Abstract
Quantitative predictions of steady-state muscle properties from the strain-dependent cross-bridge for muscle are presented. With a stiffness of 5.4 x 10(-4) N/m per head, a throw distance of 11 nm, and three allowed actin sites/head, isometric properties and their dependence on phosphate and nucleotide levels are well described if the tension-generating step occurs before phosphate release. At very low ATP levels, rigorlike states with negative strain are predicted. The rate-limiting step for cycling and ATP consumption is strain-blocked ADP release for isometric and slowly shortening muscle. Under rapid shortening, ATP hydrolysis on detached heads is the rate-limiting step, and the ratio of bound ATP to bound ADP.Pi increases by a factor of 7. At large positive strains, bound heads must be forcibly detached from actin to account for tension in rapid extension, but forced detachment in shortening has no effect without destroying isometric attached states. Strain-blocked phosphate release as proposed produces modest inhibition of the ATPase rate under rapid shortening, sufficient to give a maximum for one actin site per helix turn. Alternative cross-bridge models are discussed in the light of these predictions.
Full text
PDF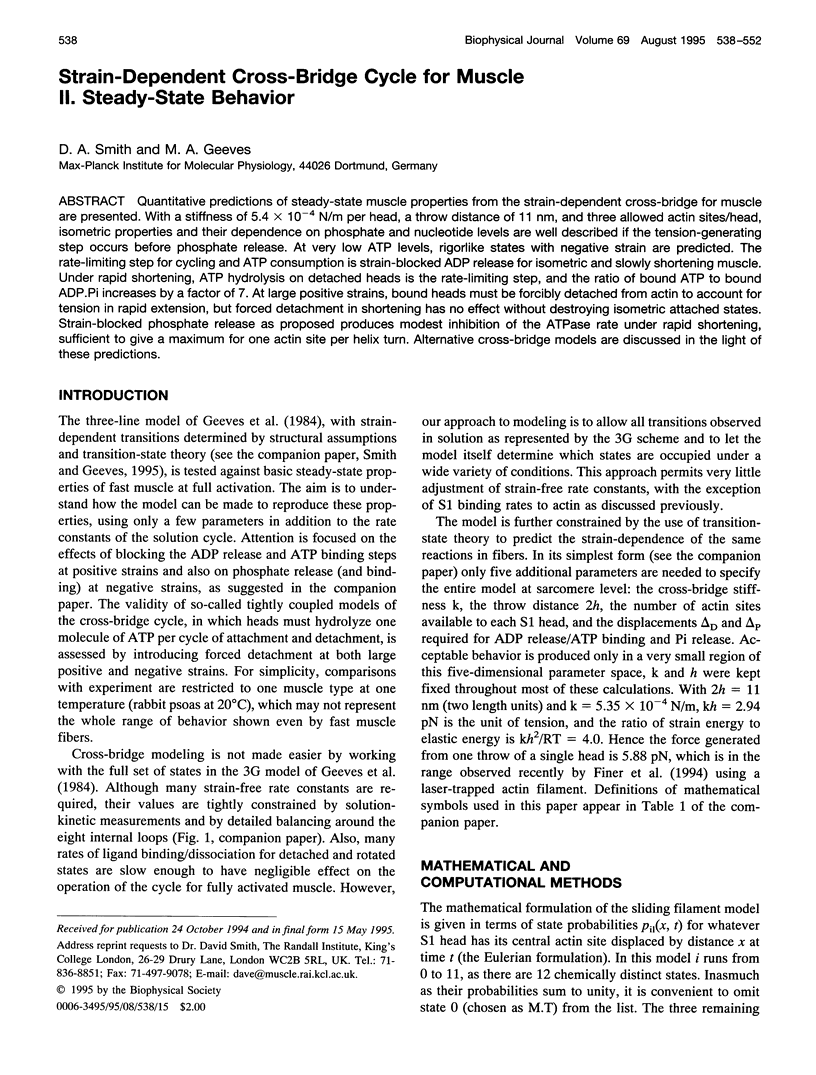
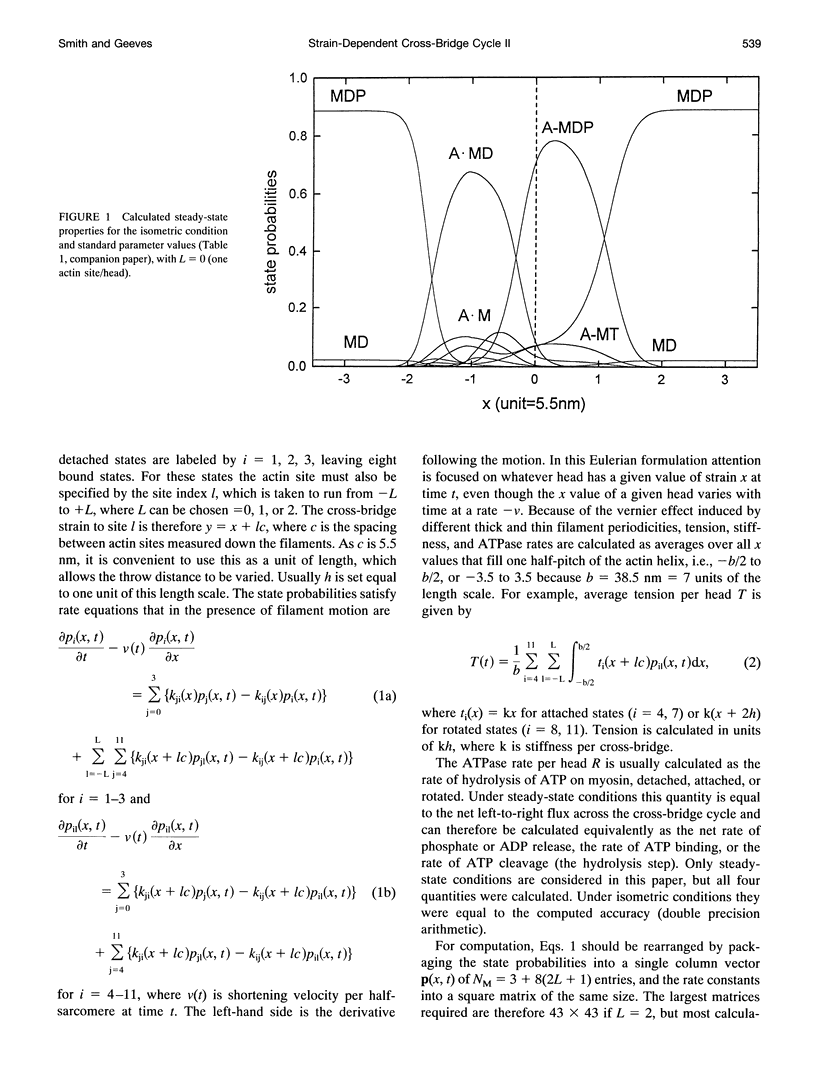
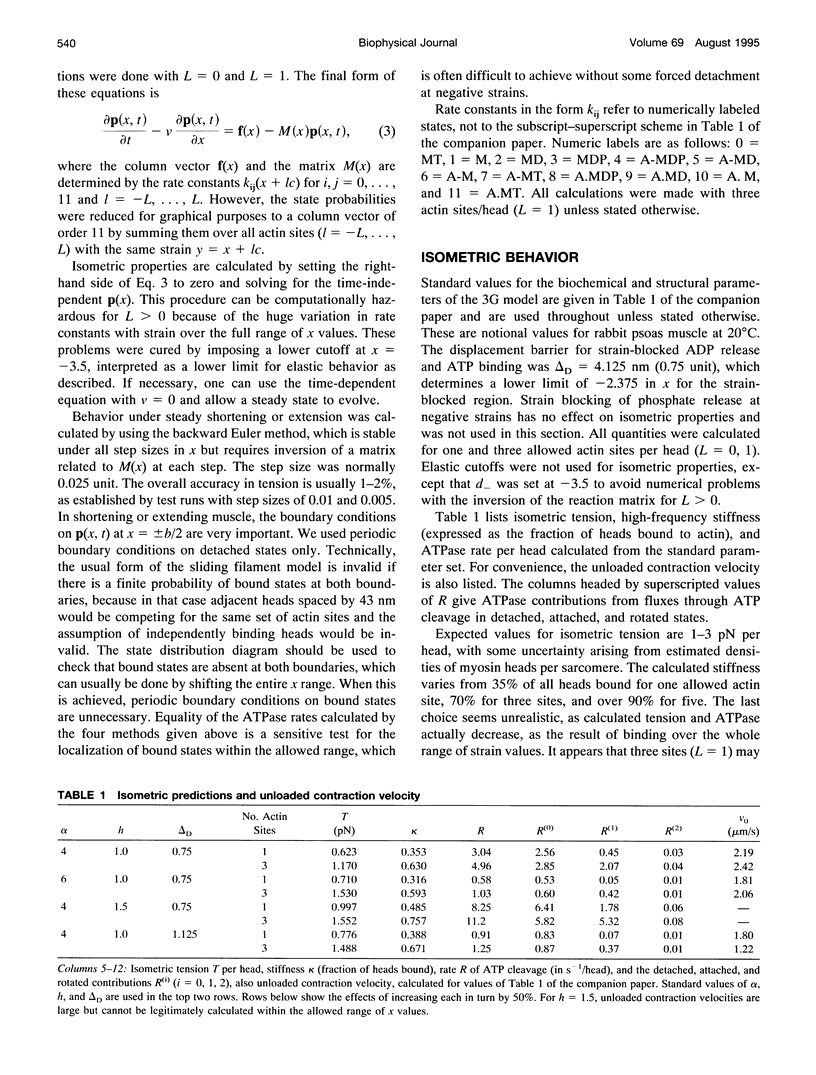
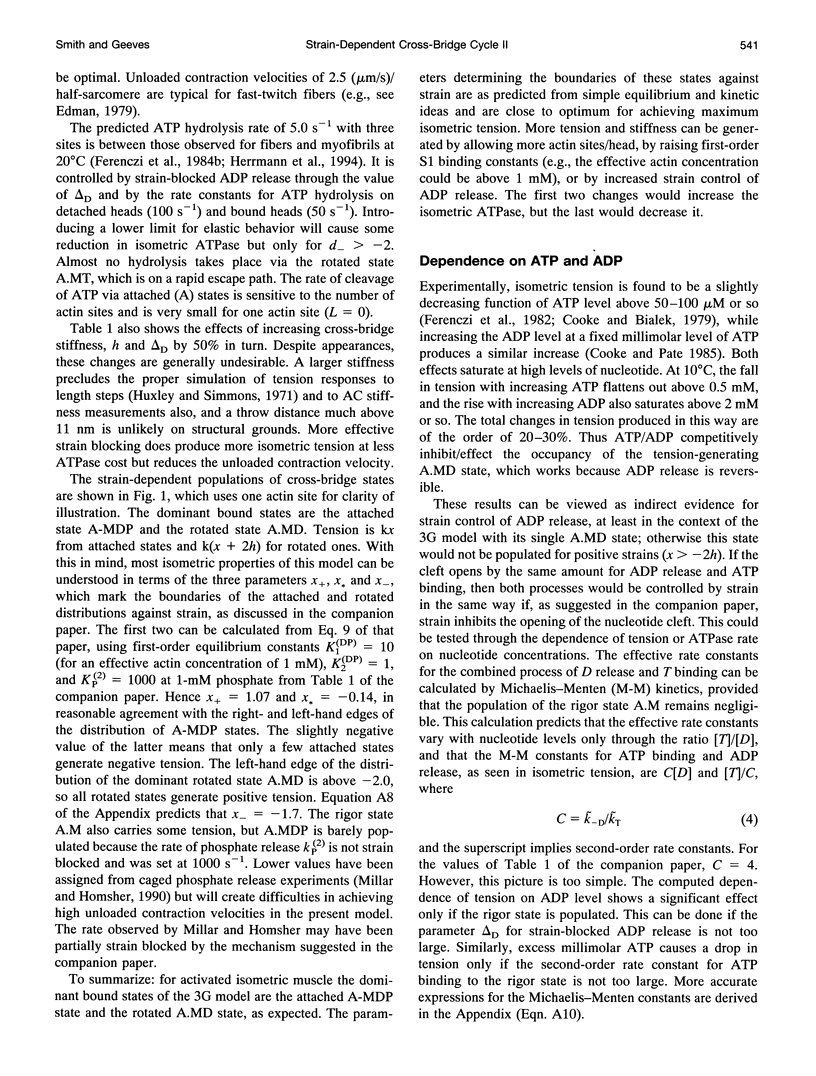
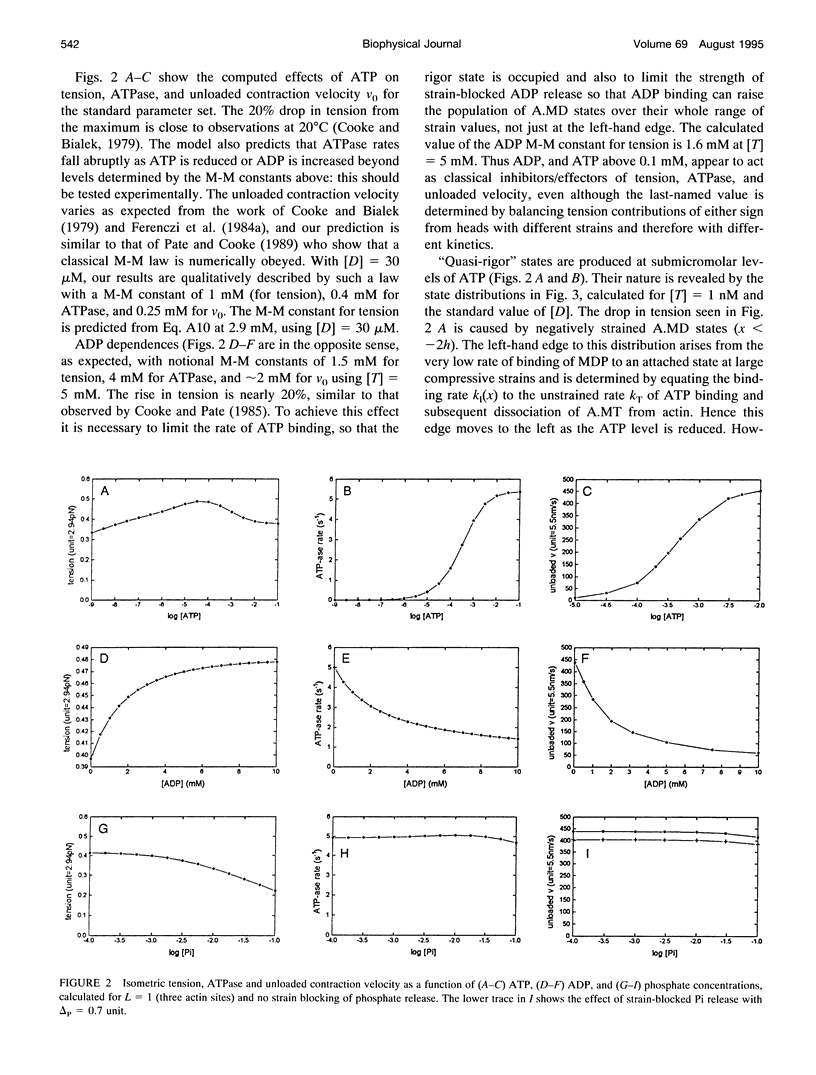
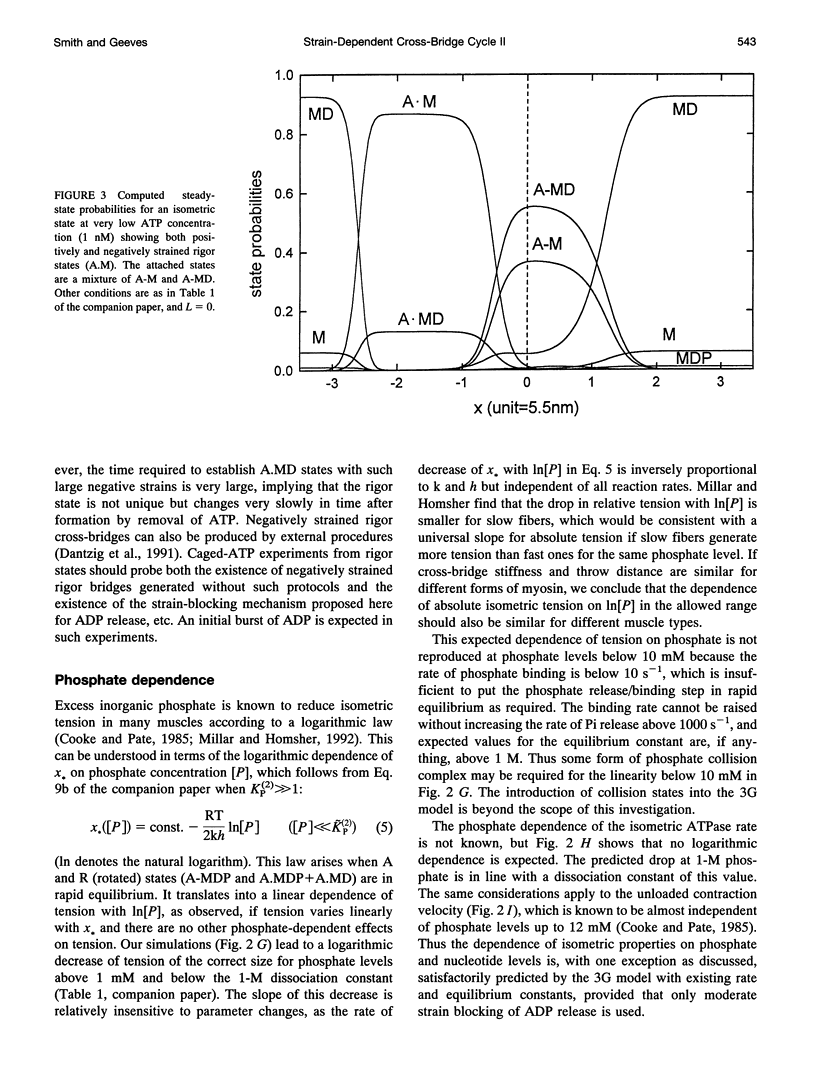
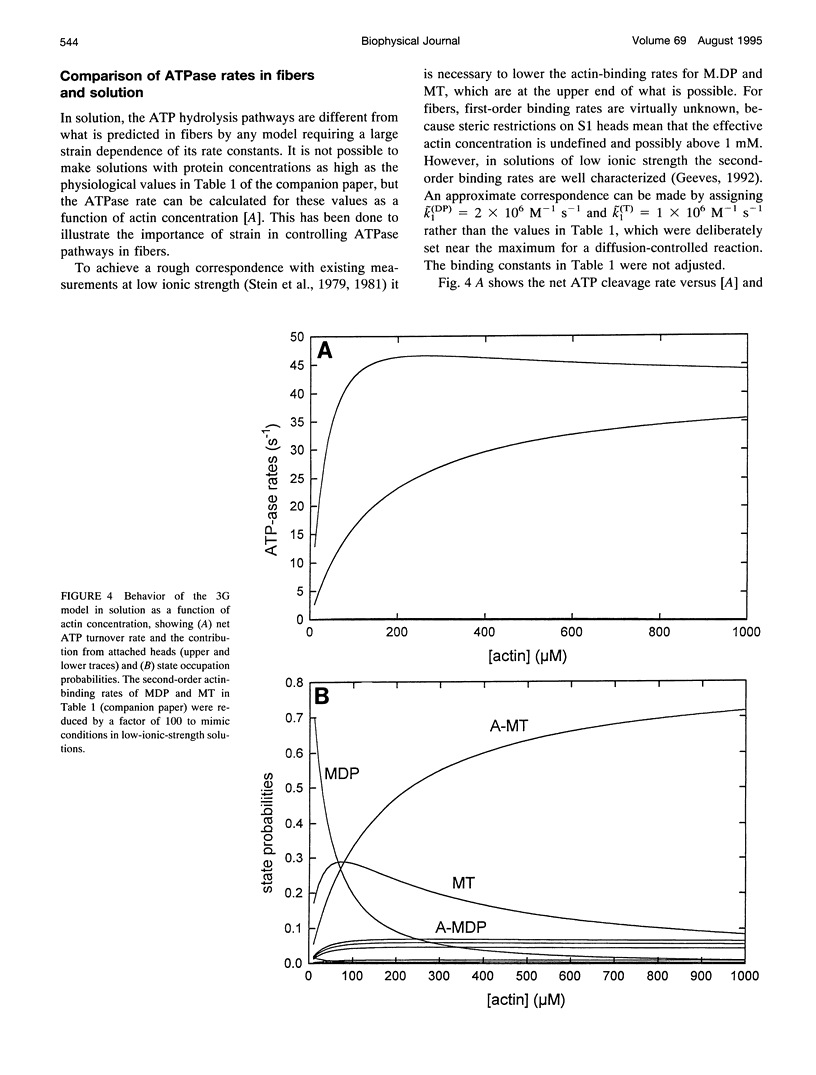
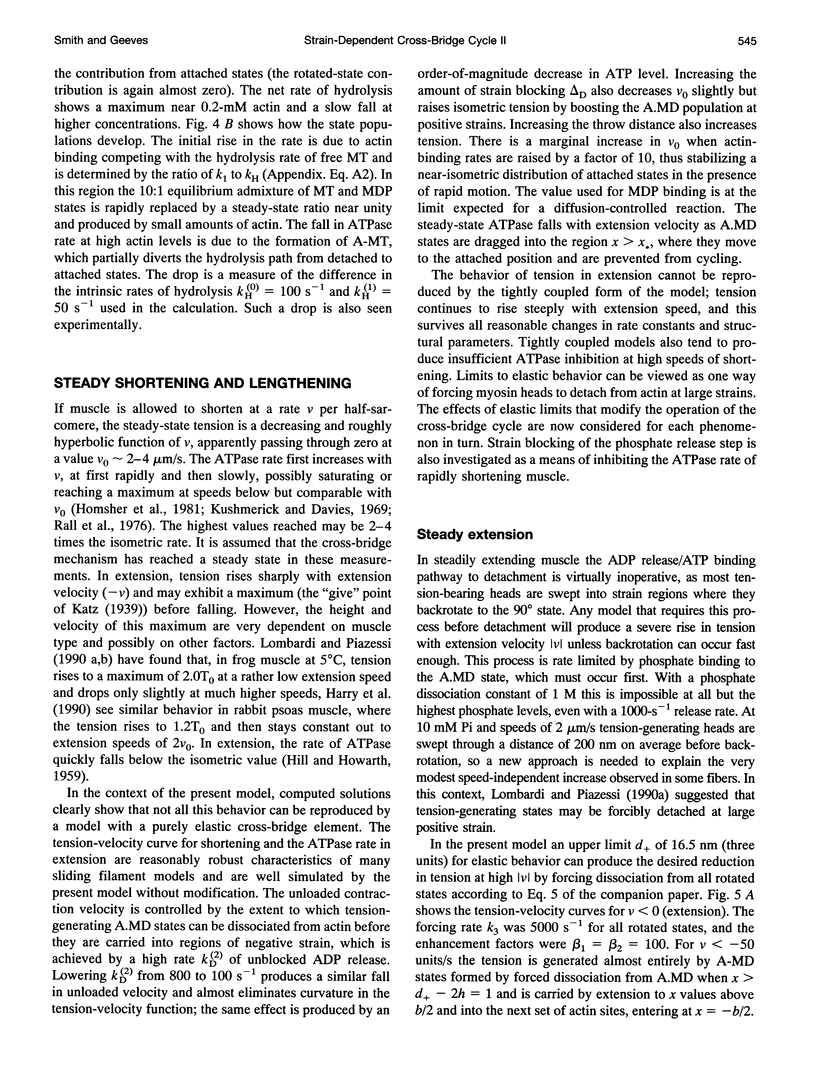
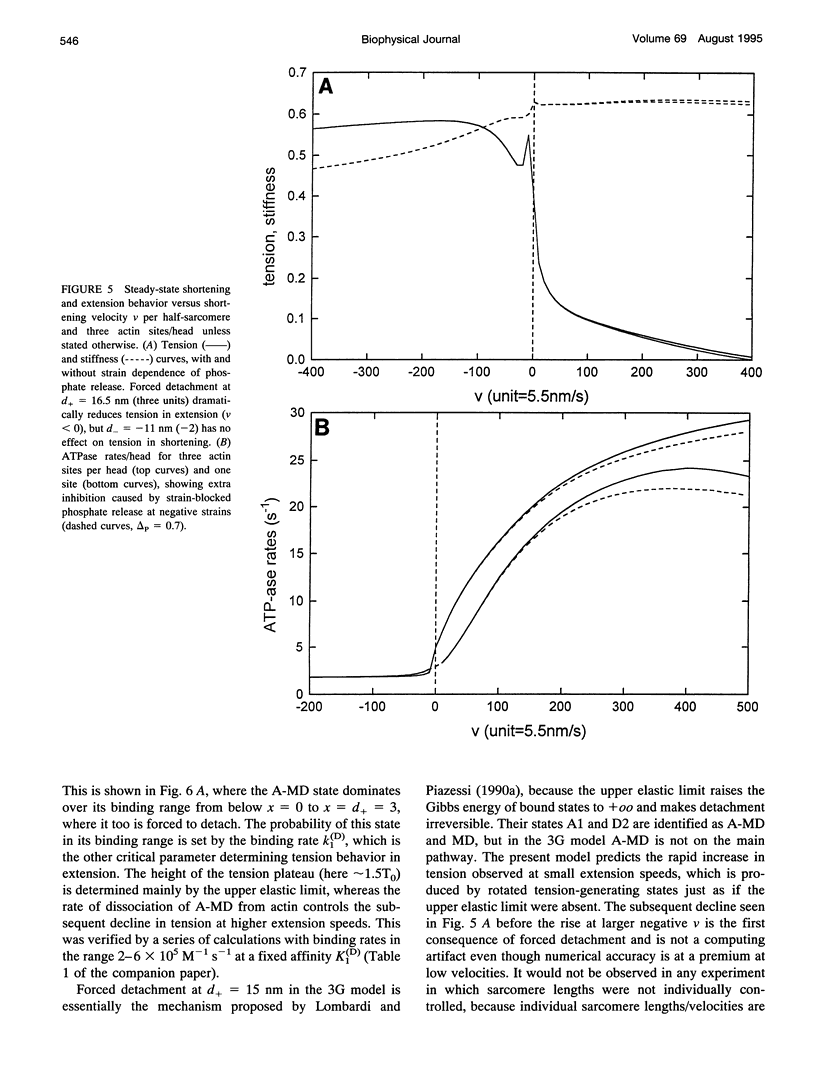
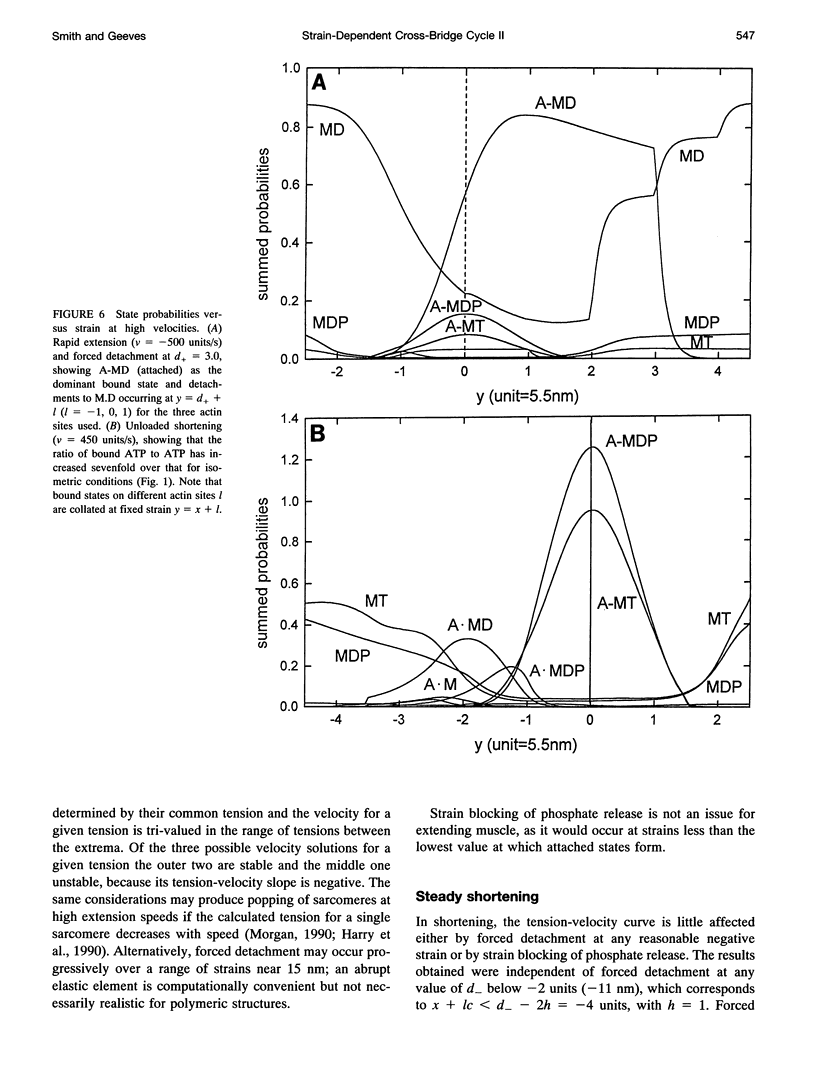
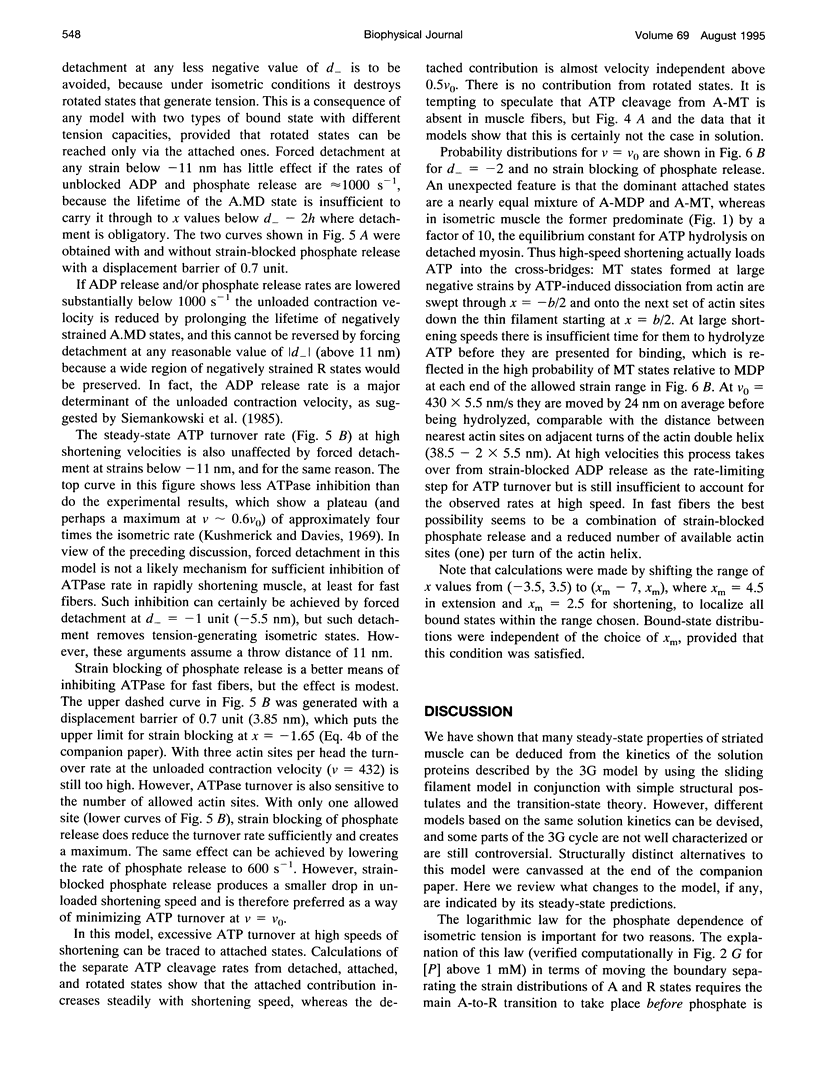
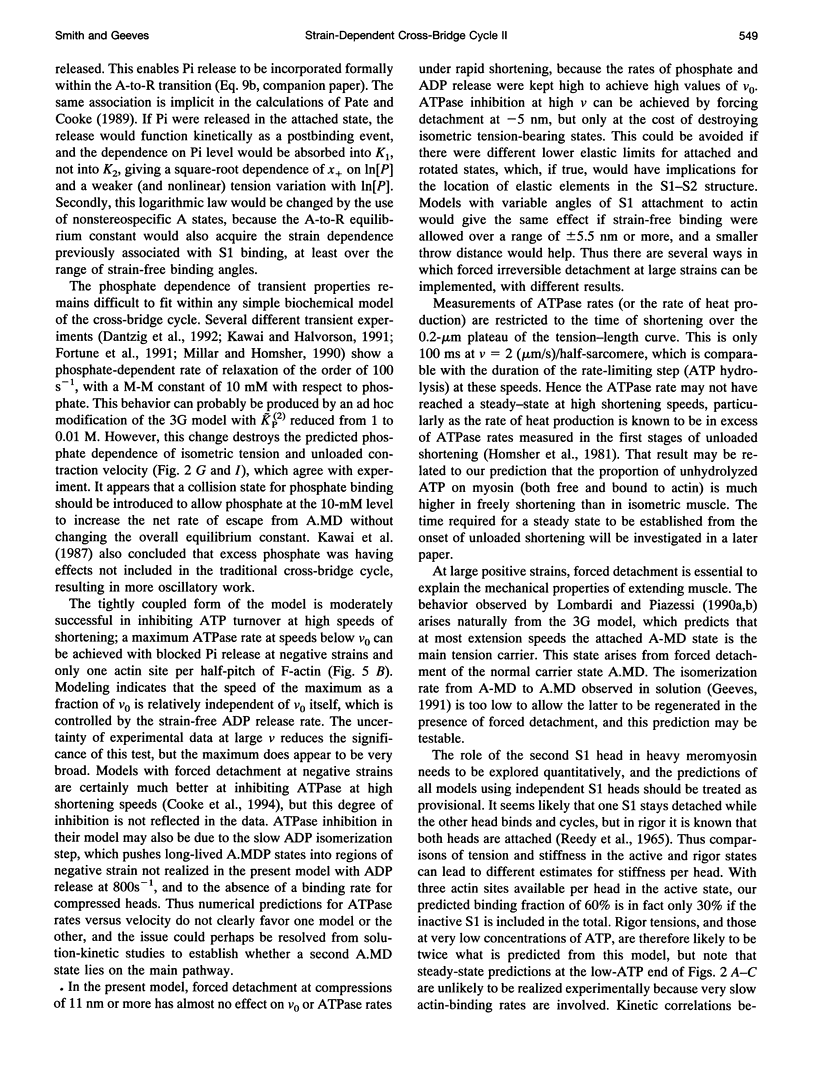
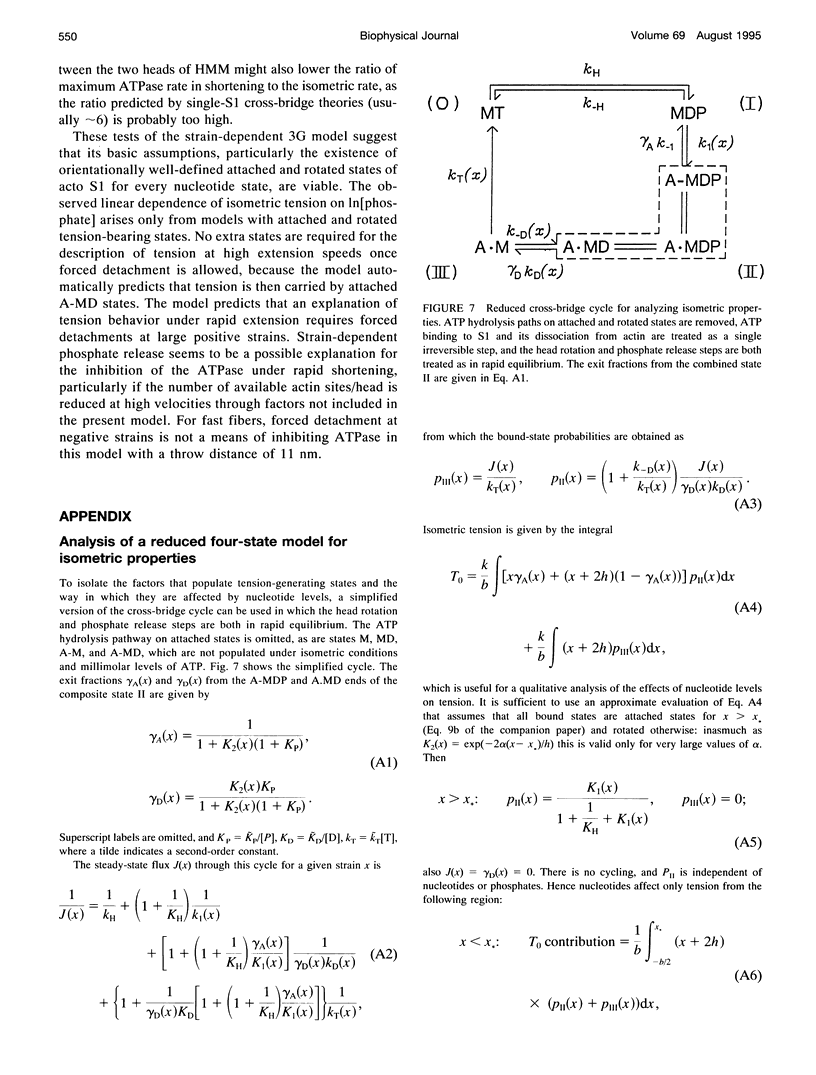
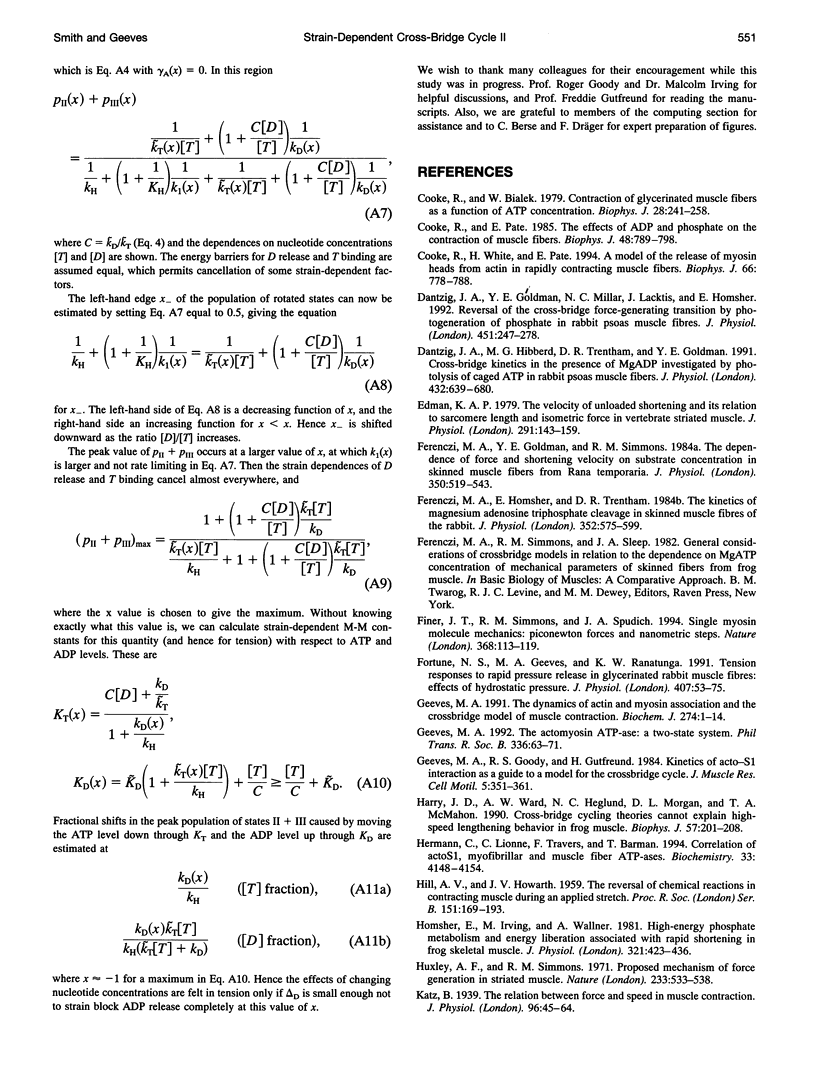
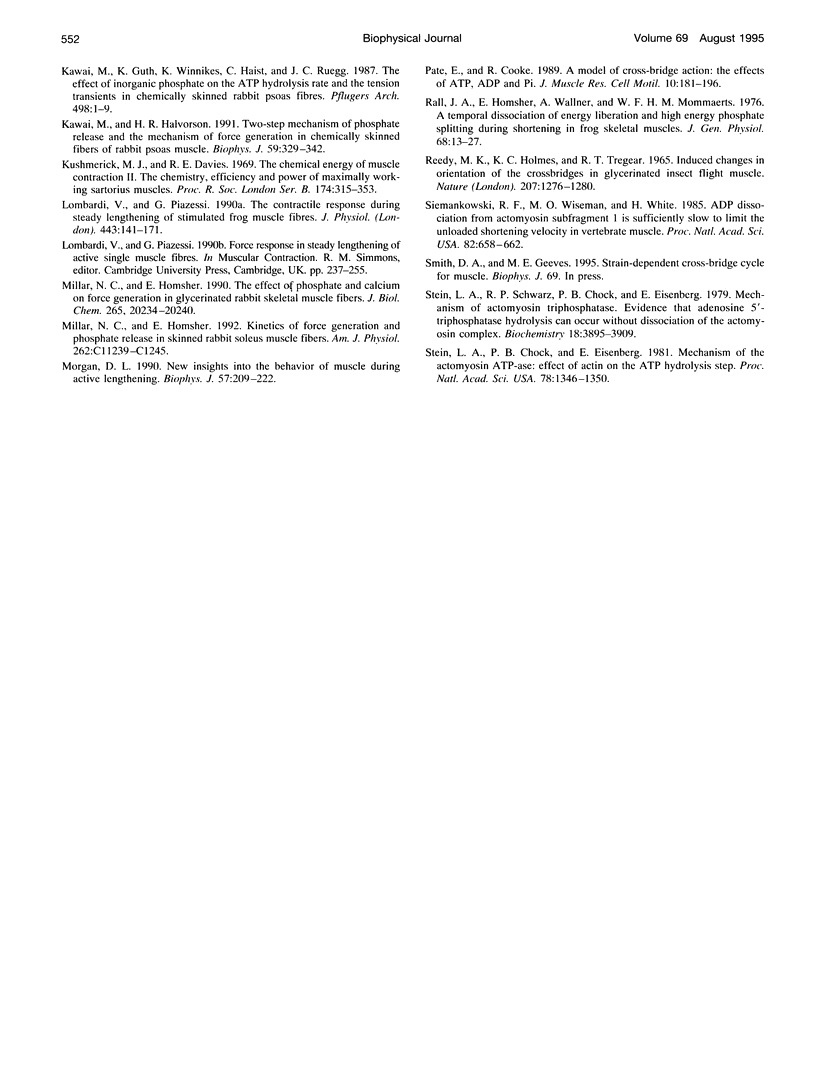
Selected References
These references are in PubMed. This may not be the complete list of references from this article.
- Cooke R., Bialek W. Contraction of glycerinated muscle fibers as a function of the ATP concentration. Biophys J. 1979 Nov;28(2):241–258. doi: 10.1016/S0006-3495(79)85174-7. [DOI] [PMC free article] [PubMed] [Google Scholar]
- Cooke R., Pate E. The effects of ADP and phosphate on the contraction of muscle fibers. Biophys J. 1985 Nov;48(5):789–798. doi: 10.1016/S0006-3495(85)83837-6. [DOI] [PMC free article] [PubMed] [Google Scholar]
