Abstract
Two ampullary epithelial properties necessary for electroreception were used to identify the types of ion channels and transporters found in apical and basal membranes of ampullary receptor cells of skates and to assess their individual role under voltage-clamp conditions. The two essential properties are (1) a steady-state negative conductance generated in apical membranes and (2) a small, spontaneous current oscillation originating in basal membranes (Lu and Fishman, 1995). The effects of pharmacological agents and ion substitutions on these properties were evaluated from transorgan or transepithelial complex admittance determinations in the frequency range 0.125 to 50 Hz measured in individual, isolated ampullary organs. In apical membranes, L-type Ca channels were found to be responsible for generation of the steady-state negative conductance. In basal membranes, K and Ca-dependent Cl (Cl(Ca)) channels were demonstrated to contribute to a net positive membrane conductance. L-type Ca channels were also evident in basal membranes and are thought to function in synaptic transmission from the electroreceptive epithelium to the primary afferent nerve. In addition to ion channels in basal membranes, two transporters (Na+/K+ pump and Na(+)-Ca+ exchanger) were apparent. Rapid (minutes) cessation of the current oscillation after blockage of any of the basal ion channels (Ca, Cl(Ca), K) suggests critical involvement of each of these channel types in the generation of the oscillation. Suppression of either Na+/K+ transport or Na(+)-Ca2+ exchange also eliminated the oscillation but at a slower rate, indicating an indirect effect.
Full text
PDF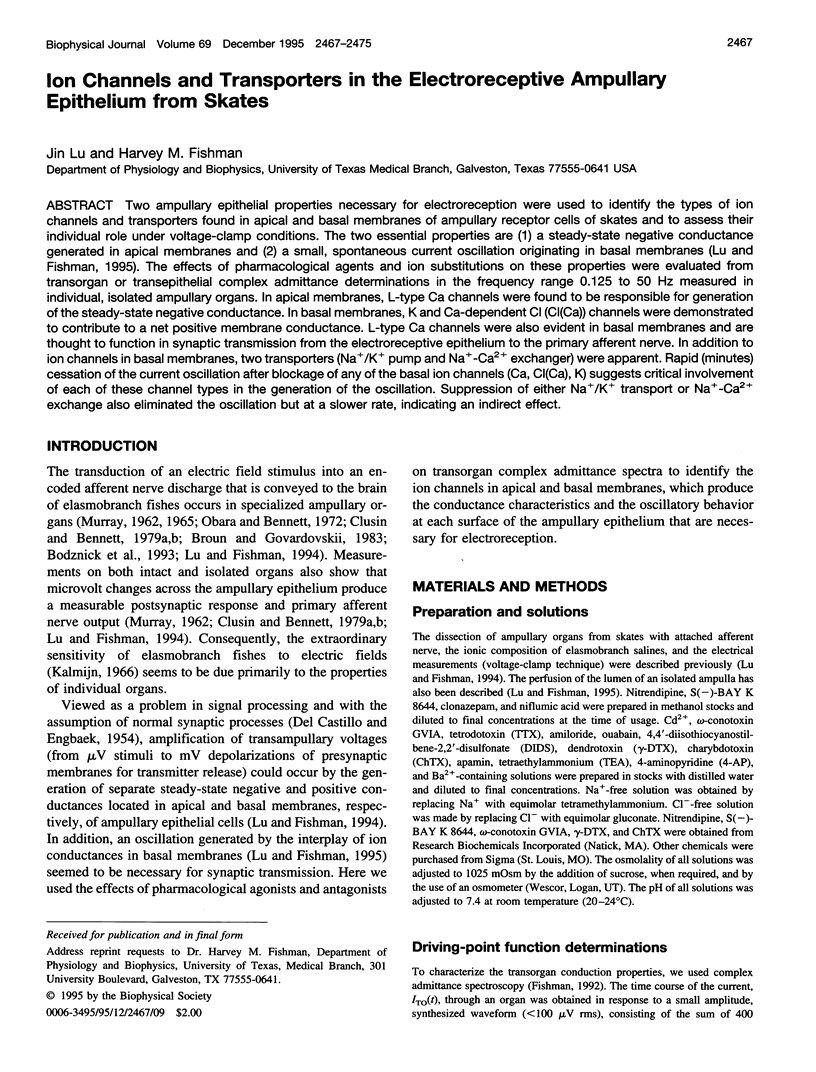
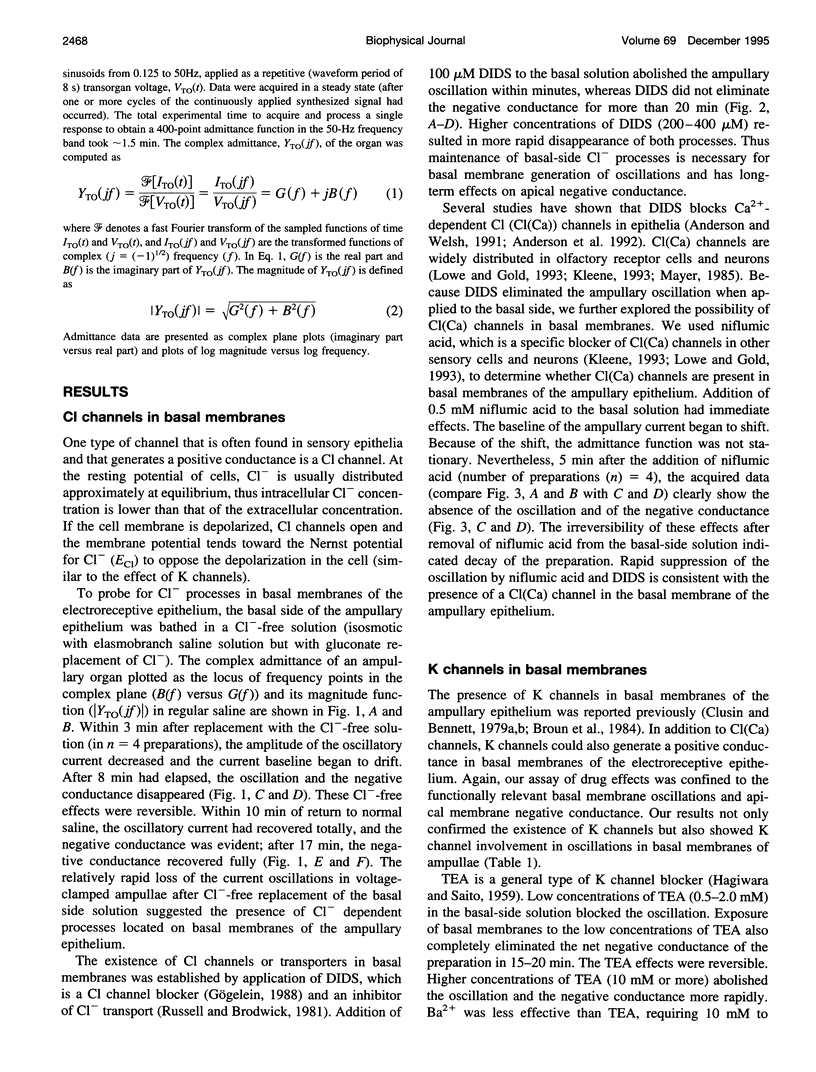
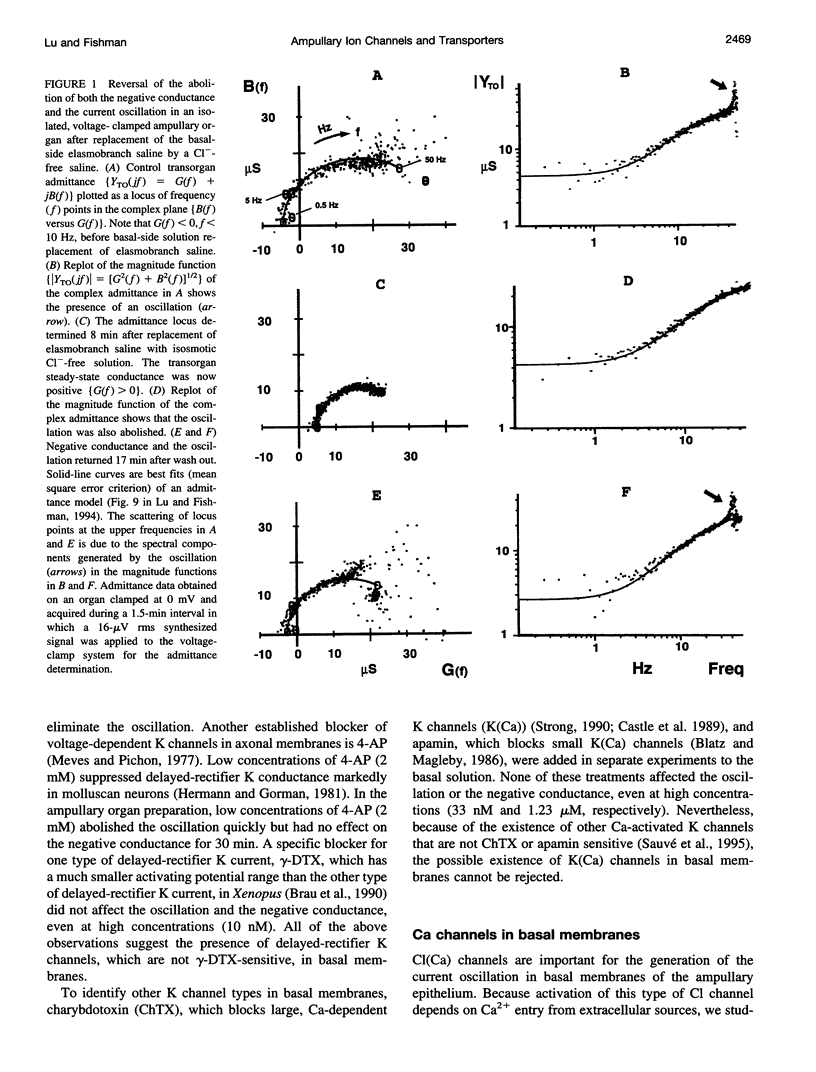
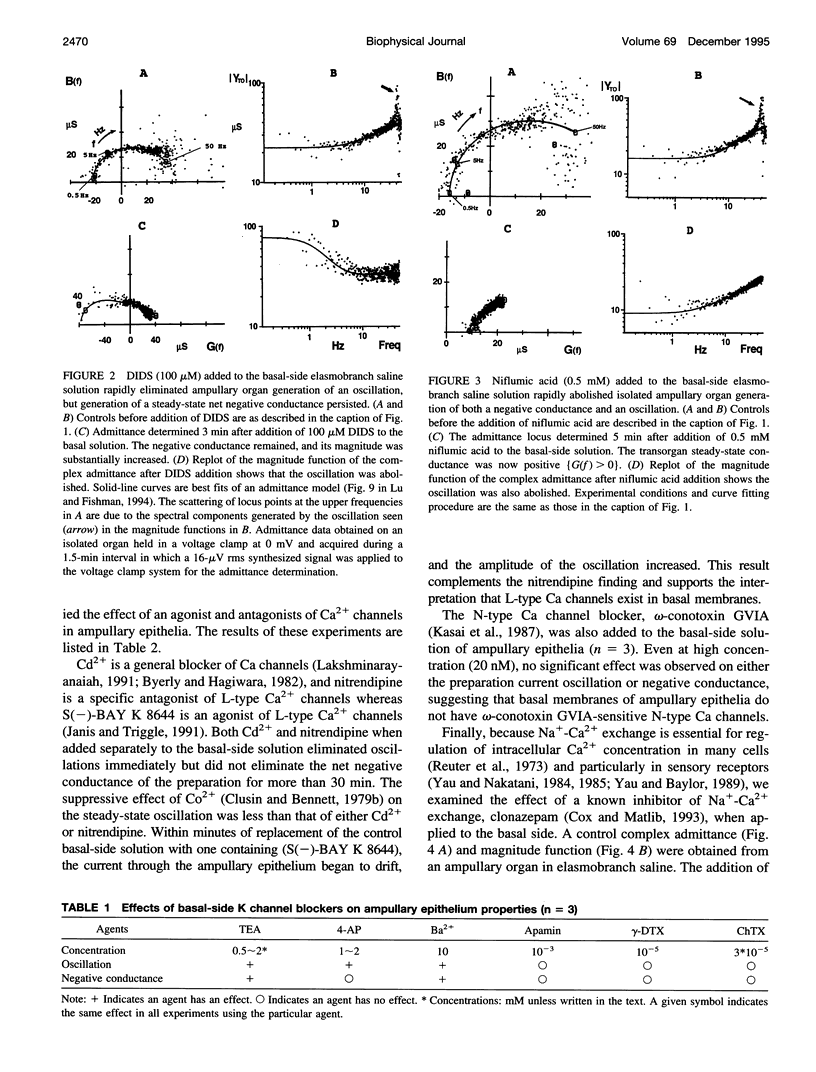
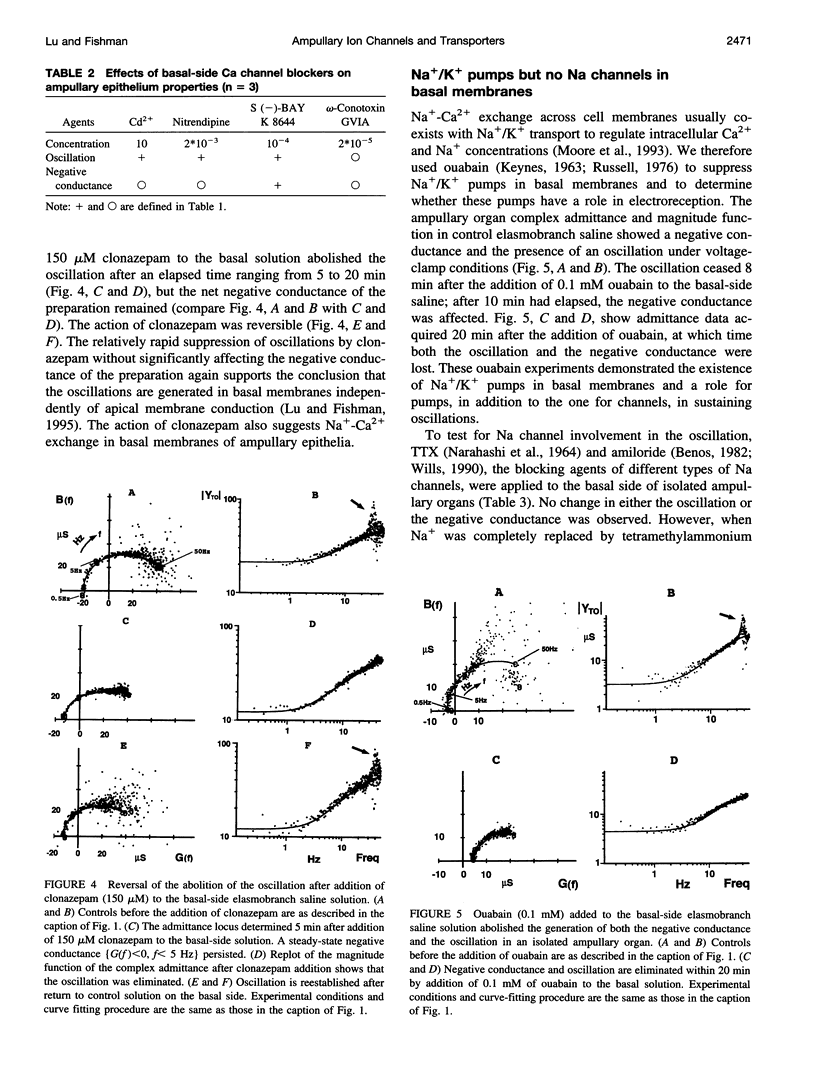
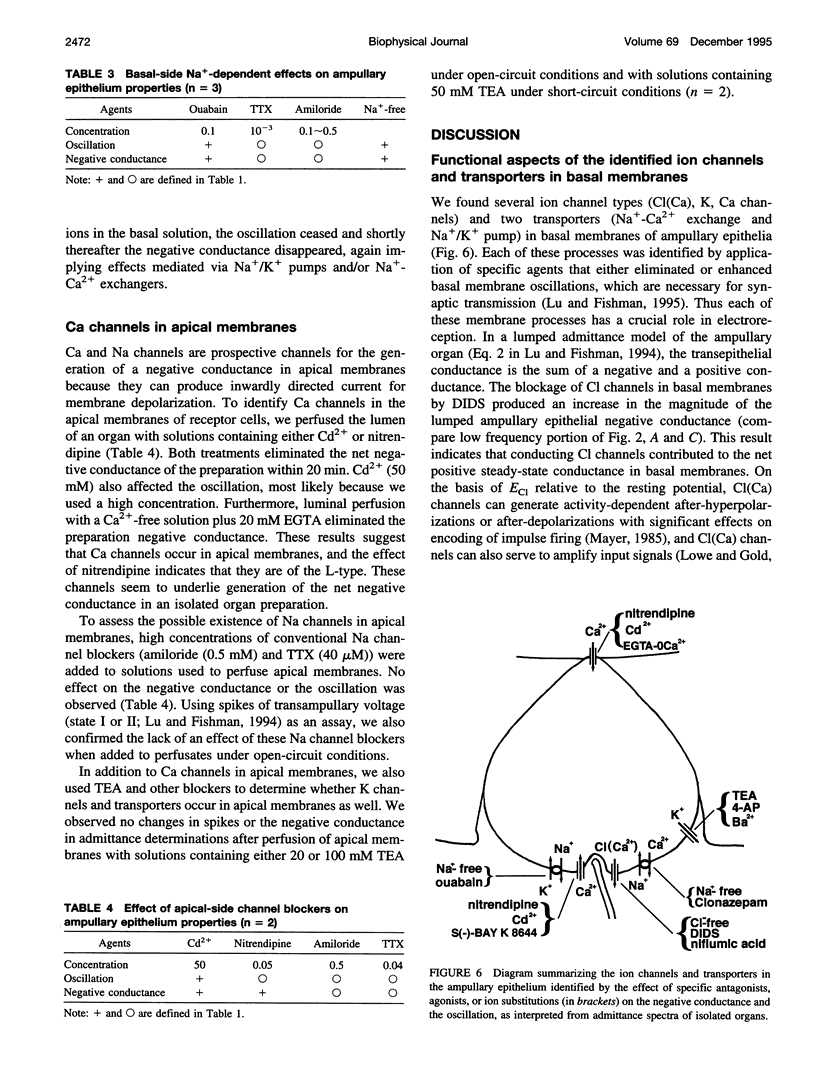
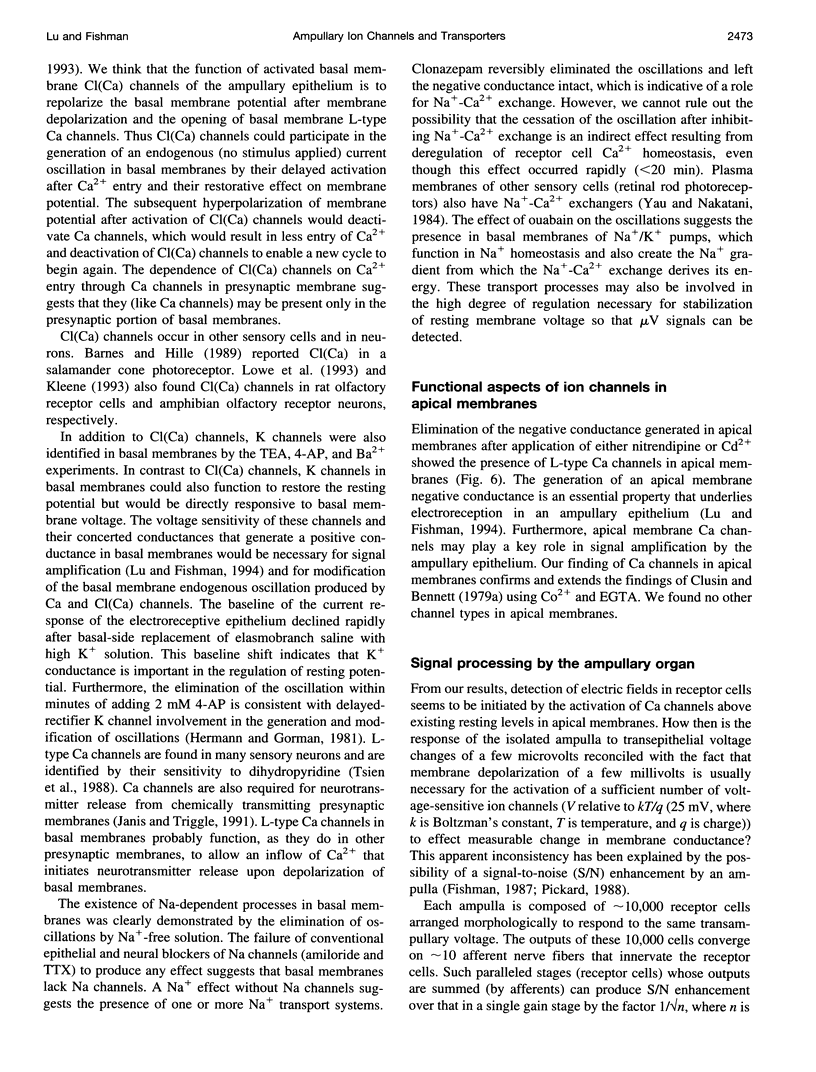
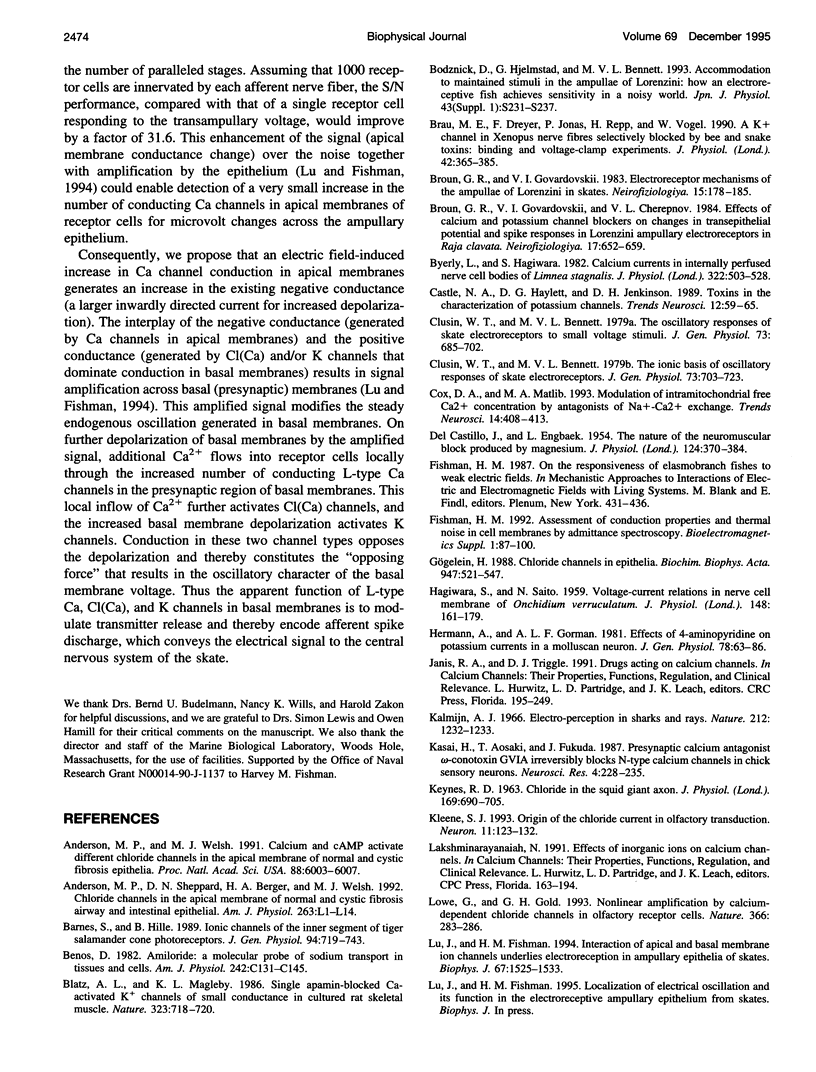
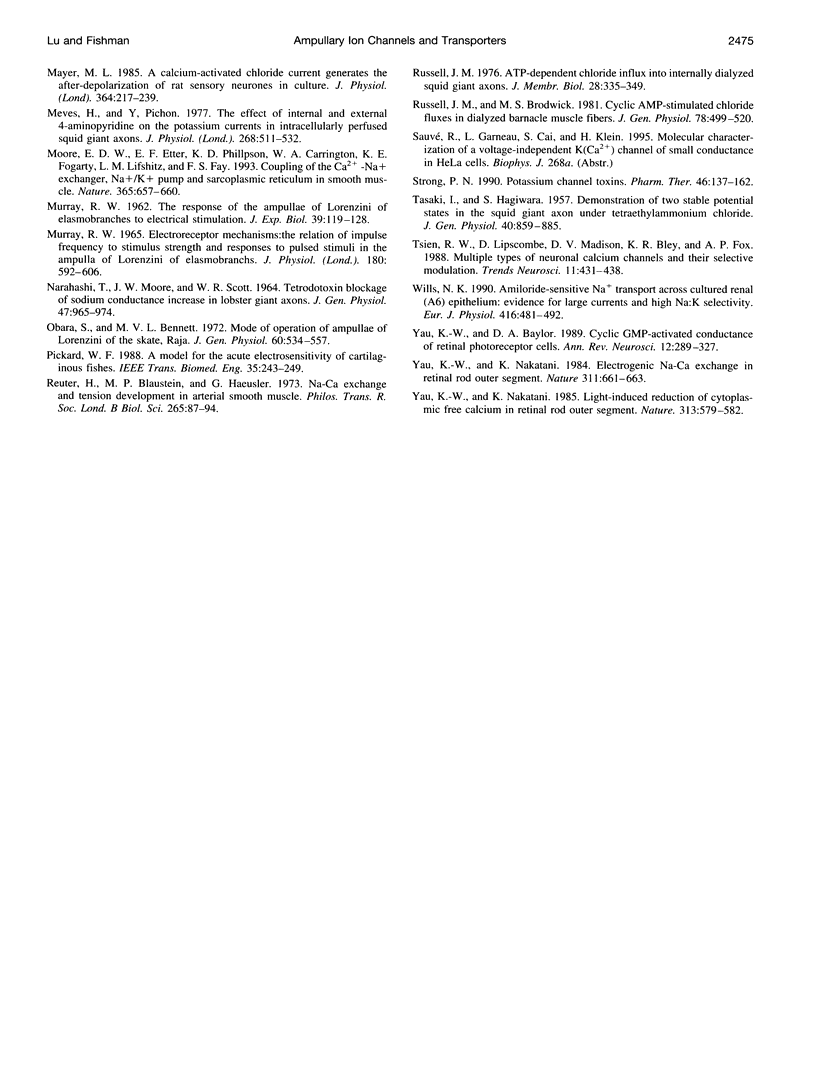
Selected References
These references are in PubMed. This may not be the complete list of references from this article.
- Anderson M. P., Welsh M. J. Calcium and cAMP activate different chloride channels in the apical membrane of normal and cystic fibrosis epithelia. Proc Natl Acad Sci U S A. 1991 Jul 15;88(14):6003–6007. doi: 10.1073/pnas.88.14.6003. [DOI] [PMC free article] [PubMed] [Google Scholar]
- Barnes S., Hille B. Ionic channels of the inner segment of tiger salamander cone photoreceptors. J Gen Physiol. 1989 Oct;94(4):719–743. doi: 10.1085/jgp.94.4.719. [DOI] [PMC free article] [PubMed] [Google Scholar]
- Benos D. J. Amiloride: a molecular probe of sodium transport in tissues and cells. Am J Physiol. 1982 Mar;242(3):C131–C145. doi: 10.1152/ajpcell.1982.242.3.C131. [DOI] [PubMed] [Google Scholar]
