Abstract
Immobilized protein solute, approximately 20 wt %, alters the longitudinal and transverse nuclear magnetic relaxation rates 1/T1 and 1/T2 of solvent water protons in a manner that makes their values indistinguishable from those of a typical human tissue. There is now a quantitative theory at the molecular level (S.H. Koenig and R. D. Brown III (1993) Magn. Reson. Med. 30:685-695) that accounts for this, as a function of magnetic field strength, in terms of several distinguishable classes of water-binding sites at the protein-water interface at which significant relaxation and solute-solvent transfer of proton Zeeman energy occur. We review the arguments that these several classes of sites, characterized by widely disparate values of the resident lifetimes tau M of the bound waters, are associated with different numbers of hydrogen bonds that stabilize the particular protein-water complex. The sites that dominate relaxation-and produce contrast in magnetic resonance imaging (MRI), which derives from 1/T1 and 1/T2 of tissue water protons-have tau M approximately 10(-6)s. These, which involve four hydrogen bonds, occupy < or = 1% of the protein-water interface. Sites that involve three bonds, although more numerous, have < or = 20% smaller intrinsic effect on relaxation. The greater part of the "traditional" hydration monolayer, with even shorter-lived hydrogen-bonded waters, has little influence on solvent relaxation and is relatively unimportant in MRI. Finally, we argue, from the data, that most of the protein of tissue (a typical tissue is mostly protein) must be rotationally immobile (with Brownian rotational relaxation times slower than that of a 5 x 10(7) Da (very heavy) globular protein). We propose