Abstract
The effect of cholesterol on the bilayer partitioning of the peptide GALA (WEAALAEALAEALAEHLAEALAEALEALAA) and its assembly into a pore in large unilamellar vesicles composed of neutral and negatively charged phospholipids has been determined. GALA undergoes a conformational change from a random coil to an amphipathic alpha-helix when the pH is reduced from 7.0 to 5.0, inducing at low pH leakage of contents from vesicles. Leakage from neutral or negatively charged vesicles at pH 5.0 was similar and could be adequately explained by the mathematical model (Parente, R. A., S. Nir, and F. C. Szoka, Jr., 1990. Mechanism of leakage of phospholipid vesicle contents induced by the peptide GALA. Biochemistry. 29:8720-8728) which assumed that GALA becomes incorporated into the vesicle bilayer and irreversibly aggregates to form a pore consisting of 10 +/- 2 peptides. Increasing cholesterol content in the membranes resulted in a reduced efficiency of the peptide to induce leakage. Part of the cholesterol effect was due to reduced binding of the peptide to cholesterol-containing membranes. An additional effect of cholesterol was to increase reversibility of surface aggregation of the peptide in the membrane. Results could be explained and predicted with a model that retains the same pore size, i.e., 10 +/- 2 peptides, but includes reversible aggregation of the monomers to form the pore. Resonance energy transfer experiments using fluorescently labeled peptides confirmed that the degree of reversibility of surface aggregation of GALA was significantly larger