- Blatz A. L., Magleby K. L. Single apamin-blocked Ca-activated K+ channels of small conductance in cultured rat skeletal muscle. Nature. 1986 Oct 23;323(6090):718–720. doi: 10.1038/323718a0. [DOI] [PubMed] [Google Scholar]
- Bodznick D., Hjelmstad G., Bennett M. V. Accommodation to maintained stimuli in the ampullae of Lorenzini: how an electroreceptive fish achieves sensitivity in a noisy world. Jpn J Physiol. 1993;43 (Suppl 1):S231–S237. [PubMed] [Google Scholar]
- Broun G. R., Govardovskii V. I., Cherepnov V. L. Vliianie blokatorov kalievykh o kal'tsievykh kanalov na izmeneniia transépitelial'nogo potentsiala i impul'snye otvety élektroretseptorov ampul Lorentsini skata. Neirofiziologiia. 1985;17(5):652–660. [PubMed] [Google Scholar]
- Broun G. R., Govardovskii V. I. Issledovanie retseptornykh mekhanizmov élektroretseptorov ampul Lorentsini skata. Neirofiziologiia. 1983;15(2):178–185. [PubMed] [Google Scholar]
- Bräu M. E., Dreyer F., Jonas P., Repp H., Vogel W. A K+ channel in Xenopus nerve fibres selectively blocked by bee and snake toxins: binding and voltage-clamp experiments. J Physiol. 1990 Jan;420:365–385. doi: 10.1113/jphysiol.1990.sp017918. [DOI] [PMC free article] [PubMed] [Google Scholar]
- Byerly L., Hagiwara S. Calcium currents in internally perfused nerve cell bodies of Limnea stagnalis. J Physiol. 1982 Jan;322:503–528. doi: 10.1113/jphysiol.1982.sp014052. [DOI] [PMC free article] [PubMed] [Google Scholar]