- Cooke R., White H., Pate E. A model of the release of myosin heads from actin in rapidly contracting muscle fibers. Biophys J. 1994 Mar;66(3 Pt 1):778–788. doi: 10.1016/s0006-3495(94)80854-9. [DOI] [PMC free article] [PubMed] [Google Scholar]
- Dantzig J. A., Goldman Y. E., Millar N. C., Lacktis J., Homsher E. Reversal of the cross-bridge force-generating transition by photogeneration of phosphate in rabbit psoas muscle fibres. J Physiol. 1992;451:247–278. doi: 10.1113/jphysiol.1992.sp019163. [DOI] [PMC free article] [PubMed] [Google Scholar]
- Dantzig J. A., Hibberd M. G., Trentham D. R., Goldman Y. E. Cross-bridge kinetics in the presence of MgADP investigated by photolysis of caged ATP in rabbit psoas muscle fibres. J Physiol. 1991 Jan;432:639–680. doi: 10.1113/jphysiol.1991.sp018405. [DOI] [PMC free article] [PubMed] [Google Scholar]
- Edman K. A. The velocity of unloaded shortening and its relation to sarcomere length and isometric force in vertebrate muscle fibres. J Physiol. 1979 Jun;291:143–159. doi: 10.1113/jphysiol.1979.sp012804. [DOI] [PMC free article] [PubMed] [Google Scholar]