in cholesterol-containing liposomes, thus reducing the efficiency of pore formation.
Full text
PDF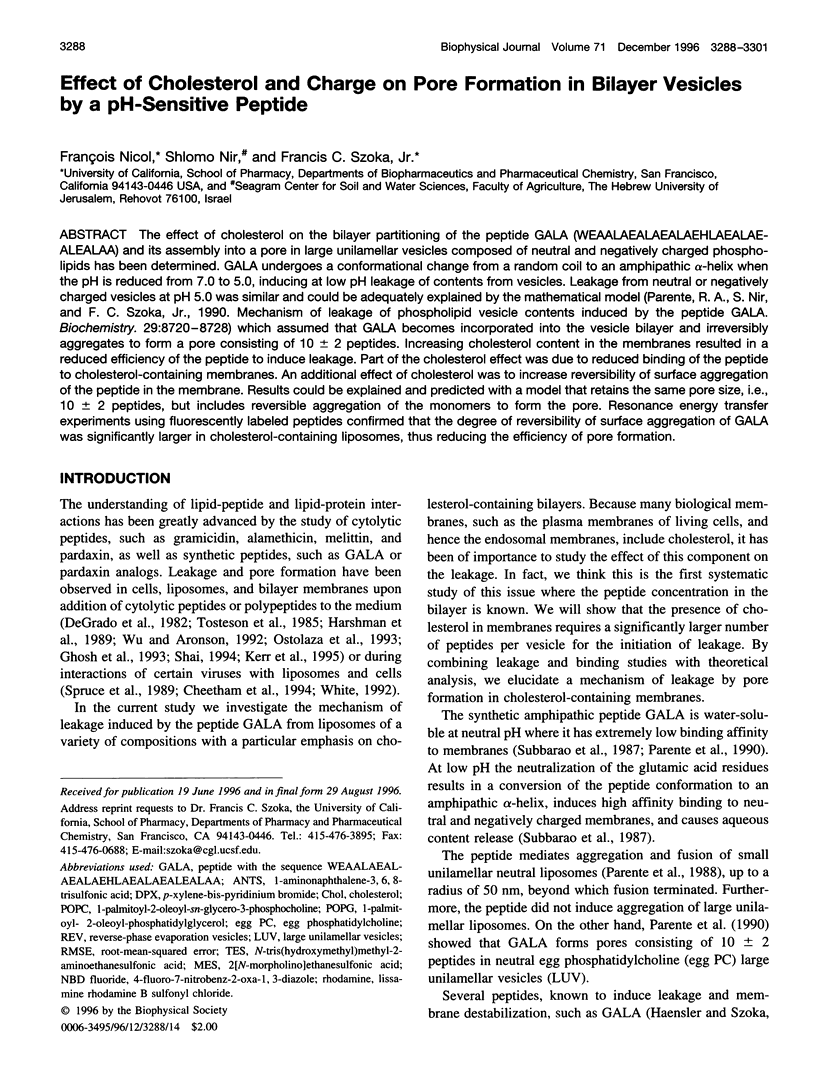
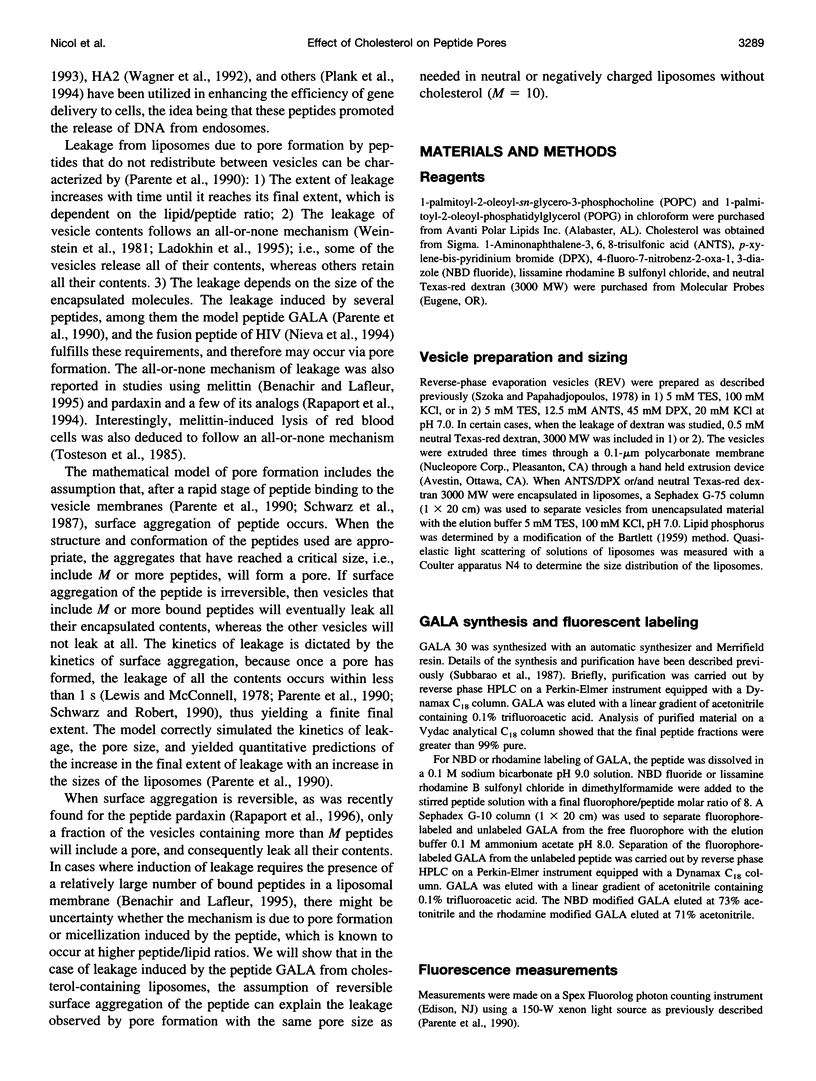
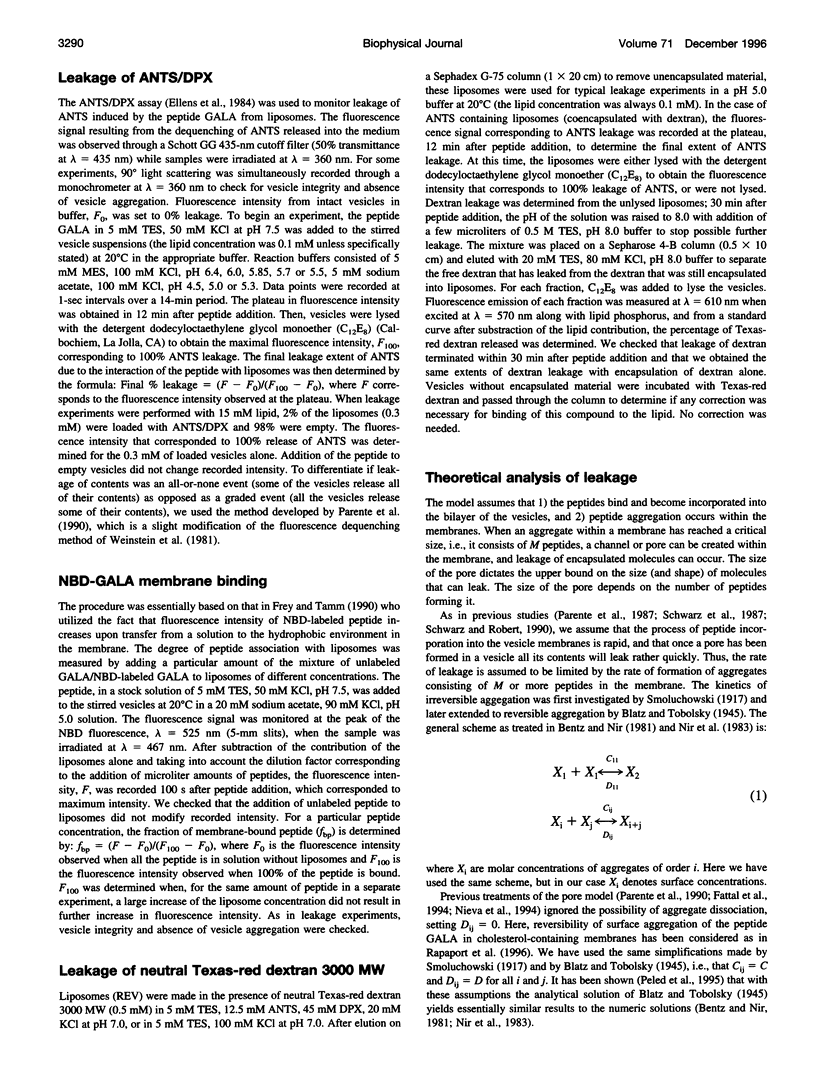
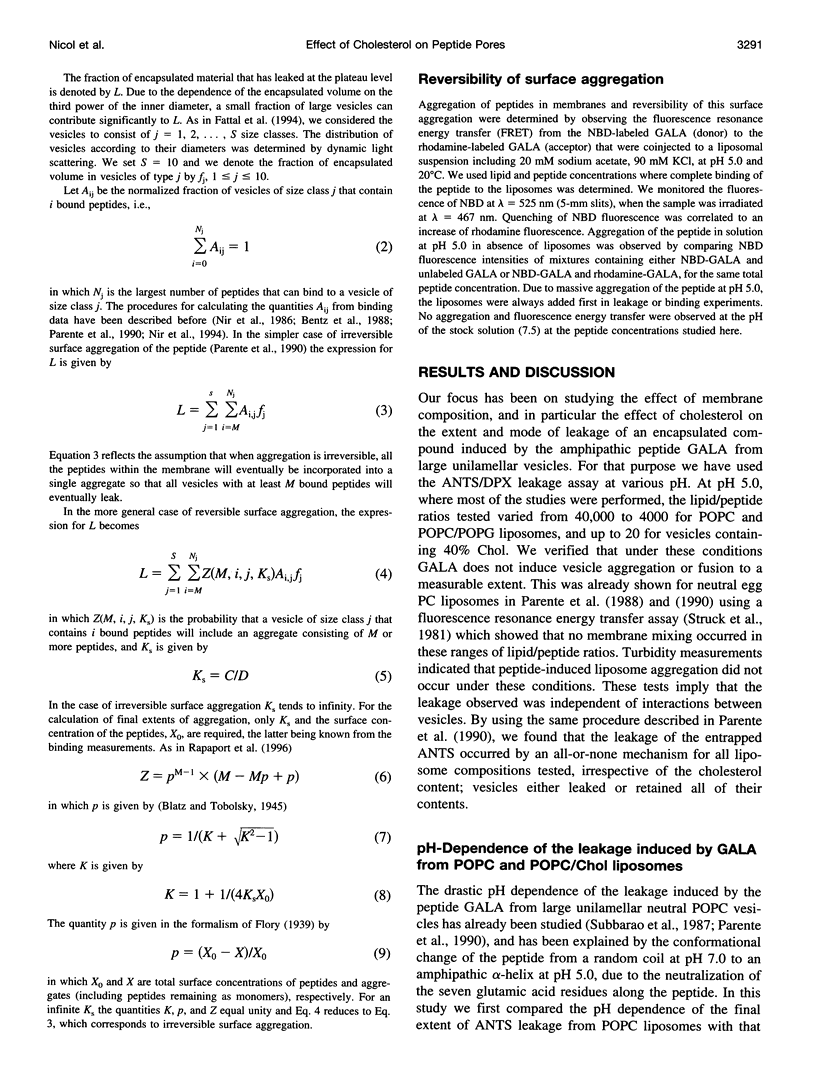
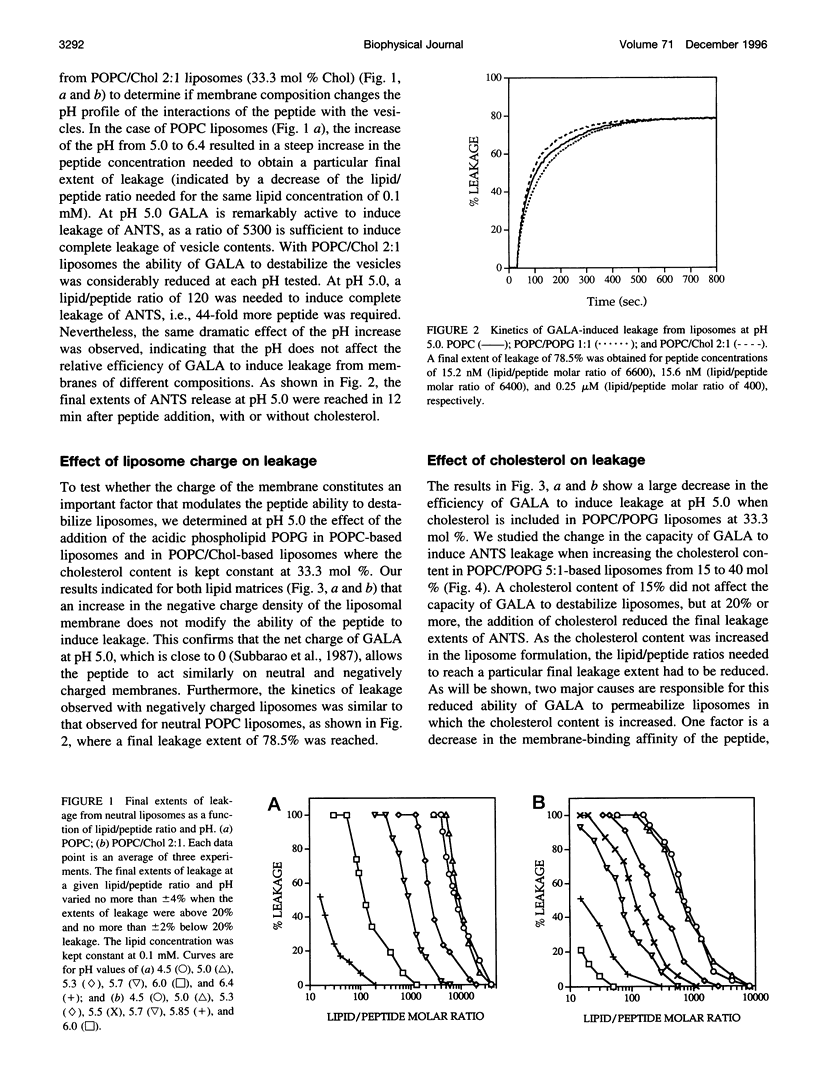
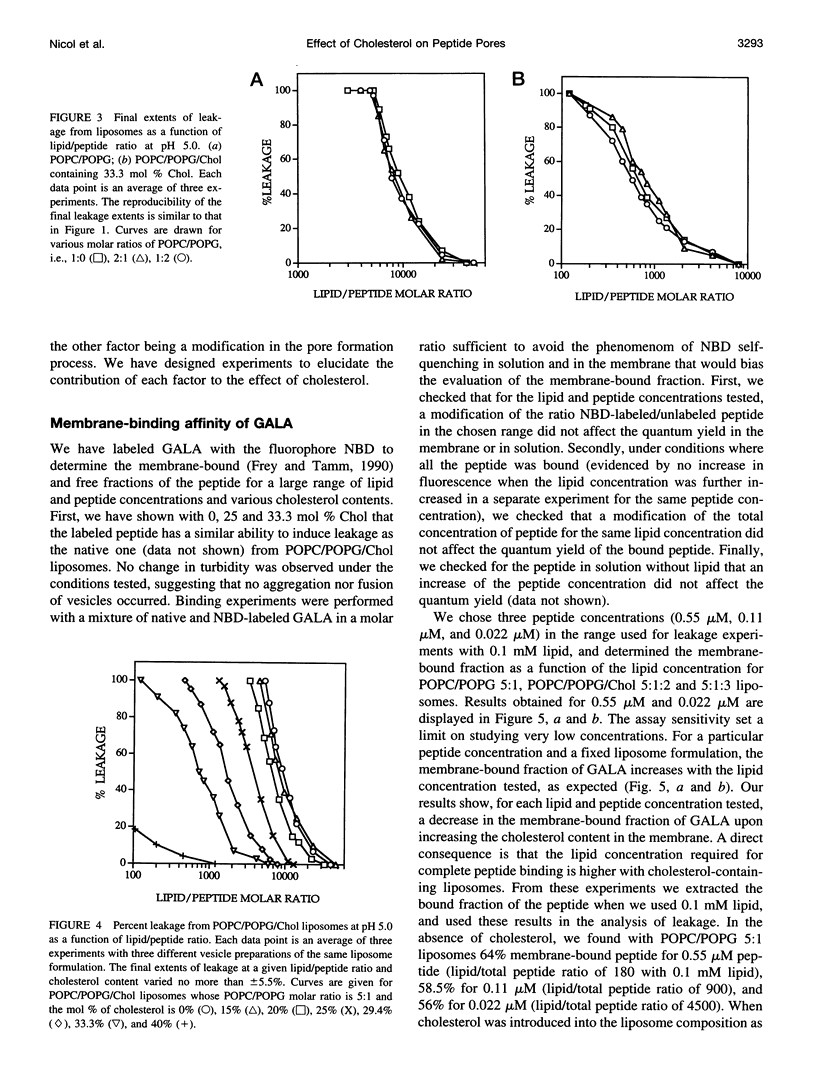
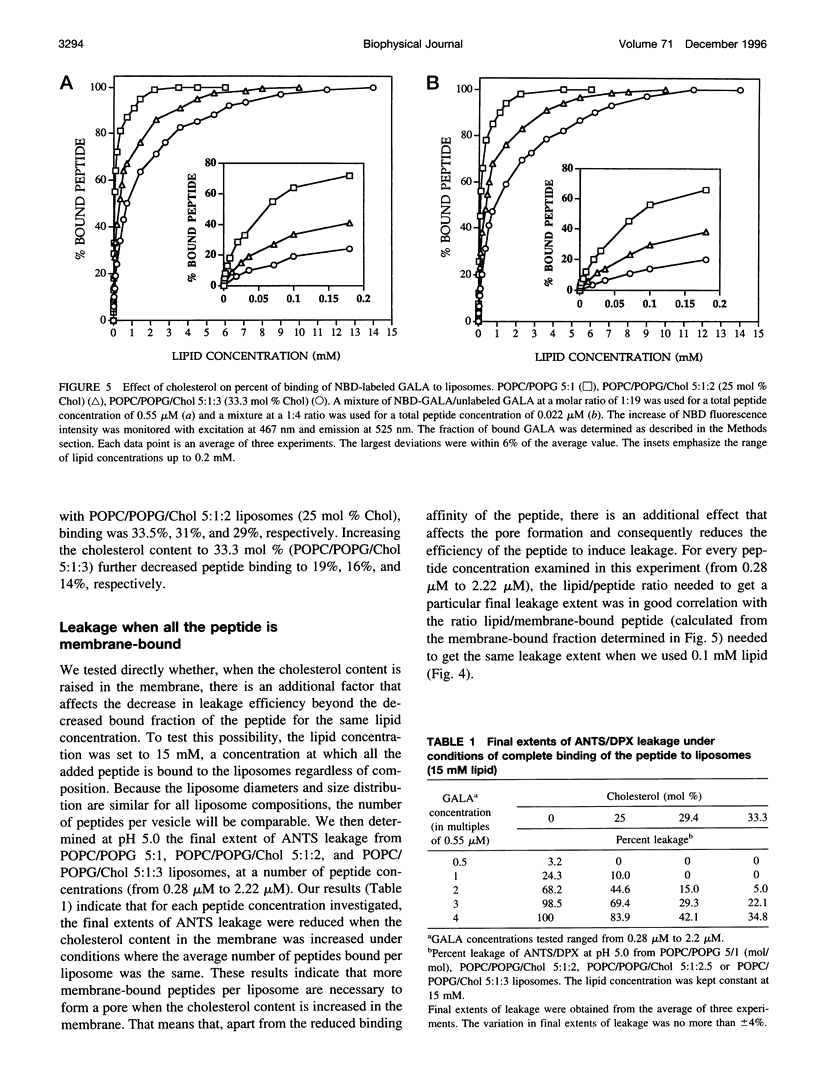