- Castle N. A., Haylett D. G., Jenkinson D. H. Toxins in the characterization of potassium channels. Trends Neurosci. 1989 Feb;12(2):59–65. doi: 10.1016/0166-2236(89)90137-9. [DOI] [PubMed] [Google Scholar]
- Clusin W. T., Bennett M. V. The ionic basis of oscillatory responses of skate electroreceptors. J Gen Physiol. 1979 Jun;73(6):703–723. doi: 10.1085/jgp.73.6.703. [DOI] [PMC free article] [PubMed] [Google Scholar]
- Clusin W. T., Bennett M. V. The oscillatory responses of skate electroreceptors to small voltage stimuli. J Gen Physiol. 1979 Jun;73(6):685–702. doi: 10.1085/jgp.73.6.685. [DOI] [PMC free article] [PubMed] [Google Scholar]
- Cox D. A., Matlib M. A. Modulation of intramitochondrial free Ca2+ concentration by antagonists of Na(+)-Ca2+ exchange. Trends Pharmacol Sci. 1993 Nov;14(11):408–413. doi: 10.1016/0165-6147(93)90063-P. [DOI] [PubMed] [Google Scholar]
- DEL CASTILLO J., ENGBAEK L. The nature of the neuromuscular block produced by magnesium. J Physiol. 1954 May 28;124(2):370–384. doi: 10.1113/jphysiol.1954.sp005114. [DOI] [PMC free article] [PubMed] [Google Scholar]
- Fishman H. M. Assessment of conduction properties and thermal noise in cell membranes by admittance spectroscopy. Bioelectromagnetics. 1992;Suppl 1:87–100. doi: 10.1002/bem.2250130709. [DOI] [PubMed] [Google Scholar]
