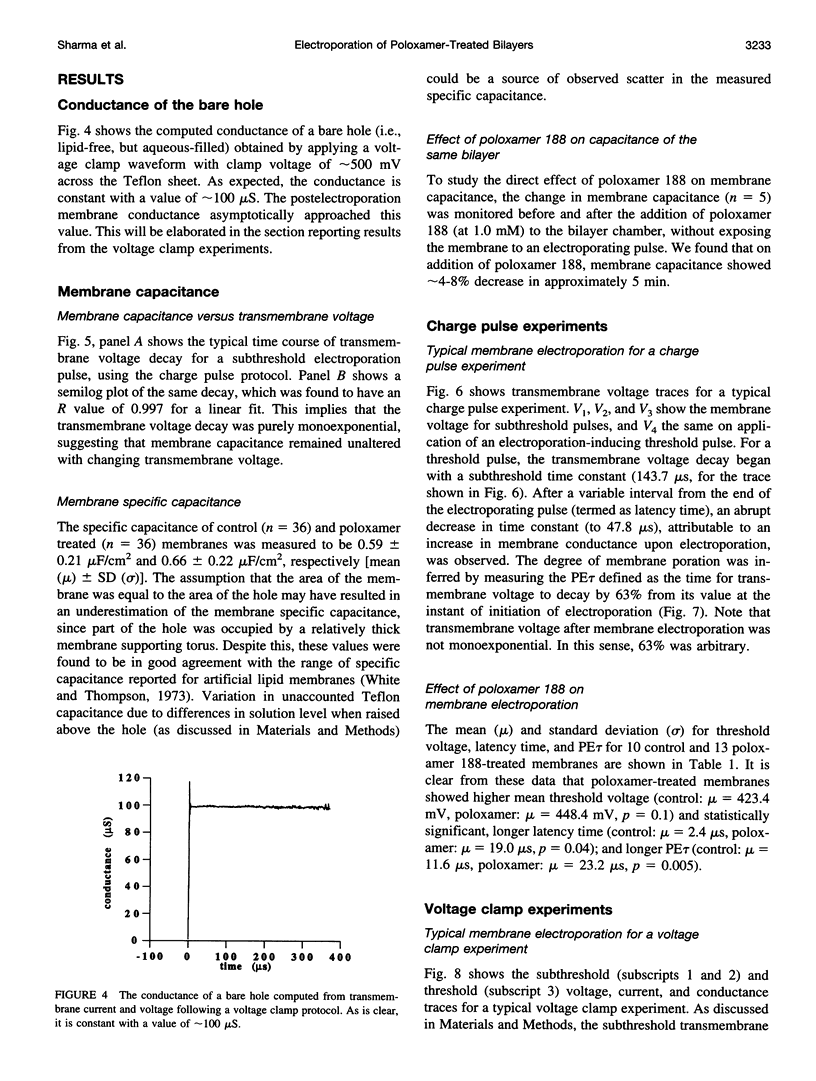
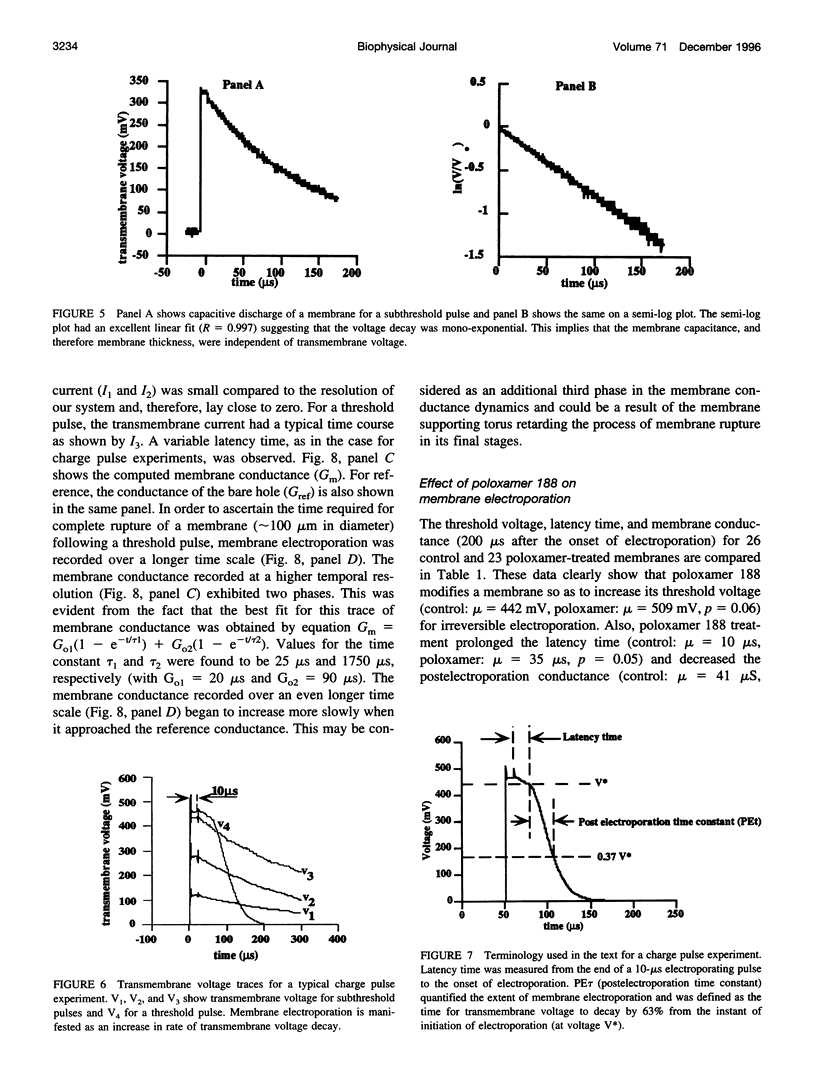
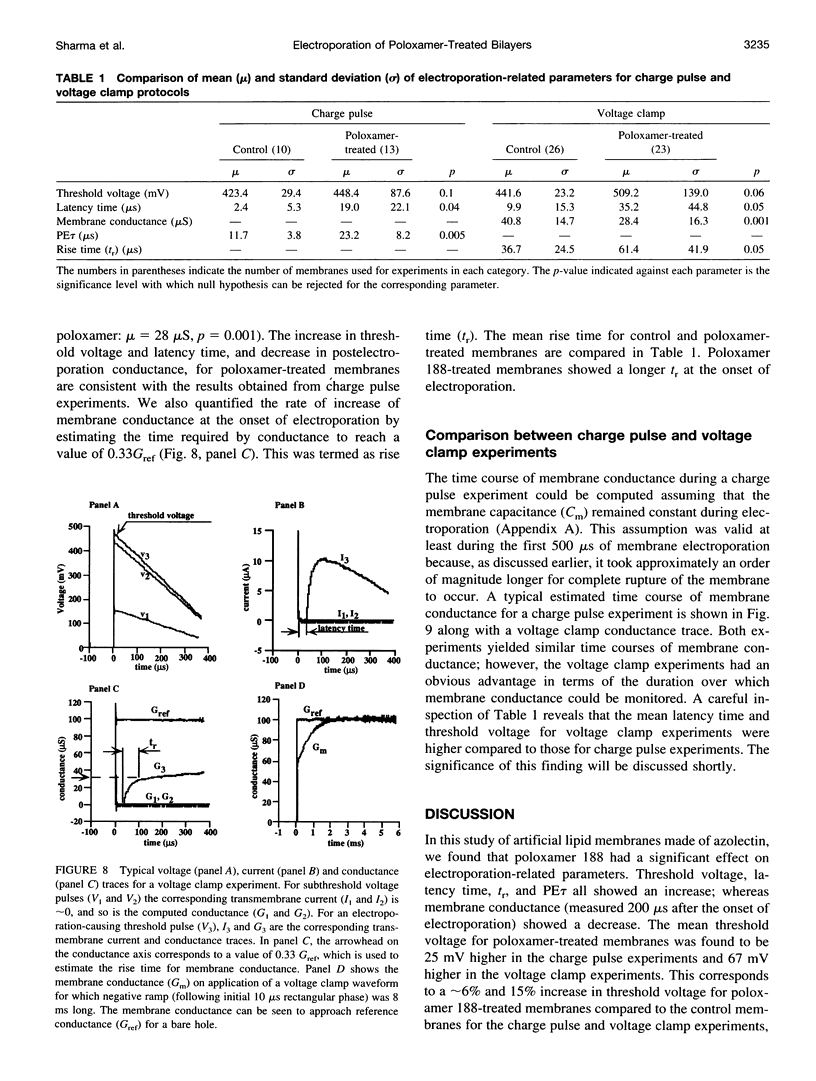
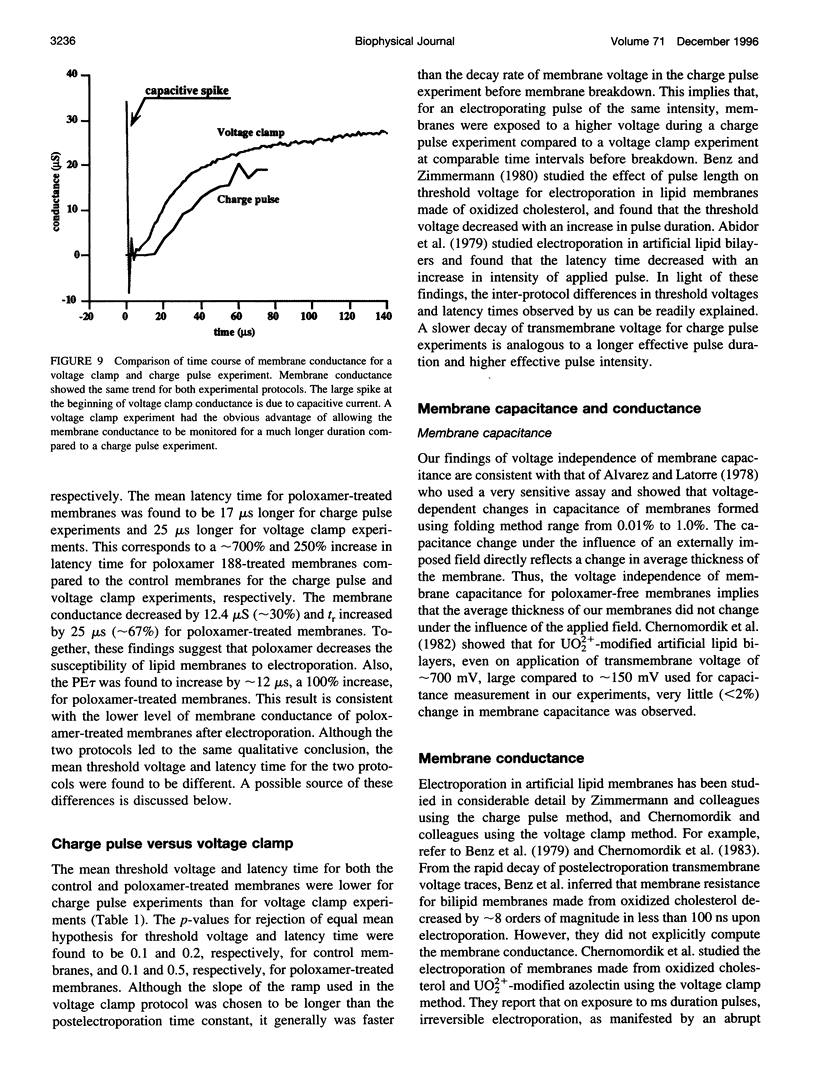
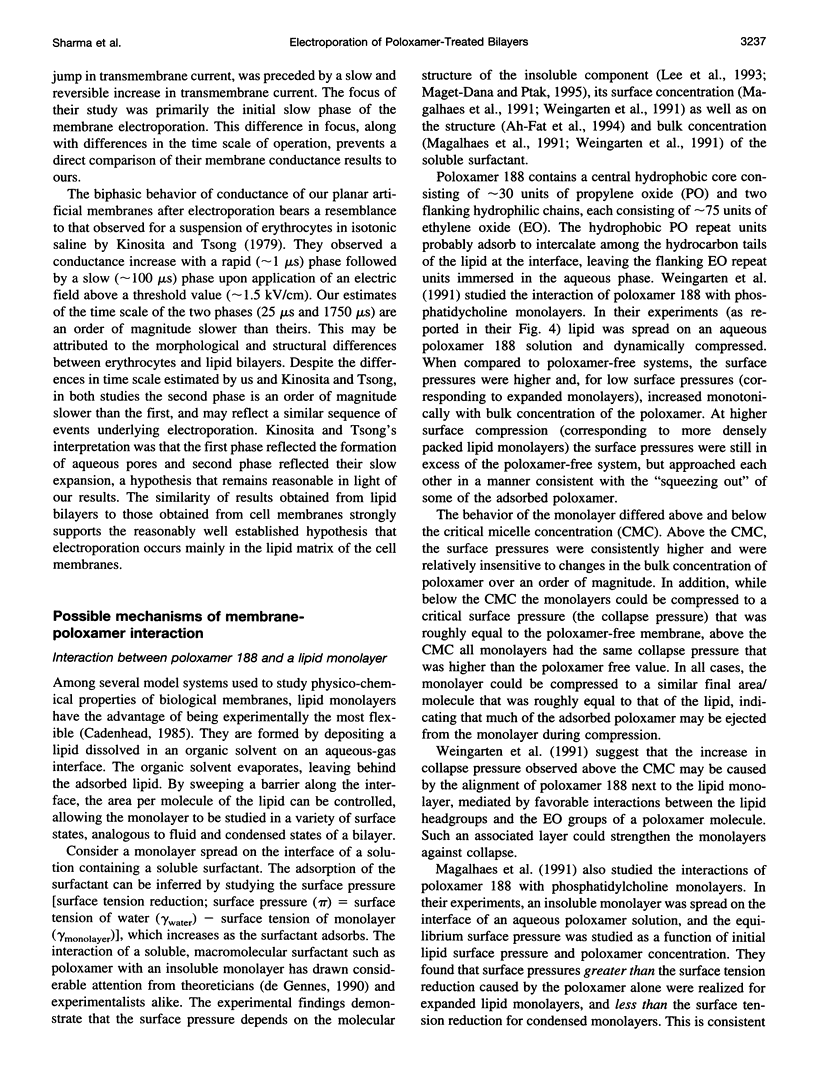
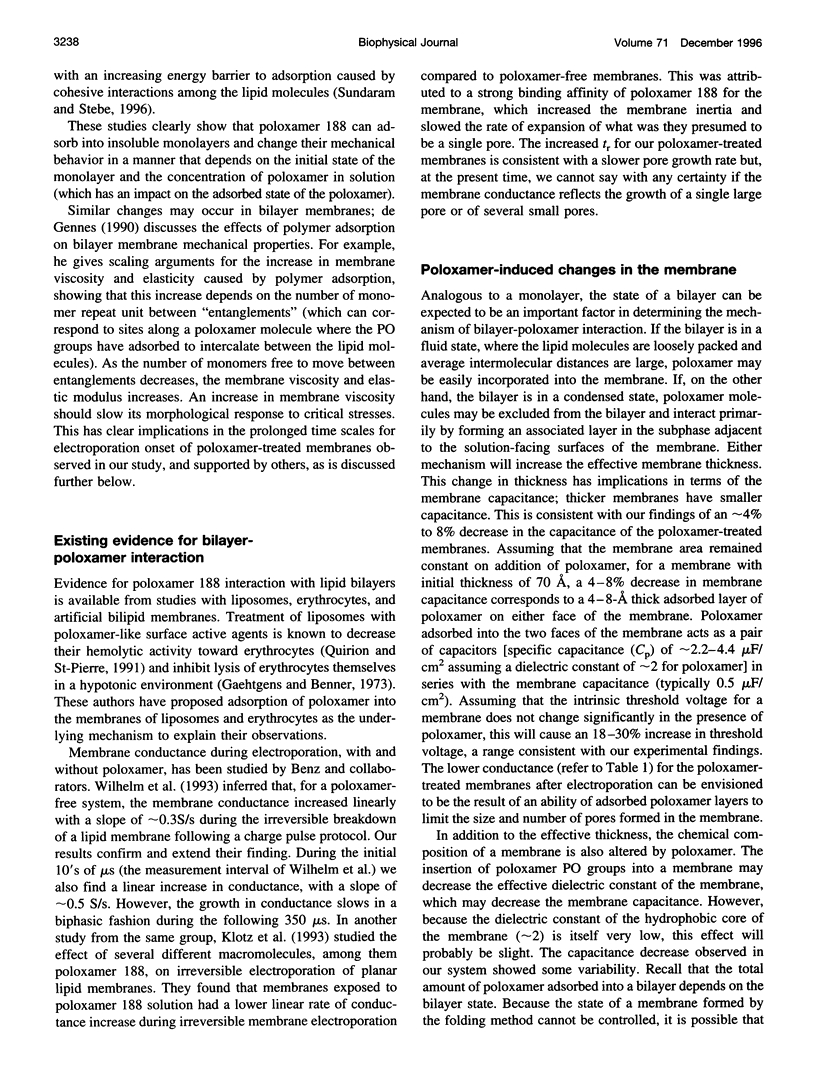
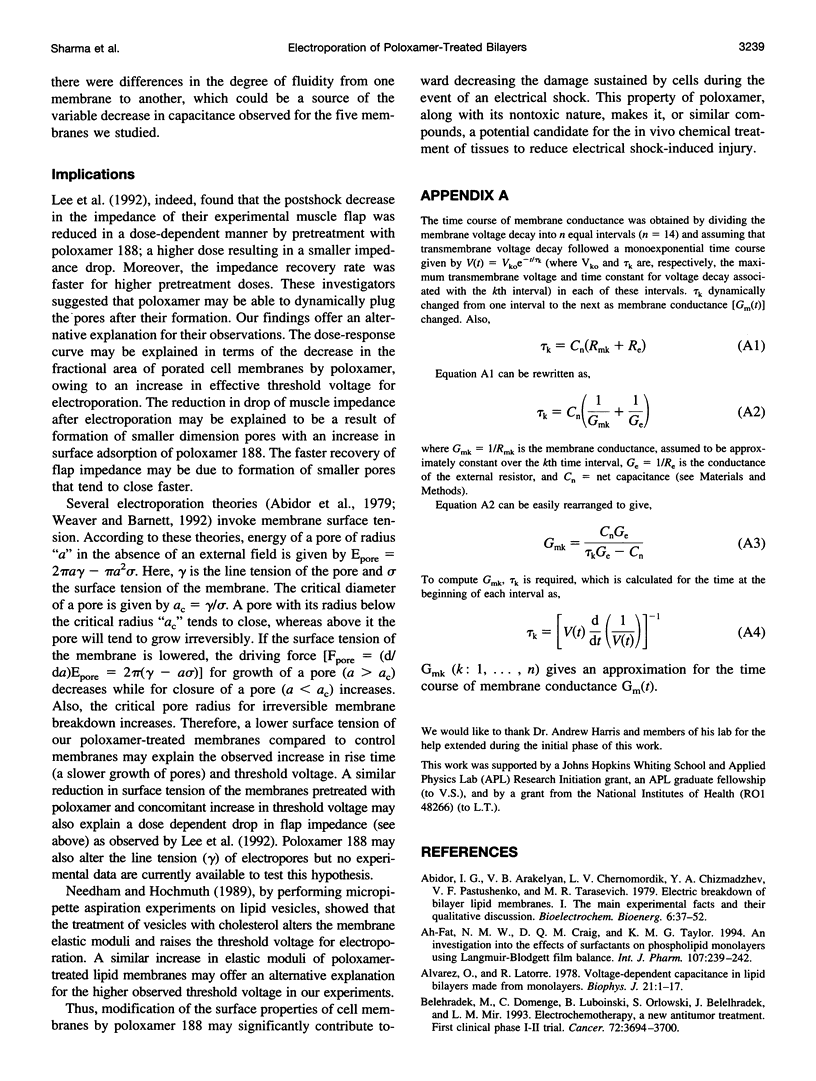
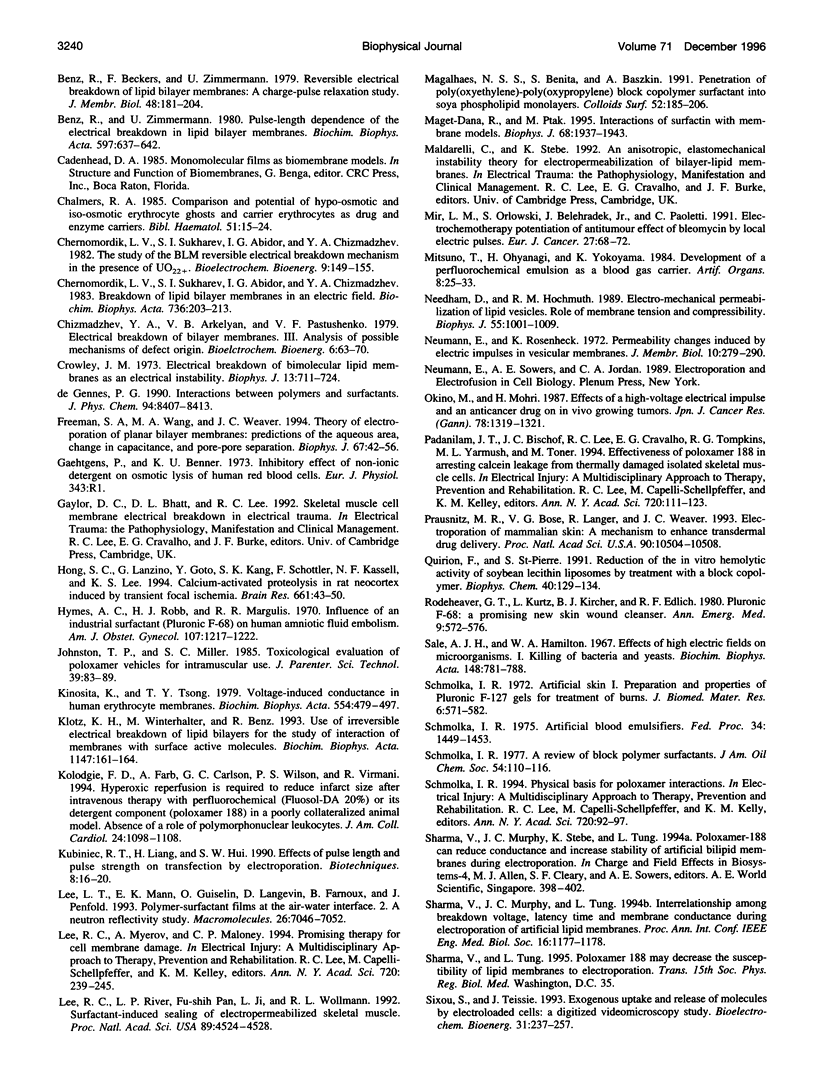
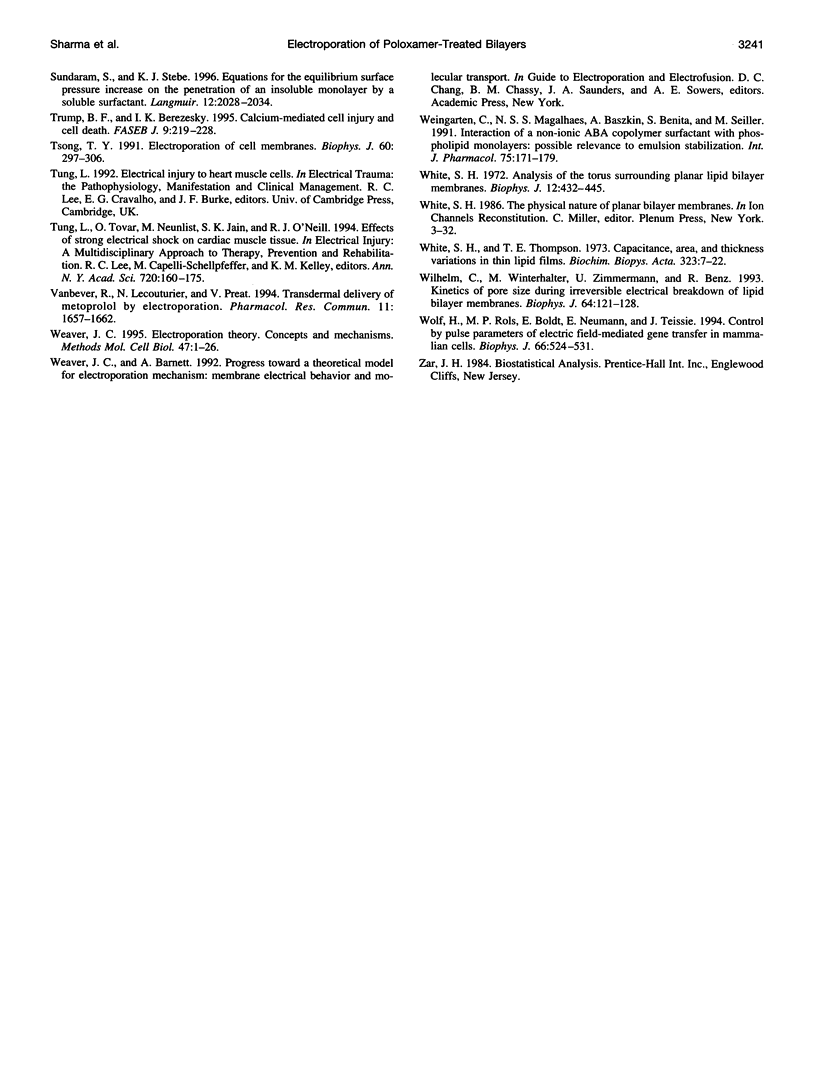
Images in this article
Selected References
These references are in PubMed. This may not be the complete list of references from this article.
- Alvarez O., Latorre R. Voltage-dependent capacitance in lipid bilayers made from monolayers. Biophys J. 1978 Jan;21(1):1–17. doi: 10.1016/S0006-3495(78)85505-2. [DOI] [PMC free article] [PubMed] [Google Scholar]
- Belehradek M., Domenge C., Luboinski B., Orlowski S., Belehradek J., Jr, Mir L. M. Electrochemotherapy, a new antitumor treatment. First clinical phase I-II trial. Cancer. 1993 Dec 15;72(12):3694–3700. doi: 10.1002/1097-0142(19931215)72:12<3694::aid-cncr2820721222>3.0.co;2-2. [DOI] [PubMed] [Google Scholar]
- Benz R., Beckers F., Zimmermann U. Reversible electrical breakdown of lipid bilayer membranes: a charge-pulse relaxation study. J Membr Biol. 1979 Jul 16;48(2):181–204. doi: 10.1007/BF01872858. [DOI] [PubMed] [Google Scholar]