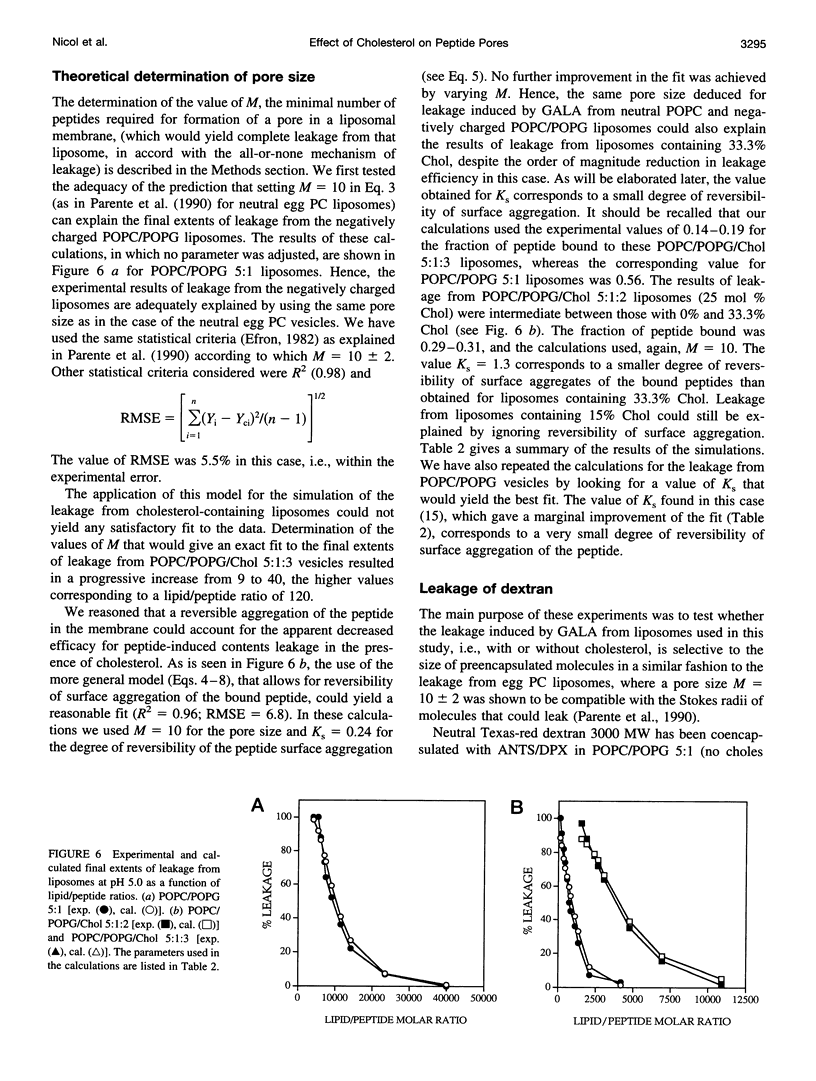
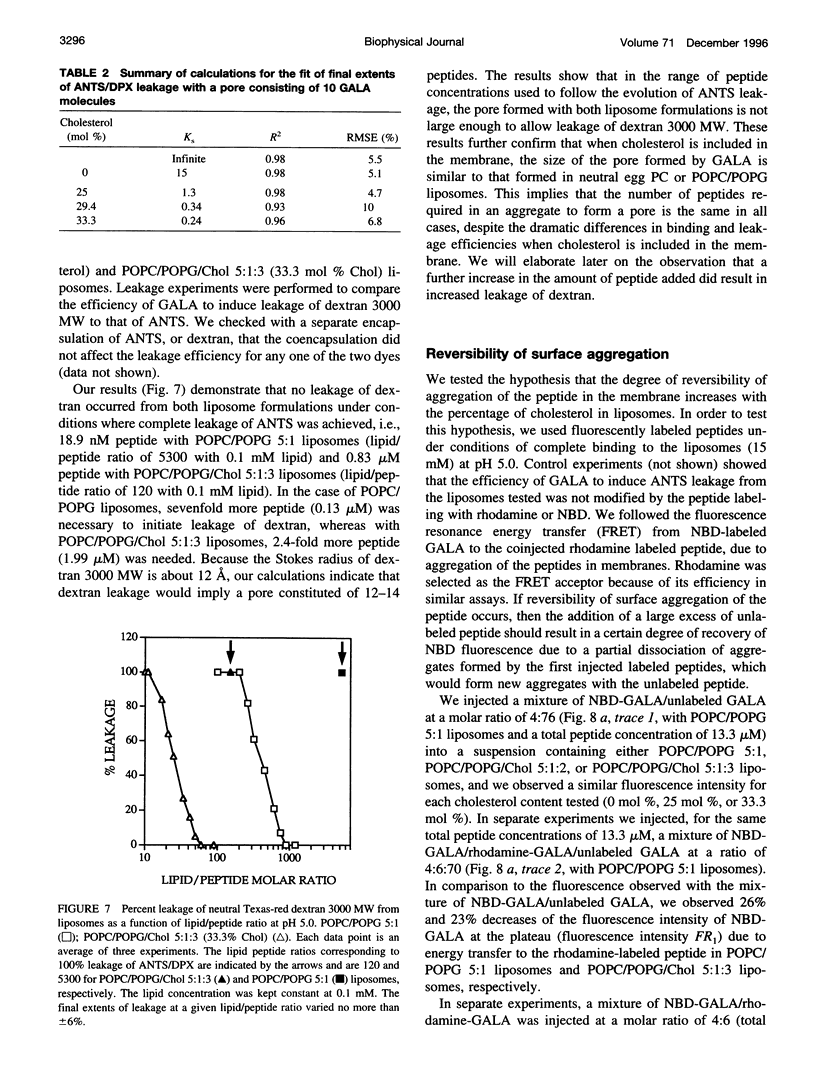
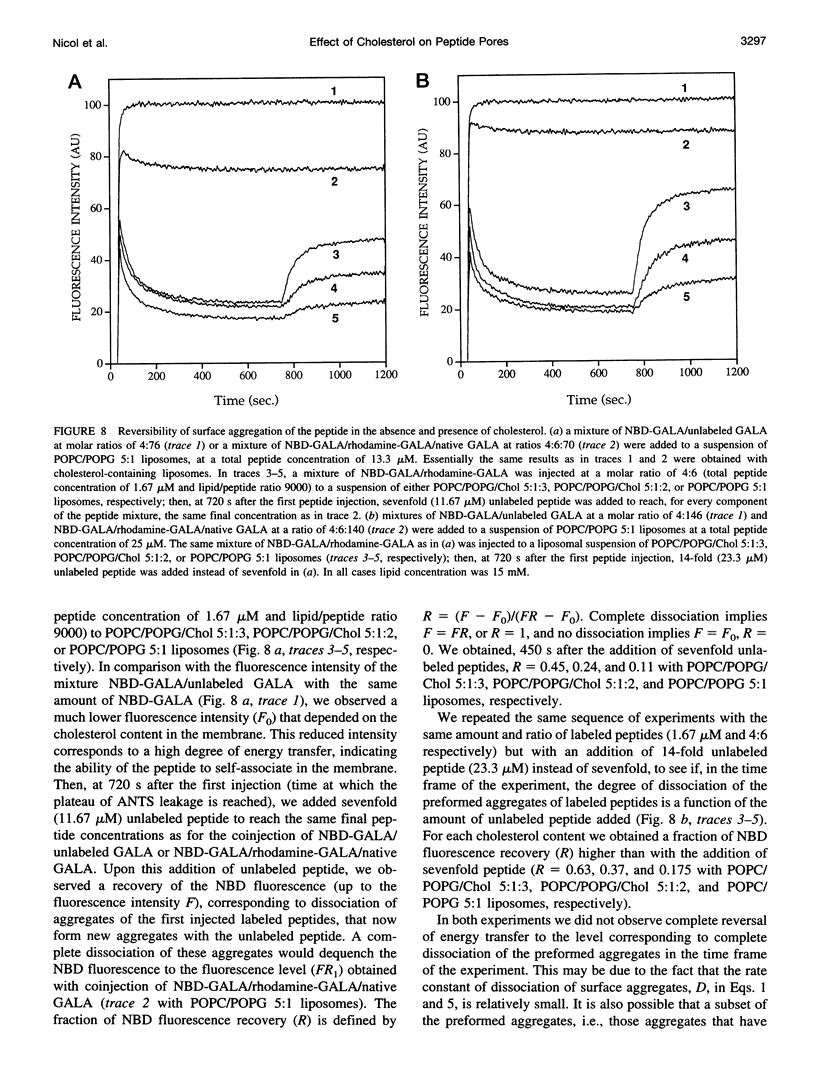
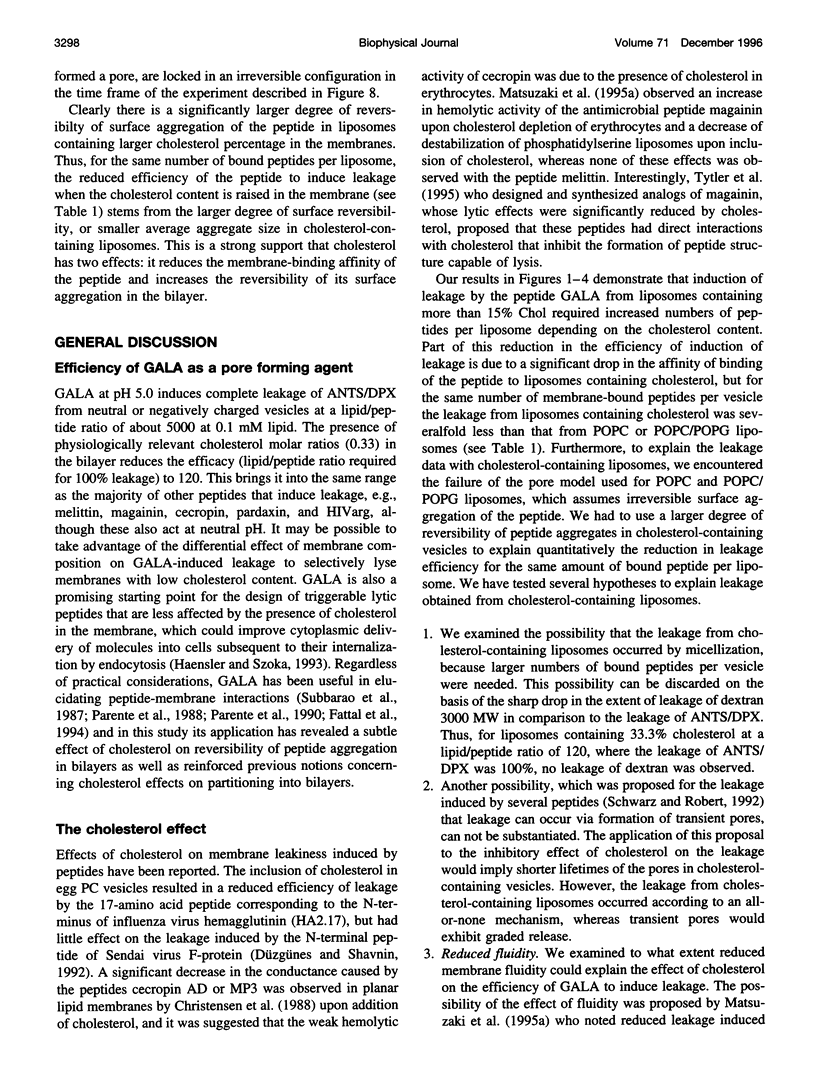
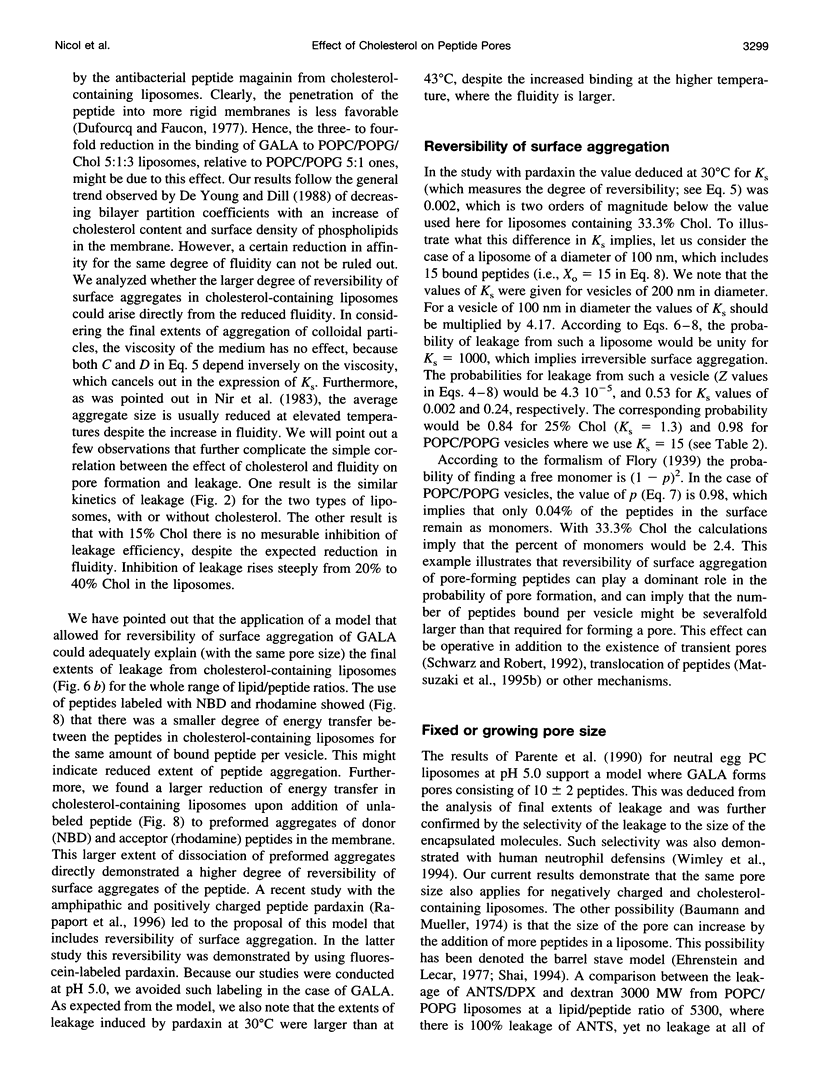
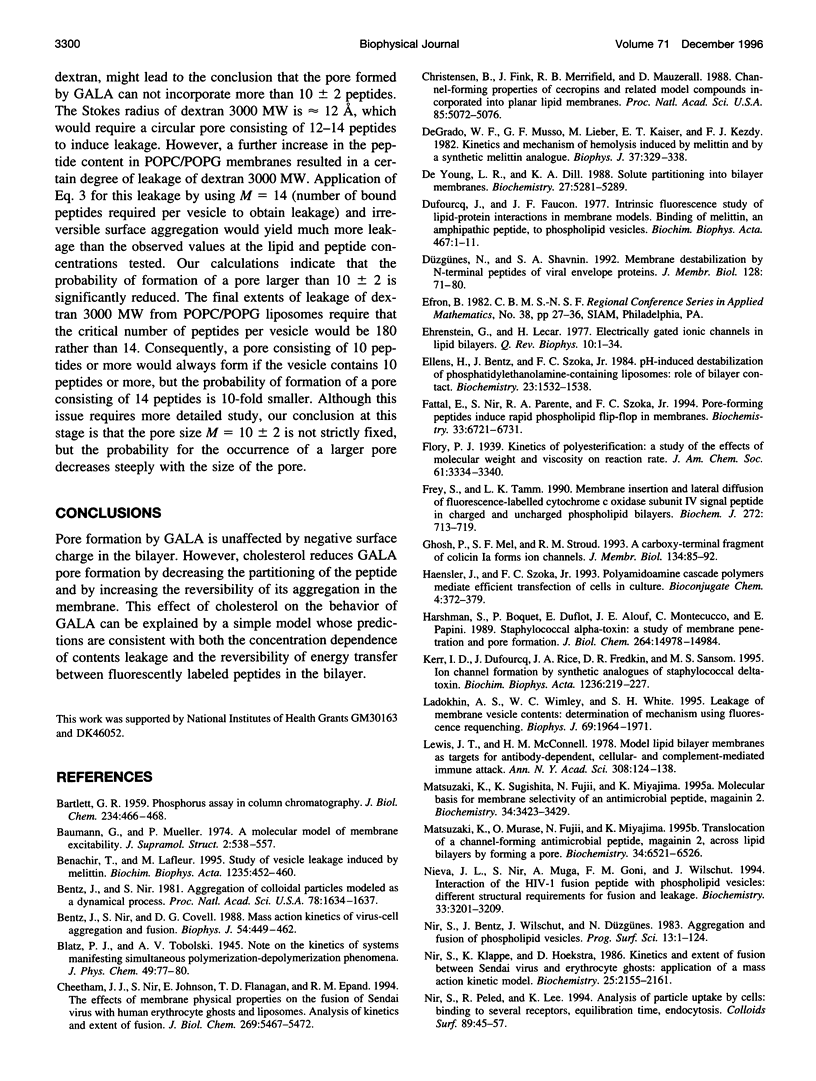
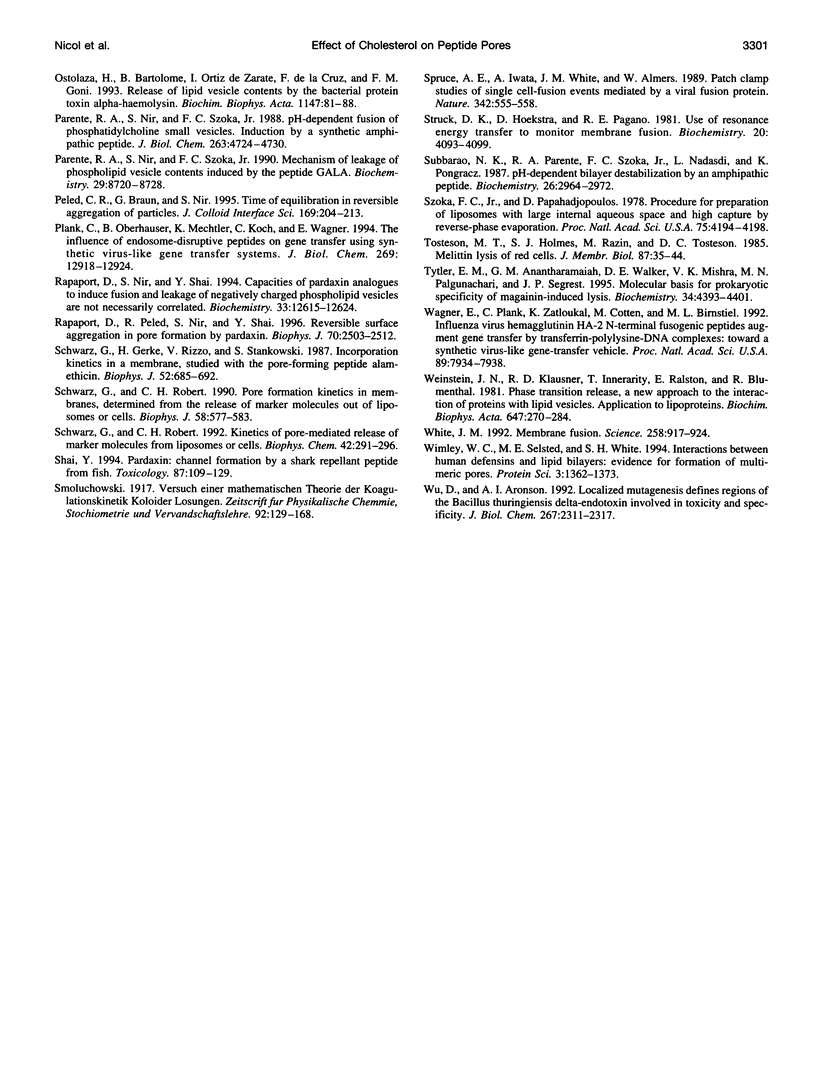
Selected References
These references are in PubMed. This may not be the complete list of references from this article.