- Gögelein H. Chloride channels in epithelia. Biochim Biophys Acta. 1988 Oct 11;947(3):521–547. doi: 10.1016/0304-4157(88)90006-8. [DOI] [PubMed] [Google Scholar]
- HAGIWARA S., SAITO N. Voltage-current relations in nerve cell membrane of Onchidium verruculatum. J Physiol. 1959 Oct;148:161–179. doi: 10.1113/jphysiol.1959.sp006279. [DOI] [PMC free article] [PubMed] [Google Scholar]
- Hermann A., Gorman A. L. Effects of 4-aminopyridine on potassium currents in a molluscan neuron. J Gen Physiol. 1981 Jul;78(1):63–86. doi: 10.1085/jgp.78.1.63. [DOI] [PMC free article] [PubMed] [Google Scholar]
- KEYNES R. D. CHLORIDE IN THE SQUID GIANT AXON. J Physiol. 1963 Dec;169:690–705. doi: 10.1113/jphysiol.1963.sp007289. [DOI] [PMC free article] [PubMed] [Google Scholar]
- Kasai H., Aosaki T., Fukuda J. Presynaptic Ca-antagonist omega-conotoxin irreversibly blocks N-type Ca-channels in chick sensory neurons. Neurosci Res. 1987 Feb;4(3):228–235. doi: 10.1016/0168-0102(87)90014-9. [DOI] [PubMed] [Google Scholar]
- Kleene S. J. Origin of the chloride current in olfactory transduction. Neuron. 1993 Jul;11(1):123–132. doi: 10.1016/0896-6273(93)90276-w. [DOI] [PubMed] [Google Scholar]
- Lowe G., Gold G. H. Nonlinear amplification by calcium-dependent chloride channels in olfactory receptor cells. Nature. 1993 Nov 18;366(6452):283–286. doi: 10.1038/366283a0. [DOI] [PubMed] [Google Scholar]