- Benz R., Zimmermann U. Pulse-length dependence of the electrical breakdown in lipid bilayer membranes. Biochim Biophys Acta. 1980 Apr 24;597(3):637–642. doi: 10.1016/0005-2736(80)90236-9. [DOI] [PubMed] [Google Scholar]
- Chalmers R. A. Comparison and potential of hypo-osmotic and iso-osmotic erythrocyte ghosts and carrier erythrocytes as drug and enzyme carriers. Bibl Haematol. 1985;(51):15–24. doi: 10.1159/000410223. [DOI] [PubMed] [Google Scholar]
- Crowley J. M. Electrical breakdown of bimolecular lipid membranes as an electromechanical instability. Biophys J. 1973 Jul;13(7):711–724. doi: 10.1016/S0006-3495(73)86017-5. [DOI] [PMC free article] [PubMed] [Google Scholar]
- Freeman S. A., Wang M. A., Weaver J. C. Theory of electroporation of planar bilayer membranes: predictions of the aqueous area, change in capacitance, and pore-pore separation. Biophys J. 1994 Jul;67(1):42–56. doi: 10.1016/S0006-3495(94)80453-9. [DOI] [PMC free article] [PubMed] [Google Scholar]
- Hong S. C., Lanzino G., Goto Y., Kang S. K., Schottler F., Kassell N. F., Lee K. S. Calcium-activated proteolysis in rat neocortex induced by transient focal ischemia. Brain Res. 1994 Oct 24;661(1-2):43–50. doi: 10.1016/0006-8993(94)91178-9. [DOI] [PubMed] [Google Scholar]
- Hymes A. C., Robb H. J., Margulis R. R. Influence of an industrial surfactant (pluronic F-68) on human amniotic fluid embolism. Am J Obstet Gynecol. 1970 Aug 15;107(8):1217–1222. doi: 10.1016/s0002-9378(15)30372-0. [DOI] [PubMed] [Google Scholar]
- Johnston T. P., Miller S. C. Toxicological evaluation of poloxamer vehicles for intramuscular use. J Parenter Sci Technol. 1985 Mar-Apr;39(2):83–89. [PubMed] [Google Scholar]
- Kinosita K., Jr, Tsong T. Y. Voltage-induced conductance in human erythrocyte membranes. Biochim Biophys Acta. 1979 Jul 5;554(2):479–497. doi: 10.1016/0005-2736(79)90386-9. [DOI] [PubMed] [Google Scholar]
- Klotz K. H., Winterhalter M., Benz R. Use of irreversible electrical breakdown of lipid bilayers for the study of interaction of membranes with surface active molecules. Biochim Biophys Acta. 1993 Apr 8;1147(1):161–164. doi: 10.1016/0005-2736(93)90327-v. [DOI] [PubMed] [Google Scholar]