- Ferenczi M. A., Goldman Y. E., Simmons R. M. The dependence of force and shortening velocity on substrate concentration in skinned muscle fibres from Rana temporaria. J Physiol. 1984 May;350:519–543. doi: 10.1113/jphysiol.1984.sp015216. [DOI] [PMC free article] [PubMed] [Google Scholar]
- Ferenczi M. A., Homsher E., Trentham D. R. The kinetics of magnesium adenosine triphosphate cleavage in skinned muscle fibres of the rabbit. J Physiol. 1984 Jul;352:575–599. doi: 10.1113/jphysiol.1984.sp015311. [DOI] [PMC free article] [PubMed] [Google Scholar]
- Finer J. T., Simmons R. M., Spudich J. A. Single myosin molecule mechanics: piconewton forces and nanometre steps. Nature. 1994 Mar 10;368(6467):113–119. doi: 10.1038/368113a0. [DOI] [PubMed] [Google Scholar]
- Geeves M. A., Goody R. S., Gutfreund H. Kinetics of acto-S1 interaction as a guide to a model for the crossbridge cycle. J Muscle Res Cell Motil. 1984 Aug;5(4):351–361. doi: 10.1007/BF00818255. [DOI] [PubMed] [Google Scholar]
- Geeves M. A. The actomyosin ATPase: a two-state system. Philos Trans R Soc Lond B Biol Sci. 1992 Apr 29;336(1276):63–71. doi: 10.1098/rstb.1992.0045. [DOI] [PubMed] [Google Scholar]