- Lu J., Fishman H. M. Interaction of apical and basal membrane ion channels underlies electroreception in ampullary epithelia of skates. Biophys J. 1994 Oct;67(4):1525–1533. doi: 10.1016/S0006-3495(94)80626-5. [DOI] [PMC free article] [PubMed] [Google Scholar]
- MURRAY R. W. The response of the ampullae of Lorenzini of elasmobranchs to electrical stimulation. J Exp Biol. 1962 Mar;39:119–128. doi: 10.1242/jeb.39.1.119. [DOI] [PubMed] [Google Scholar]
- Mayer M. L. A calcium-activated chloride current generates the after-depolarization of rat sensory neurones in culture. J Physiol. 1985 Jul;364:217–239. doi: 10.1113/jphysiol.1985.sp015740. [DOI] [PMC free article] [PubMed] [Google Scholar]
- Meves H., Pichon Y. The effect of internal and external 4-aminopyridine on the potassium currents in intracellularly perfused squid giant axons. J Physiol. 1977 Jun;268(2):511–532. doi: 10.1113/jphysiol.1977.sp011869. [DOI] [PMC free article] [PubMed] [Google Scholar]
- Moore E. D., Etter E. F., Philipson K. D., Carrington W. A., Fogarty K. E., Lifshitz L. M., Fay F. S. Coupling of the Na+/Ca2+ exchanger, Na+/K+ pump and sarcoplasmic reticulum in smooth muscle. Nature. 1993 Oct 14;365(6447):657–660. doi: 10.1038/365657a0. [DOI] [PubMed] [Google Scholar]
- Murray R. W. Electroreceptor mechanisms: the relation of impulse frequency to stimulus strength and responses to pulsed stimuli in the ampullae of Lorenzini of elasmobranchs. J Physiol. 1965 Oct;180(3):592–606. doi: 10.1113/jphysiol.1965.sp007719. [DOI] [PMC free article] [PubMed] [Google Scholar]