- Kolodgie F. D., Farb A., Carlson G. C., Wilson P. S., Virmani R. Hyperoxic reperfusion is required to reduce infarct size after intravenous therapy with perfluorochemical (Fluosol-DA 20%) or its detergent component (poloxamer 188) in a poorly collateralized animal model. Absence of a role of polymorphonuclear leukocytes. J Am Coll Cardiol. 1994 Oct;24(4):1098–1108. doi: 10.1016/0735-1097(94)90876-1. [DOI] [PubMed] [Google Scholar]
- Kubiniec R. T., Liang H., Hui S. W. Effects of pulse length and pulse strength on transfection by electroporation. Biotechniques. 1990 Jan;8(1):16–20. [PubMed] [Google Scholar]
- Lee R. C., Myerov A., Maloney C. P. Promising therapy for cell membrane damage. Ann N Y Acad Sci. 1994 May 31;720:239–245. doi: 10.1111/j.1749-6632.1994.tb30453.x. [DOI] [PubMed] [Google Scholar]
- Lee R. C., River L. P., Pan F. S., Ji L., Wollmann R. L. Surfactant-induced sealing of electropermeabilized skeletal muscle membranes in vivo. Proc Natl Acad Sci U S A. 1992 May 15;89(10):4524–4528. doi: 10.1073/pnas.89.10.4524. [DOI] [PMC free article] [PubMed] [Google Scholar]
- Maget-Dana R., Ptak M. Interactions of surfactin with membrane models. Biophys J. 1995 May;68(5):1937–1943. doi: 10.1016/S0006-3495(95)80370-X. [DOI] [PMC free article] [PubMed] [Google Scholar]
