Abstract
The major ampullate glands of the spider Nephila clavipes contain approximately 0.2 microliter each of a highly concentrated (approximately 50%) solution of silk fibroin. Therefore, the reservoir of silk in these glands presents an ideal opportunity to observe prefolded conformations of a protein in its native state. To this end, the structure and conformation of major ampullate gland silk fibroin within the glands of the spider N. clavipes were examined by 13C NMR spectroscopy. These results were compared to those from silk protein first drawn from the spinneret and then denatured. The 13C NMR chemical shifts, along with infrared and circular dichroism data, suggest that the silk fibroin in the glands exists in dynamically averaged helical conformations. Furthermore, there is no evidence of proline residues in U-(13)C-D-glucose-labeled silk. This transient prefolded "molten fibril" state may correspond to the silk I form found in Bombyx mori silk. There is no evidence of the final beta-sheet structure in the ampullate gland silk fibroin before final silk processing. However, the conformation of silk in the glands appears to be in a highly metastable state, as plasticization with water produces the beta-sheet structure. Therefore, the ducts connecting the ampullate glands to the spinnerets play a larger role in silk processing than previously thought.
Full text
PDF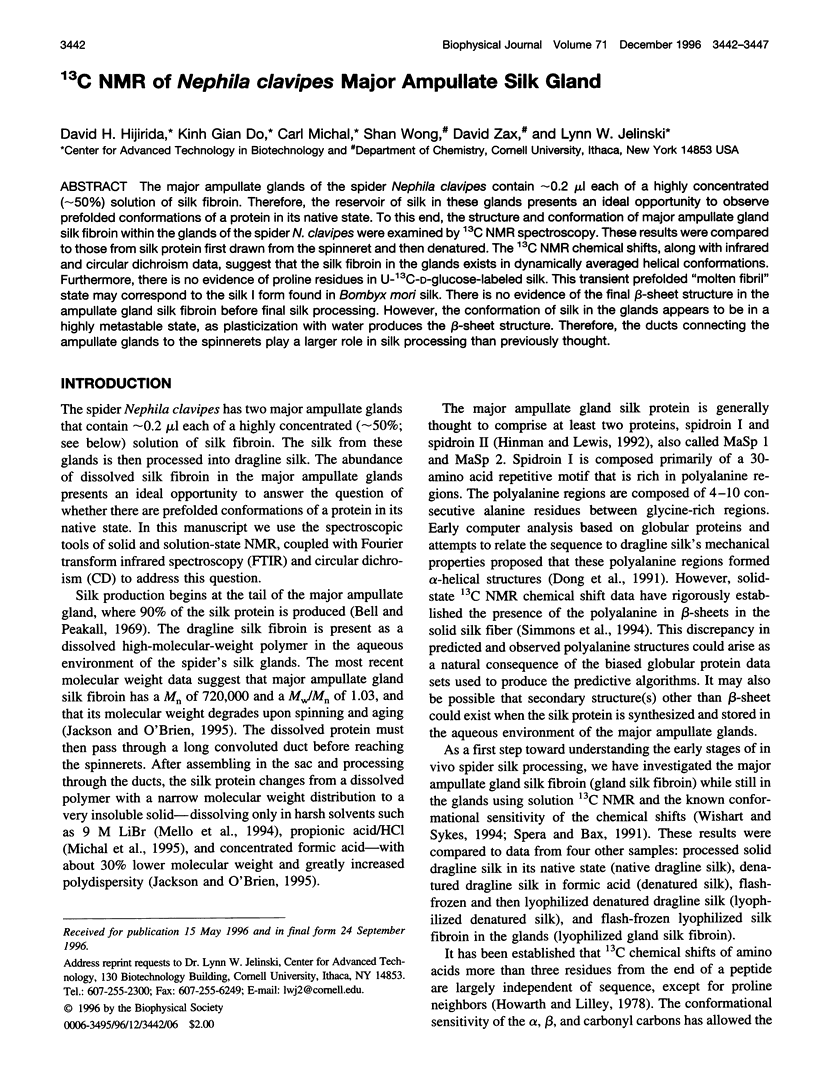
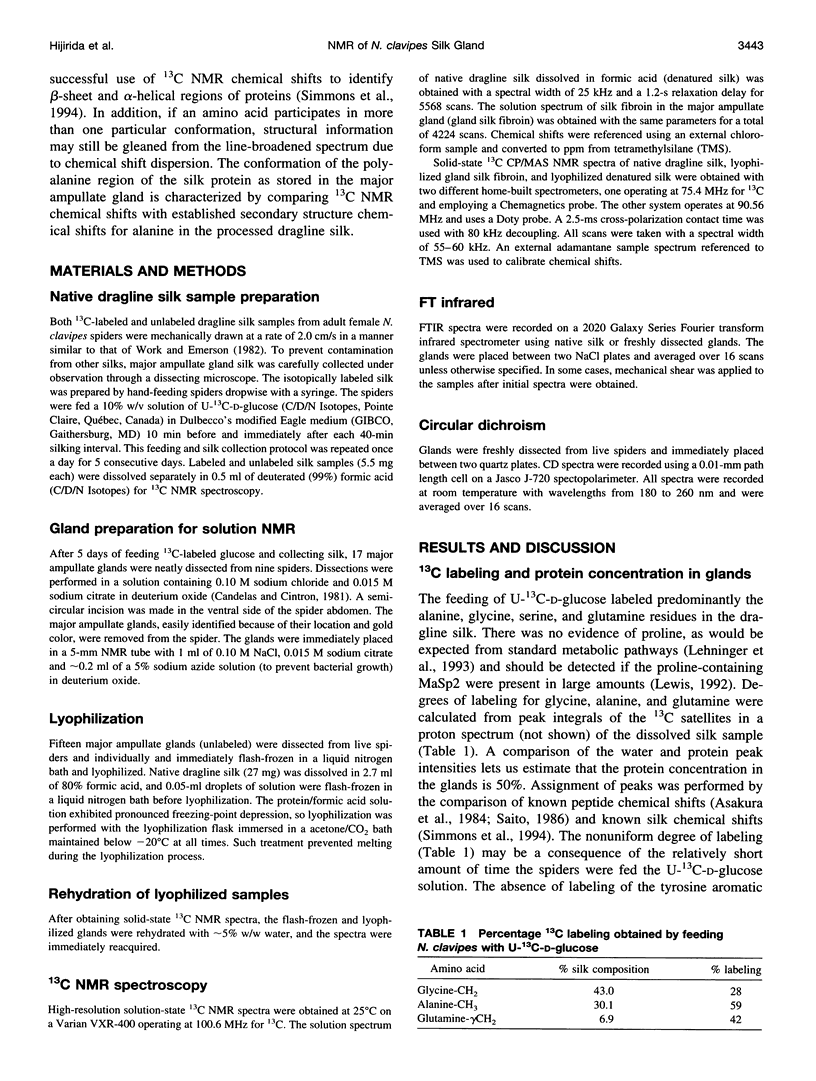
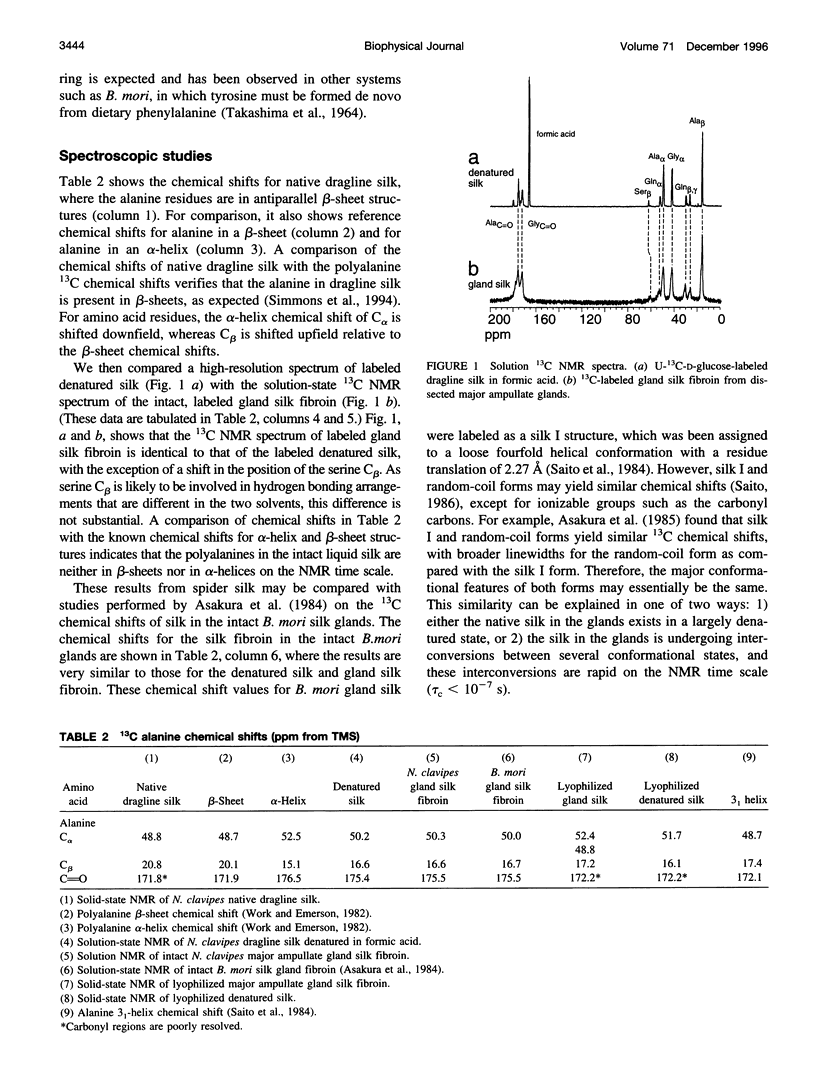
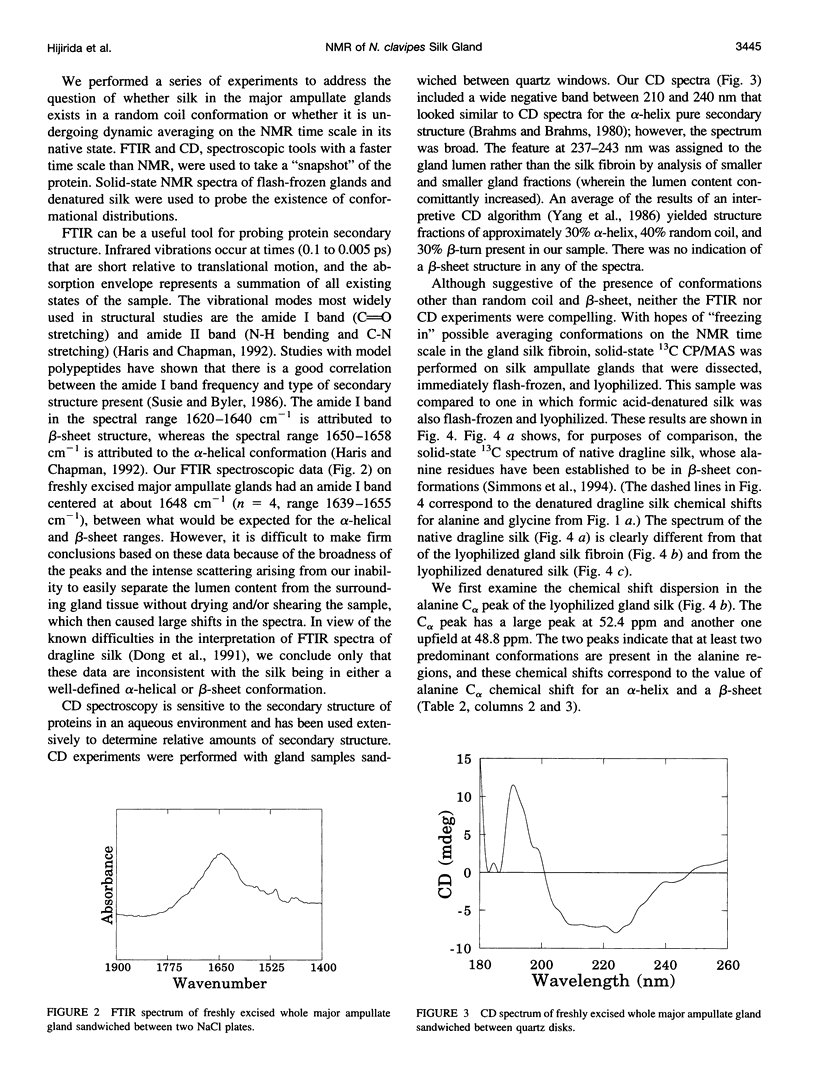
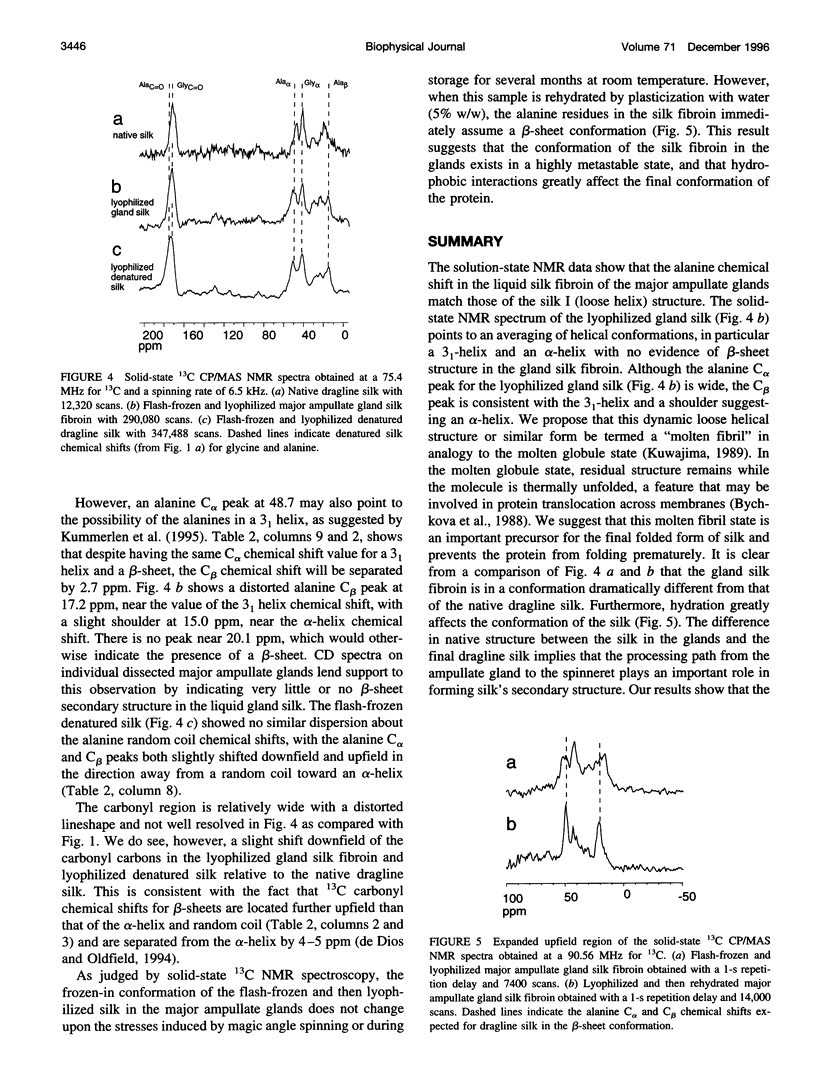
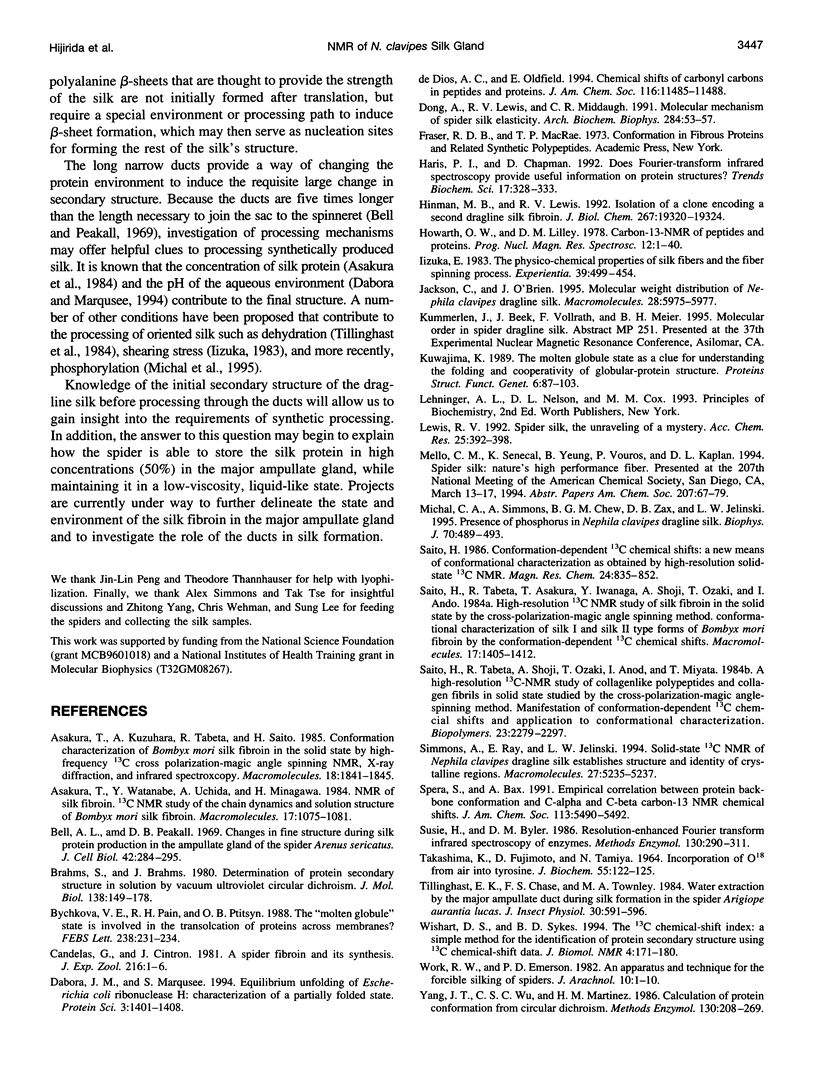
Selected References
These references are in PubMed. This may not be the complete list of references from this article.