a functional basis for this immobilization ("cytoplasmic order"), and then indicate a way in which this order can break down ("cytoplasmic chaos") as a result of neoplastic transformation (cancer) and alter water-proton rates of pathological tissue and, hence, image contrast in MRI.
Full text
PDF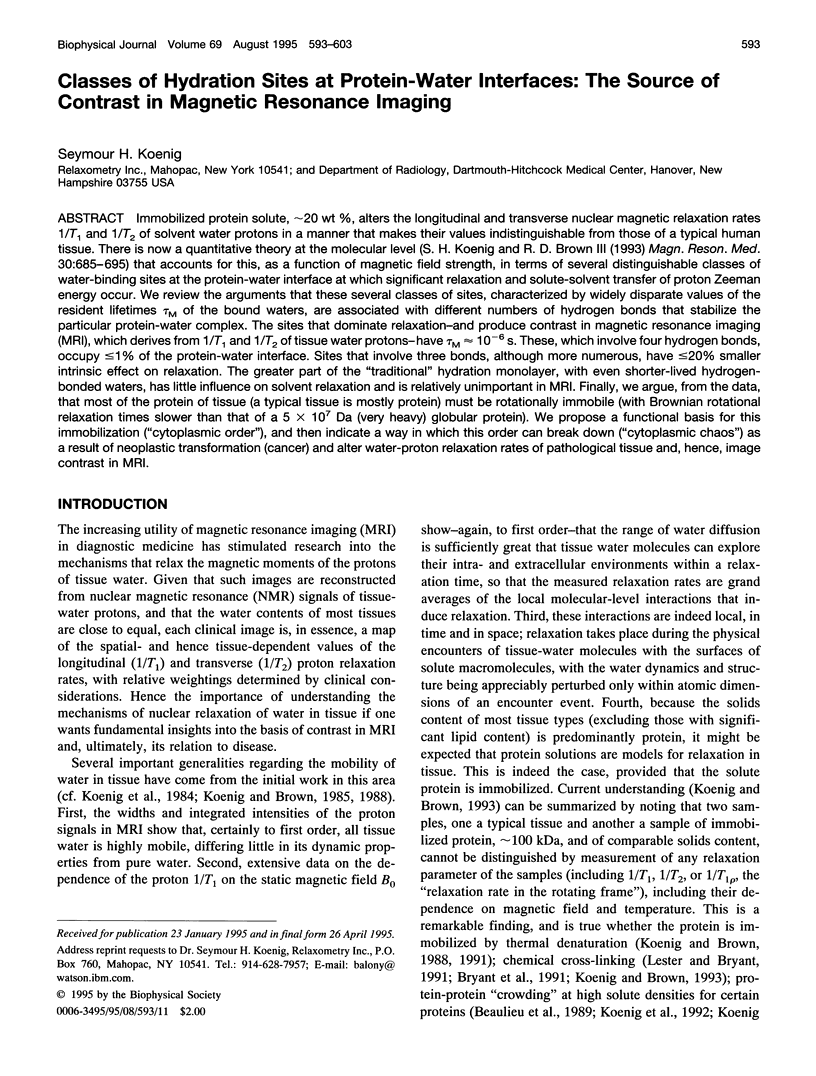
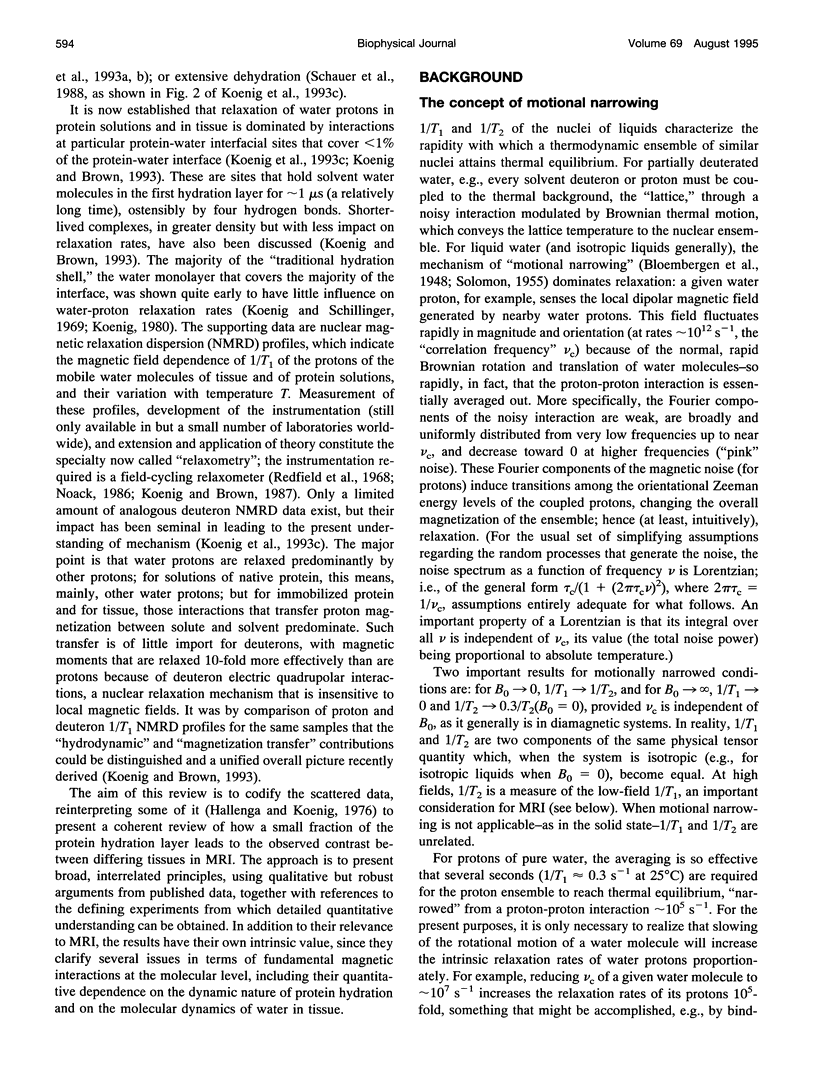
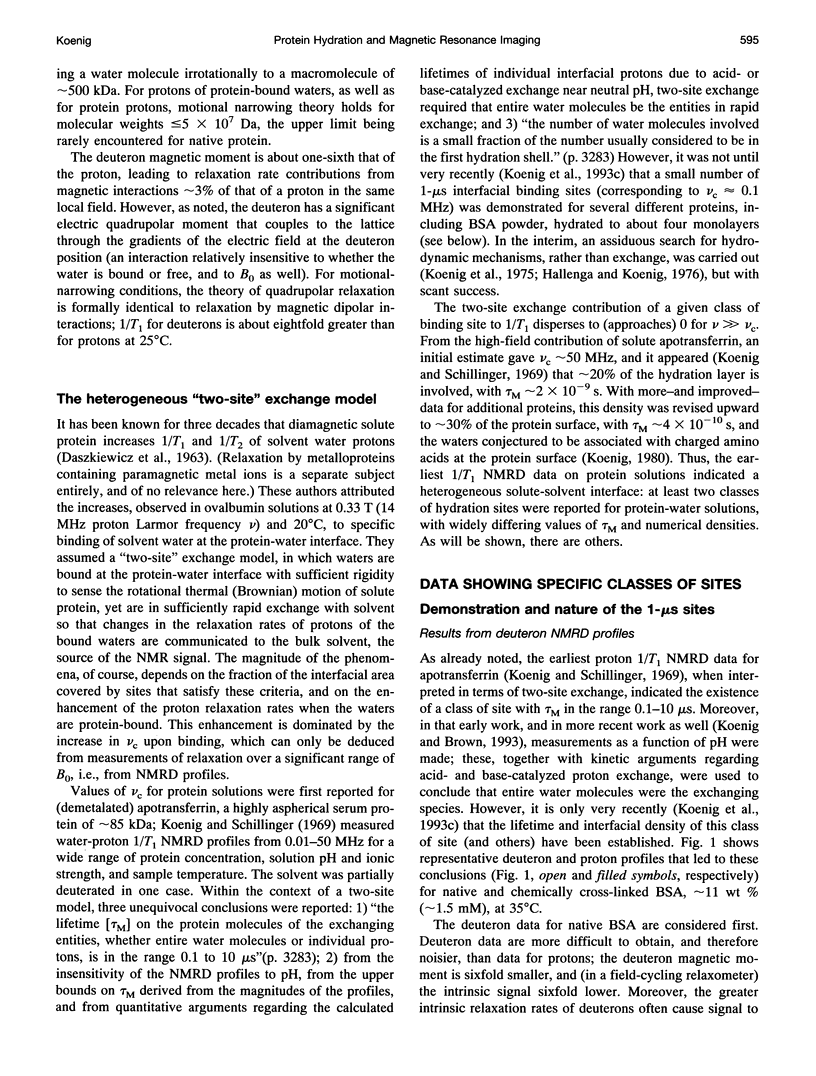