- Geeves M. A. The dynamics of actin and myosin association and the crossbridge model of muscle contraction. Biochem J. 1991 Feb 15;274(Pt 1):1–14. doi: 10.1042/bj2740001. [DOI] [PMC free article] [PubMed] [Google Scholar]
- Harry J. D., Ward A. W., Heglund N. C., Morgan D. L., McMahon T. A. Cross-bridge cycling theories cannot explain high-speed lengthening behavior in frog muscle. Biophys J. 1990 Feb;57(2):201–208. doi: 10.1016/S0006-3495(90)82523-6. [DOI] [PMC free article] [PubMed] [Google Scholar]
- Herrmann C., Lionne C., Travers F., Barman T. Correlation of ActoS1, myofibrillar, and muscle fiber ATPases. Biochemistry. 1994 Apr 12;33(14):4148–4154. doi: 10.1021/bi00180a007. [DOI] [PubMed] [Google Scholar]
- Homsher E., Irving M., Wallner A. High-energy phosphate metabolism and energy liberation associated with rapid shortening in frog skeletal muscle. J Physiol. 1981 Dec;321:423–436. doi: 10.1113/jphysiol.1981.sp013994. [DOI] [PMC free article] [PubMed] [Google Scholar]
- Huxley A. F., Simmons R. M. Proposed mechanism of force generation in striated muscle. Nature. 1971 Oct 22;233(5321):533–538. doi: 10.1038/233533a0. [DOI] [PubMed] [Google Scholar]