- BARTLETT G. R. Phosphorus assay in column chromatography. J Biol Chem. 1959 Mar;234(3):466–468. [PubMed] [Google Scholar]
- Baumann G., Mueller P. A molecular model of membrane excitability. J Supramol Struct. 1974;2(5-6):538–557. doi: 10.1002/jss.400020504. [DOI] [PubMed] [Google Scholar]
- Benachir T., Lafleur M. Study of vesicle leakage induced by melittin. Biochim Biophys Acta. 1995 May 4;1235(2):452–460. doi: 10.1016/0005-2736(95)80035-e. [DOI] [PubMed] [Google Scholar]
- Bentz J., Nir S. Aggregation of colloidal particles modeled as a dynamical process. Proc Natl Acad Sci U S A. 1981 Mar;78(3):1634–1637. doi: 10.1073/pnas.78.3.1634. [DOI] [PMC free article] [PubMed] [Google Scholar]
- Bentz J., Nir S., Covell D. G. Mass action kinetics of virus-cell aggregation and fusion. Biophys J. 1988 Sep;54(3):449–462. doi: 10.1016/S0006-3495(88)82978-3. [DOI] [PMC free article] [PubMed] [Google Scholar]
- Cheetham J. J., Nir S., Johnson E., Flanagan T. D., Epand R. M. The effects of membrane physical properties on the fusion of Sendai virus with human erythrocyte ghosts and liposomes. Analysis of kinetics and extent of fusion. J Biol Chem. 1994 Feb 18;269(7):5467–5472. [PubMed] [Google Scholar]
- Christensen B., Fink J., Merrifield R. B., Mauzerall D. Channel-forming properties of cecropins and related model compounds incorporated into planar lipid membranes. Proc Natl Acad Sci U S A. 1988 Jul;85(14):5072–5076. doi: 10.1073/pnas.85.14.5072. [DOI] [PMC free article] [PubMed] [Google Scholar]
- De Young L. R., Dill K. A. Solute partitioning into lipid bilayer membranes. Biochemistry. 1988 Jul 12;27(14):5281–5289. doi: 10.1021/bi00414a050. [DOI] [PubMed] [Google Scholar]
- DeGrado W. F., Musso G. F., Lieber M., Kaiser E. T., Kézdy F. J. Kinetics and mechanism of hemolysis induced by melittin and by a synthetic melittin analogue. Biophys J. 1982 Jan;37(1):329–338. doi: 10.1016/S0006-3495(82)84681-X. [DOI] [PMC free article] [PubMed] [Google Scholar]
- Dufourcq J., Faucon J. F. Intrinsic fluorescence study of lipid-protein interactions in membrane models. Binding of melittin, an amphipathic peptide, to phospholipid vesicles. Biochim Biophys Acta. 1977 May 16;467(1):1–11. doi: 10.1016/0005-2736(77)90236-x. [DOI] [PubMed] [Google Scholar]
- Düzgüneş N., Shavnin S. A. Membrane destabilization by N-terminal peptides of viral envelope proteins. J Membr Biol. 1992 May;128(1):71–80. doi: 10.1007/BF00231872. [DOI] [PubMed] [Google Scholar]
- Ehrenstein G., Lecar H. Electrically gated ionic channels in lipid bilayers. Q Rev Biophys. 1977 Feb;10(1):1–34. doi: 10.1017/s0033583500000123. [DOI] [PubMed] [Google Scholar]
- Ellens H., Bentz J., Szoka F. C. pH-induced destabilization of phosphatidylethanolamine-containing liposomes: role of bilayer contact. Biochemistry. 1984 Mar 27;23(7):1532–1538. doi: 10.1021/bi00302a029. [DOI] [PubMed] [Google Scholar]
- Fattal E., Nir S., Parente R. A., Szoka F. C., Jr Pore-forming peptides induce rapid phospholipid flip-flop in membranes. Biochemistry. 1994 May 31;33(21):6721–6731. doi: 10.1021/bi00187a044. [DOI] [PubMed] [Google Scholar]
- Frey S., Tamm L. K. Membrane insertion and lateral diffusion of fluorescence-labelled cytochrome c oxidase subunit IV signal peptide in charged and uncharged phospholipid bilayers. Biochem J. 1990 Dec 15;272(3):713–719. doi: 10.1042/bj2720713. [DOI] [PMC free article] [PubMed] [Google Scholar]
- Ghosh P., Mel S. F., Stroud R. M. A carboxy-terminal fragment of colicin Ia forms ion channels. J Membr Biol. 1993 Jun;134(2):85–92. doi: 10.1007/BF00232745. [DOI] [PubMed] [Google Scholar]
- Haensler J., Szoka F. C., Jr Polyamidoamine cascade polymers mediate efficient transfection of cells in culture. Bioconjug Chem. 1993 Sep-Oct;4(5):372–379. doi: 10.1021/bc00023a012. [DOI] [PubMed] [Google Scholar]
- Harshman S., Boquet P., Duflot E., Alouf J. E., Montecucco C., Papini E. Staphylococcal alpha-toxin: a study of membrane penetration and pore formation. J Biol Chem. 1989 Sep 5;264(25):14978–14984. [PubMed] [Google Scholar]
- Kerr I. D., Dufourcq J., Rice J. A., Fredkin D. R., Sansom M. S. Ion channel formation by synthetic analogues of staphylococcal delta-toxin. Biochim Biophys Acta. 1995 Jun 14;1236(2):219–227. doi: 10.1016/0005-2736(95)00051-4. [DOI] [PubMed] [Google Scholar]
- Ladokhin A. S., Wimley W. C., White S. H. Leakage of membrane vesicle contents: determination of mechanism using fluorescence requenching. Biophys J. 1995 Nov;69(5):1964–1971. doi: 10.1016/S0006-3495(95)80066-4. [DOI] [PMC free article] [PubMed] [Google Scholar]
- Lewis J. T., McConnell H. M. Model lipid bilayer membranes as tragets for antibody-dependent, cellular- and complement-mediated immune attack. Ann N Y Acad Sci. 1978;308:124–138. doi: 10.1111/j.1749-6632.1978.tb22018.x. [DOI] [PubMed] [Google Scholar]
- Matsuzaki K., Murase O., Fujii N., Miyajima K. Translocation of a channel-forming antimicrobial peptide, magainin 2, across lipid bilayers by forming a pore. Biochemistry. 1995 May 16;34(19):6521–6526. doi: 10.1021/bi00019a033. [DOI] [PubMed] [Google Scholar]
- Matsuzaki K., Sugishita K., Fujii N., Miyajima K. Molecular basis for membrane selectivity of an antimicrobial peptide, magainin 2. Biochemistry. 1995 Mar 14;34(10):3423–3429. doi: 10.1021/bi00010a034. [DOI] [PubMed] [Google Scholar]
- Nieva J. L., Nir S., Muga A., Goñi F. M., Wilschut J. Interaction of the HIV-1 fusion peptide with phospholipid vesicles: different structural requirements for fusion and leakage. Biochemistry. 1994 Mar 22;33(11):3201–3209. doi: 10.1021/bi00177a009. [DOI] [PubMed] [Google Scholar]