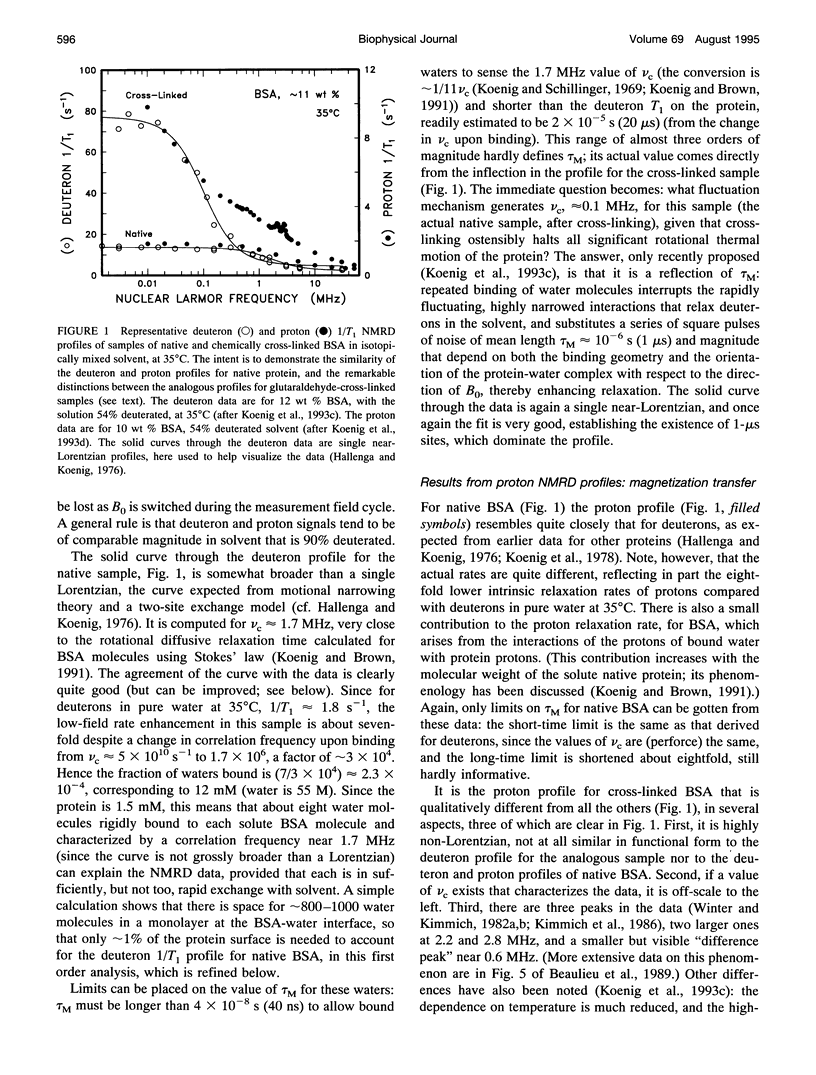
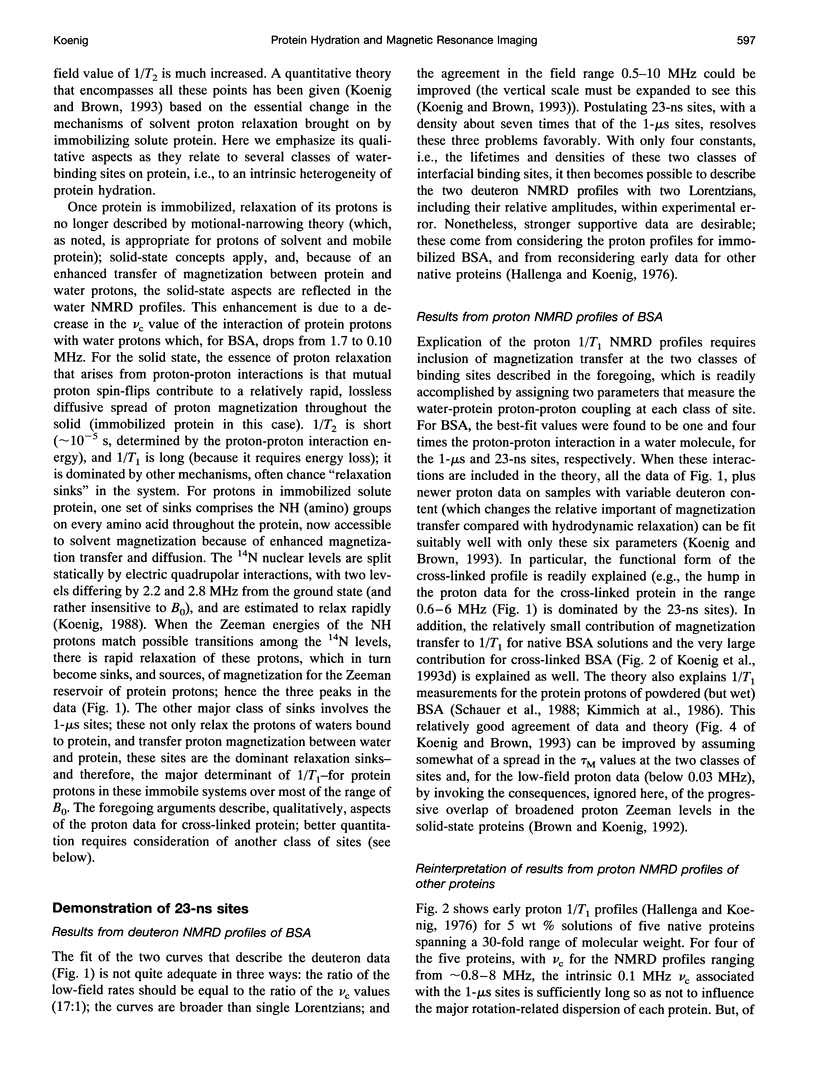
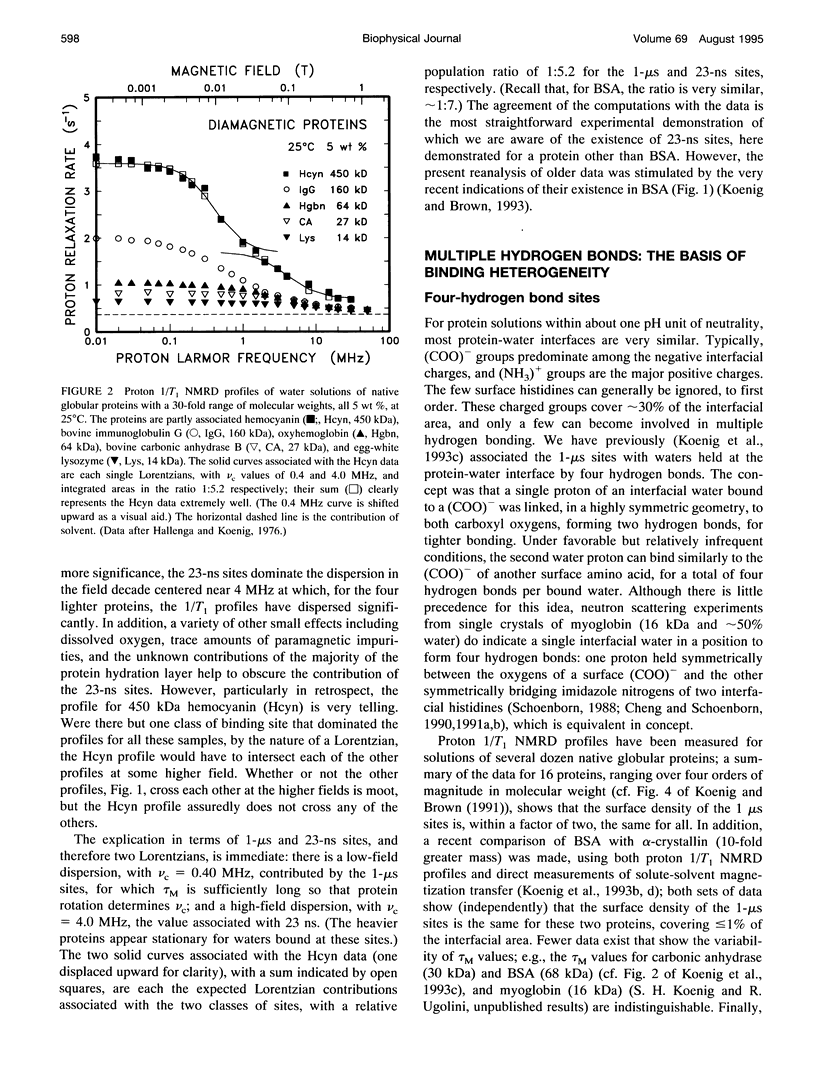
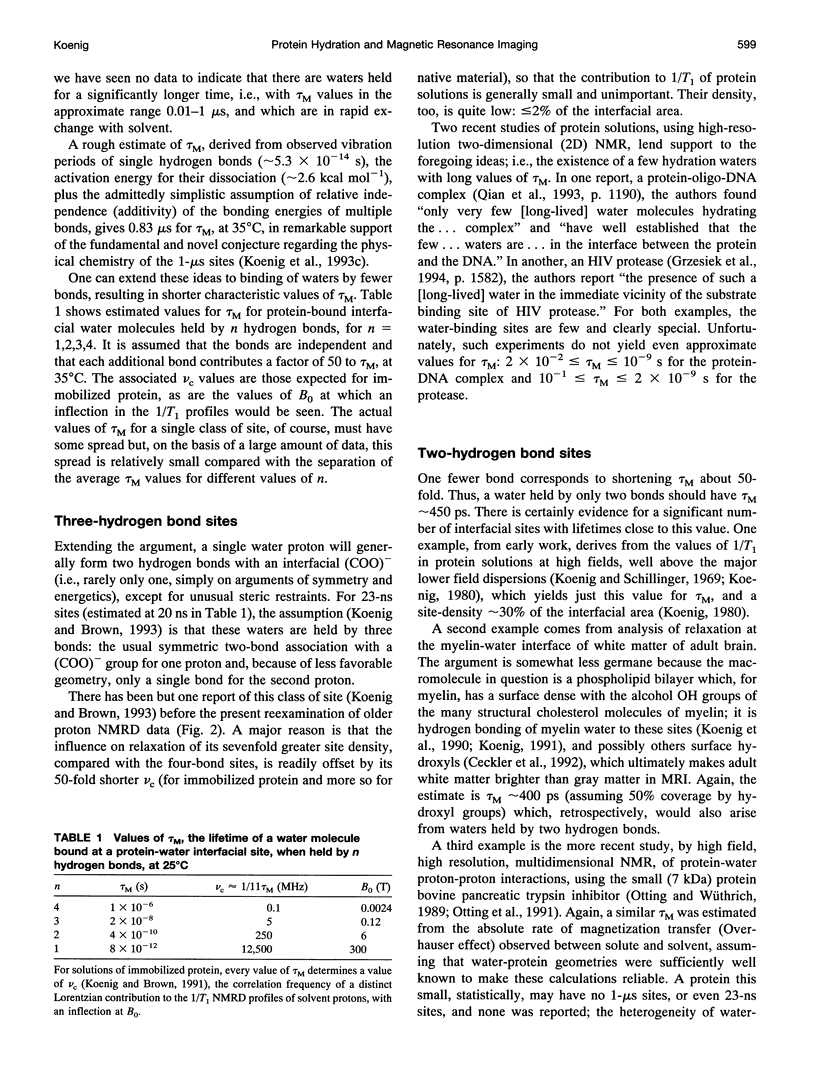
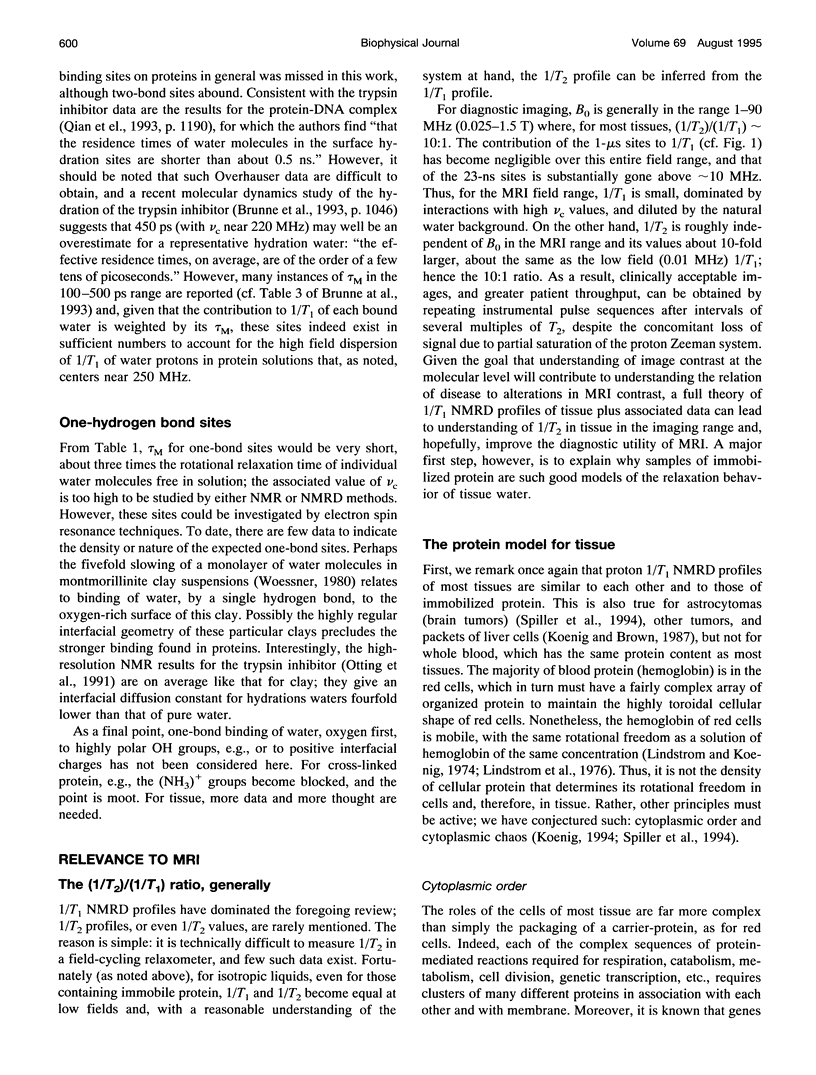
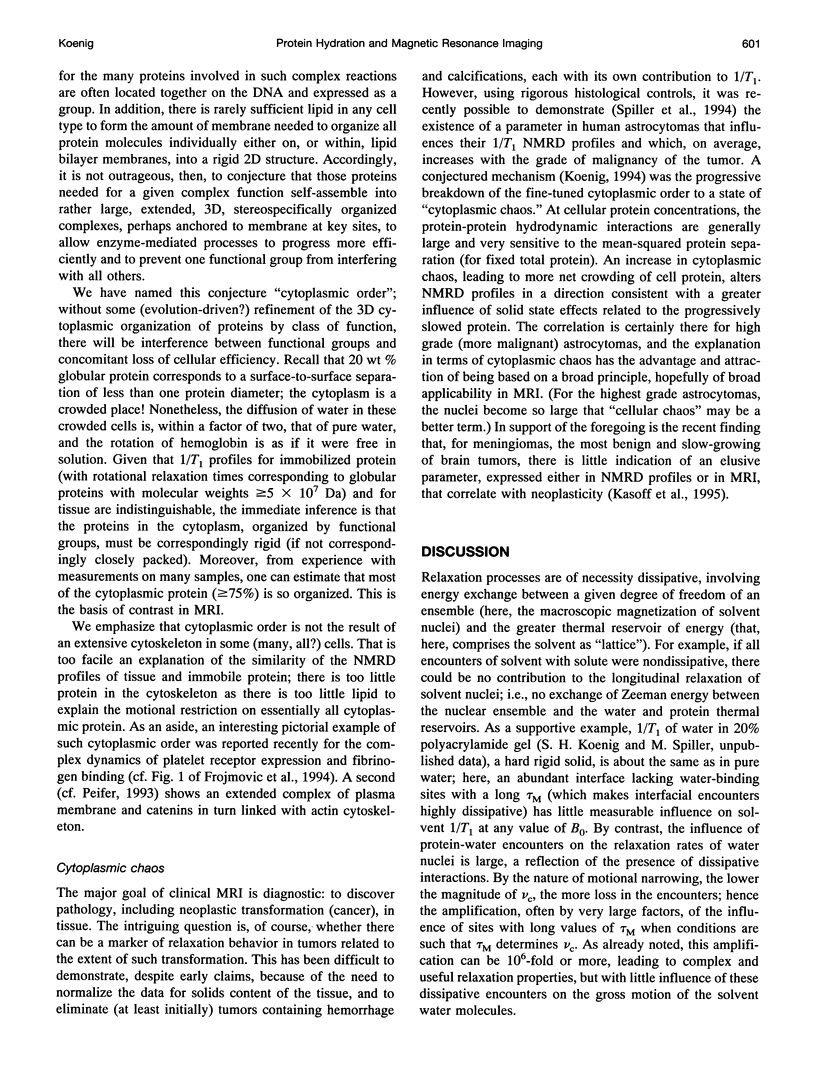
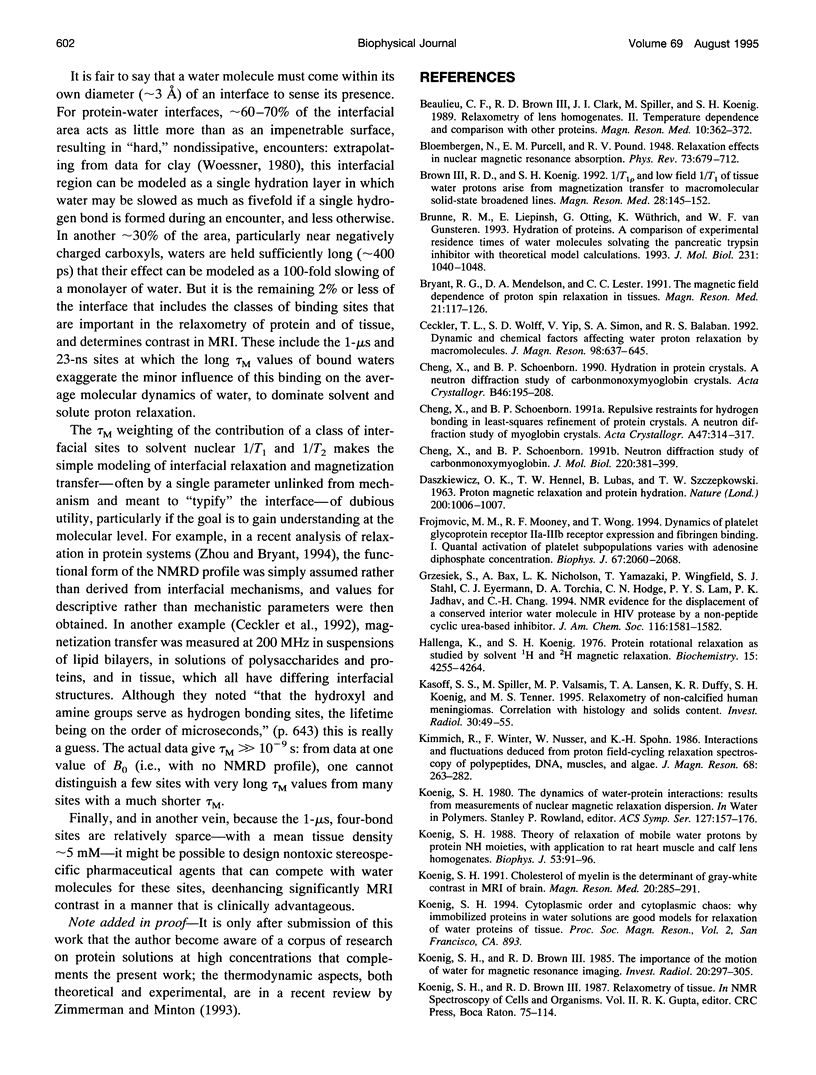
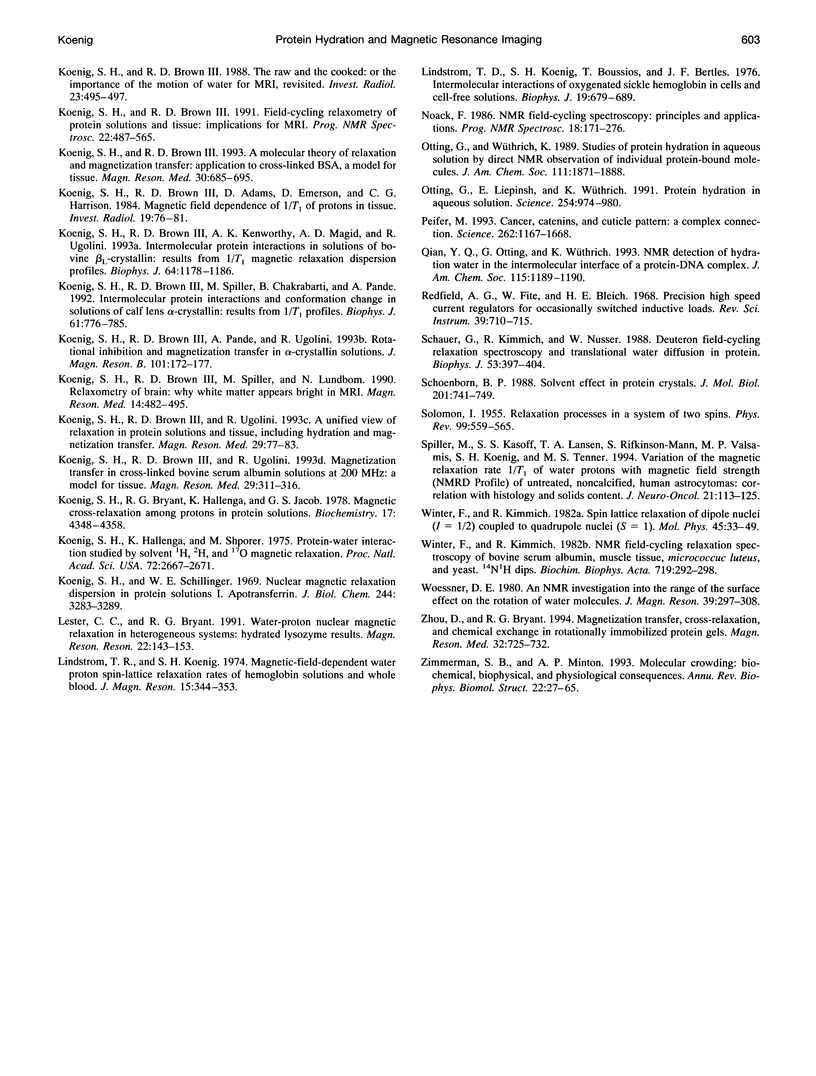
Selected References
These references are in PubMed. This may not be the complete list of references from this article.