- Katz B. The relation between force and speed in muscular contraction. J Physiol. 1939 Jun 14;96(1):45–64. doi: 10.1113/jphysiol.1939.sp003756. [DOI] [PMC free article] [PubMed] [Google Scholar]
- Kawai M., Güth K., Winnikes K., Haist C., Rüegg J. C. The effect of inorganic phosphate on the ATP hydrolysis rate and the tension transients in chemically skinned rabbit psoas fibers. Pflugers Arch. 1987 Jan;408(1):1–9. doi: 10.1007/BF00581833. [DOI] [PubMed] [Google Scholar]
- Kawai M., Halvorson H. R. Two step mechanism of phosphate release and the mechanism of force generation in chemically skinned fibers of rabbit psoas muscle. Biophys J. 1991 Feb;59(2):329–342. doi: 10.1016/S0006-3495(91)82227-5. [DOI] [PMC free article] [PubMed] [Google Scholar]
- Kushmerick M. J., Davies R. E. The chemical energetics of muscle contraction. II. The chemistry, efficiency and power of maximally working sartorius muscles. Appendix. Free energy and enthalpy of atp hydrolysis in the sarcoplasm. Proc R Soc Lond B Biol Sci. 1969 Dec 23;174(1036):315–353. doi: 10.1098/rspb.1969.0096. [DOI] [PubMed] [Google Scholar]