- Mir L. M., Orlowski S., Belehradek J., Jr, Paoletti C. Electrochemotherapy potentiation of antitumour effect of bleomycin by local electric pulses. Eur J Cancer. 1991;27(1):68–72. doi: 10.1016/0277-5379(91)90064-k. [DOI] [PubMed] [Google Scholar]
- Mitsuno T., Ohyanagi H., Yokoyama K. Development of a perfluorochemical emulsion as a blood gas carrier. Artif Organs. 1984 Feb;8(1):25–33. doi: 10.1111/j.1525-1594.1984.tb04240.x. [DOI] [PubMed] [Google Scholar]
- Needham D., Hochmuth R. M. Electro-mechanical permeabilization of lipid vesicles. Role of membrane tension and compressibility. Biophys J. 1989 May;55(5):1001–1009. doi: 10.1016/S0006-3495(89)82898-X. [DOI] [PMC free article] [PubMed] [Google Scholar]
- Neumann E., Rosenheck K. Permeability changes induced by electric impulses in vesicular membranes. J Membr Biol. 1972 Dec 29;10(3):279–290. doi: 10.1007/BF01867861. [DOI] [PubMed] [Google Scholar]
- Okino M., Mohri H. Effects of a high-voltage electrical impulse and an anticancer drug on in vivo growing tumors. Jpn J Cancer Res. 1987 Dec;78(12):1319–1321. [PubMed] [Google Scholar]