- Beaulieu C. F., Brown R. D., 3rd, Clark J. I., Spiller M., Koenig S. H. Relaxometry of lens homogenates. II. Temperature dependence and comparison with other proteins. Magn Reson Med. 1989 Jun;10(3):362–372. doi: 10.1002/mrm.1910100308. [DOI] [PubMed] [Google Scholar]
- Brown R. D., 3rd, Koenig S. H. 1/T1 rho and low-field 1/T1 of tissue water protons arise from magnetization transfer to macromolecular solid-state broadened lines. Magn Reson Med. 1992 Nov;28(1):145–152. doi: 10.1002/mrm.1910280115. [DOI] [PubMed] [Google Scholar]
- Brunne R. M., Liepinsh E., Otting G., Wüthrich K., van Gunsteren W. F. Hydration of proteins. A comparison of experimental residence times of water molecules solvating the bovine pancreatic trypsin inhibitor with theoretical model calculations. J Mol Biol. 1993 Jun 20;231(4):1040–1048. doi: 10.1006/jmbi.1993.1350. [DOI] [PubMed] [Google Scholar]
- Bryant R. G., Mendelson D. A., Lester C. C. The magnetic field dependence of proton spin relaxation in tissues. Magn Reson Med. 1991 Sep;21(1):117–126. doi: 10.1002/mrm.1910210114. [DOI] [PubMed] [Google Scholar]
- Cheng X. D., Schoenborn B. P. Neutron diffraction study of carbonmonoxymyoglobin. J Mol Biol. 1991 Jul 20;220(2):381–399. doi: 10.1016/0022-2836(91)90020-7. [DOI] [PubMed] [Google Scholar]
- Frojmovic M. M., Mooney R. F., Wong T. Dynamics of platelet glycoprotein IIb-IIIa receptor expression and fibrinogen binding. I. Quantal activation of platelet subpopulations varies with adenosine diphosphate concentration. Biophys J. 1994 Nov;67(5):2060–2068. doi: 10.1016/S0006-3495(94)80689-7. [DOI] [PMC free article] [PubMed] [Google Scholar]
- Hallenga K., Koenig S. H. Protein rotational relaxation as studied by solvent 1H and 2H magnetic relaxation. Biochemistry. 1976 Sep 21;15(19):4255–4264. doi: 10.1021/bi00664a019. [DOI] [PubMed] [Google Scholar]
- Kasoff S. S., Spiller M., Valsamis M. P., Lansen T. A., Duffy K. R., Koenig S. H., Tenner M. S. Relaxometry of noncalcified human meningiomas. Correlation with histology and solids content. Invest Radiol. 1995 Jan;30(1):49–55. [PubMed] [Google Scholar]
- Koenig S. H., Brown R. D., 3rd A molecular theory of relaxation and magnetization transfer: application to cross-linked BSA, a model for tissue. Magn Reson Med. 1993 Dec;30(6):685–695. doi: 10.1002/mrm.1910300606. [DOI] [PubMed] [Google Scholar]
- Koenig S. H., Brown R. D., 3rd, Adams D., Emerson D., Harrison C. G. Magnetic field dependence of 1/T1 of protons in tissue. Invest Radiol. 1984 Mar-Apr;19(2):76–81. doi: 10.1097/00004424-198403000-00002. [DOI] [PubMed] [Google Scholar]
- Koenig S. H., Brown R. D., 3rd, Kenworthy A. K., Magid A. D., Ugolini R. Intermolecular protein interactions in solutions of bovine lens beta L-crystallin. Results from 1/T1 nuclear magnetic relaxation dispersion profiles. Biophys J. 1993 Apr;64(4):1178–1186. doi: 10.1016/S0006-3495(93)81483-8. [DOI] [PMC free article] [PubMed] [Google Scholar]
- Koenig S. H., Brown R. D., 3rd, Spiller M., Chakrabarti B., Pande A. Intermolecular protein interactions in solutions of calf lens alpha-crystallin. Results from 1/T1 nuclear magnetic relaxation dispersion profiles. Biophys J. 1992 Mar;61(3):776–785. doi: 10.1016/S0006-3495(92)81882-9. [DOI] [PMC free article] [PubMed] [Google Scholar]
- Koenig S. H., Brown R. D., 3rd, Spiller M., Lundbom N. Relaxometry of brain: why white matter appears bright in MRI. Magn Reson Med. 1990 Jun;14(3):482–495. doi: 10.1002/mrm.1910140306. [DOI] [PubMed] [Google Scholar]
- Koenig S. H., Brown R. D., 3rd The importance of the motion of water for magnetic resonance imaging. Invest Radiol. 1985 May-Jun;20(3):297–305. doi: 10.1097/00004424-198505000-00013. [DOI] [PubMed] [Google Scholar]
- Koenig S. H., Brown R. D., 3rd The raw and the cooked, or the importance of the motion of water for MRI revisited. Invest Radiol. 1988 Jul;23(7):495–497. doi: 10.1097/00004424-198807000-00002. [DOI] [PubMed] [Google Scholar]