- Millar N. C., Homsher E. Kinetics of force generation and phosphate release in skinned rabbit soleus muscle fibers. Am J Physiol. 1992 May;262(5 Pt 1):C1239–C1245. doi: 10.1152/ajpcell.1992.262.5.C1239. [DOI] [PubMed] [Google Scholar]
- Millar N. C., Homsher E. The effect of phosphate and calcium on force generation in glycerinated rabbit skeletal muscle fibers. A steady-state and transient kinetic study. J Biol Chem. 1990 Nov 25;265(33):20234–20240. [PubMed] [Google Scholar]
- Morgan D. L. New insights into the behavior of muscle during active lengthening. Biophys J. 1990 Feb;57(2):209–221. doi: 10.1016/S0006-3495(90)82524-8. [DOI] [PMC free article] [PubMed] [Google Scholar]
- Pate E., Cooke R. A model of crossbridge action: the effects of ATP, ADP and Pi. J Muscle Res Cell Motil. 1989 Jun;10(3):181–196. doi: 10.1007/BF01739809. [DOI] [PubMed] [Google Scholar]
- Rall J. A., Homsher E., Wallner A., Mommaerts W. F. A temporal dissociation of energy liberation and high energy phosphate splitting during shortening in frog skeletal muscles. J Gen Physiol. 1976 Jul;68(1):13–27. doi: 10.1085/jgp.68.1.13. [DOI] [PMC free article] [PubMed] [Google Scholar]