- Padanilam J. T., Bischof J. C., Lee R. C., Cravalho E. G., Tompkins R. G., Yarmush M. L., Toner M. Effectiveness of poloxamer 188 in arresting calcein leakage from thermally damaged isolated skeletal muscle cells. Ann N Y Acad Sci. 1994 May 31;720:111–123. doi: 10.1111/j.1749-6632.1994.tb30439.x. [DOI] [PubMed] [Google Scholar]
- Prausnitz M. R., Bose V. G., Langer R., Weaver J. C. Electroporation of mammalian skin: a mechanism to enhance transdermal drug delivery. Proc Natl Acad Sci U S A. 1993 Nov 15;90(22):10504–10508. doi: 10.1073/pnas.90.22.10504. [DOI] [PMC free article] [PubMed] [Google Scholar]
- Quirion F., St-Pierre S. Reduction of the in vitro hemolytic activity of soybean lecithin liposomes by treatment with a block copolymer. Biophys Chem. 1991 May;40(2):129–134. doi: 10.1016/0301-4622(91)87001-l. [DOI] [PubMed] [Google Scholar]
- Rodeheaver G. T., Kurtz L., Kircher B. J., Edlich R. F. Pluronic F-68: a promising new skin wound cleanser. Ann Emerg Med. 1980 Nov;9(11):572–576. doi: 10.1016/s0196-0644(80)80228-9. [DOI] [PubMed] [Google Scholar]
- Schmolka I. R. Artificial blood emulsifiers. Fed Proc. 1975 May;34(6):1449–1453. [PubMed] [Google Scholar]