- Koenig S. H., Brown R. D., 3rd, Ugolini R. A unified view of relaxation in protein solutions and tissue, including hydration and magnetization transfer. Magn Reson Med. 1993 Jan;29(1):77–83. doi: 10.1002/mrm.1910290114. [DOI] [PubMed] [Google Scholar]
- Koenig S. H., Bryant R. G., Hallenga K., Jacob G. S. Magnetic cross-relaxation among protons in protein solutions. Biochemistry. 1978 Oct 3;17(20):4348–4358. doi: 10.1021/bi00613a037. [DOI] [PubMed] [Google Scholar]
- Koenig S. H. Cholesterol of myelin is the determinant of gray-white contrast in MRI of brain. Magn Reson Med. 1991 Aug;20(2):285–291. doi: 10.1002/mrm.1910200210. [DOI] [PubMed] [Google Scholar]
- Koenig S. H., Hallenga K., Shporer M. Protein-water interaction studied by solvent 1H, 2H, and 17O magnetic relaxation. Proc Natl Acad Sci U S A. 1975 Jul;72(7):2667–2671. doi: 10.1073/pnas.72.7.2667. [DOI] [PMC free article] [PubMed] [Google Scholar]
- Koenig S. H., Schillinger W. E. Nuclear magnetic relaxation dispersion in protein solutions. I. Apotransferrin. J Biol Chem. 1969 Jun 25;244(12):3283–3289. [PubMed] [Google Scholar]
- Koenig S. H. Theory of relaxation of mobile water protons induced by protein NH moieties, with application to rat heart muscle and calf lens homogenates. Biophys J. 1988 Jan;53(1):91–96. doi: 10.1016/S0006-3495(88)83069-8. [DOI] [PMC free article] [PubMed] [Google Scholar]
- Lester C. C., Bryant R. G. Water-proton nuclear magnetic relaxation in heterogeneous systems: hydrated lysozyme results. Magn Reson Med. 1991 Nov;22(1):143–153. doi: 10.1002/mrm.1910220115. [DOI] [PubMed] [Google Scholar]
- Lindstrom T. R., Koenig S. H., Boussios T., Bertles J. F. Intermolecular interactions of oxygenated sickle hemoglobin molecules in cells and cell-free solutions. Biophys J. 1976 Jun;16(6):679–689. doi: 10.1016/S0006-3495(76)85721-9. [DOI] [PMC free article] [PubMed] [Google Scholar]
- Otting G., Liepinsh E., Wüthrich K. Protein hydration in aqueous solution. Science. 1991 Nov 15;254(5034):974–980. doi: 10.1126/science.1948083. [DOI] [PubMed] [Google Scholar]
- Peifer M. Cancer, catenins, and cuticle pattern: a complex connection. Science. 1993 Dec 10;262(5140):1667–1668. doi: 10.1126/science.8259511. [DOI] [PubMed] [Google Scholar]
- Schauer G., Kimmich R., Nusser W. Deuteron field-cycling relaxation spectroscopy and translational water diffusion in protein hydration shells. Biophys J. 1988 Mar;53(3):397–404. doi: 10.1016/S0006-3495(88)83116-3. [DOI] [PMC free article] [PubMed] [Google Scholar]
- Schoenborn B. P. Solvent effect in protein crystals. A neutron diffraction analysis of solvent and ion density. J Mol Biol. 1988 Jun 20;201(4):741–749. doi: 10.1016/0022-2836(88)90470-6. [DOI] [PubMed] [Google Scholar]
- Spiller M., Kasoff S. S., Lansen T. A., Rifkinson-Mann S., Valsamis M. P., Koenig S. H., Tenner M. S. Variation of the magnetic relaxation rate 1/T1 of water protons with magnetic field strength (NMRD profile) of untreated, non-calcified, human astrocytomas: correlation with histology and solids content. J Neurooncol. 1994;21(2):113–125. doi: 10.1007/BF01052895. [DOI] [PubMed] [Google Scholar]
- Winter F., Kimmich R. NMR field-cycling relaxation spectroscopy of bovine serum albumin, muscle tissue, Micrococcus luteus and yeast. 14N1H-quadrupole dips. Biochim Biophys Acta. 1982 Nov 24;719(2):292–298. doi: 10.1016/0304-4165(82)90101-5. [DOI] [PubMed] [Google Scholar]
- Zhou D., Bryant R. G. Magnetization transfer, cross-relaxation, and chemical exchange in rotationally immobilized protein gels. Magn Reson Med. 1994 Dec;32(6):725–732. doi: 10.1002/mrm.1910320607. [DOI] [PubMed] [Google Scholar]
- Zimmerman S. B., Minton A. P. Macromolecular crowding: biochemical, biophysical, and physiological consequences. Annu Rev Biophys Biomol Struct. 1993;22:27–65. doi: 10.1146/annurev.bb.22.060193.000331. [DOI] [PubMed] [Google Scholar]