- Reedy M. K., Holmes K. C., Tregear R. T. Induced changes in orientation of the cross-bridges of glycerinated insect flight muscle. Nature. 1965 Sep 18;207(5003):1276–1280. doi: 10.1038/2071276a0. [DOI] [PubMed] [Google Scholar]
- Siemankowski R. F., Wiseman M. O., White H. D. ADP dissociation from actomyosin subfragment 1 is sufficiently slow to limit the unloaded shortening velocity in vertebrate muscle. Proc Natl Acad Sci U S A. 1985 Feb;82(3):658–662. doi: 10.1073/pnas.82.3.658. [DOI] [PMC free article] [PubMed] [Google Scholar]
- Stein L. A., Chock P. B., Eisenberg E. Mechanism of the actomyosin ATPase: effect of actin on the ATP hydrolysis step. Proc Natl Acad Sci U S A. 1981 Mar;78(3):1346–1350. doi: 10.1073/pnas.78.3.1346. [DOI] [PMC free article] [PubMed] [Google Scholar]
- Stein L. A., Schwarz R. P., Jr, Chock P. B., Eisenberg E. Mechanism of actomyosin adenosine triphosphatase. Evidence that adenosine 5'-triphosphate hydrolysis can occur without dissociation of the actomyosin complex. Biochemistry. 1979 Sep 4;18(18):3895–3909. doi: 10.1021/bi00585a009. [DOI] [PubMed] [Google Scholar]