- Nir S., Klappe K., Hoekstra D. Kinetics and extent of fusion between Sendai virus and erythrocyte ghosts: application of a mass action kinetic model. Biochemistry. 1986 Apr 22;25(8):2155–2161. doi: 10.1021/bi00356a046. [DOI] [PubMed] [Google Scholar]
- Ostolaza H., Bartolomé B., Ortiz de Zárate I., de la Cruz F., Goñi F. M. Release of lipid vesicle contents by the bacterial protein toxin alpha-haemolysin. Biochim Biophys Acta. 1993 Apr 8;1147(1):81–88. doi: 10.1016/0005-2736(93)90318-t. [DOI] [PubMed] [Google Scholar]
- Parente R. A., Nir S., Szoka F. C., Jr Mechanism of leakage of phospholipid vesicle contents induced by the peptide GALA. Biochemistry. 1990 Sep 18;29(37):8720–8728. doi: 10.1021/bi00489a031. [DOI] [PubMed] [Google Scholar]
- Parente R. A., Nir S., Szoka F. C., Jr pH-dependent fusion of phosphatidylcholine small vesicles. Induction by a synthetic amphipathic peptide. J Biol Chem. 1988 Apr 5;263(10):4724–4730. [PubMed] [Google Scholar]
- Plank C., Oberhauser B., Mechtler K., Koch C., Wagner E. The influence of endosome-disruptive peptides on gene transfer using synthetic virus-like gene transfer systems. J Biol Chem. 1994 Apr 29;269(17):12918–12924. [PubMed] [Google Scholar]
- Rapaport D., Nir S., Shai Y. Capacities of pardaxin analogues to induce fusion and leakage of negatively charged phospholipid vesicles are not necessarily correlated. Biochemistry. 1994 Oct 25;33(42):12615–12624. doi: 10.1021/bi00208a012. [DOI] [PubMed] [Google Scholar]
- Rapaport D., Peled R., Nir S., Shai Y. Reversible surface aggregation in pore formation by pardaxin. Biophys J. 1996 Jun;70(6):2502–2512. doi: 10.1016/S0006-3495(96)79822-3. [DOI] [PMC free article] [PubMed] [Google Scholar]
- Schwarz G., Gerke H., Rizzo V., Stankowski S. Incorporation kinetics in a membrane, studied with the pore-forming peptide alamethicin. Biophys J. 1987 Nov;52(5):685–692. doi: 10.1016/S0006-3495(87)83263-0. [DOI] [PMC free article] [PubMed] [Google Scholar]
- Schwarz G., Robert C. H. Kinetics of pore-mediated release of marker molecules from liposomes or cells. Biophys Chem. 1992 Apr;42(3):291–296. doi: 10.1016/0301-4622(92)80021-v. [DOI] [PubMed] [Google Scholar]
- Schwarz G., Robert C. H. Pore formation kinetics in membranes, determined from the release of marker molecules out of liposomes or cells. Biophys J. 1990 Sep;58(3):577–583. doi: 10.1016/S0006-3495(90)82401-2. [DOI] [PMC free article] [PubMed] [Google Scholar]
- Shai Y. Pardaxin: channel formation by a shark repellant peptide from fish. Toxicology. 1994 Feb 28;87(1-3):109–129. doi: 10.1016/0300-483x(94)90157-0. [DOI] [PubMed] [Google Scholar]
- Spruce A. E., Iwata A., White J. M., Almers W. Patch clamp studies of single cell-fusion events mediated by a viral fusion protein. Nature. 1989 Nov 30;342(6249):555–558. doi: 10.1038/342555a0. [DOI] [PubMed] [Google Scholar]
- Struck D. K., Hoekstra D., Pagano R. E. Use of resonance energy transfer to monitor membrane fusion. Biochemistry. 1981 Jul 7;20(14):4093–4099. doi: 10.1021/bi00517a023. [DOI] [PubMed] [Google Scholar]
- Subbarao N. K., Parente R. A., Szoka F. C., Jr, Nadasdi L., Pongracz K. pH-dependent bilayer destabilization by an amphipathic peptide. Biochemistry. 1987 Jun 2;26(11):2964–2972. doi: 10.1021/bi00385a002. [DOI] [PubMed] [Google Scholar]
- Szoka F., Jr, Papahadjopoulos D. Procedure for preparation of liposomes with large internal aqueous space and high capture by reverse-phase evaporation. Proc Natl Acad Sci U S A. 1978 Sep;75(9):4194–4198. doi: 10.1073/pnas.75.9.4194. [DOI] [PMC free article] [PubMed] [Google Scholar]
- Tosteson M. T., Holmes S. J., Razin M., Tosteson D. C. Melittin lysis of red cells. J Membr Biol. 1985;87(1):35–44. doi: 10.1007/BF01870697. [DOI] [PubMed] [Google Scholar]
- Tytler E. M., Anantharamaiah G. M., Walker D. E., Mishra V. K., Palgunachari M. N., Segrest J. P. Molecular basis for prokaryotic specificity of magainin-induced lysis. Biochemistry. 1995 Apr 4;34(13):4393–4401. doi: 10.1021/bi00013a031. [DOI] [PubMed] [Google Scholar]
- Wagner E., Plank C., Zatloukal K., Cotten M., Birnstiel M. L. Influenza virus hemagglutinin HA-2 N-terminal fusogenic peptides augment gene transfer by transferrin-polylysine-DNA complexes: toward a synthetic virus-like gene-transfer vehicle. Proc Natl Acad Sci U S A. 1992 Sep 1;89(17):7934–7938. doi: 10.1073/pnas.89.17.7934. [DOI] [PMC free article] [PubMed] [Google Scholar]
- Weinstein J. N., Klausner R. D., Innerarity T., Ralston E., Blumenthal R. Phase transition release, a new approach to the interaction of proteins with lipid vesicles. Application to lipoproteins. Biochim Biophys Acta. 1981 Oct 2;647(2):270–284. doi: 10.1016/0005-2736(81)90255-8. [DOI] [PubMed] [Google Scholar]
- White J. M. Membrane fusion. Science. 1992 Nov 6;258(5084):917–924. doi: 10.1126/science.1439803. [DOI] [PubMed] [Google Scholar]
- Wimley W. C., Selsted M. E., White S. H. Interactions between human defensins and lipid bilayers: evidence for formation of multimeric pores. Protein Sci. 1994 Sep;3(9):1362–1373. doi: 10.1002/pro.5560030902. [DOI] [PMC free article] [PubMed] [Google Scholar]
- Wu D., Aronson A. I. Localized mutagenesis defines regions of the Bacillus thuringiensis delta-endotoxin involved in toxicity and specificity. J Biol Chem. 1992 Feb 5;267(4):2311–2317. [PubMed] [Google Scholar]