- Bell A. L., Peakall D. B. Changes in fine structure during silk protein production in the ampullate gland of the spider Araneus sericatus. J Cell Biol. 1969 Jul;42(1):284–295. doi: 10.1083/jcb.42.1.284. [DOI] [PMC free article] [PubMed] [Google Scholar]
- Brahms S., Brahms J. Determination of protein secondary structure in solution by vacuum ultraviolet circular dichroism. J Mol Biol. 1980 Apr;138(2):149–178. doi: 10.1016/0022-2836(80)90282-x. [DOI] [PubMed] [Google Scholar]
- Bychkova V. E., Pain R. H., Ptitsyn O. B. The 'molten globule' state is involved in the translocation of proteins across membranes? FEBS Lett. 1988 Oct 10;238(2):231–234. doi: 10.1016/0014-5793(88)80485-x. [DOI] [PubMed] [Google Scholar]
- Dabora J. M., Marqusee S. Equilibrium unfolding of Escherichia coli ribonuclease H: characterization of a partially folded state. Protein Sci. 1994 Sep;3(9):1401–1408. doi: 10.1002/pro.5560030906. [DOI] [PMC free article] [PubMed] [Google Scholar]
- Dong Z., Lewis R. V., Middaugh C. R. Molecular mechanism of spider silk elasticity. Arch Biochem Biophys. 1991 Jan;284(1):53–57. doi: 10.1016/0003-9861(91)90262-h. [DOI] [PubMed] [Google Scholar]
- Haris P. I., Chapman D. Does Fourier-transform infrared spectroscopy provide useful information on protein structures? Trends Biochem Sci. 1992 Sep;17(9):328–333. doi: 10.1016/0968-0004(92)90305-s. [DOI] [PubMed] [Google Scholar]
- Hinman M. B., Lewis R. V. Isolation of a clone encoding a second dragline silk fibroin. Nephila clavipes dragline silk is a two-protein fiber. J Biol Chem. 1992 Sep 25;267(27):19320–19324. [PubMed] [Google Scholar]
- Kuwajima K. The molten globule state as a clue for understanding the folding and cooperativity of globular-protein structure. Proteins. 1989;6(2):87–103. doi: 10.1002/prot.340060202. [DOI] [PubMed] [Google Scholar]
- Michal C. A., Simmons A. H., Chew B. G., Zax D. B., Jelinski L. W. Presence of phosphorus in Nephila clavipes dragline silk. Biophys J. 1996 Jan;70(1):489–493. doi: 10.1016/S0006-3495(96)79594-2. [DOI] [PMC free article] [PubMed] [Google Scholar]
- Saitô H., Tabeta R., Shoji A., Ozaki T., Ando I., Miyata T. A high-resolution 13C-NMR study of collagenlike polypeptides and collagen fibrils in solid state studied by the cross-polarization-magic angle-spinning method. Manifestation of conformation-dependent 13C chemical shifts and application to conformational characterization. Biopolymers. 1984 Nov;23(11 Pt 1):2279–2297. doi: 10.1002/bip.360231111. [DOI] [PubMed] [Google Scholar]
- Susi H., Byler D. M. Resolution-enhanced Fourier transform infrared spectroscopy of enzymes. Methods Enzymol. 1986;130:290–311. doi: 10.1016/0076-6879(86)30015-6. [DOI] [PubMed] [Google Scholar]
- TAKASHIMA K., FUJIMOTO D., TAMIYA N. INCORPORATION OF O18 FROM AIR INTO TYROSINE. J Biochem. 1964 Feb;55:122–125. [PubMed] [Google Scholar]
- Wishart D. S., Sykes B. D. The 13C chemical-shift index: a simple method for the identification of protein secondary structure using 13C chemical-shift data. J Biomol NMR. 1994 Mar;4(2):171–180. doi: 10.1007/BF00175245. [DOI] [PubMed] [Google Scholar]
- Yang J. T., Wu C. S., Martinez H. M. Calculation of protein conformation from circular dichroism. Methods Enzymol. 1986;130:208–269. doi: 10.1016/0076-6879(86)30013-2. [DOI] [PubMed] [Google Scholar]