- Schmolka I. R. Artificial skin. I. Preparation and properties of pluronic F-127 gels for treatment of burns. J Biomed Mater Res. 1972 Nov;6(6):571–582. doi: 10.1002/jbm.820060609. [DOI] [PubMed] [Google Scholar]
- Schmolka I. R. Physical basis for poloxamer interactions. Ann N Y Acad Sci. 1994 May 31;720:92–97. doi: 10.1111/j.1749-6632.1994.tb30437.x. [DOI] [PubMed] [Google Scholar]
- Trump B. F., Berezesky I. K. Calcium-mediated cell injury and cell death. FASEB J. 1995 Feb;9(2):219–228. doi: 10.1096/fasebj.9.2.7781924. [DOI] [PubMed] [Google Scholar]
- Tsong T. Y. Electroporation of cell membranes. Biophys J. 1991 Aug;60(2):297–306. doi: 10.1016/S0006-3495(91)82054-9. [DOI] [PMC free article] [PubMed] [Google Scholar]
- Tung L., Tovar O., Neunlist M., Jain S. K., O'Neill R. J. Effects of strong electrical shock on cardiac muscle tissue. Ann N Y Acad Sci. 1994 May 31;720:160–175. doi: 10.1111/j.1749-6632.1994.tb30444.x. [DOI] [PubMed] [Google Scholar]
- Vanbever R., Lecouturier N., Préat V. Transdermal delivery of metoprolol by electroporation. Pharm Res. 1994 Nov;11(11):1657–1662. doi: 10.1023/a:1018930425591. [DOI] [PubMed] [Google Scholar]