- NARAHASHI T., MOORE J. W., SCOTT W. R. TETRODOTOXIN BLOCKAGE OF SODIUM CONDUCTANCE INCREASE IN LOBSTER GIANT AXONS. J Gen Physiol. 1964 May;47:965–974. doi: 10.1085/jgp.47.5.965. [DOI] [PMC free article] [PubMed] [Google Scholar]
- Obara S., Bennett M. V. Mode of operation of ampullae of Lorenzini of the skate, Raja. J Gen Physiol. 1972 Nov;60(5):534–557. doi: 10.1085/jgp.60.5.534. [DOI] [PMC free article] [PubMed] [Google Scholar]
- Pickard W. F. A model for the acute electrosensitivity of cartilaginous fishes. IEEE Trans Biomed Eng. 1988 Apr;35(4):243–249. doi: 10.1109/10.1372. [DOI] [PubMed] [Google Scholar]
- Reuter H., Blaustein M. P., Haeusler G. Na-Ca exchange and tension development in arterial smooth muscle. Philos Trans R Soc Lond B Biol Sci. 1973 Mar 15;265(867):87–94. doi: 10.1098/rstb.1973.0011. [DOI] [PubMed] [Google Scholar]
- Russell J. M. ATP-Dependent chloride influx into internally dialyzed squid giant axons. J Membr Biol. 1976 Sep 17;28(4):335–349. doi: 10.1007/BF01869704. [DOI] [PubMed] [Google Scholar]
- Russell J. M., Brodwick M. S. Cyclic AMP-stimulated chloride fluxes in dialyzed barnacle muscle fibers. J Gen Physiol. 1981 Nov;78(5):499–520. doi: 10.1085/jgp.78.5.499. [DOI] [PMC free article] [PubMed] [Google Scholar]