- Weaver J. C. Electroporation theory. Concepts and mechanisms. Methods Mol Biol. 1995;47:1–26. doi: 10.1385/0-89603-310-4:1. [DOI] [PubMed] [Google Scholar]
- White S. H. Analysis of the torus surrounding planar lipid bilayer membranes. Biophys J. 1972 Apr;12(4):432–445. doi: 10.1016/S0006-3495(72)86095-8. [DOI] [PMC free article] [PubMed] [Google Scholar]
- White S. H., Thompson T. E. Capacitance, area, and thickness variations in thin lipid films. Biochim Biophys Acta. 1973 Sep 27;323(1):7–22. doi: 10.1016/0005-2736(73)90428-8. [DOI] [PubMed] [Google Scholar]
- Wilhelm C., Winterhalter M., Zimmermann U., Benz R. Kinetics of pore size during irreversible electrical breakdown of lipid bilayer membranes. Biophys J. 1993 Jan;64(1):121–128. doi: 10.1016/S0006-3495(93)81346-8. [DOI] [PMC free article] [PubMed] [Google Scholar]
- Wolf H., Rols M. P., Boldt E., Neumann E., Teissié J. Control by pulse parameters of electric field-mediated gene transfer in mammalian cells. Biophys J. 1994 Feb;66(2 Pt 1):524–531. doi: 10.1016/s0006-3495(94)80805-7. [DOI] [PMC free article] [PubMed] [Google Scholar]