- Strong P. N. Potassium channel toxins. Pharmacol Ther. 1990;46(1):137–162. doi: 10.1016/0163-7258(90)90040-9. [DOI] [PubMed] [Google Scholar]
- TASAKI I., HAGIWAR A. S. Demonstration of two stable potential states in the squid giant axon under tetraethylammonium chloride. J Gen Physiol. 1957 Jul 20;40(6):859–885. doi: 10.1085/jgp.40.6.859. [DOI] [PMC free article] [PubMed] [Google Scholar]
- Tsien R. W., Lipscombe D., Madison D. V., Bley K. R., Fox A. P. Multiple types of neuronal calcium channels and their selective modulation. Trends Neurosci. 1988 Oct;11(10):431–438. doi: 10.1016/0166-2236(88)90194-4. [DOI] [PubMed] [Google Scholar]
- Wills N. K., Millinoff L. P. Amiloride-sensitive Na+ transport across cultured renal (A6) epithelium: evidence for large currents and high Na:K selectivity. Pflugers Arch. 1990 Jul;416(5):481–492. doi: 10.1007/BF00382680. [DOI] [PubMed] [Google Scholar]
- Yau K. W., Baylor D. A. Cyclic GMP-activated conductance of retinal photoreceptor cells. Annu Rev Neurosci. 1989;12:289–327. doi: 10.1146/annurev.ne.12.030189.001445. [DOI] [PubMed] [Google Scholar]
- Yau K. W., Nakatani K. Electrogenic Na-Ca exchange in retinal rod outer segment. Nature. 1984 Oct 18;311(5987):661–663. doi: 10.1038/311661a0. [DOI] [PubMed] [Google Scholar]
- Yau K. W., Nakatani K. Light-induced reduction of cytoplasmic free calcium in retinal rod outer segment. Nature. 1985 Feb 14;313(6003):579–582. doi: 10.1038/313579a0. [DOI] [PubMed] [Google Scholar]